Abstract
1. Intracellular Ca2+ transients were recorded from frog twitch muscle fibres, using arsenazo III as a Ca2+ monitor. When fibres were stimulated by two action potentials, the arsenazo signal to the second stimulus was smaller than the first, for stimulus intervals of up to several seconds.
2. The recovery of the amplitude of the second response followed two exponential time courses; a fast one with a time constant of about 70 msec giving recovery to about 90% of the control value, followed by a slow recovery to 100%, with a time constant of about 12 sec (at 10 °C).
3. The time constant of the fast recovery component was strongly temperature-dependent, with a Q10 of approximately 2·7, whilst the Q10 of the slow component was about 1·4.
4. Removal of Ca2+ in the bathing medium lengthened the time constant of the slow recovery component by a factor of three, but had little effect on the fast recovery component. The lengthening of the slow component was not reversed by addition of Mg2+, but Sr2+ ions could substitute for Ca2+.
5. The influence of membrane potential on the recovery time-course was investigated after blocking action potentials with tetrodotoxin, using a voltage clamp to control membrane potential. Paired depolarizing stimuli were used, with the potential held to either low (-60 or -80 mV) or high (-110 or -140 mV) potentials between stimuli. No differences were apparent in either the fast or slow recovery components at these holding potentials.
6. The arsenazo response elicited by an action potential following a conditioning tetanus was reduced in size even more strongly than following a single action potential. The time course of recovery of the response following a tetanus again comprised two exponential components. After a 20 Hz tetanus for 0·5 sec, the fast component had a time constant of about 400 msec, and gave a recovery to about 60% of