Abstract
Details of the blocking action of chlorisondamine, a ganglionic nicotinic blocker, on the excitatory cholinergic currents of the spiny lobster gastric mill 1 (g.m.1) muscle are described. The steady-state block of cholinergic ionophoretic currents produced by chlorisondamine is strongly voltage-dependent. During a hyperpolarizing voltage step, a sequence of ionophoretic agonist pulses in the presence of chlorisondamine shows a large interpulse interaction manifested as a gradual diminution in response amplitude. The extent of diminution is dependent on the number of the pulse in a series and not on the duration of the interval between pulses. The slowly developing blockade is entirely dependent on agonist application. If agonist application is suspended for various time intervals following the development of a given blocked level in chlorisondamine, no recovery from the block is observed whether the rest interval is at the step potential or at more depolarized potentials. Recovery from a given blocked level can be observed if, during a depolarizing voltage step (to -60 mV) away from the potential at which the block was established (-140 mV), agonist is applied before return to the initial potential (-140 mV). Chlorisondamine produces a dose-dependent reduction in excitatory junctional current (e.j.c.) decay rate that is linear with chlorisondamine concentration and markedly dependent on voltage (approximately equal to 35 mV/e-fold change). Reduction in the amplitude of e.j.c.s occurred at concentrations of chlorisondamine that produced no detectable effect on e.j.c. decay. Alterations in e.j.c. amplitude showed time- and use-dependent aspects similar to those observed for ionophoretic currents. These results are discussed primarily in terms of a sequential