Abstract
K+-selective micropipettes were used to measure external K+ concentration [( K+]o) in the immediate vicinity of Purkinje cells in slices from guinea-pig cerebellum. The cells were either spontaneously active or were polarized via a separate intracellular micro-electrode. The level of [K+]o rose by 1-3 mM around the soma and dendrites of Purkinje cells during spike activity. The increases in [K+]o were usually greater during Ca2+-mediated spikes than during Na+-mediated spikes. This was even true at the soma where the Ca2+ spike only invaded electrotonically from the dendrites, in contrast to the Na+ spikes which were generated at the soma. No [K+]o changes were seen in the vicinity of Purkinje cells when the cells were hyperpolarized with current passage nor was any [K+]o change seen during subthreshold depolarizations. In glial cells, however, a hyperpolarizing current reduced [K+]o while a depolarizing current increased [K+]o in a symmetrical manner. When Ba2+ was substituted for Ca2+ in the bathing Ringer solution, prolonged plateau-potential spikes could be evoked from Purkinje cells. These spikes were accompanied by large [K+]o elevations but the plateau potentials outlasted the [K+]o elevations. These experiments suggest that large [K+]o increases can occur in the absence of Ca2+-mediated K+ conductances. Substitution of Mn2+ for Ca2+ in the Ringer solution removed some of the [K+]o increases at the Purkinje cell soma. Addition