the control value. Subsequent recovery to 100% occurred with a time constant of about 12 sec.
7. The time constant of the fast recovery component increased markedly with increasing frequency or duration of the conditioning tetanus. The time constant of the slow component was not appreciably altered by conditioning tetani varying between one impulse and sixty impulses. However, the reduction in response size due to the slow component, extrapolated to zero stimulus interval, increased with increasing number of impulses in the tetanus.
8. The time constant of the fast recovery component corresponded closely with the decay time constant of the arsenazo response to the conditioning stimulus. This correspondence held over a nearly fifty-fold range of time constants, and for two different conditions which affected the decay time constant (temperature, and frequency of tetanic stimulation).
9. The decay time constant of the arsenazo response elicited by an action potential was slowed by a preceding impulse or tetanus. Following a 20 Hz tetanus for 0·5 sec, recovery of the half decay time appeared to follow an exponential time course, with a time constant of about 12 sec.
10. These results suggest that the fast recovery component reflects the re-filling of release stores in the sarcoplasmic reticulum by Ca2+ ions taken up from the cytoplasm. The origin of the slow component is less clear, but it may arise from inactivation of the excitation—contraction (e—c) coupling process between T-tubule depolarization and Ca2+ release from the sarcoplasmic reticulum.
Full text
PDF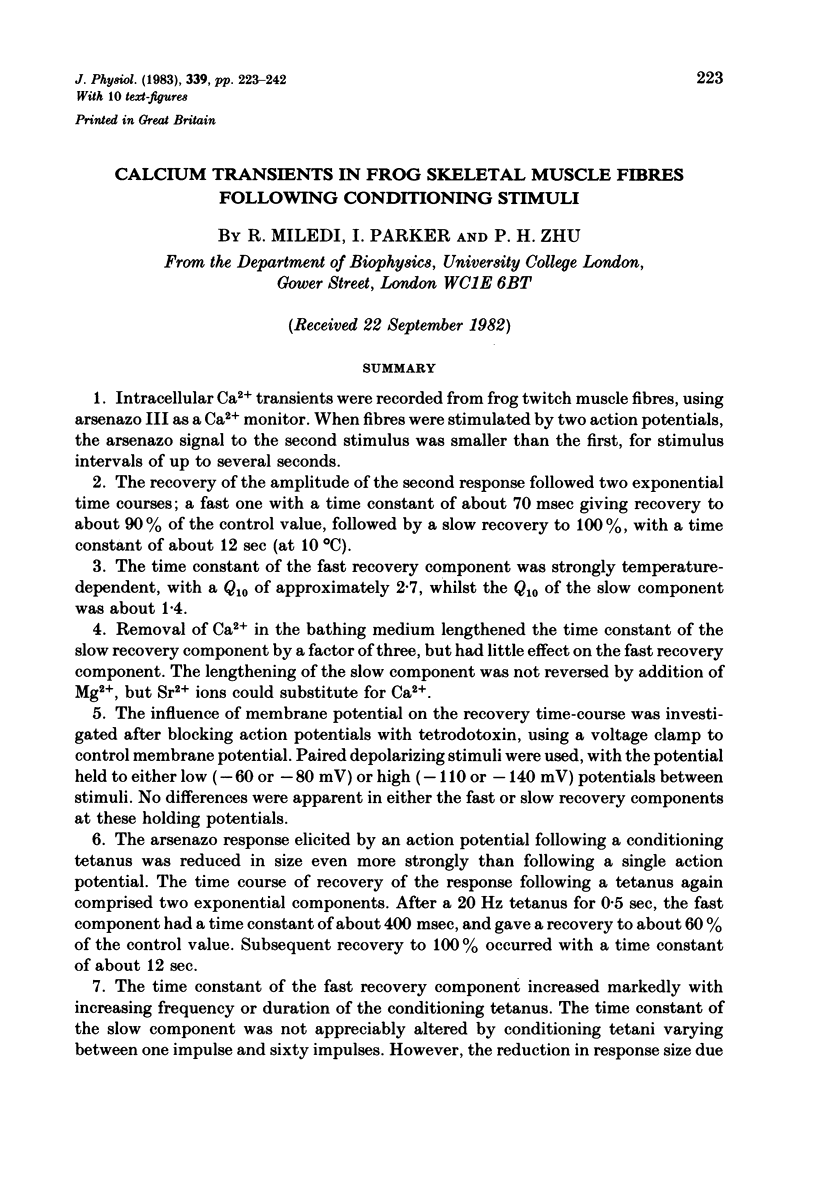
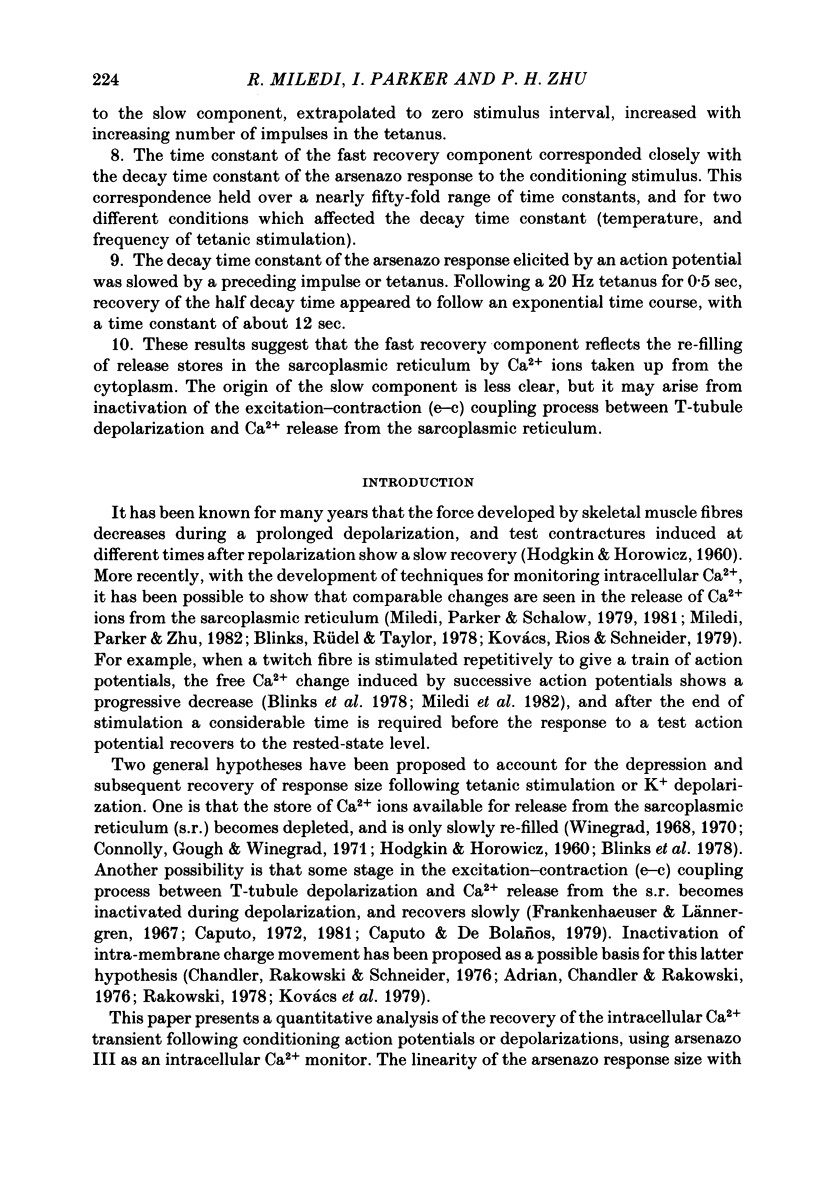
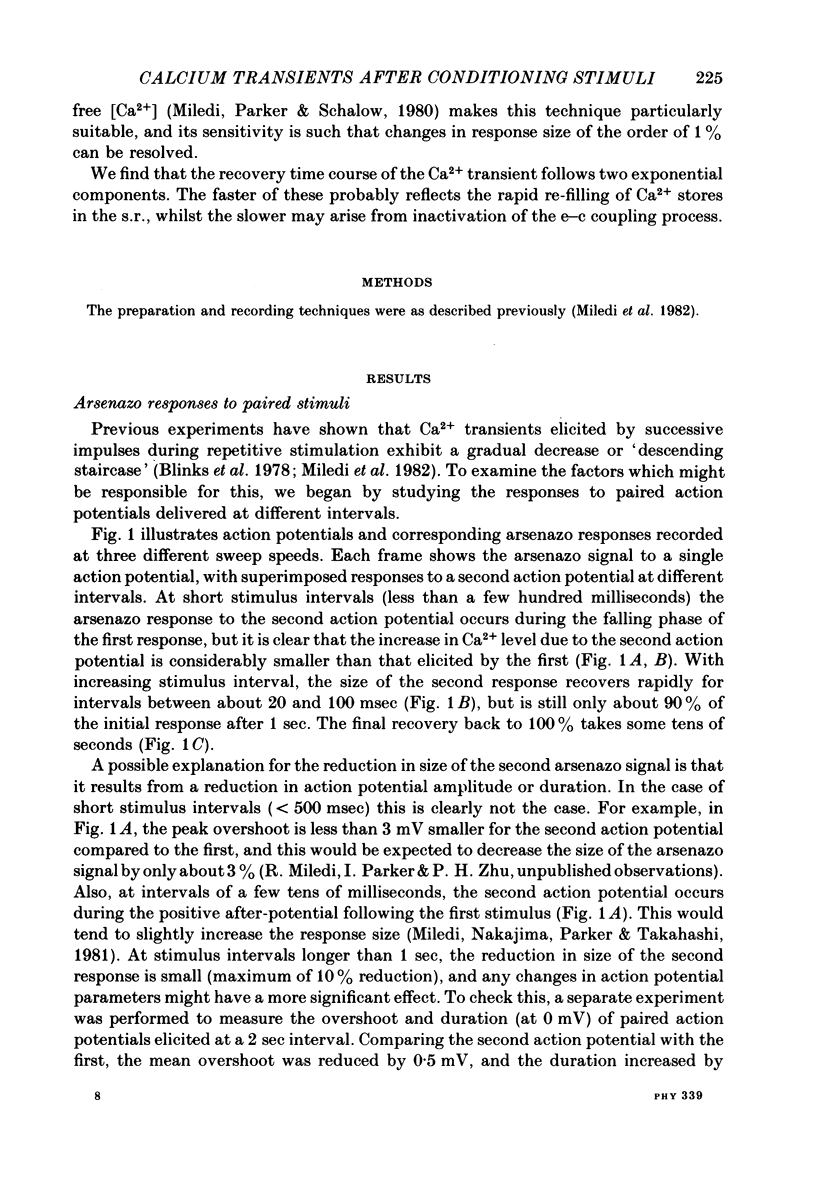
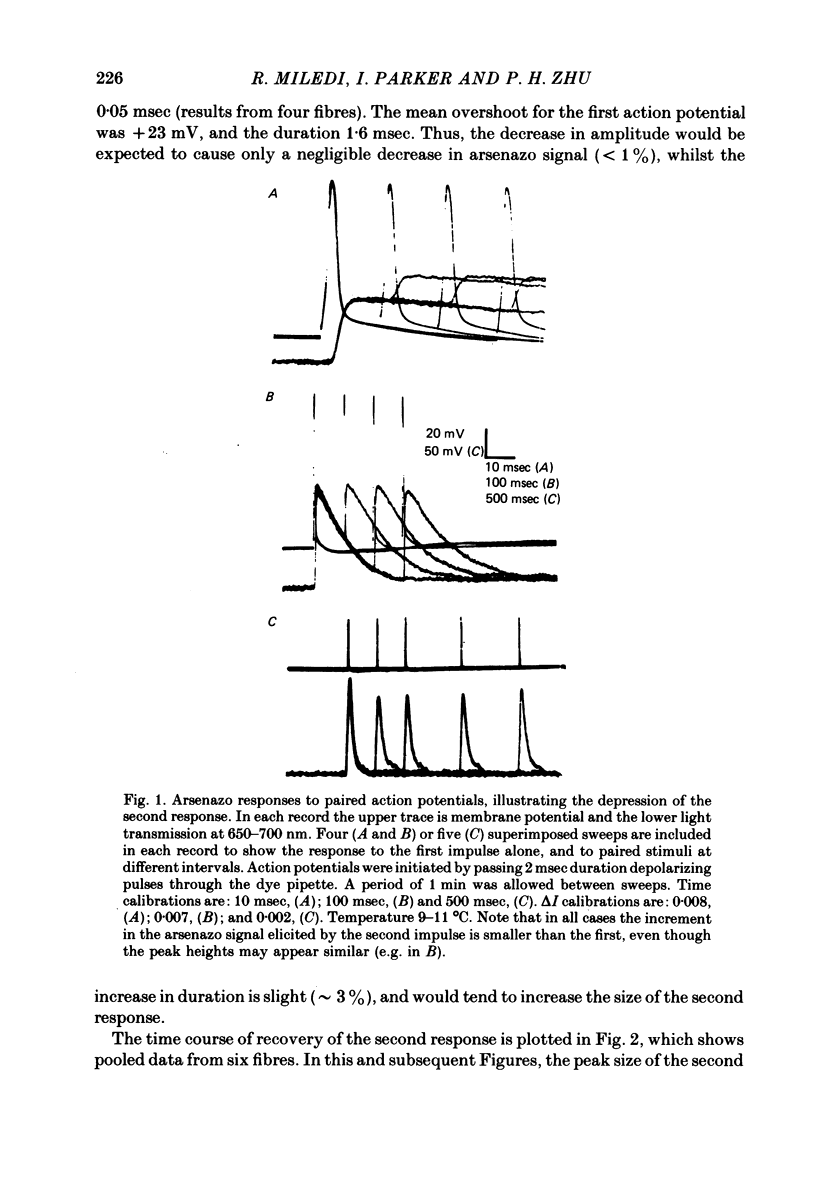
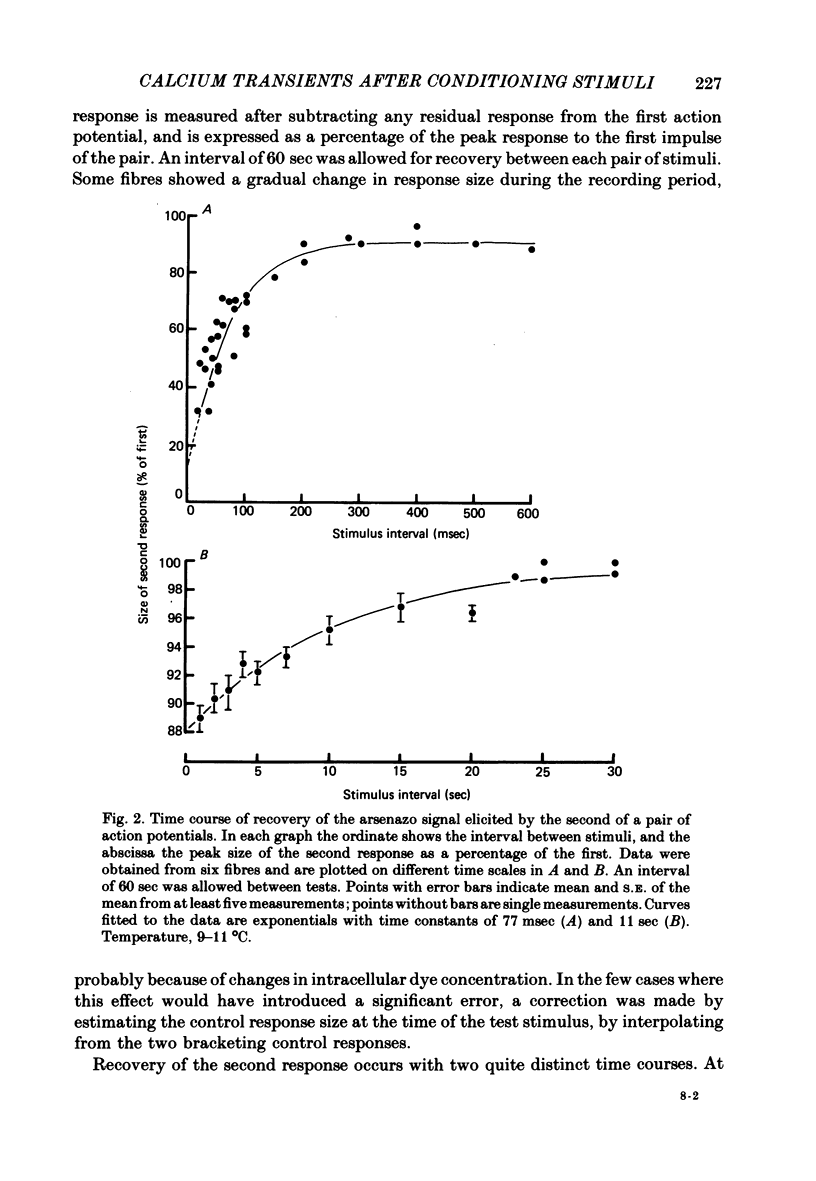
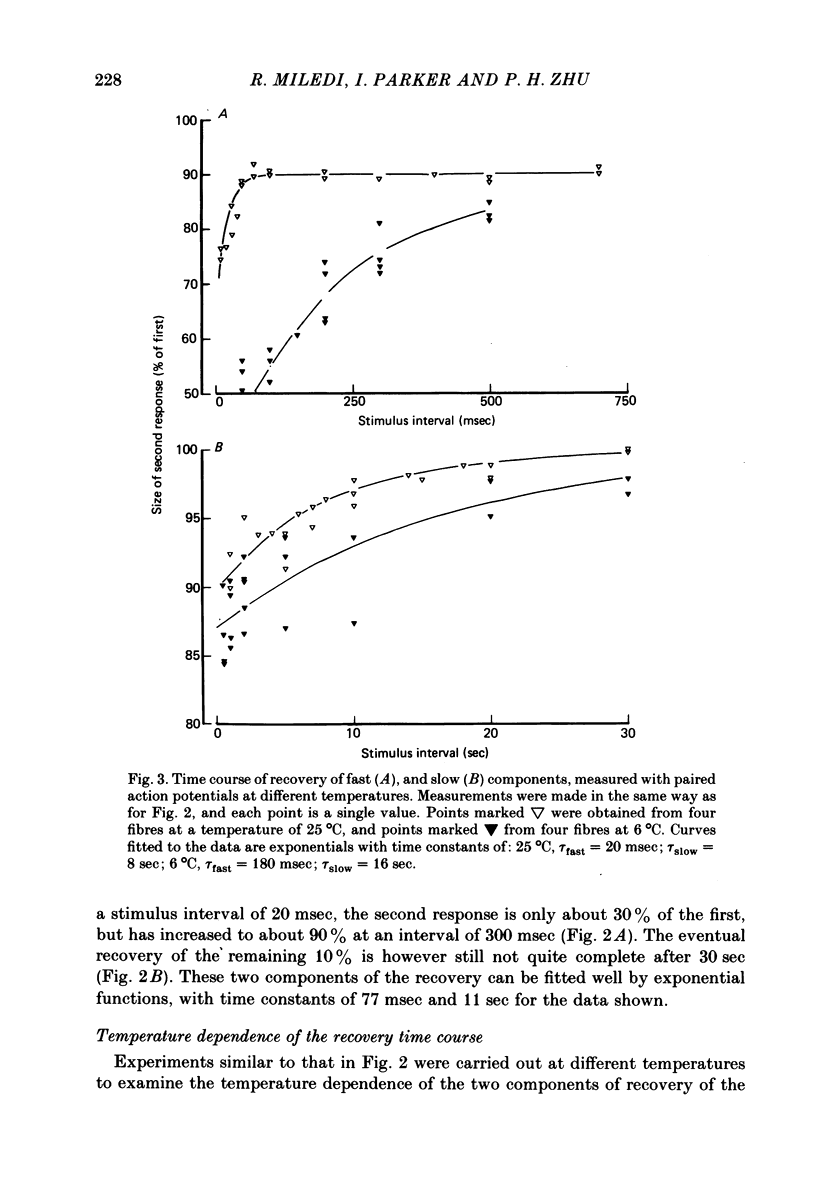
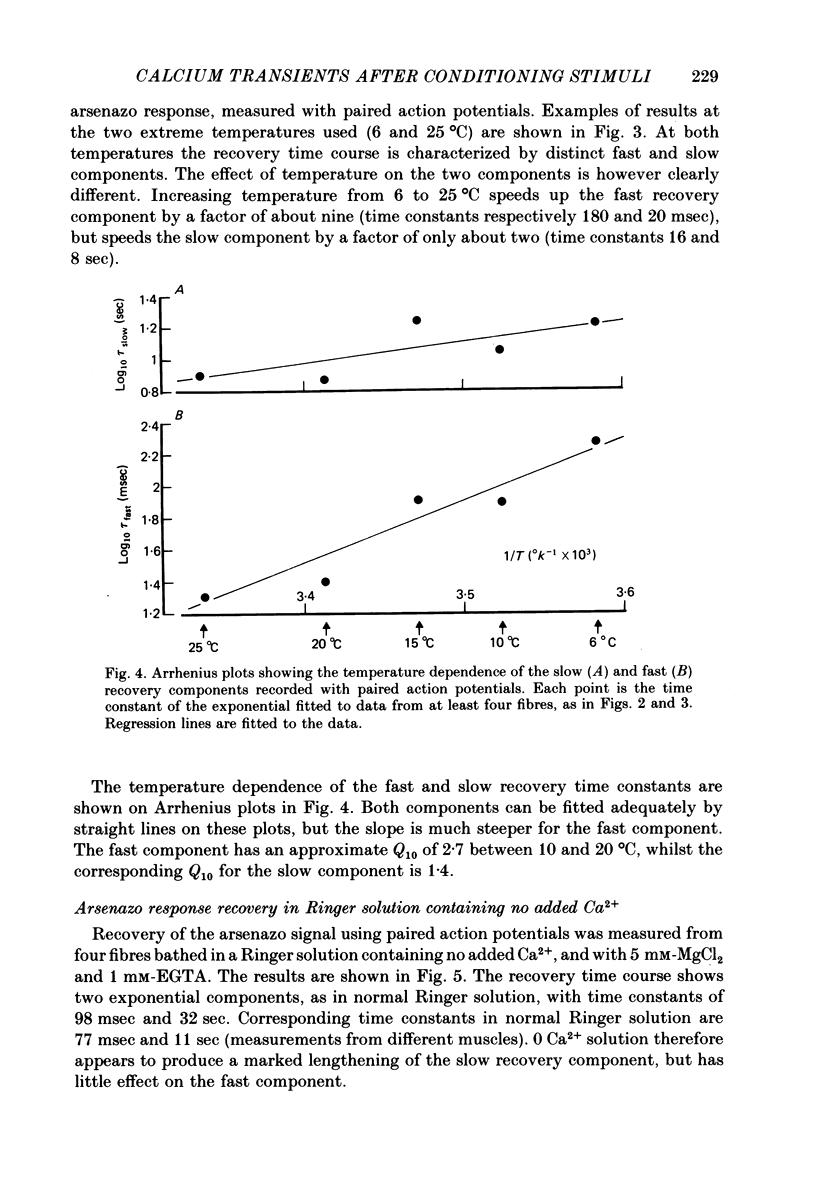