model in which, following the binding of chlorisondamine to the opened ion channel, the channel can undergo a transition to a stable-blocked state that requires reactivation by agonist to become unblocked. This stable-blocked state is considered a closed-blocked channel.
Full text
PDF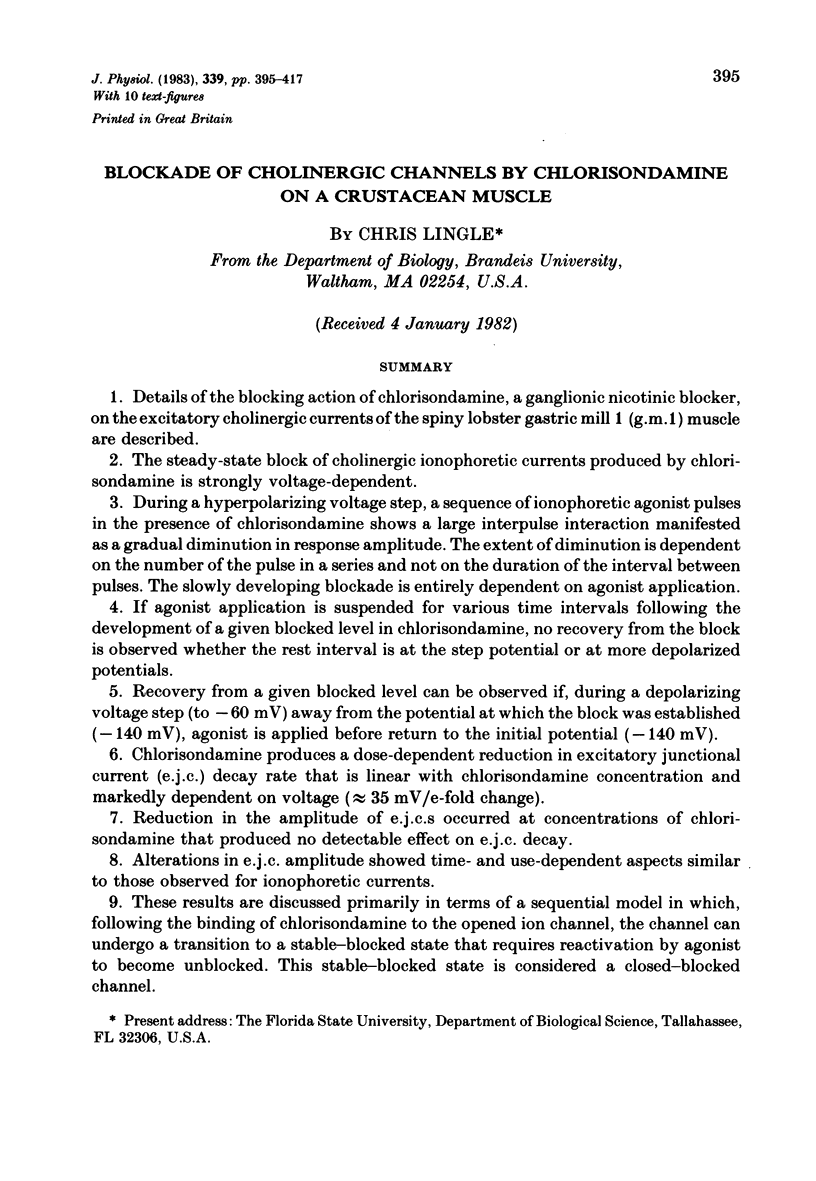
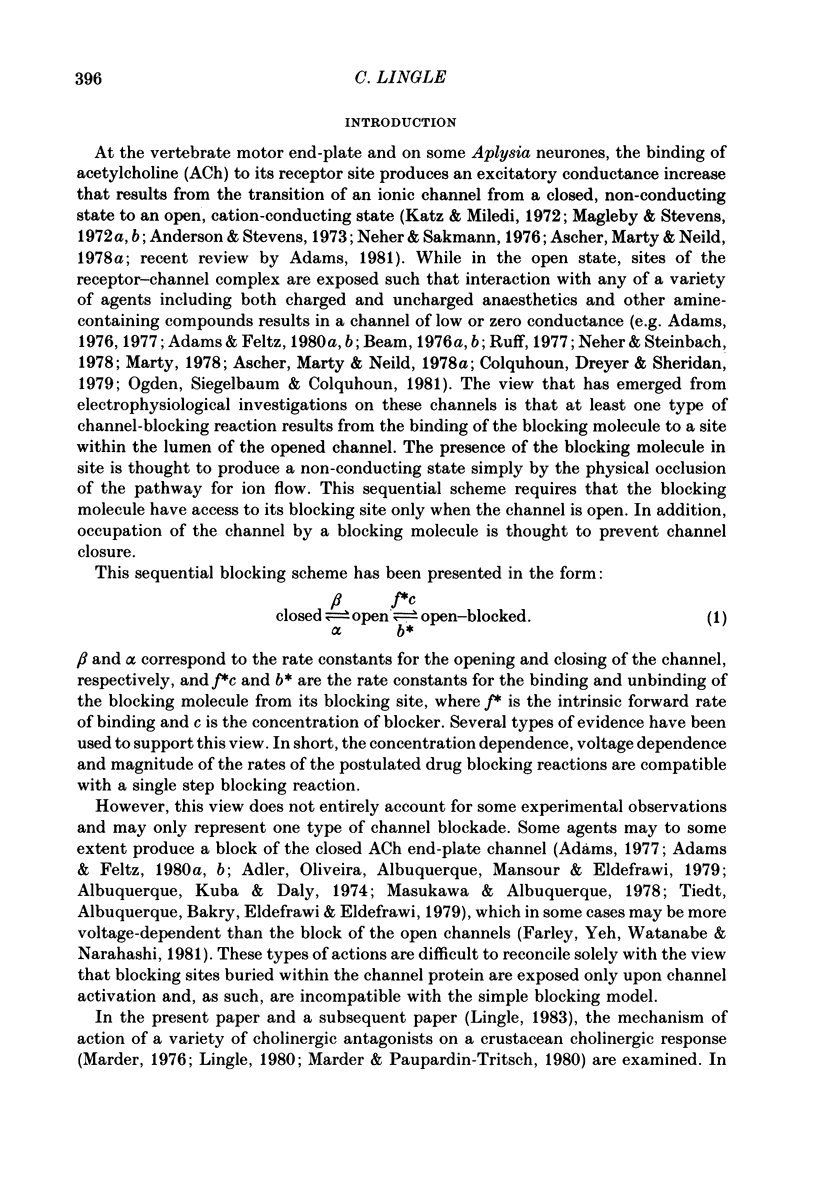
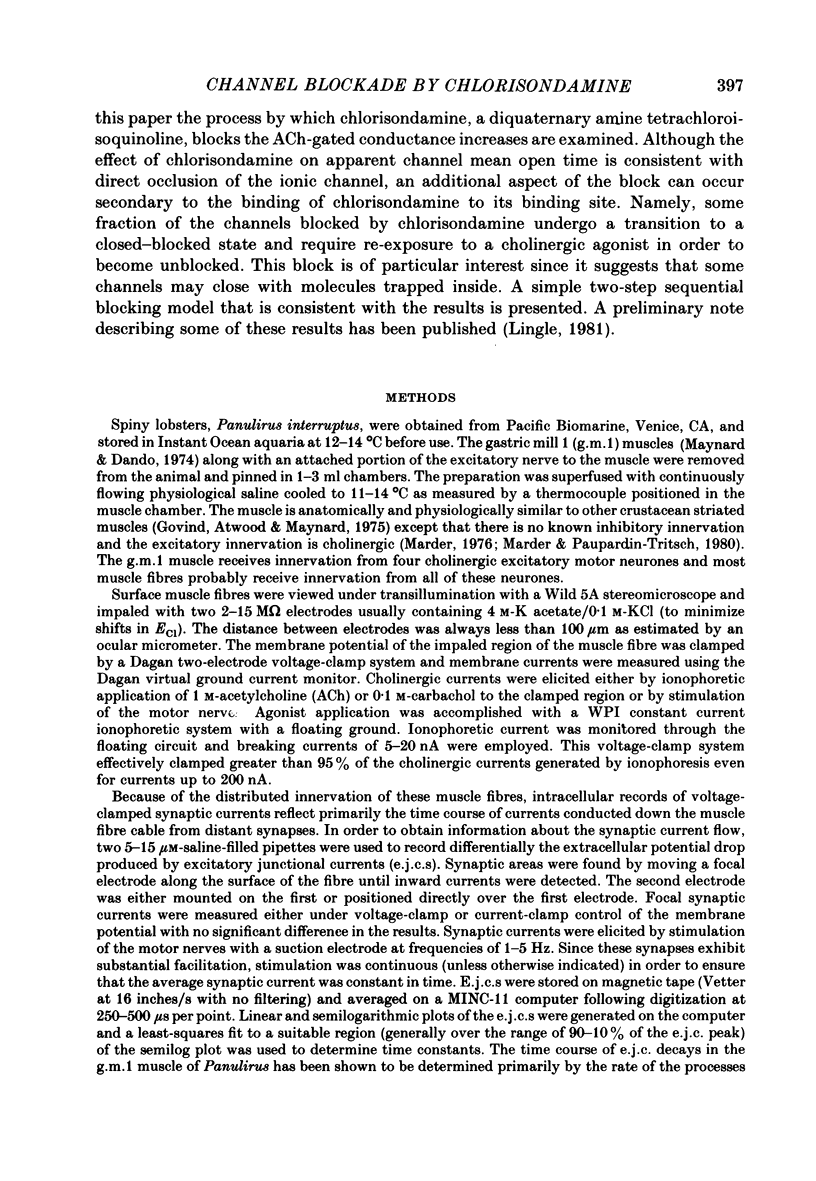
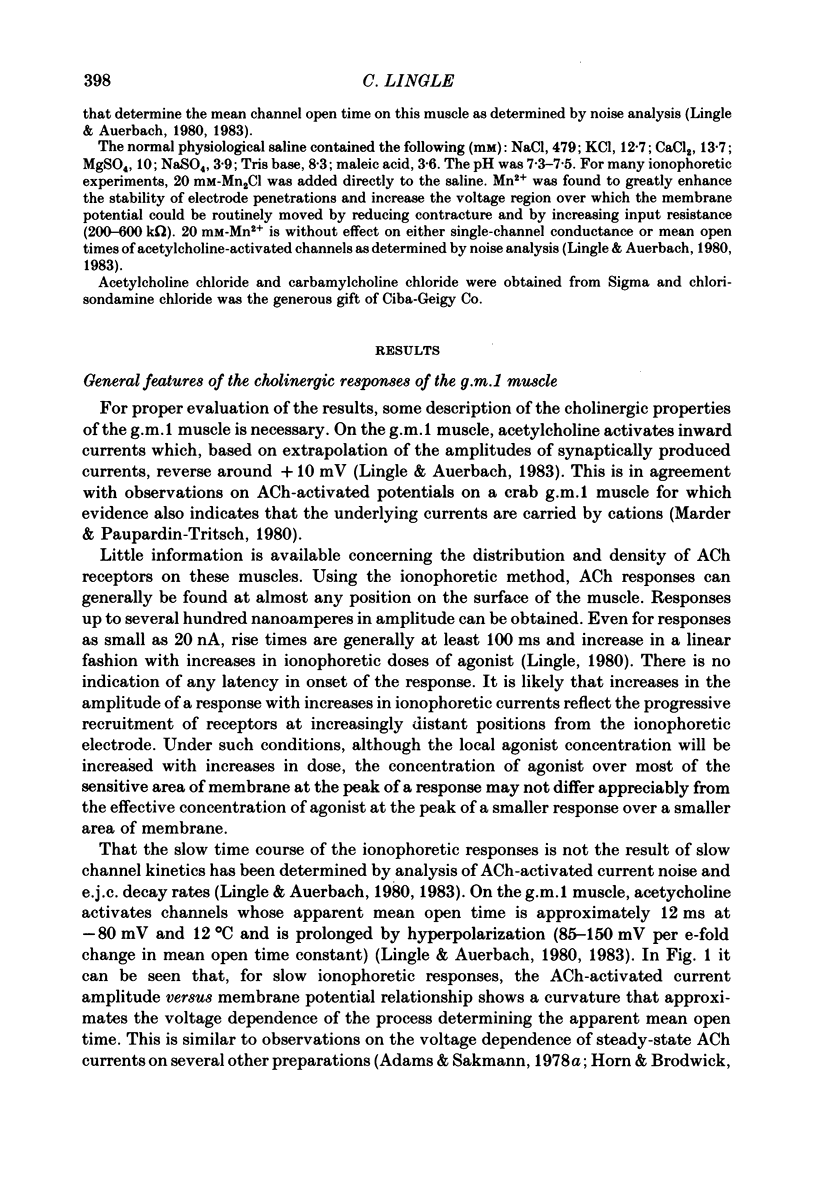
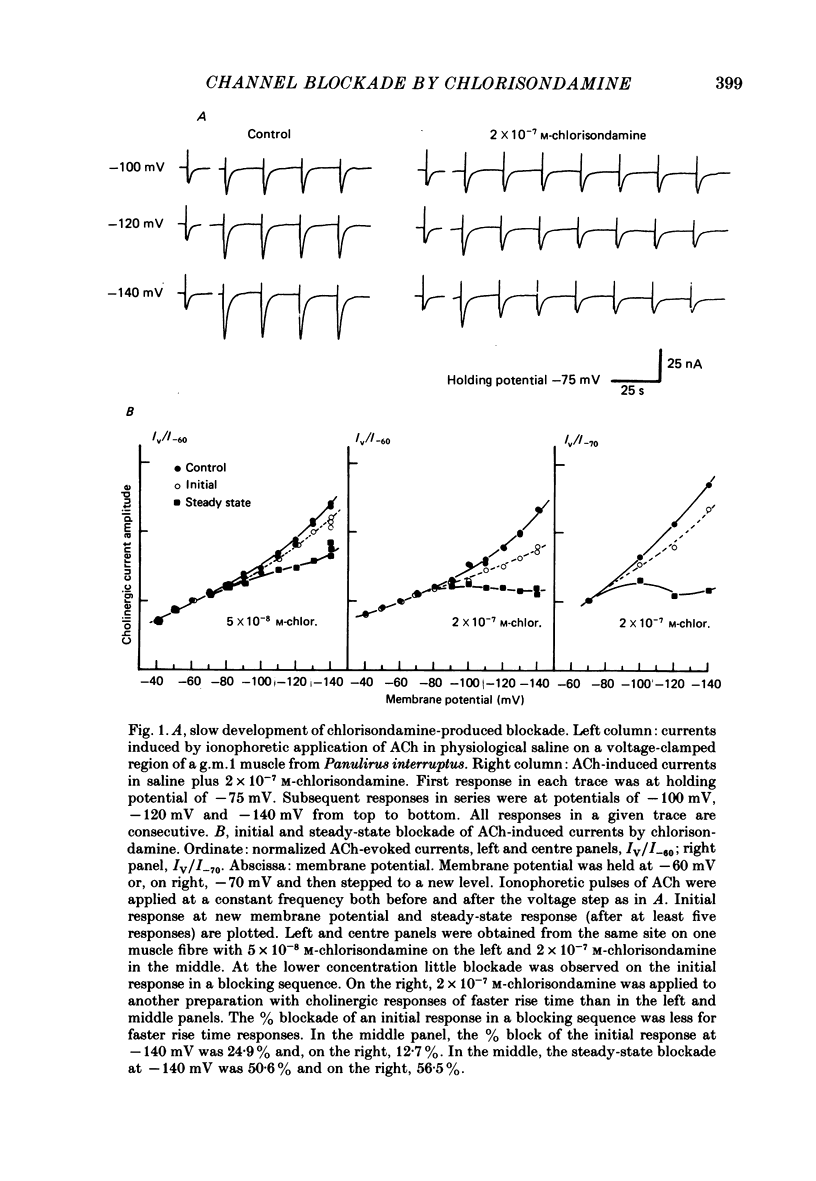
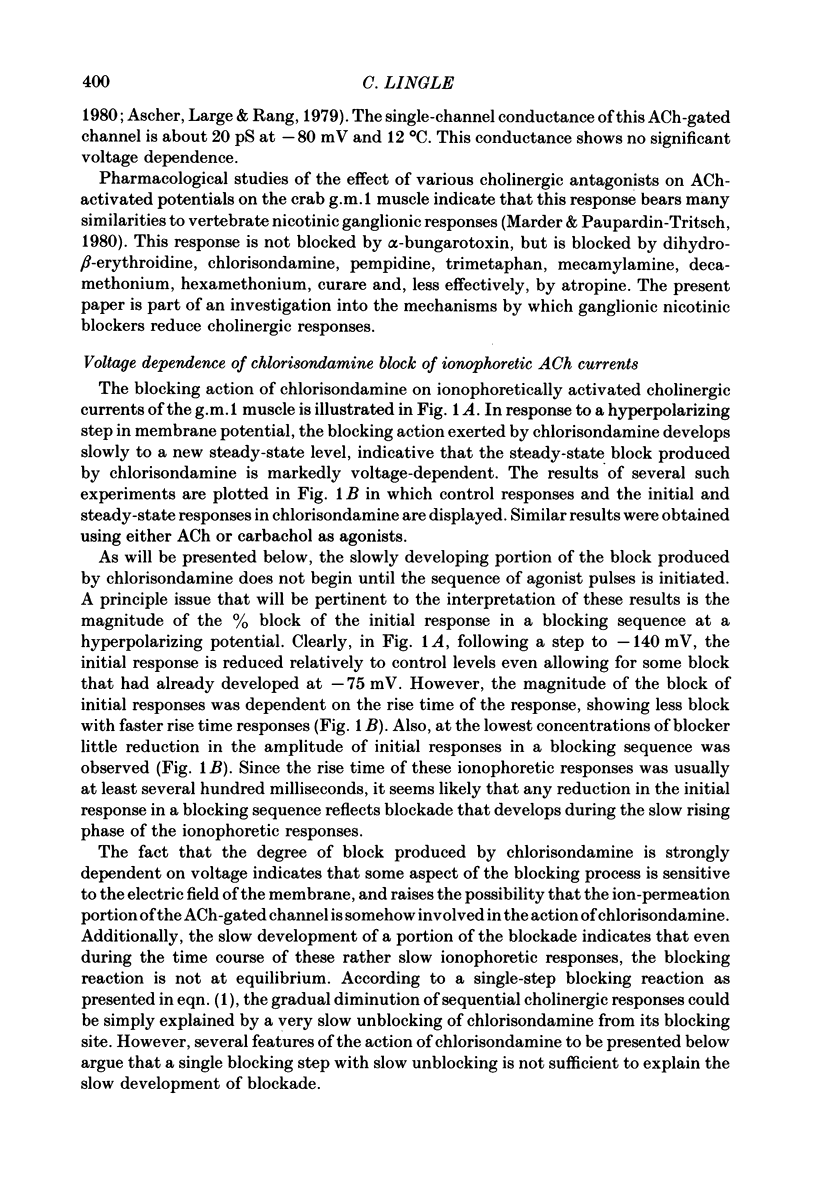
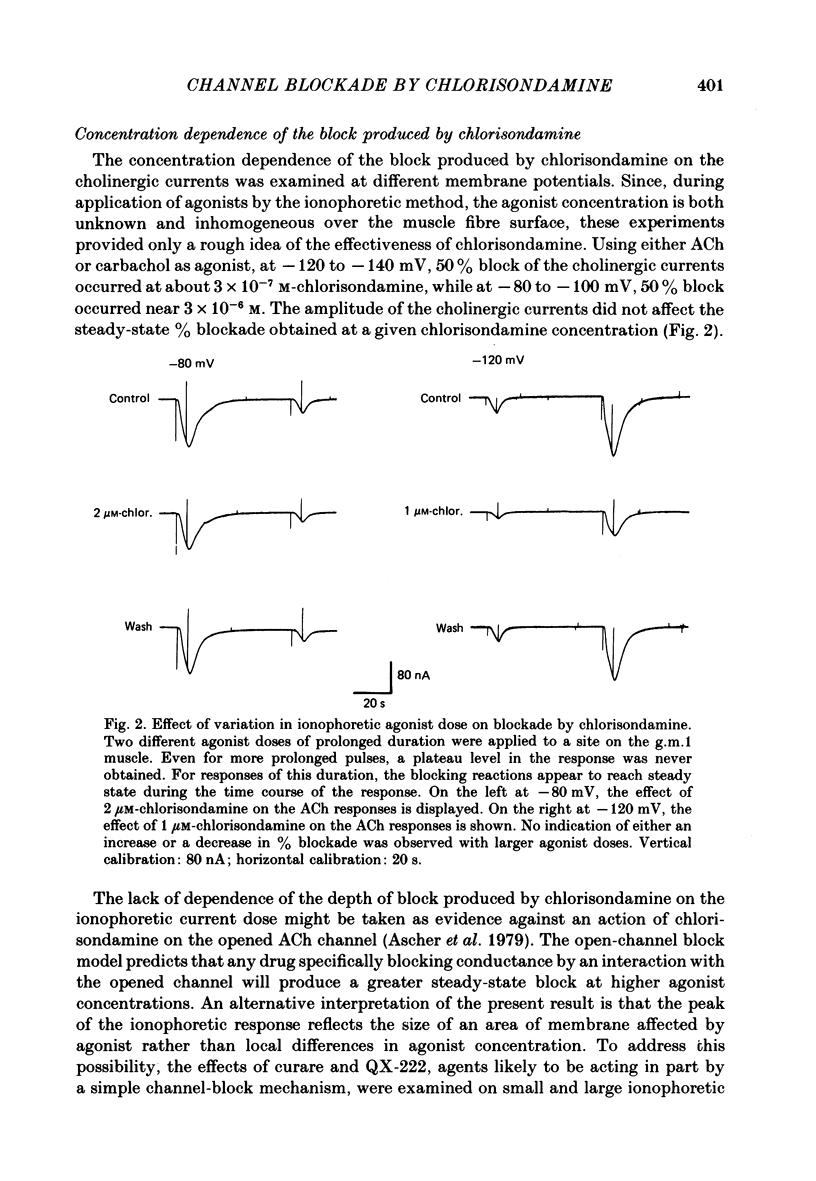
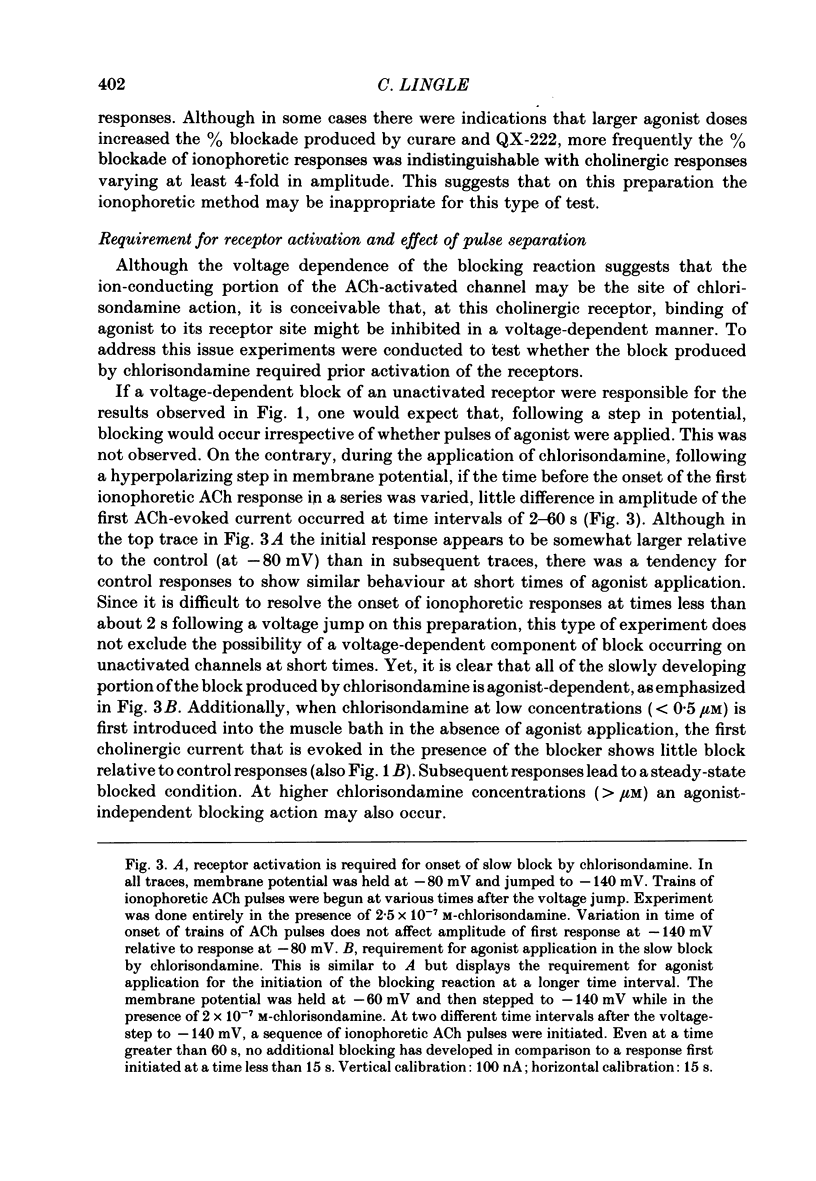
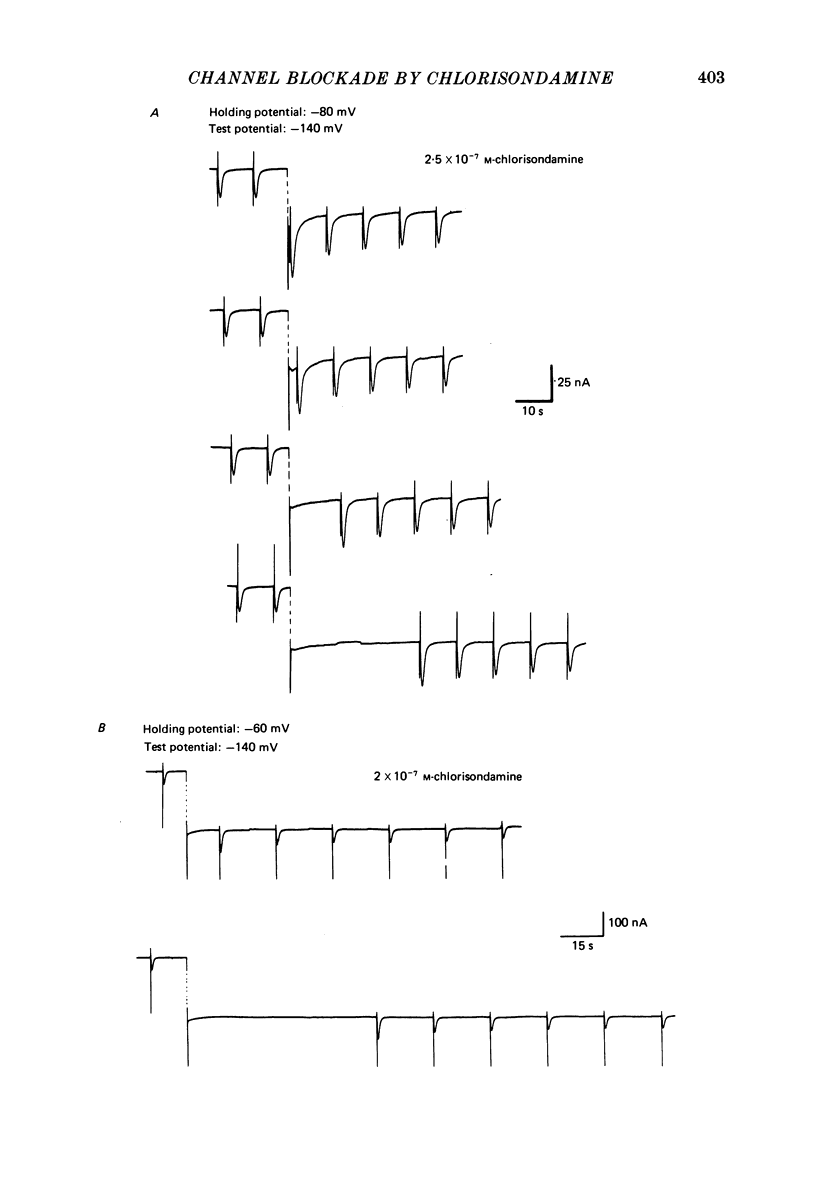
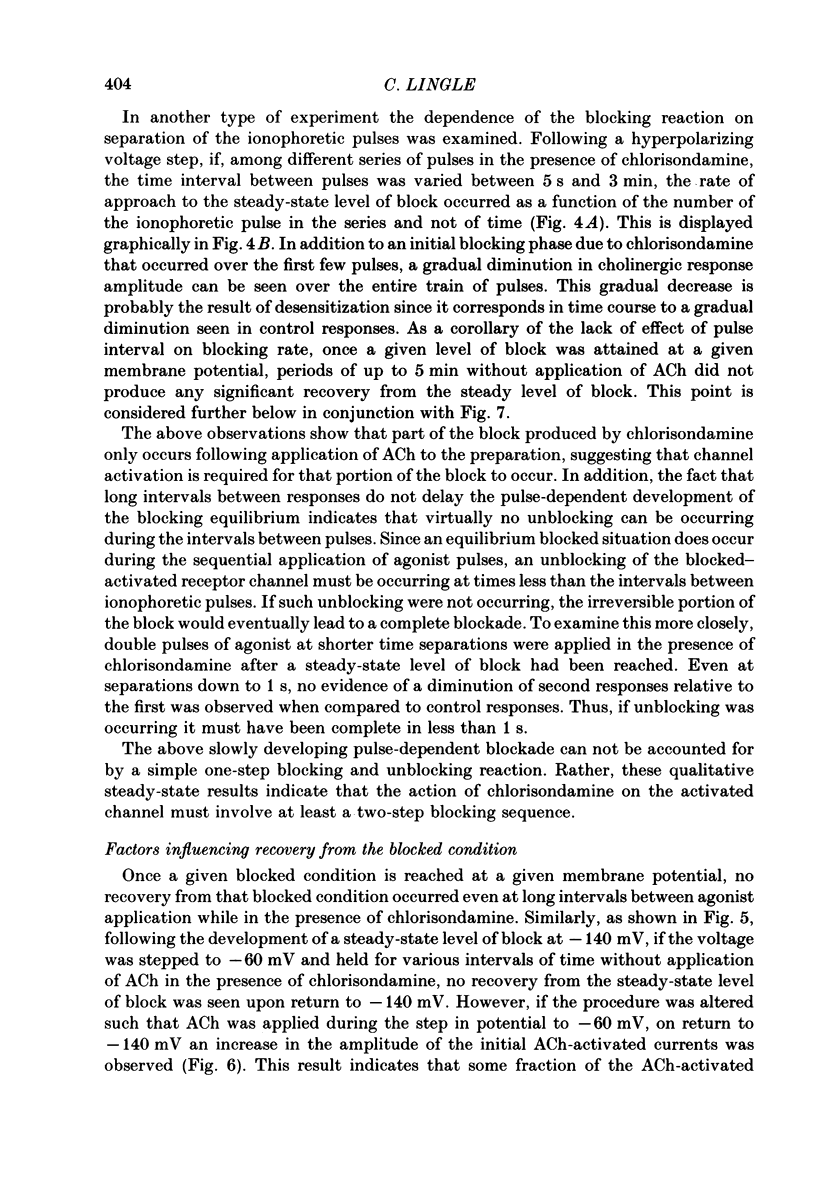
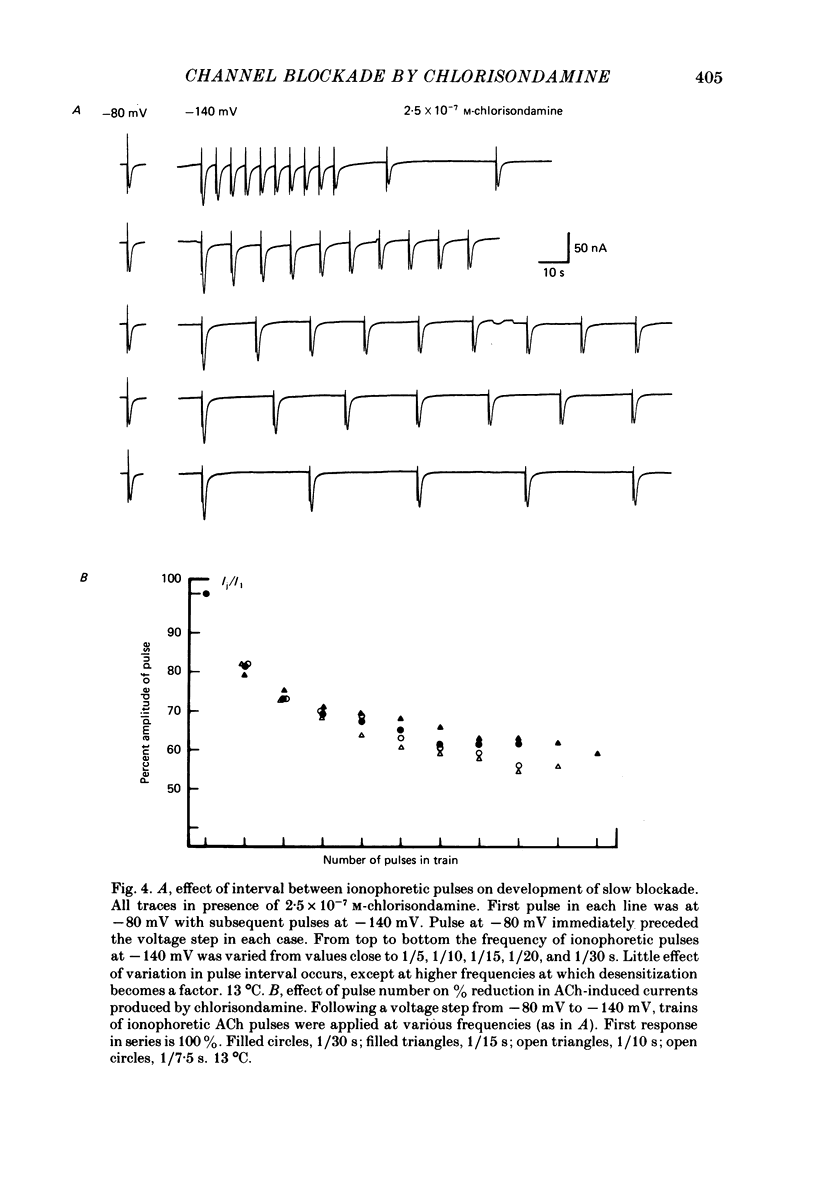
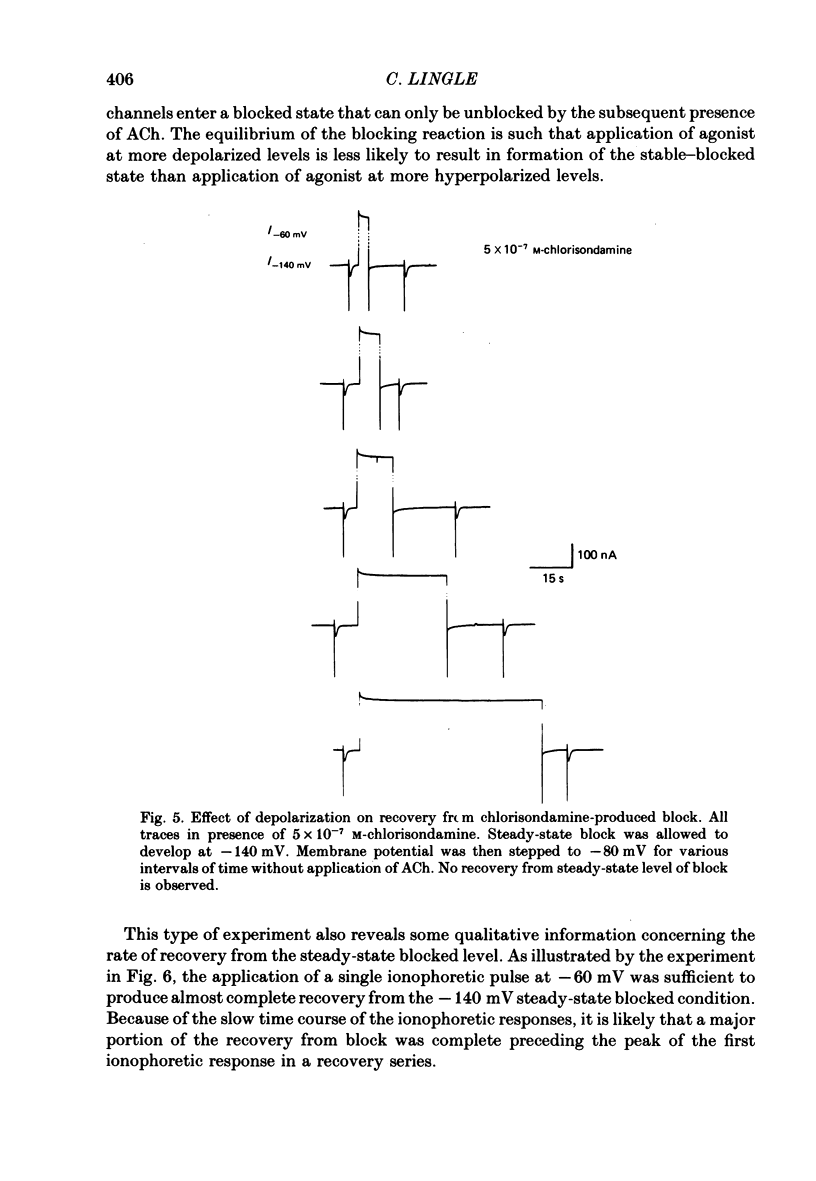
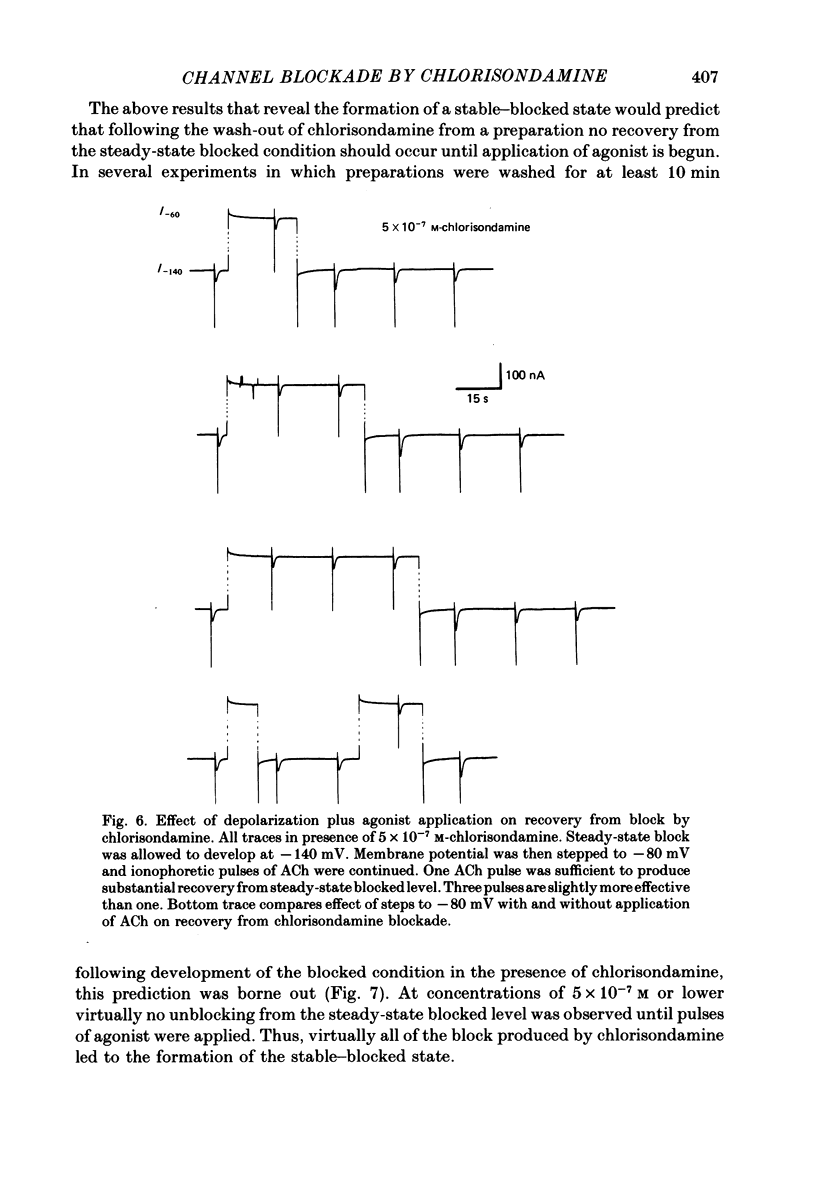
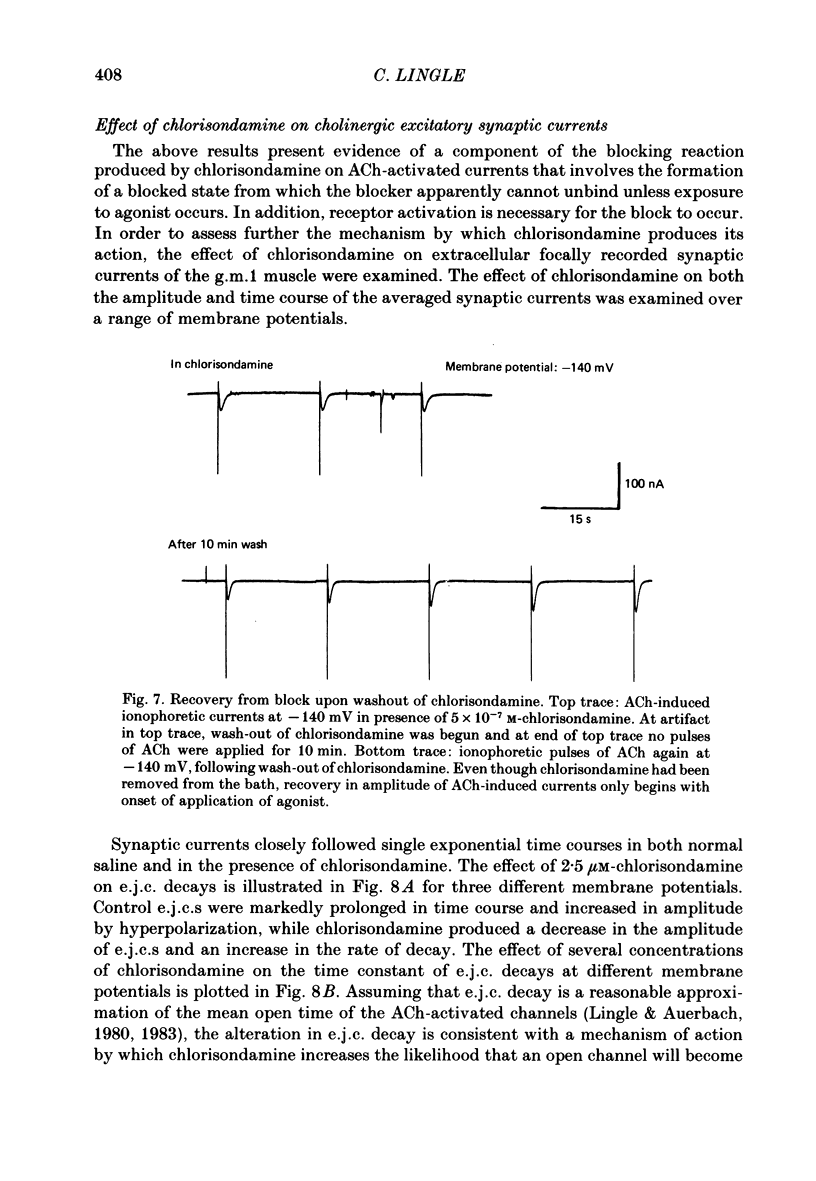
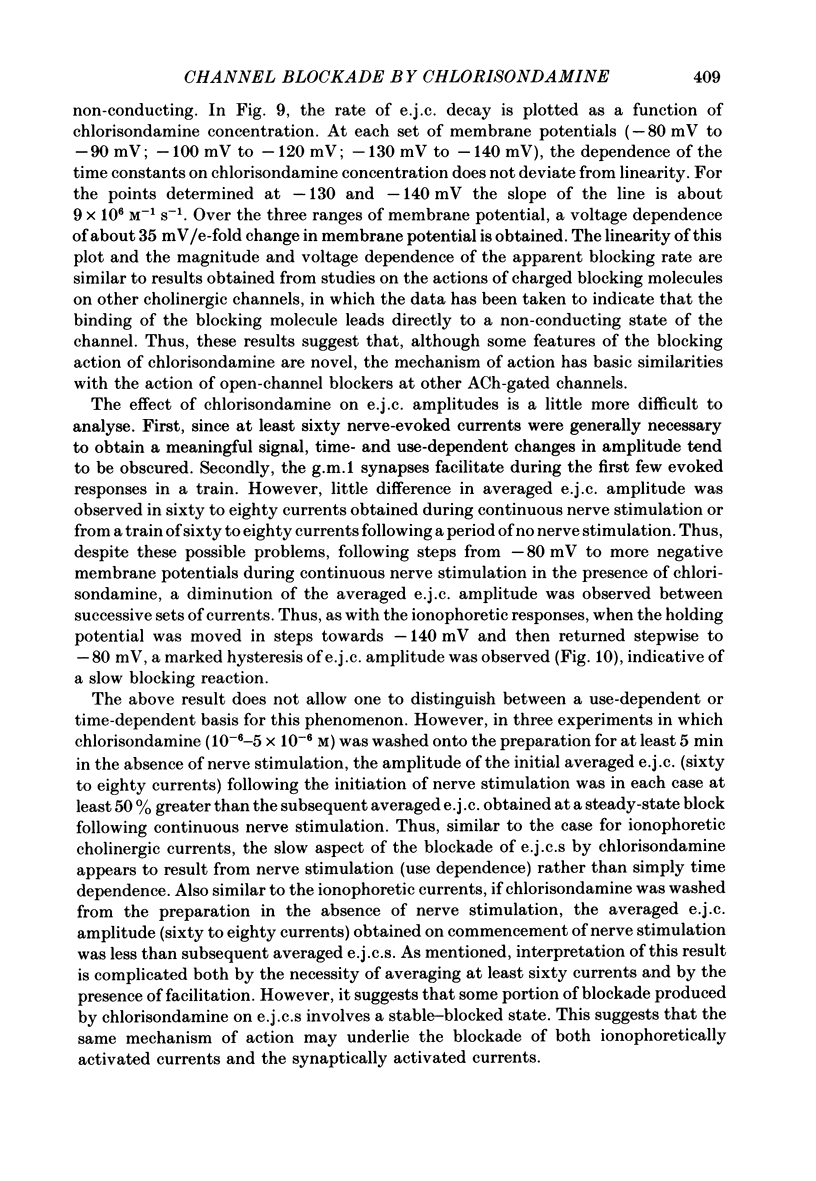