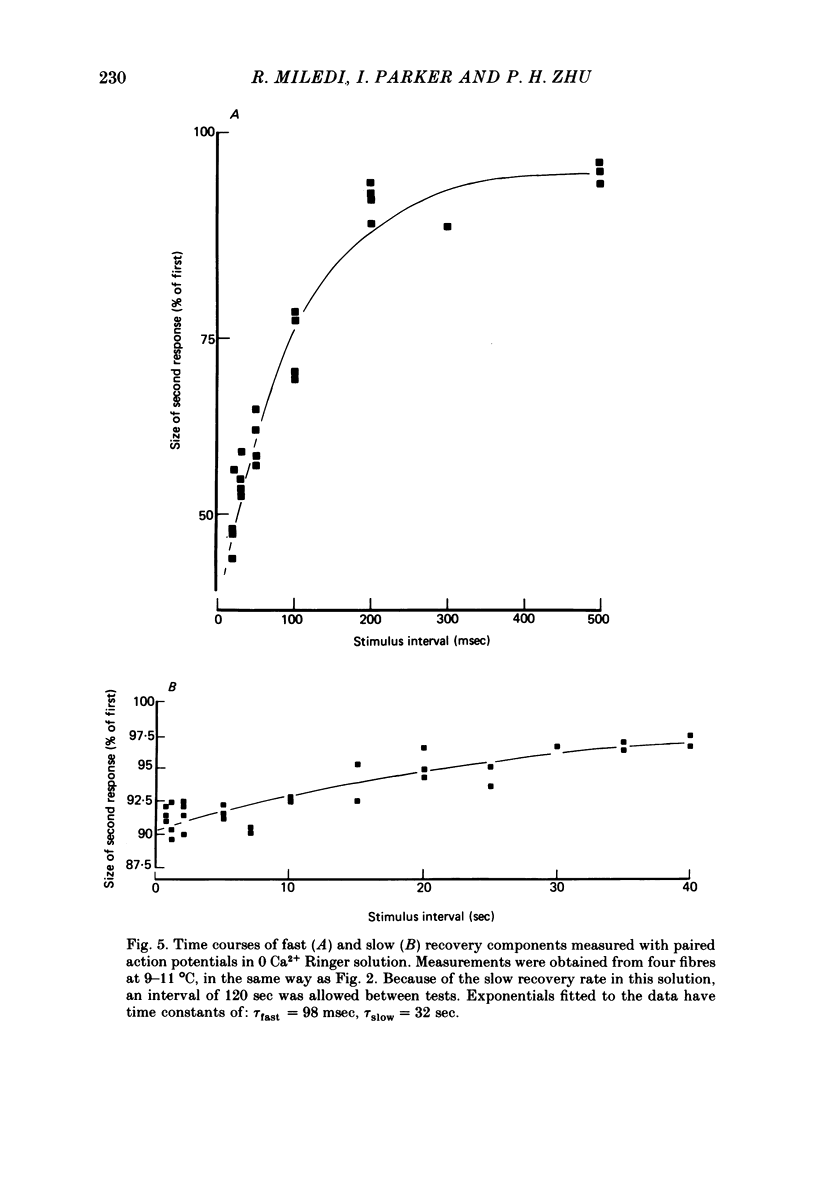
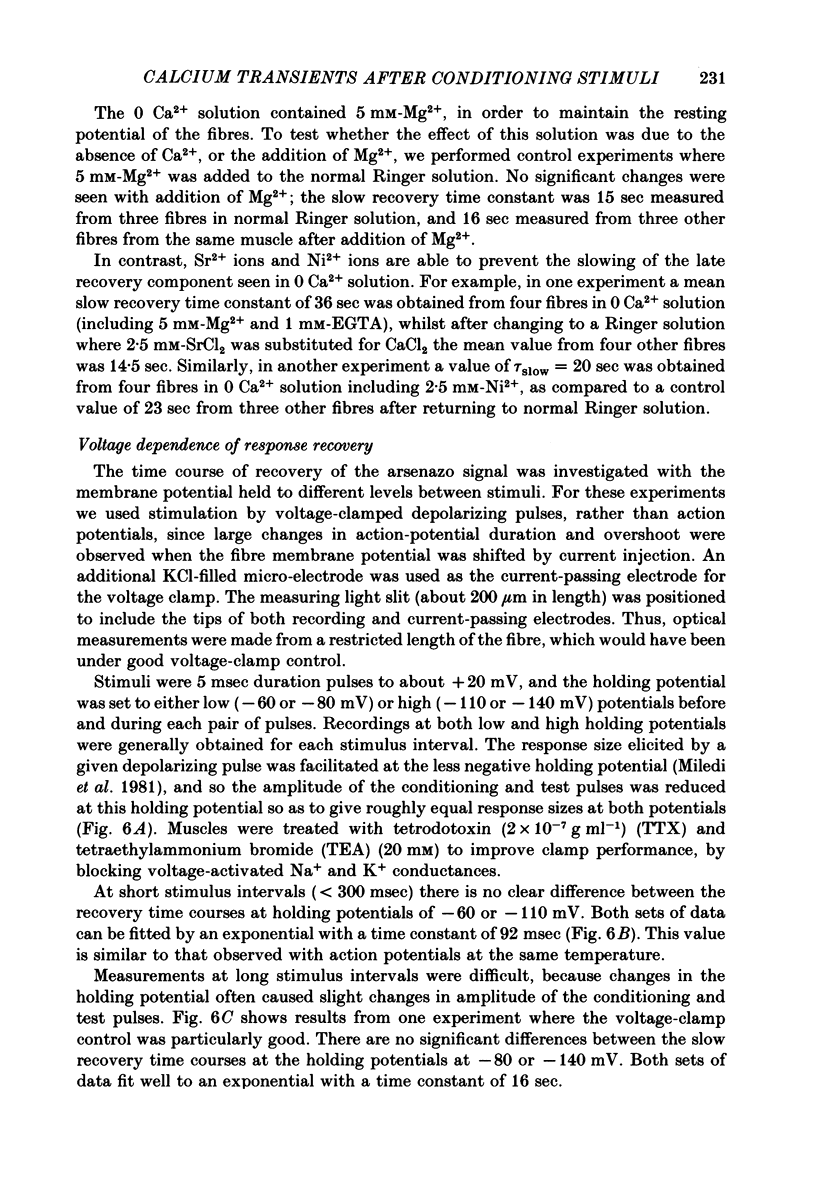
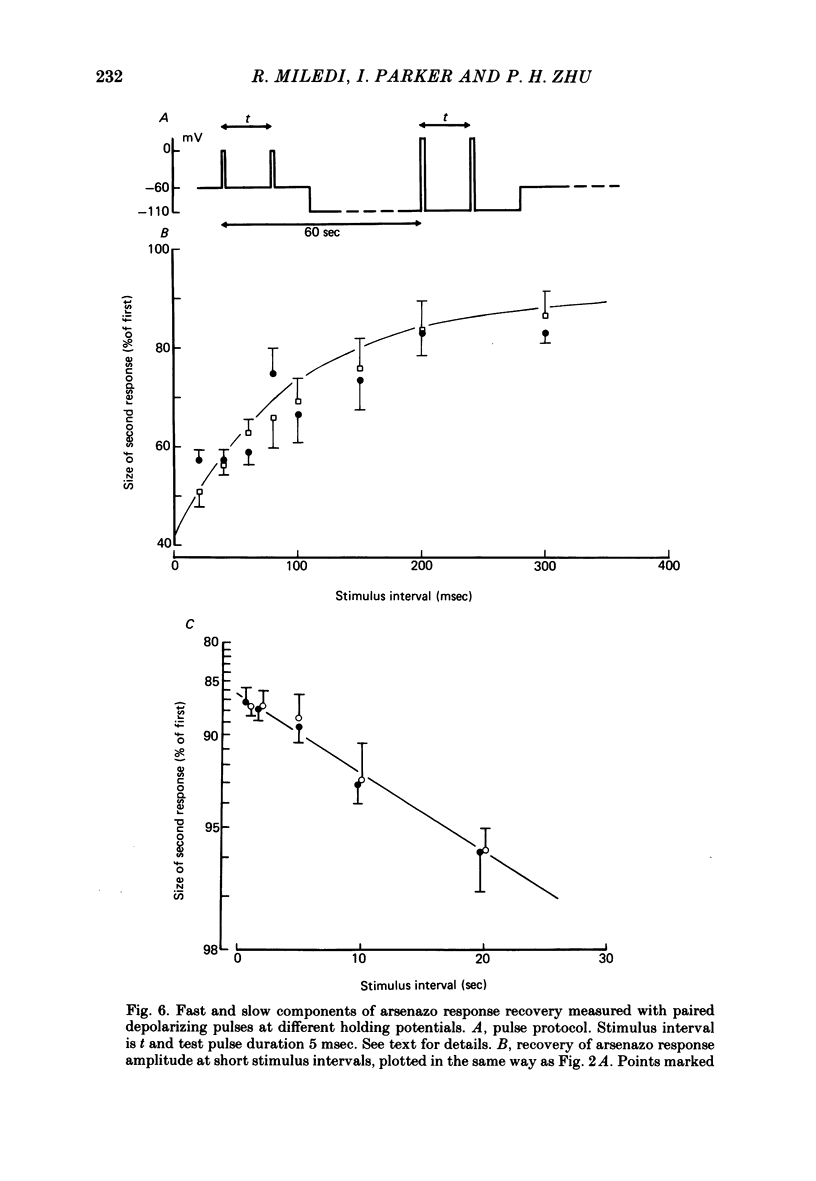
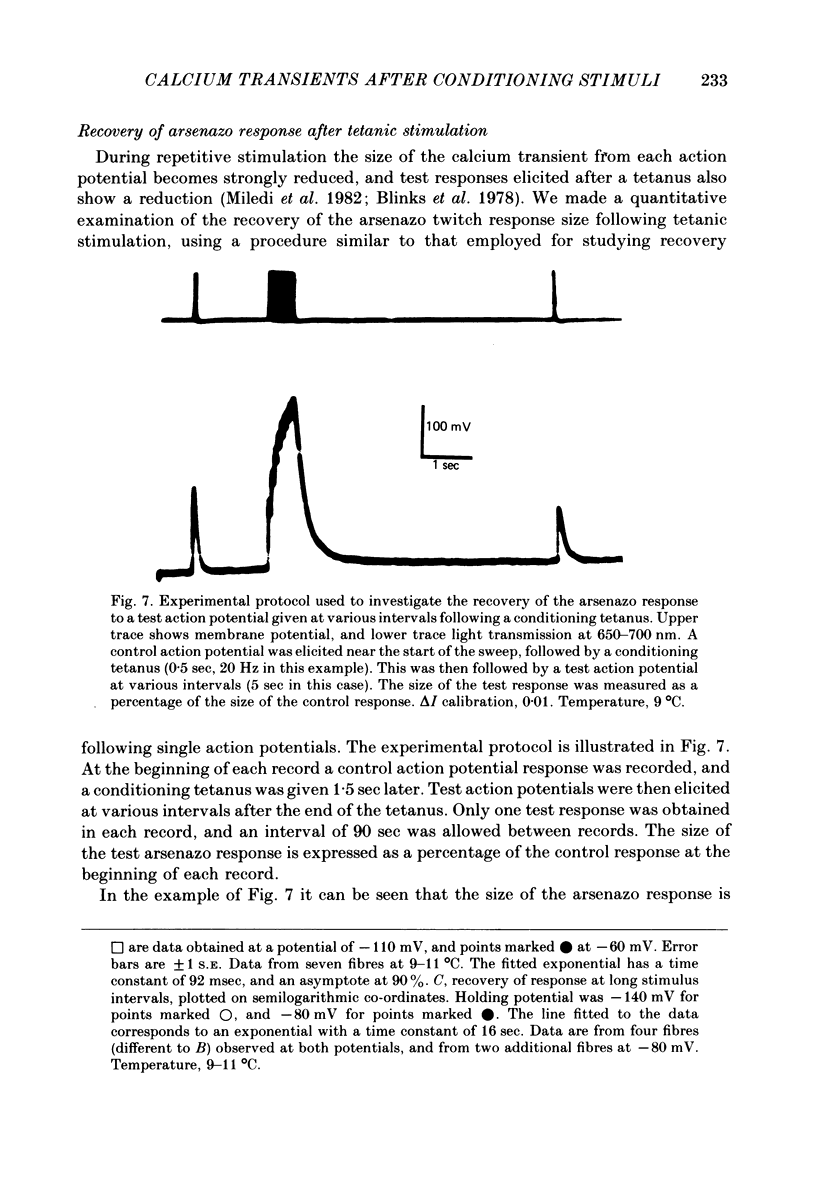
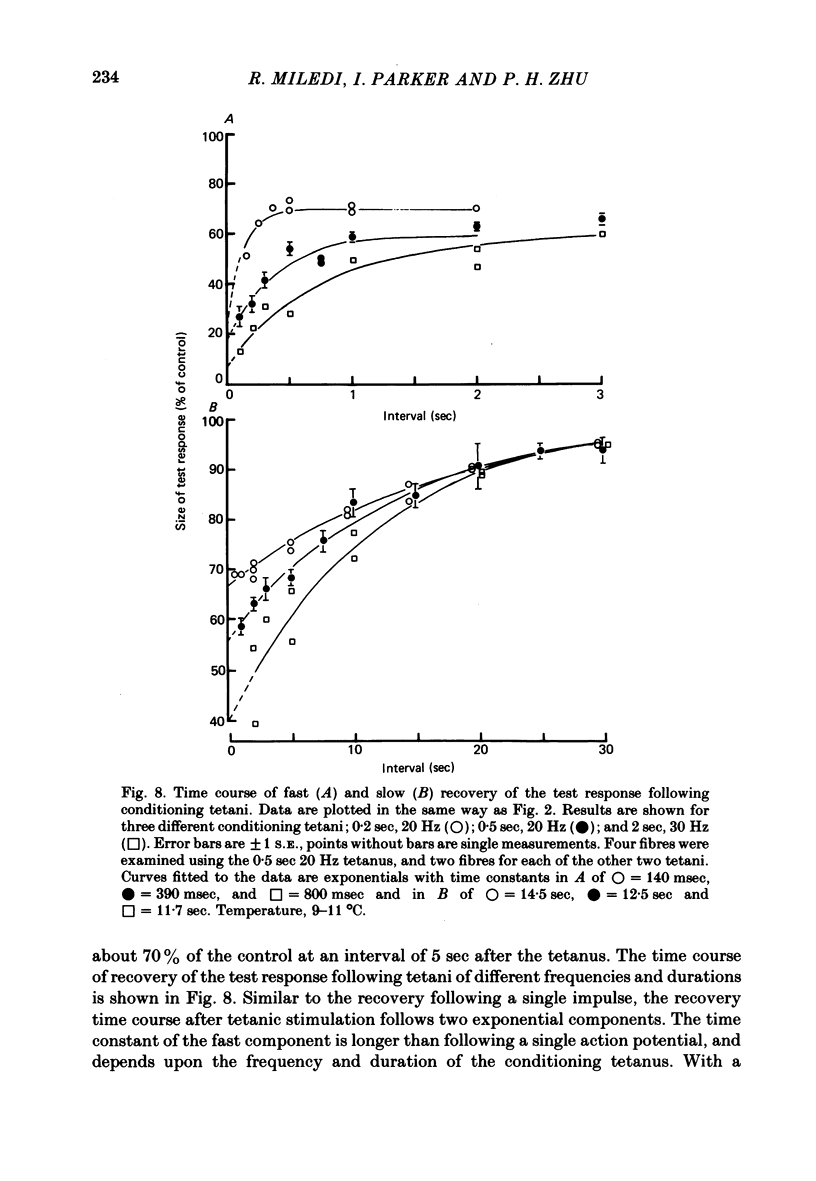
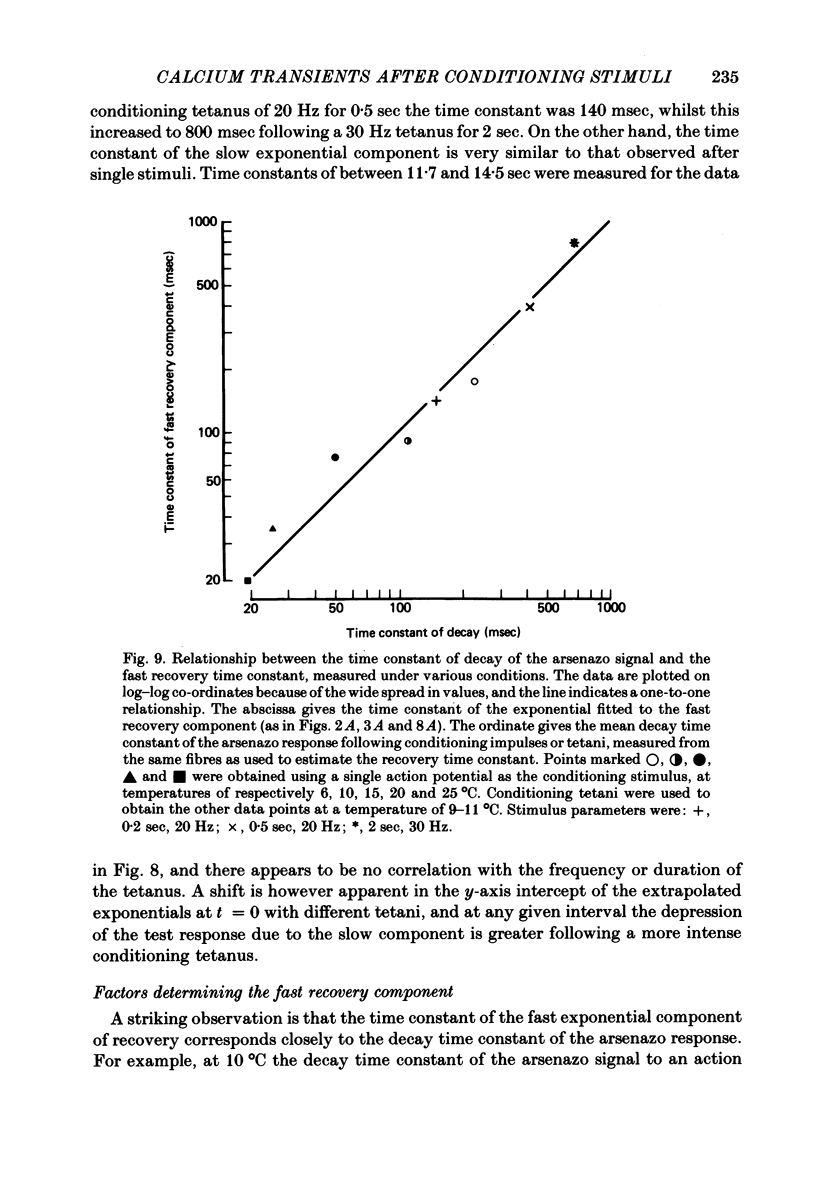
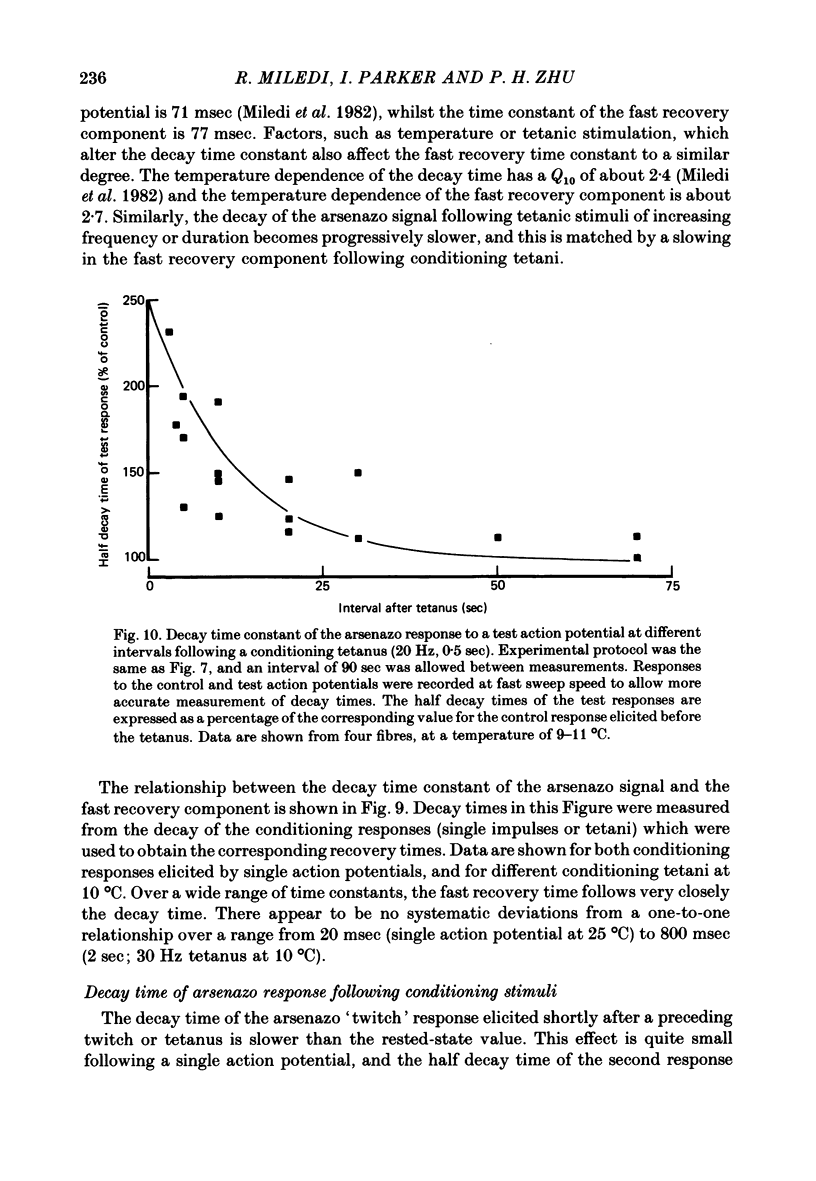
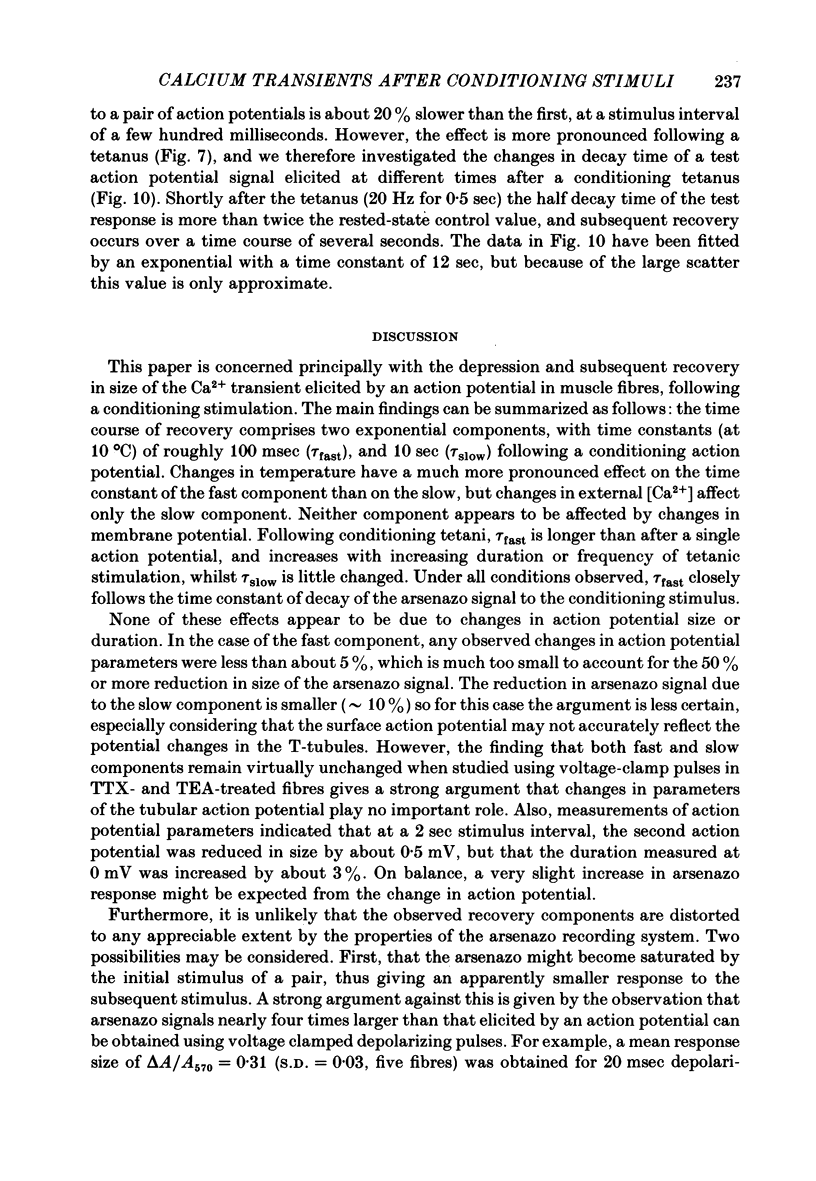
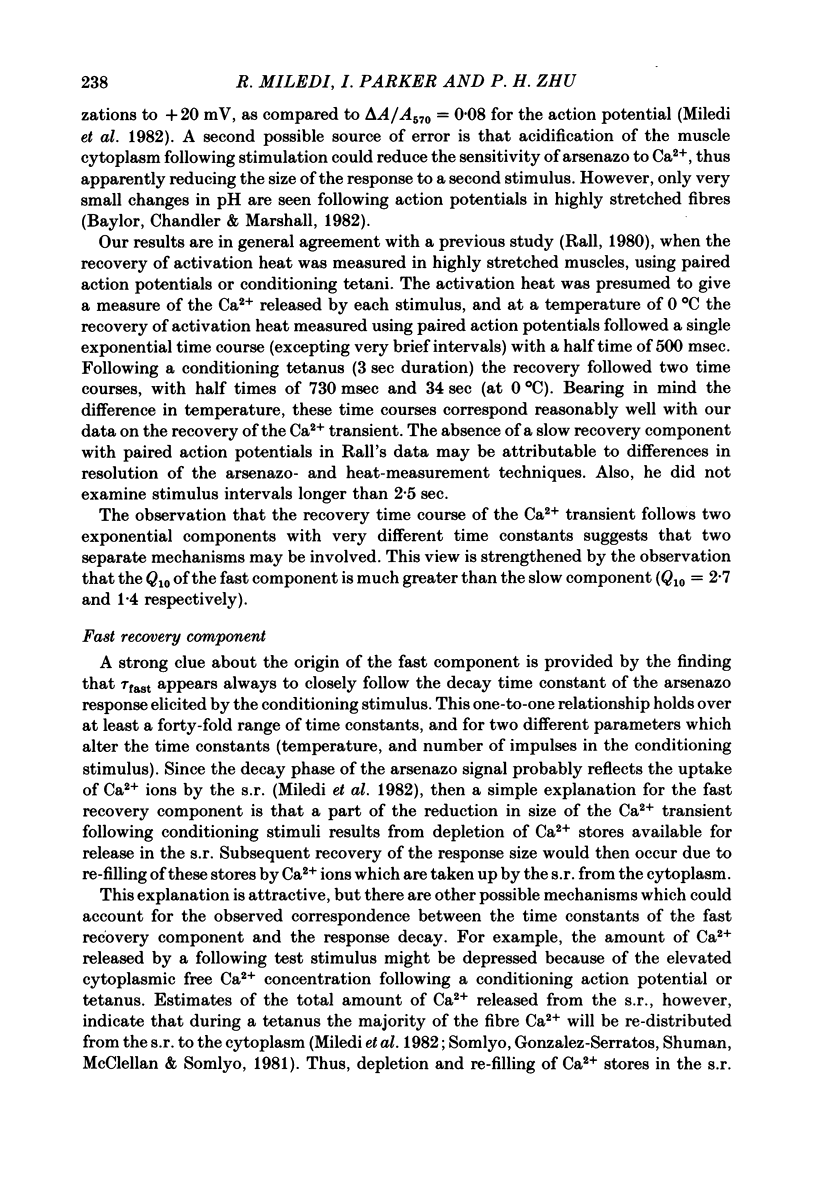
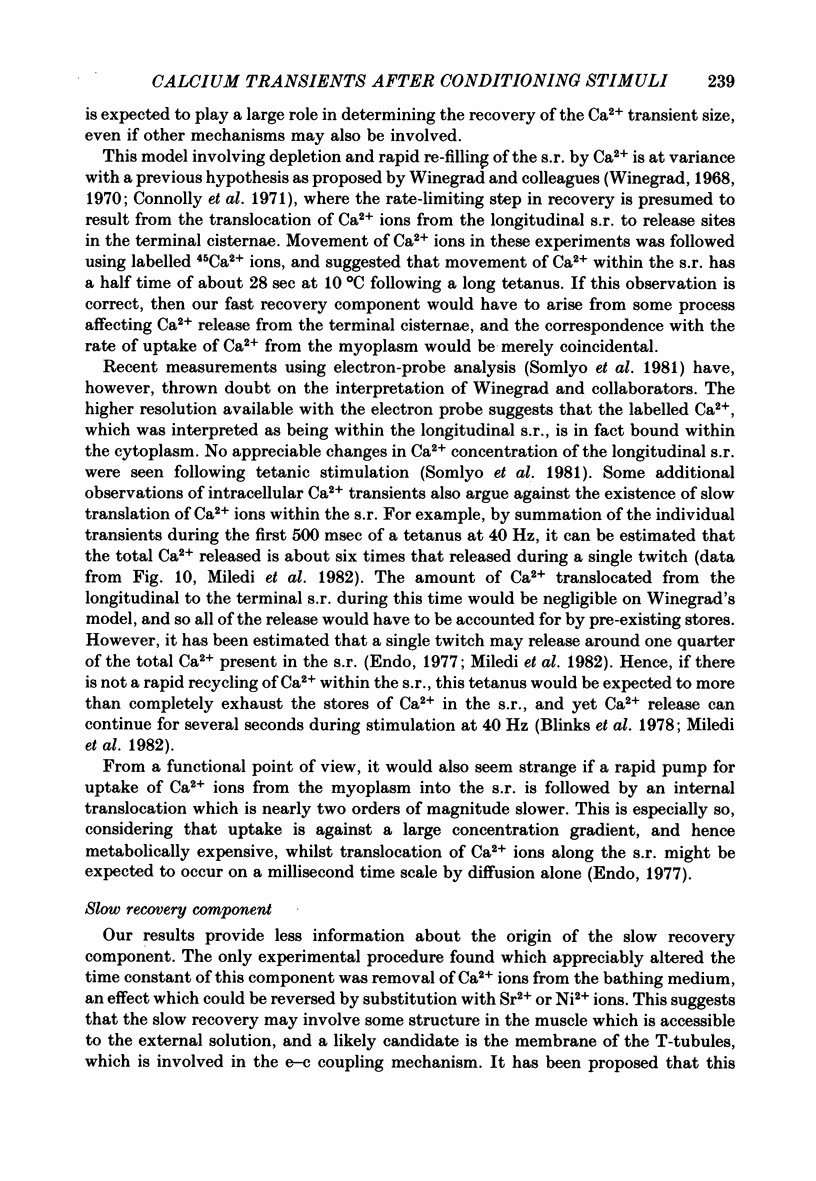
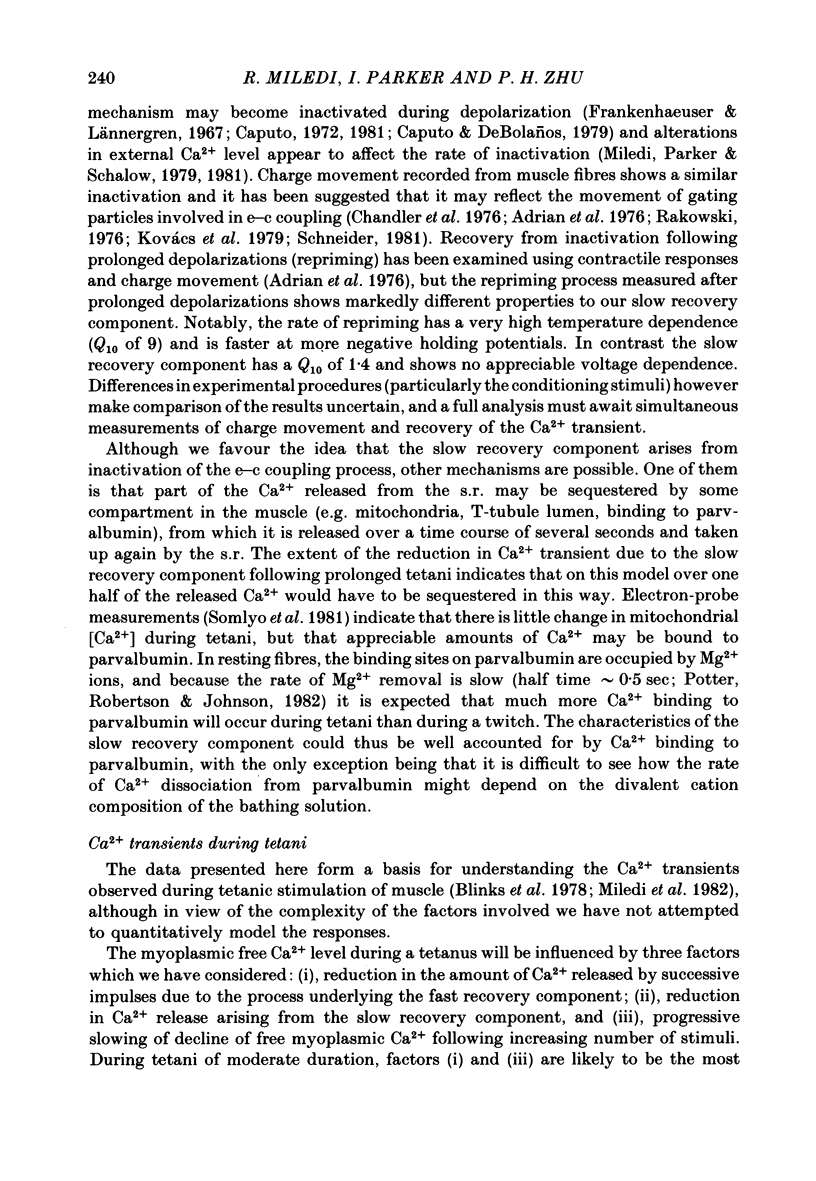
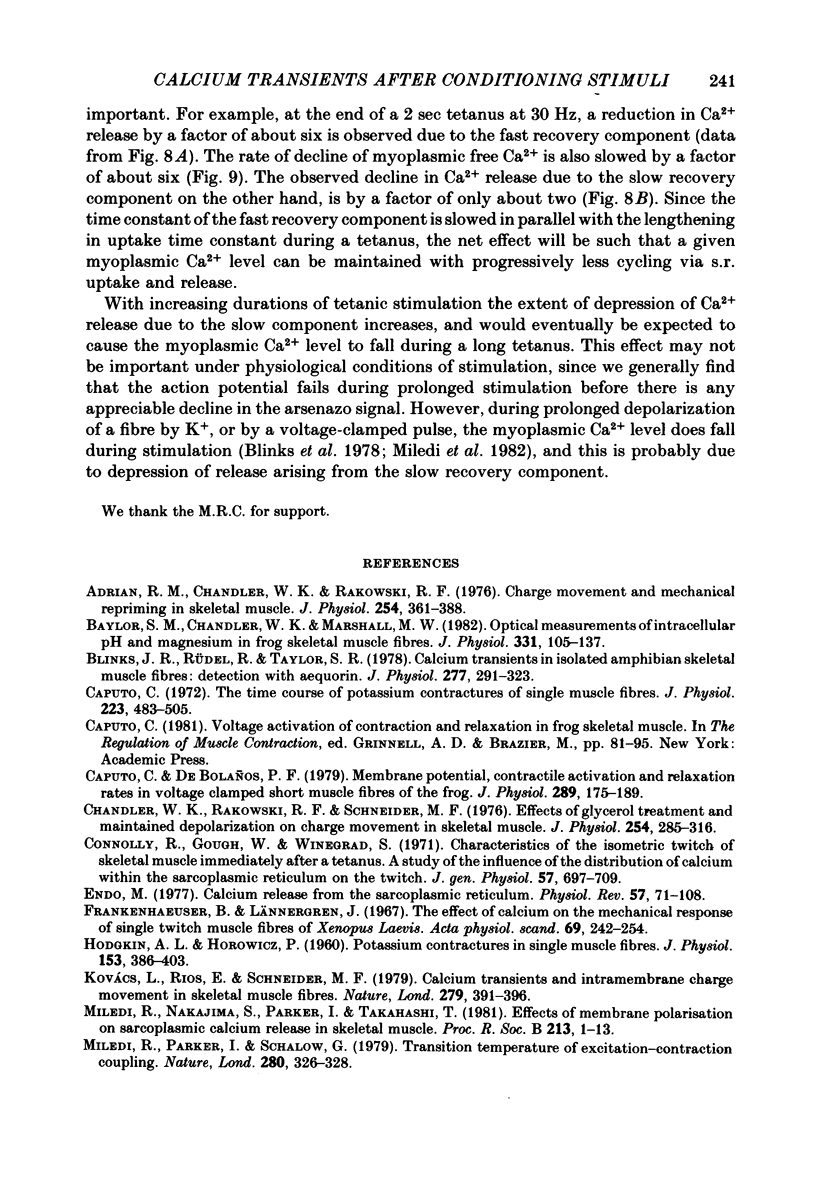
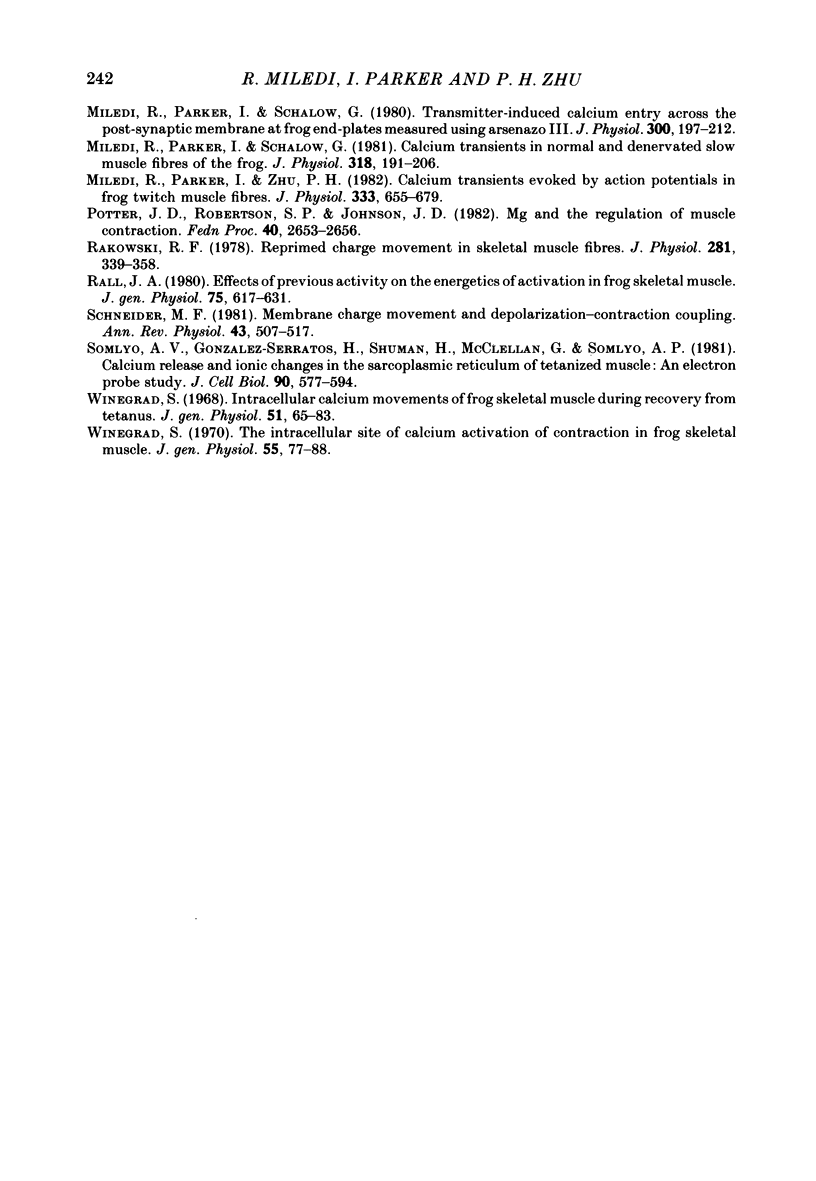
Selected References
These references are in PubMed. This may not be the complete list of references from this article.