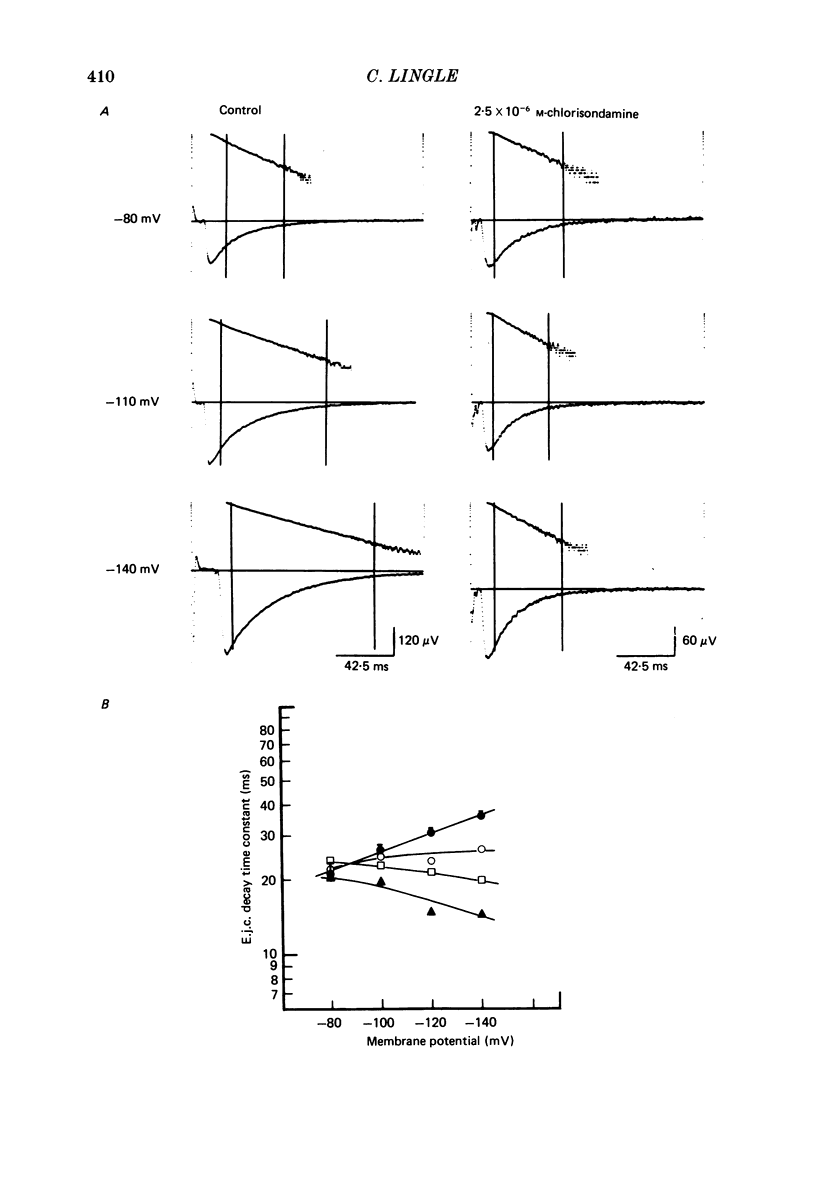
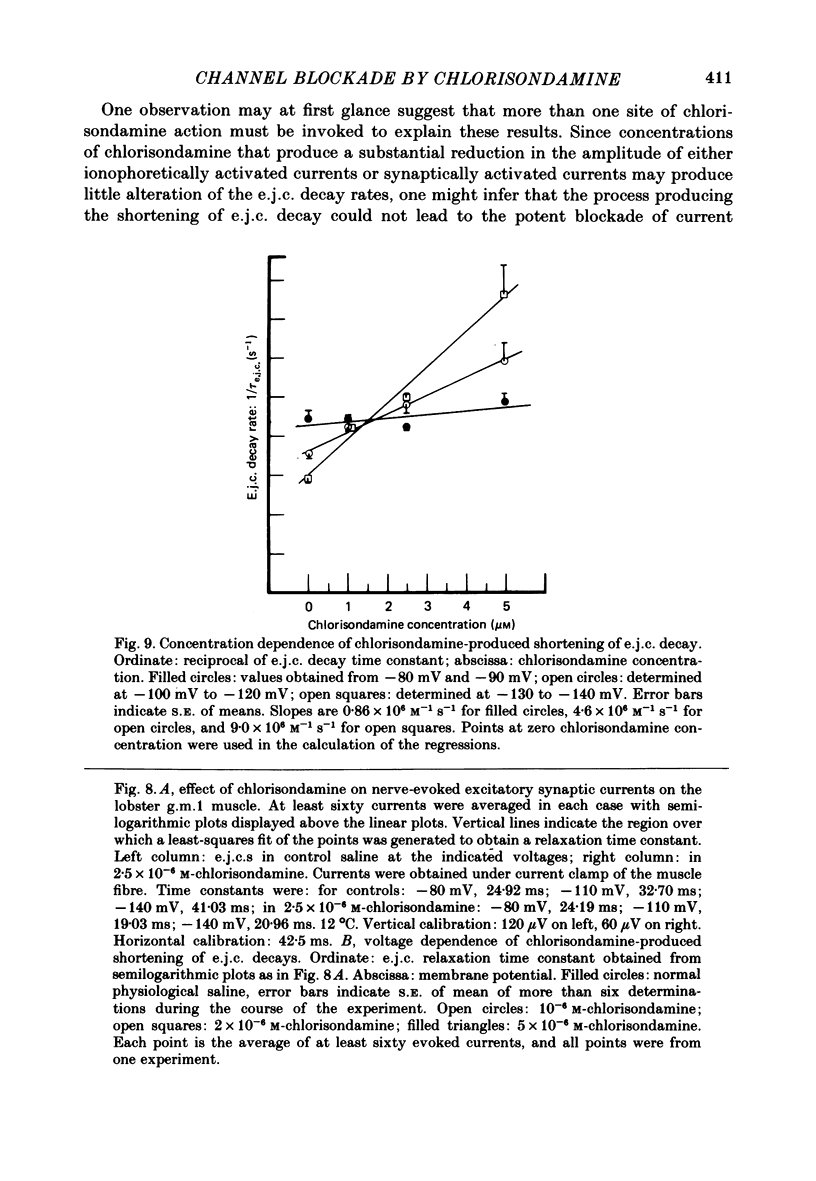
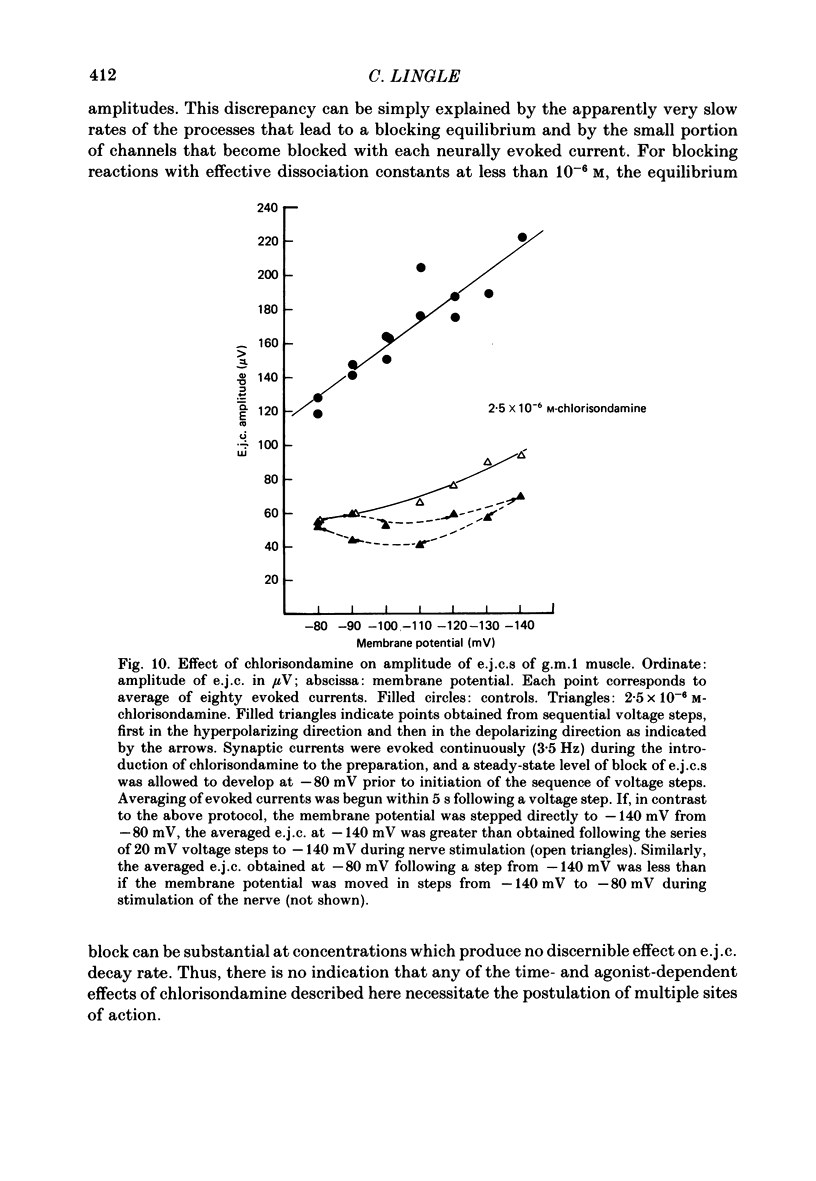
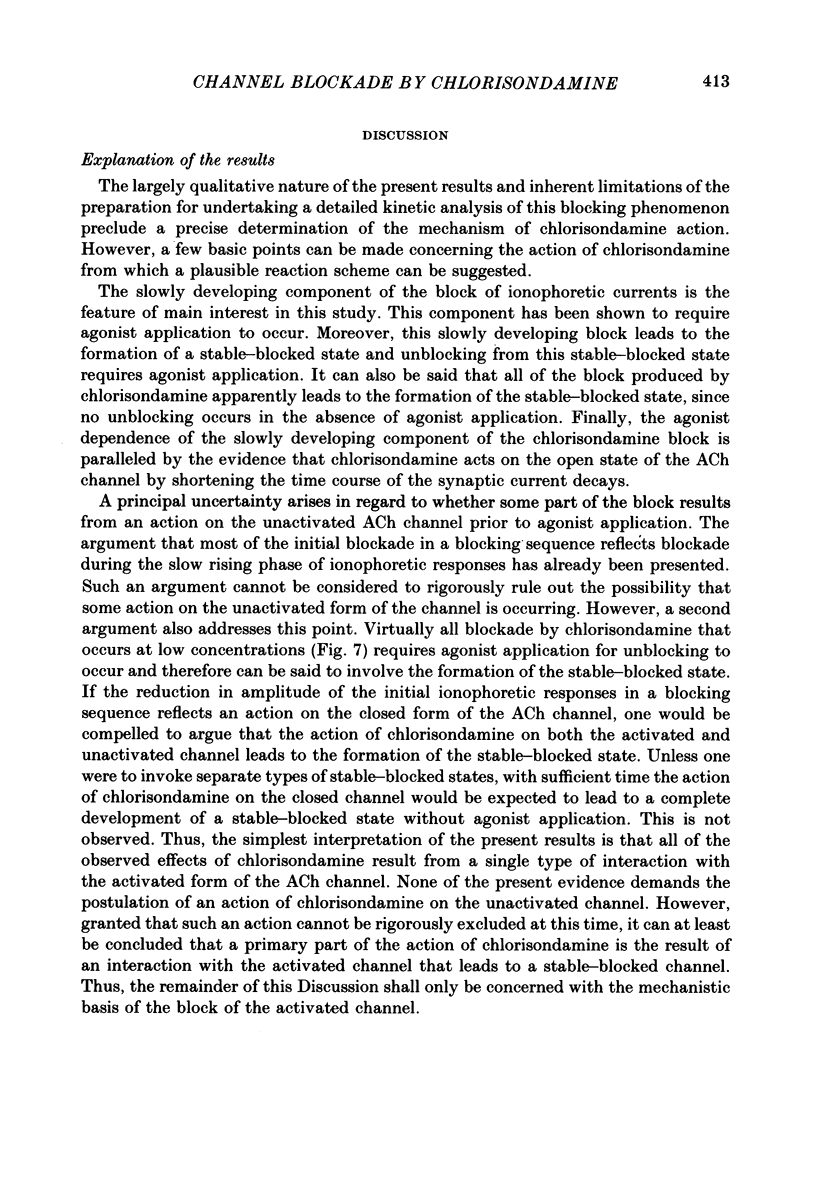
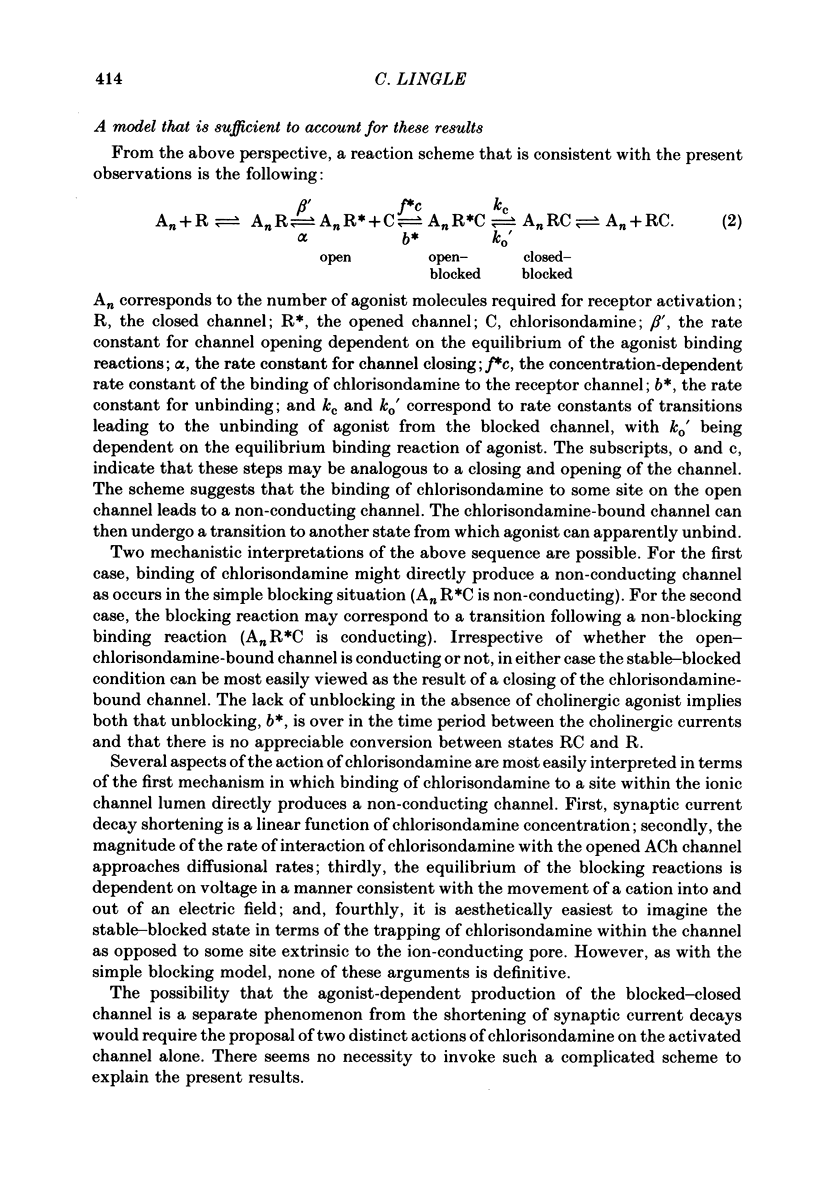
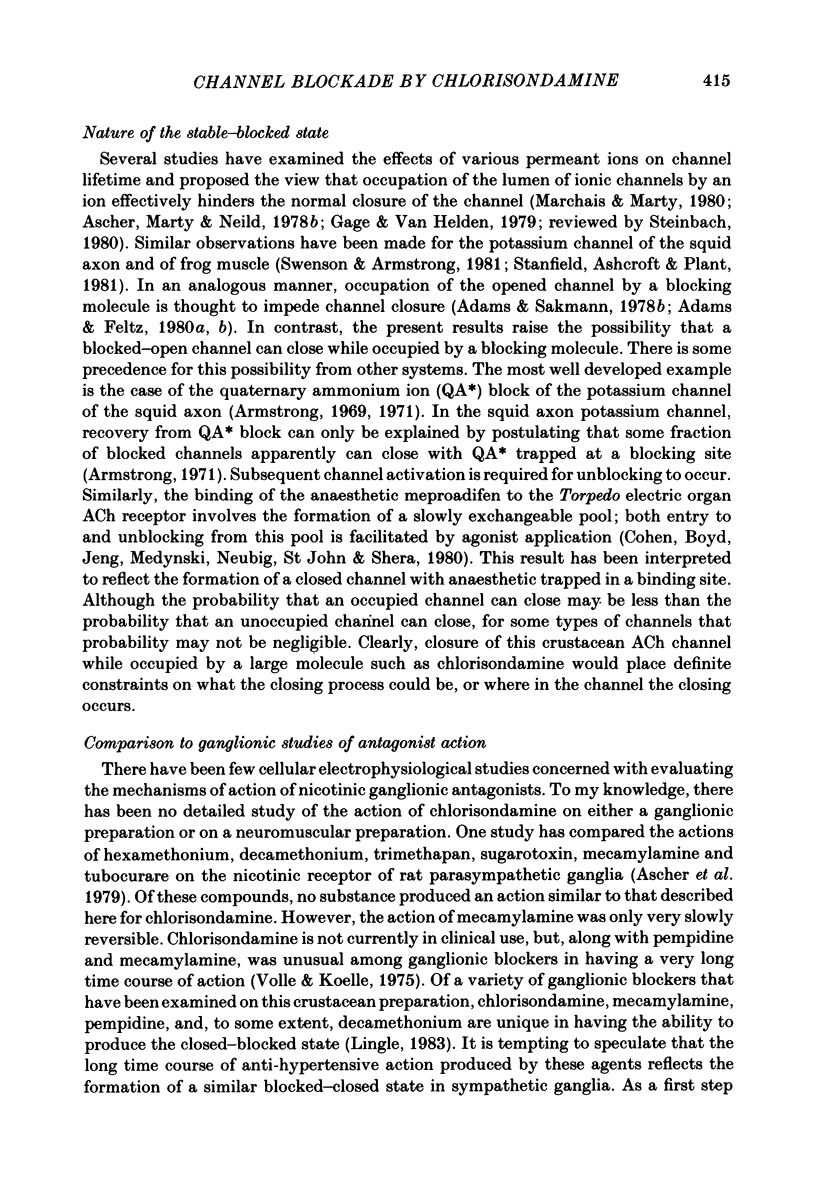
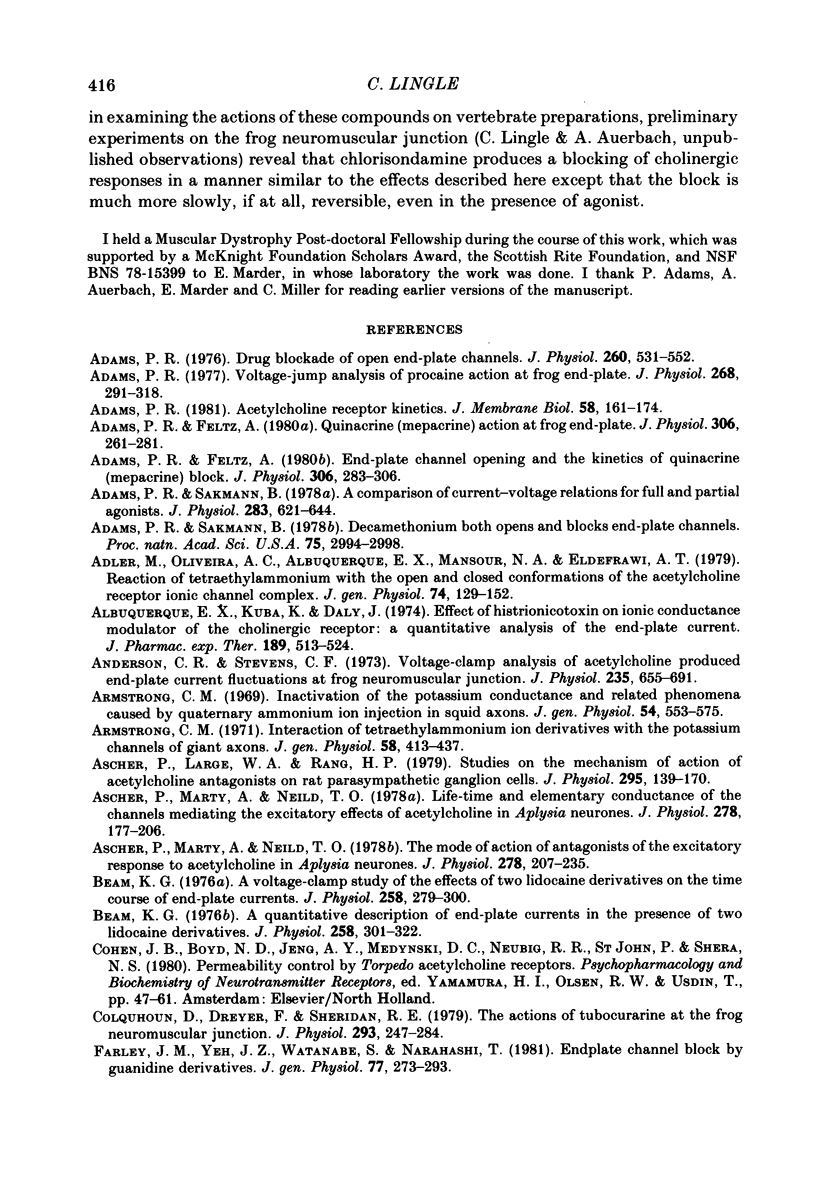
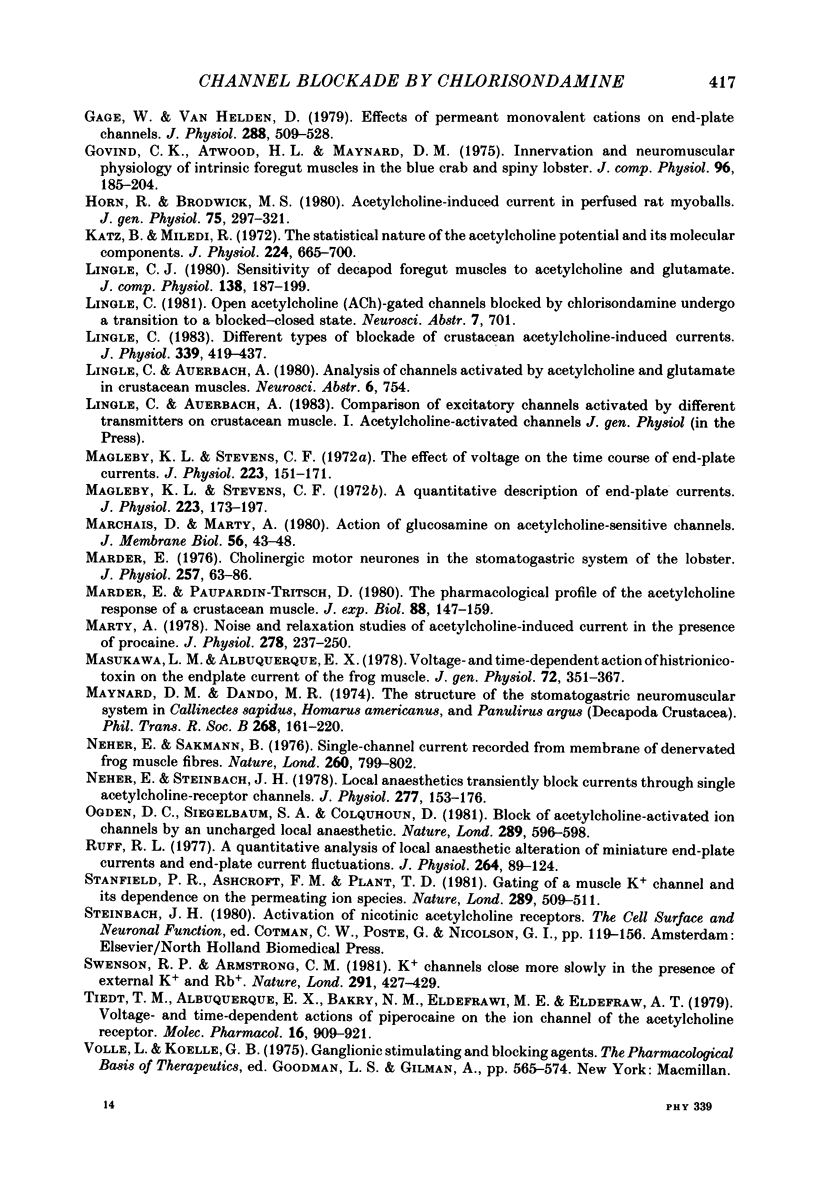
Selected References
These references are in PubMed. This may not be the complete list of references from this article.