- Adrian R. H., Chandler W. K., Rakowski R. F. Charge movement and mechanical repriming in skeletal muscle. J Physiol. 1976 Jan;254(2):361–388. doi: 10.1113/jphysiol.1976.sp011236. [DOI] [PMC free article] [PubMed] [Google Scholar]
- Baylor S. M., Chandler W. K., Marshall M. W. Optical measurements of intracellular pH and magnesium in frog skeletal muscle fibres. J Physiol. 1982 Oct;331:105–137. doi: 10.1113/jphysiol.1982.sp014367. [DOI] [PMC free article] [PubMed] [Google Scholar]
- Blinks J. R., Rüdel R., Taylor S. R. Calcium transients in isolated amphibian skeletal muscle fibres: detection with aequorin. J Physiol. 1978 Apr;277:291–323. doi: 10.1113/jphysiol.1978.sp012273. [DOI] [PMC free article] [PubMed] [Google Scholar]
- Caputo C., Fernandez de Bolaños P. Membrane potential, contractile activation and relaxation rates in voltage clamped short muscle fibres of the frog. J Physiol. 1979 Apr;289:175–189. doi: 10.1113/jphysiol.1979.sp012731. [DOI] [PMC free article] [PubMed] [Google Scholar]
- Caputo C. The time course of potassium contractures of single muscle fibres. J Physiol. 1972 Jun;223(2):483–505. doi: 10.1113/jphysiol.1972.sp009859. [DOI] [PMC free article] [PubMed] [Google Scholar]
- Chandler W. K., Rakowski R. F., Schneider M. F. Effects of glycerol treatment and maintained depolarization on charge movement in skeletal muscle. J Physiol. 1976 Jan;254(2):285–316. doi: 10.1113/jphysiol.1976.sp011233. [DOI] [PMC free article] [PubMed] [Google Scholar]
- Connolly R., Gough W., Winegrad S. Characteristics of the isometric twitch of skeletal muscle immediately after a tetanus. A study of the influence of the distribution of calcium within the sarcoplasmic reticulum on the twitch. J Gen Physiol. 1971 Jun;57(6):697–709. [PubMed] [Google Scholar]
- Endo M. Calcium release from the sarcoplasmic reticulum. Physiol Rev. 1977 Jan;57(1):71–108. doi: 10.1152/physrev.1977.57.1.71. [DOI] [PubMed] [Google Scholar]
- Frankenhaeuser B., Lännergren J. The effect of calcium on the mechanical response of single twitch muscle fibres of Xenopus laevis. Acta Physiol Scand. 1967 Mar;69(3):242–254. doi: 10.1111/j.1748-1716.1967.tb03518.x. [DOI] [PubMed] [Google Scholar]
- HODGKIN A. L., HOROWICZ P. Potassium contractures in single muscle fibres. J Physiol. 1960 Sep;153:386–403. doi: 10.1113/jphysiol.1960.sp006541. [DOI] [PMC free article] [PubMed] [Google Scholar]
- Kovács L., Ríos E., Schneider M. F. Calcium transients and intramembrane charge movement in skeletal muscle fibres. Nature. 1979 May 31;279(5712):391–396. doi: 10.1038/279391a0. [DOI] [PubMed] [Google Scholar]
- Miledi R., Nakajima S., Parker I., Takahashi T. Effects of membrane polarization on sarcoplasmic calcium release in skeletal muscle. Proc R Soc Lond B Biol Sci. 1981 Sep 17;213(1190):1–13. doi: 10.1098/rspb.1981.0049. [DOI] [PubMed] [Google Scholar]
- Miledi R., Parker I., Schalow G. Calcium transients in normal and denervated slow muscle fibres of the frog. J Physiol. 1981 Sep;318:191–206. doi: 10.1113/jphysiol.1981.sp013858. [DOI] [PMC free article] [PubMed] [Google Scholar]
- Miledi R., Parker I., Schalow G. Transition temperature of excitation-contraction coupling in frog twitch muscle fibres. Nature. 1979 Jul 26;280(5720):326–328. doi: 10.1038/280326a0. [DOI] [PubMed] [Google Scholar]
- Miledi R., Parker I., Schalow G. Transmitter induced calcium entry across the post-synaptic membrane at frog end-plates measured using arsenazo III. J Physiol. 1980 Mar;300:197–212. doi: 10.1113/jphysiol.1980.sp013158. [DOI] [PMC free article] [PubMed] [Google Scholar]
- Miledi R., Parker I., Zhu P. H. Calcium transients evoked by action potentials in frog twitch muscle fibres. J Physiol. 1982 Dec;333:655–679. doi: 10.1113/jphysiol.1982.sp014474. [DOI] [PMC free article] [PubMed] [Google Scholar]
- Potter J. D., Robertson S. P., Johnson J. D. Magnesium and the regulation of muscle contraction. Fed Proc. 1981 Oct;40(12):2653–2656. [PubMed] [Google Scholar]
- Rakowski R. F. Reprimed charge movement in skeletal muscle fibres. J Physiol. 1978 Aug;281:339–358. doi: 10.1113/jphysiol.1978.sp012426. [DOI] [PMC free article] [PubMed] [Google Scholar]
- Rall J. A. Effects of previous activity on the energetics of activation in frog skeletal muscle. J Gen Physiol. 1980 Jun;75(6):617–631. doi: 10.1085/jgp.75.6.617. [DOI] [PMC free article] [PubMed] [Google Scholar]
- Schneider M. F. Membrane charge movement and depolarization-contraction coupling. Annu Rev Physiol. 1981;43:507–517. doi: 10.1146/annurev.ph.43.030181.002451. [DOI] [PubMed] [Google Scholar]
- Somlyo A. V., Gonzalez-Serratos H. G., Shuman H., McClellan G., Somlyo A. P. Calcium release and ionic changes in the sarcoplasmic reticulum of tetanized muscle: an electron-probe study. J Cell Biol. 1981 Sep;90(3):577–594. doi: 10.1083/jcb.90.3.577. [DOI] [PMC free article] [PubMed] [Google Scholar]
- Winegrad S. Intracellular calcium movements of frog skeletal muscle during recovery from tetanus. J Gen Physiol. 1968 Jan;51(1):65–83. doi: 10.1085/jgp.51.1.65. [DOI] [PMC free article] [PubMed] [Google Scholar]
- Winegrad S. The intracellular site of calcium activaton of contraction in frog skeletal muscle. J Gen Physiol. 1970 Jan;55(1):77–88. doi: 10.1085/jgp.55.1.77. [DOI] [PMC free article] [PubMed] [Google Scholar]