- Adams P. R. Acetylcholine receptor kinetics. J Membr Biol. 1981 Feb 28;58(3):161–174. doi: 10.1007/BF01870902. [DOI] [PubMed] [Google Scholar]
- Adams P. R. Drug blockade of open end-plate channels. J Physiol. 1976 Sep;260(3):531–552. doi: 10.1113/jphysiol.1976.sp011530. [DOI] [PMC free article] [PubMed] [Google Scholar]
- Adams P. R., Feltz A. End-plate channel opening and the kinetics of quinacrine (mepacrine) block. J Physiol. 1980 Sep;306:283–306. doi: 10.1113/jphysiol.1980.sp013397. [DOI] [PMC free article] [PubMed] [Google Scholar]
- Adams P. R., Feltz A. Quinacrine (mepacrine) action at frog end-plate. J Physiol. 1980 Sep;306:261–281. doi: 10.1113/jphysiol.1980.sp013396. [DOI] [PMC free article] [PubMed] [Google Scholar]
- Adams P. R., Sakmann B. A comparison of current-voltage relations for full and partial agonists. J Physiol. 1978 Oct;283:621–644. doi: 10.1113/jphysiol.1978.sp012523. [DOI] [PMC free article] [PubMed] [Google Scholar]
- Adams P. R., Sakmann B. Decamethonium both opens and blocks endplate channels. Proc Natl Acad Sci U S A. 1978 Jun;75(6):2994–2998. doi: 10.1073/pnas.75.6.2994. [DOI] [PMC free article] [PubMed] [Google Scholar]
- Adams P. R. Voltage jump analysis of procaine action at frog end-plate. J Physiol. 1977 Jun;268(2):291–318. doi: 10.1113/jphysiol.1977.sp011858. [DOI] [PMC free article] [PubMed] [Google Scholar]
- Adler M., Oliveira A. C., Albuquerque E. X., Mansour N. A., Eldefrawi A. T. Reaction of tetraethylammonium with the open and closed conformations of the acetylcholine receptor ionic channel complex. J Gen Physiol. 1979 Jul;74(1):129–152. doi: 10.1085/jgp.74.1.129. [DOI] [PMC free article] [PubMed] [Google Scholar]
- Albuquerque E. X., Kuba K., Daly J. Effect of histrionicotoxin on the ionic conductance modulator of the cholinergic receptor: a quantitative analysis of the end-plate current. J Pharmacol Exp Ther. 1974 May;189(2):513–524. [PubMed] [Google Scholar]
- Anderson C. R., Stevens C. F. Voltage clamp analysis of acetylcholine produced end-plate current fluctuations at frog neuromuscular junction. J Physiol. 1973 Dec;235(3):655–691. doi: 10.1113/jphysiol.1973.sp010410. [DOI] [PMC free article] [PubMed] [Google Scholar]
- Armstrong C. M. Inactivation of the potassium conductance and related phenomena caused by quaternary ammonium ion injection in squid axons. J Gen Physiol. 1969 Nov;54(5):553–575. doi: 10.1085/jgp.54.5.553. [DOI] [PMC free article] [PubMed] [Google Scholar]
- Armstrong C. M. Interaction of tetraethylammonium ion derivatives with the potassium channels of giant axons. J Gen Physiol. 1971 Oct;58(4):413–437. doi: 10.1085/jgp.58.4.413. [DOI] [PMC free article] [PubMed] [Google Scholar]
- Ascher P., Large W. A., Rang H. P. Studies on the mechanism of action of acetylcholine antagonists on rat parasympathetic ganglion cells. J Physiol. 1979 Oct;295:139–170. doi: 10.1113/jphysiol.1979.sp012958. [DOI] [PMC free article] [PubMed] [Google Scholar]
- Ascher P., Marty A., Neild T. O. Life time and elementary conductance of the channels mediating the excitatory effects of acetylcholine in Aplysia neurones. J Physiol. 1978 May;278:177–206. doi: 10.1113/jphysiol.1978.sp012299. [DOI] [PMC free article] [PubMed] [Google Scholar]
- Ascher P., Marty A., Neild T. O. The mode of action of antagonists of the excitatory response to acetylcholine in Aplysia neurones. J Physiol. 1978 May;278:207–235. doi: 10.1113/jphysiol.1978.sp012300. [DOI] [PMC free article] [PubMed] [Google Scholar]
- Beam K. G. A quantitative description of end-plate currents in the presence of two lidocaine derivatives. J Physiol. 1976 Jun;258(2):301–322. doi: 10.1113/jphysiol.1976.sp011421. [DOI] [PMC free article] [PubMed] [Google Scholar]
- Beam K. G. A voltage-clamp study of the effect of two lidocaine derivatives on the time course of end-plate currents. J Physiol. 1976 Jun;258(2):279–300. doi: 10.1113/jphysiol.1976.sp011420. [DOI] [PMC free article] [PubMed] [Google Scholar]
- Colquhoun D., Dreyer F., Sheridan R. E. The actions of tubocurarine at the frog neuromuscular junction. J Physiol. 1979 Aug;293:247–284. doi: 10.1113/jphysiol.1979.sp012888. [DOI] [PMC free article] [PubMed] [Google Scholar]
- Farley J. M., Yeh J. Z., Watanabe S., Narahashi T. Endplate channel block by guanidine derivatives. J Gen Physiol. 1981 Mar;77(3):273–293. doi: 10.1085/jgp.77.3.273. [DOI] [PMC free article] [PubMed] [Google Scholar]
- Gage P. W., Van Helden D. Effects of permeant monovalent cations on end-plate channels. J Physiol. 1979 Mar;288:509–528. [PMC free article] [PubMed] [Google Scholar]
- Horn R., Brodwick M. S. Acetylcholine-induced current in perfused rat myoballs. J Gen Physiol. 1980 Mar;75(3):297–321. doi: 10.1085/jgp.75.3.297. [DOI] [PMC free article] [PubMed] [Google Scholar]
- Katz B., Miledi R. The statistical nature of the acetycholine potential and its molecular components. J Physiol. 1972 Aug;224(3):665–699. doi: 10.1113/jphysiol.1972.sp009918. [DOI] [PMC free article] [PubMed] [Google Scholar]
- Lingle C. Different types of blockade of crustacean acetylcholine-induced currents. J Physiol. 1983 Jun;339:419–437. doi: 10.1113/jphysiol.1983.sp014724. [DOI] [PMC free article] [PubMed] [Google Scholar]
- Magleby K. L., Stevens C. F. A quantitative description of end-plate currents. J Physiol. 1972 May;223(1):173–197. doi: 10.1113/jphysiol.1972.sp009840. [DOI] [PMC free article] [PubMed] [Google Scholar]
- Magleby K. L., Stevens C. F. The effect of voltage on the time course of end-plate currents. J Physiol. 1972 May;223(1):151–171. doi: 10.1113/jphysiol.1972.sp009839. [DOI] [PMC free article] [PubMed] [Google Scholar]
- Marchais D., Marty A. Action of glucosamine on acetylcholine-sensitive channels. J Membr Biol. 1980 Aug 21;56(1):43–48. doi: 10.1007/BF01869350. [DOI] [PubMed] [Google Scholar]
- Marder E. Cholinergic motor neurones in the stomatogastric system of the lobster. J Physiol. 1976 May;257(1):63–86. doi: 10.1113/jphysiol.1976.sp011356. [DOI] [PMC free article] [PubMed] [Google Scholar]
- Marder E., Paupardin-Tritsch D. The pharmacological profile of the acetylcholine response of a crustacean muscle. J Exp Biol. 1980 Oct;88:147–159. doi: 10.1242/jeb.88.1.147. [DOI] [PubMed] [Google Scholar]
- Marty A. Noise and relaxation studies of acetylcholine induced currents in the presence of procaine. J Physiol. 1978 May;278:237–250. doi: 10.1113/jphysiol.1978.sp012301. [DOI] [PMC free article] [PubMed] [Google Scholar]
- Masukawa L. M., Albuquerque E. X. Voltage- and time-dependent action of histrionicotoxin on the endplate current of the frog muscle. J Gen Physiol. 1978 Sep;72(3):351–367. doi: 10.1085/jgp.72.3.351. [DOI] [PMC free article] [PubMed] [Google Scholar]
- Maynard D. M., Dando M. R. The structure of the stomatogastric neuromuscular system in Callinectes sapidus, Homarus americanus and Panulirus argus (Decapoda Crustacea). Philos Trans R Soc Lond B Biol Sci. 1974 Aug 1;268(892):161–220. doi: 10.1098/rstb.1974.0024. [DOI] [PubMed] [Google Scholar]
- Neher E., Sakmann B. Single-channel currents recorded from membrane of denervated frog muscle fibres. Nature. 1976 Apr 29;260(5554):799–802. doi: 10.1038/260799a0. [DOI] [PubMed] [Google Scholar]
- Neher E., Steinbach J. H. Local anaesthetics transiently block currents through single acetylcholine-receptor channels. J Physiol. 1978 Apr;277:153–176. doi: 10.1113/jphysiol.1978.sp012267. [DOI] [PMC free article] [PubMed] [Google Scholar]
- Ogden D. C., Siegelbaum S. A., Colquhoun D. Block of acetylcholine-activated ion channels by an uncharged local anaesthetic. Nature. 1981 Feb 12;289(5798):596–598. doi: 10.1038/289596a0. [DOI] [PubMed] [Google Scholar]
- Ruff R. L. A quantitative analysis of local anaesthetic alteration of miniature end-plate currents and end-plate current fluctuations. J Physiol. 1977 Jan;264(1):89–124. doi: 10.1113/jphysiol.1977.sp011659. [DOI] [PMC free article] [PubMed] [Google Scholar]
- Stanfield P. R., Ashcroft F. M., Plant T. D. Gating of a muscle K+ channel and its dependence on the permeating ion species. Nature. 1981 Feb 5;289(5797):509–511. doi: 10.1038/289509a0. [DOI] [PubMed] [Google Scholar]
- Swenson R. P., Jr, Armstrong C. M. K+ channels close more slowly in the presence of external K+ and Rb+. Nature. 1981 Jun 4;291(5814):427–429. doi: 10.1038/291427a0. [DOI] [PubMed] [Google Scholar]
- Tiedt T. N., Albuquerque E. X., Bakry N. M., Eldefrawi M. E., Eldefrawi A. T. Voltage- and time-dependent actions of piperocaine on the ion channel of the acetylcholine receptor. Mol Pharmacol. 1979 Nov;16(3):909–921. [PubMed] [Google Scholar]