of tetrodotoxin to normal Ringer solution also reduced, but did not abolish the [K+]o increases at the soma. These experiments confirmed that both Na+ and Ca2+ spikes were involved in the [K+]o change. The diffusion characteristics of the slices were determined by an ionophoretic method using tetramethylammonium and ion-selective micropipettes. The extracellular volume fraction of the slice averaged 0.28 while the tortuosity averaged 1.84. These values were close to those found previously in the intact rat cerebellum. These data were used to make quantitative estimates of the expected [K+]o accumulation in the vicinity of a single cell (see Appendix). Such estimates showed reasonable agreement with the measured values. Our data show that quite large increases in [K+]o may occur around single Purkinje cells. Such increases have previously only been evident during the activation of cell populations in mammalian preparations. The present results are probably due to the superior recording conditions of the slice. Implications for intercellular communication are discussed.
Full text
PDF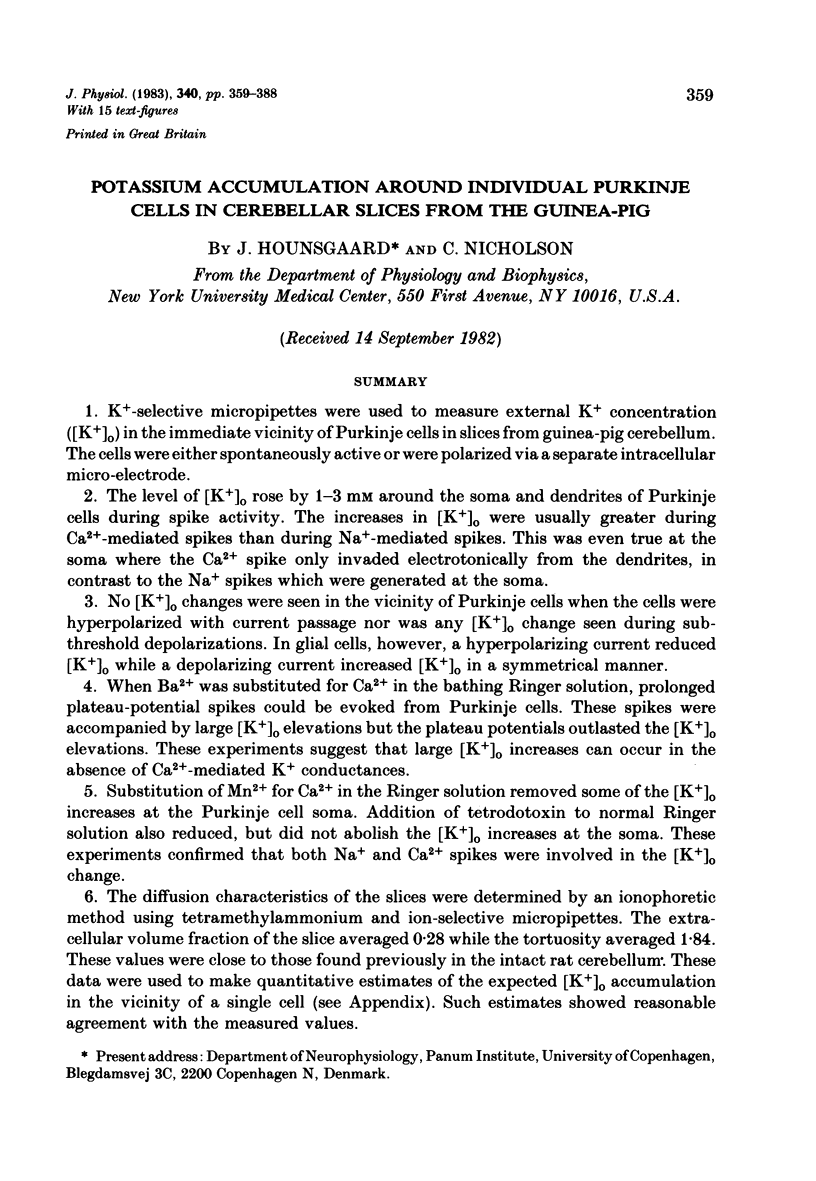
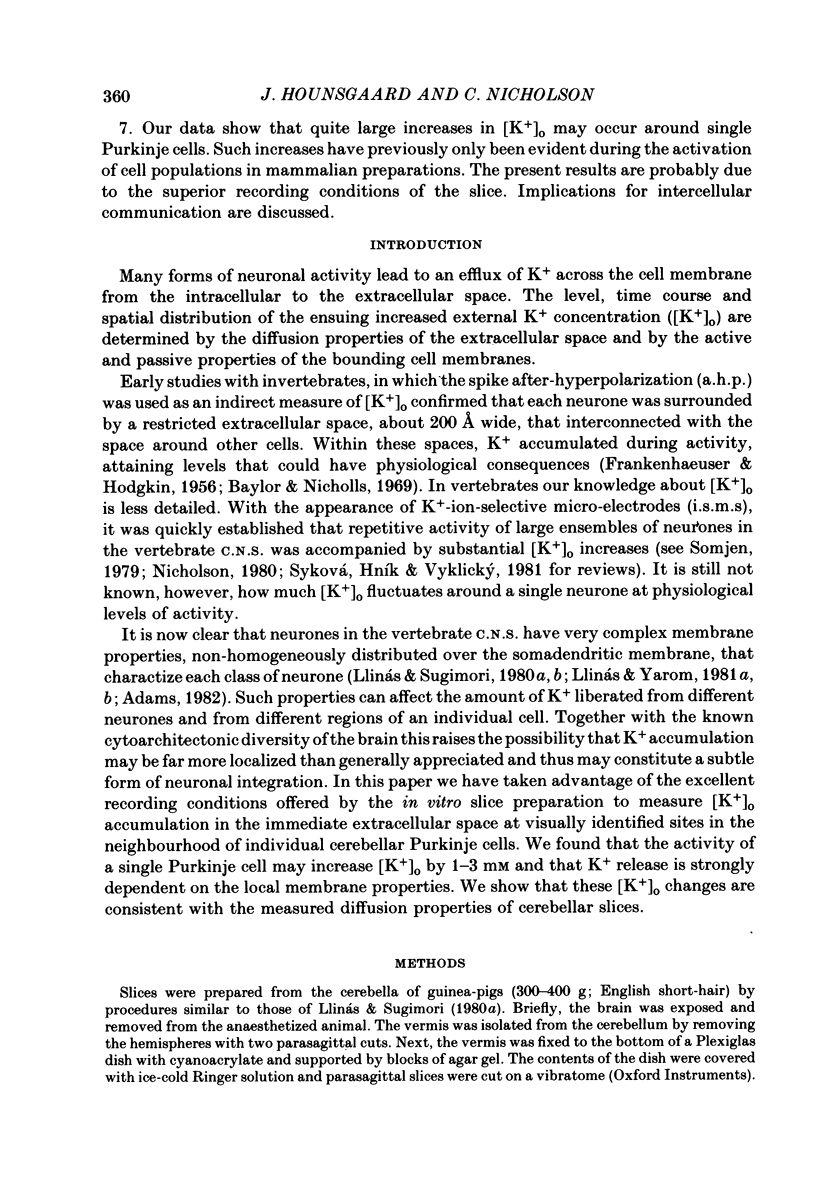
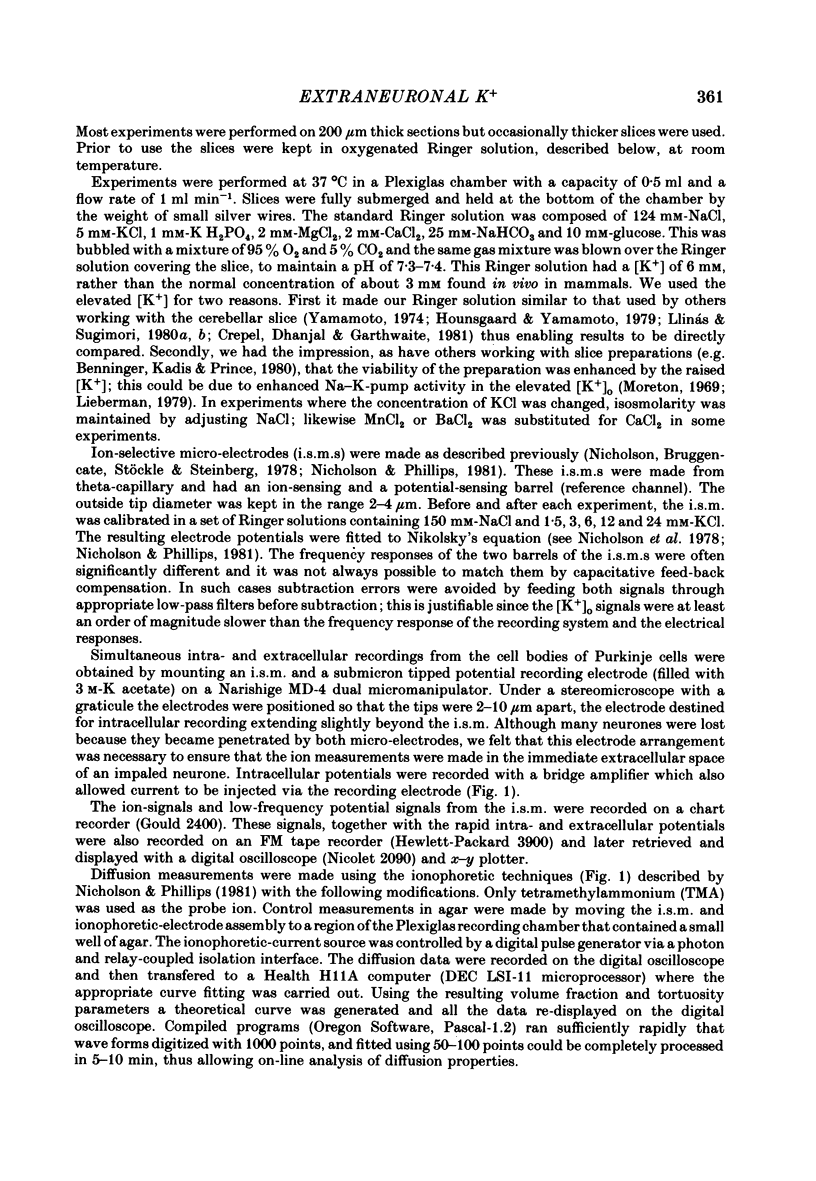
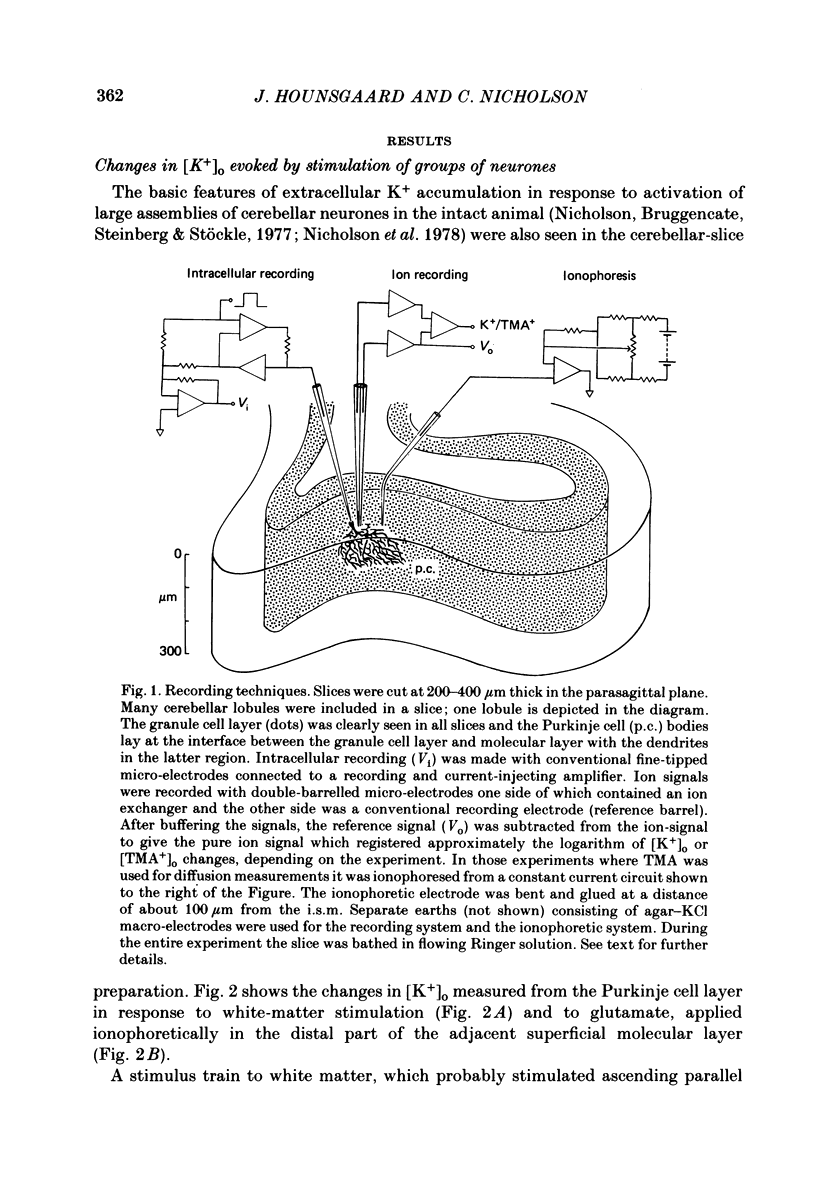
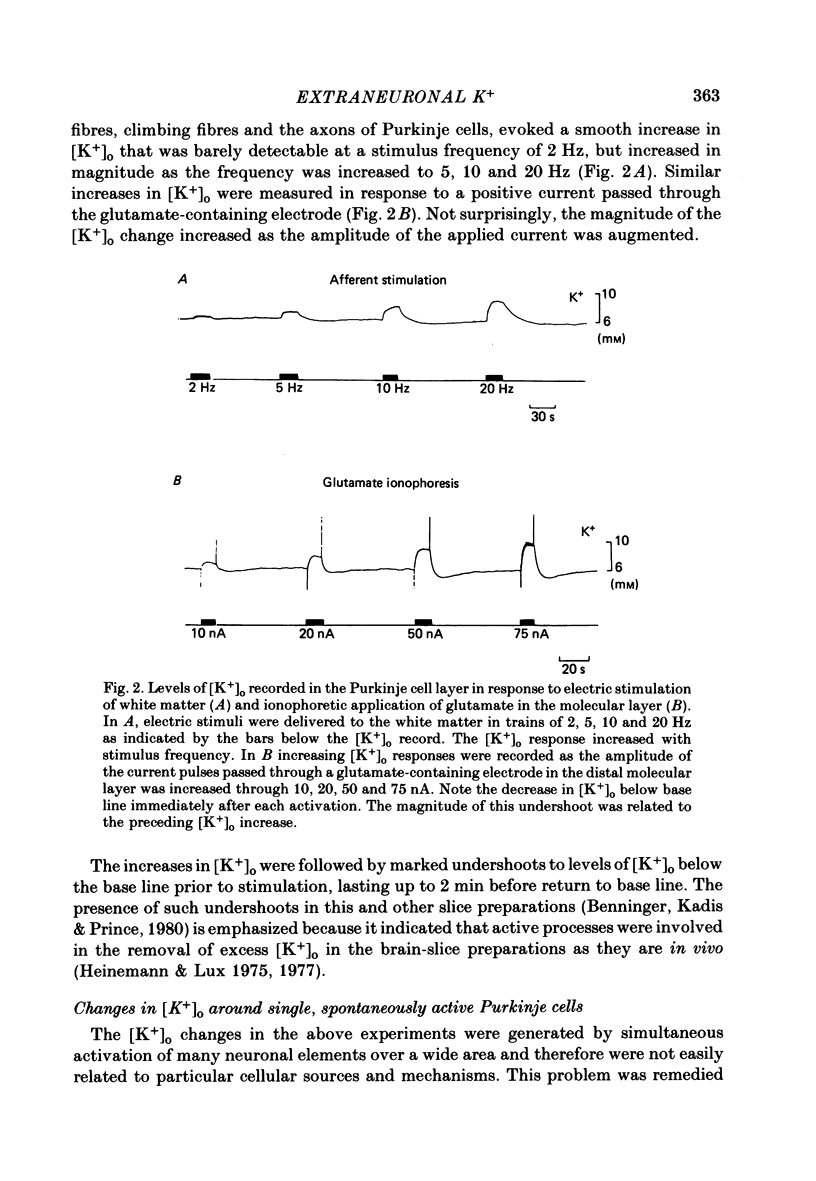
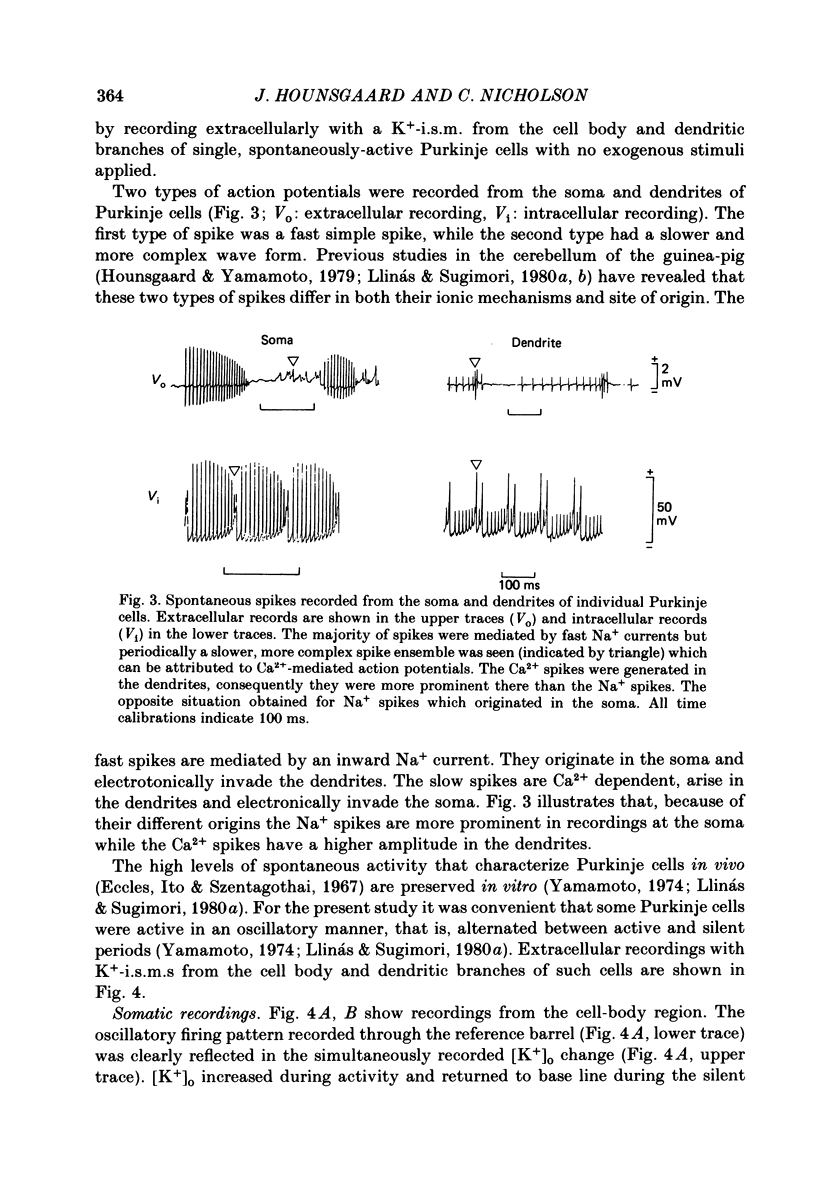
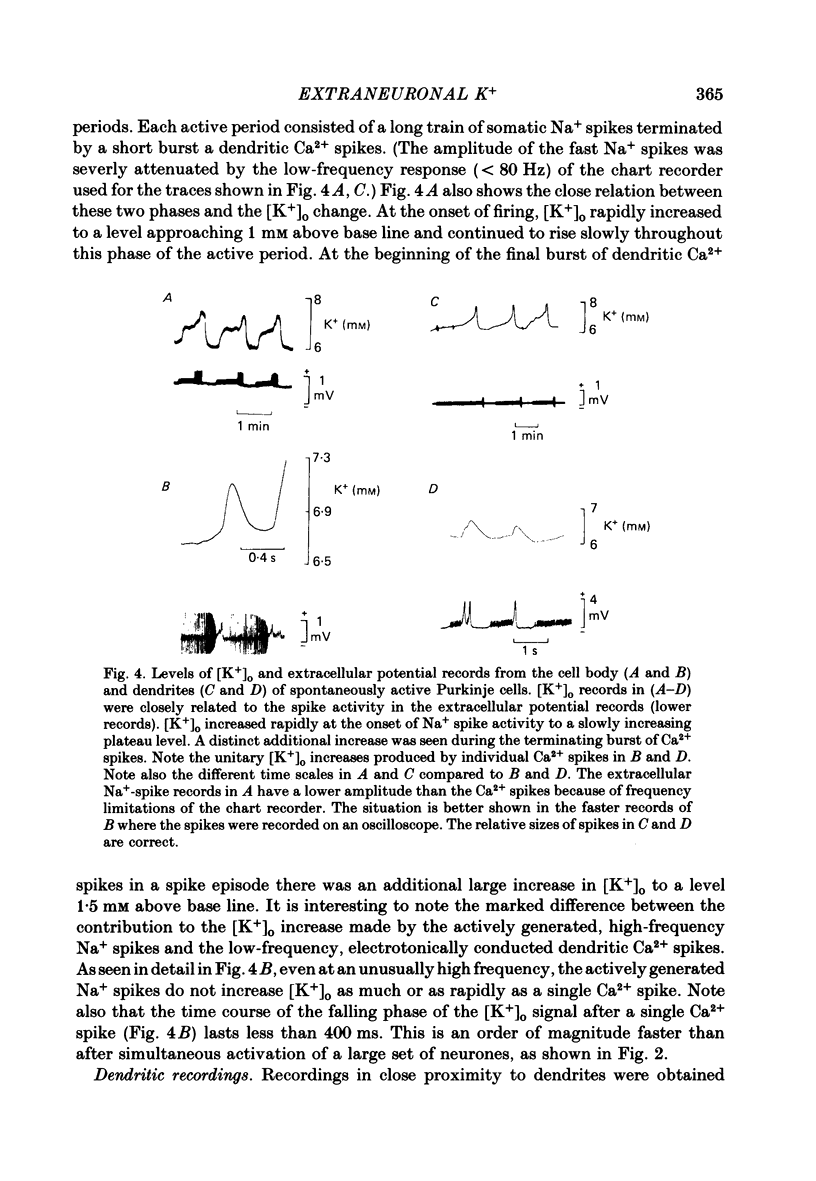
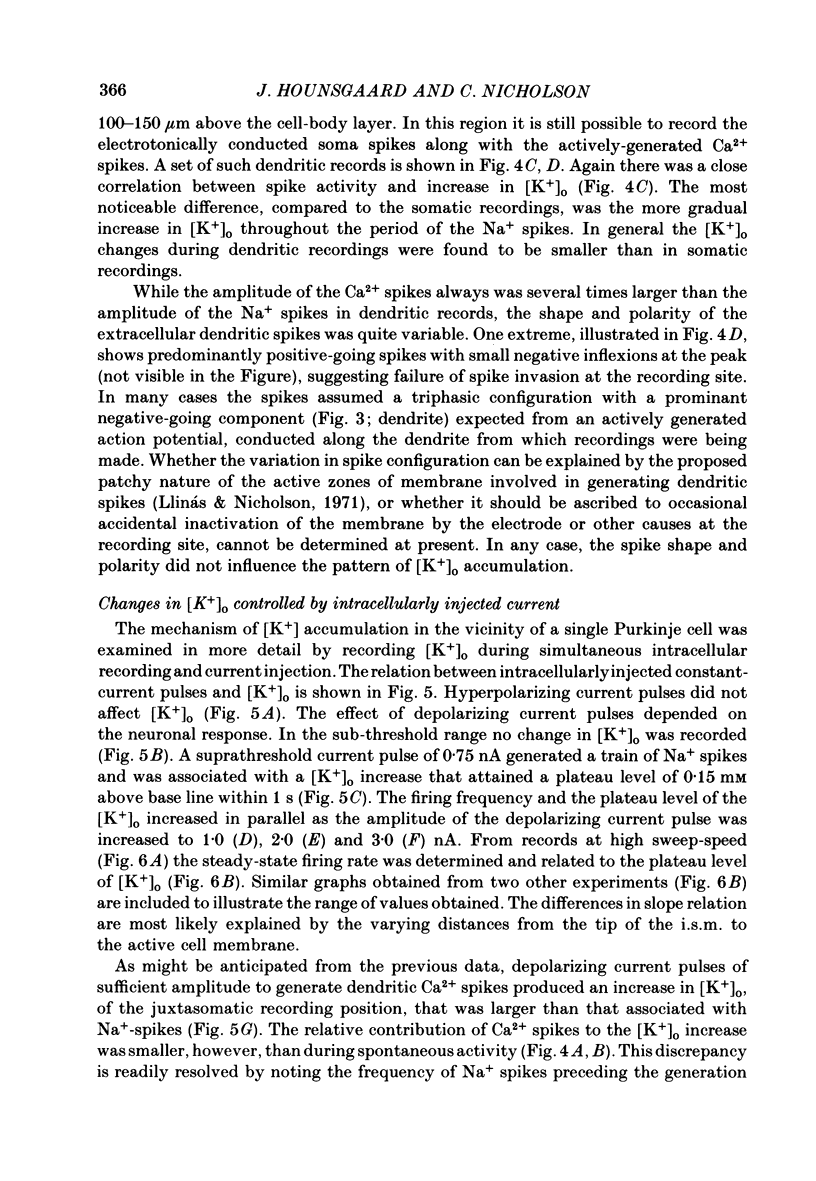
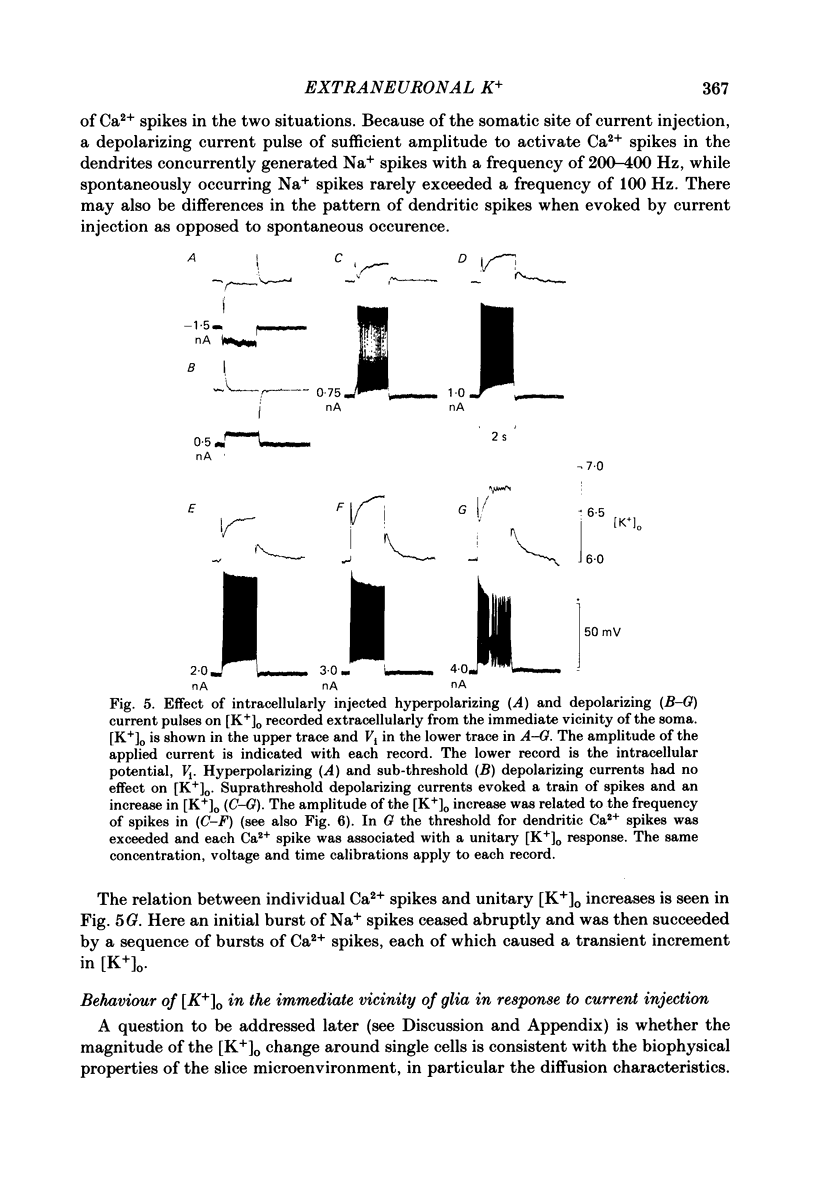
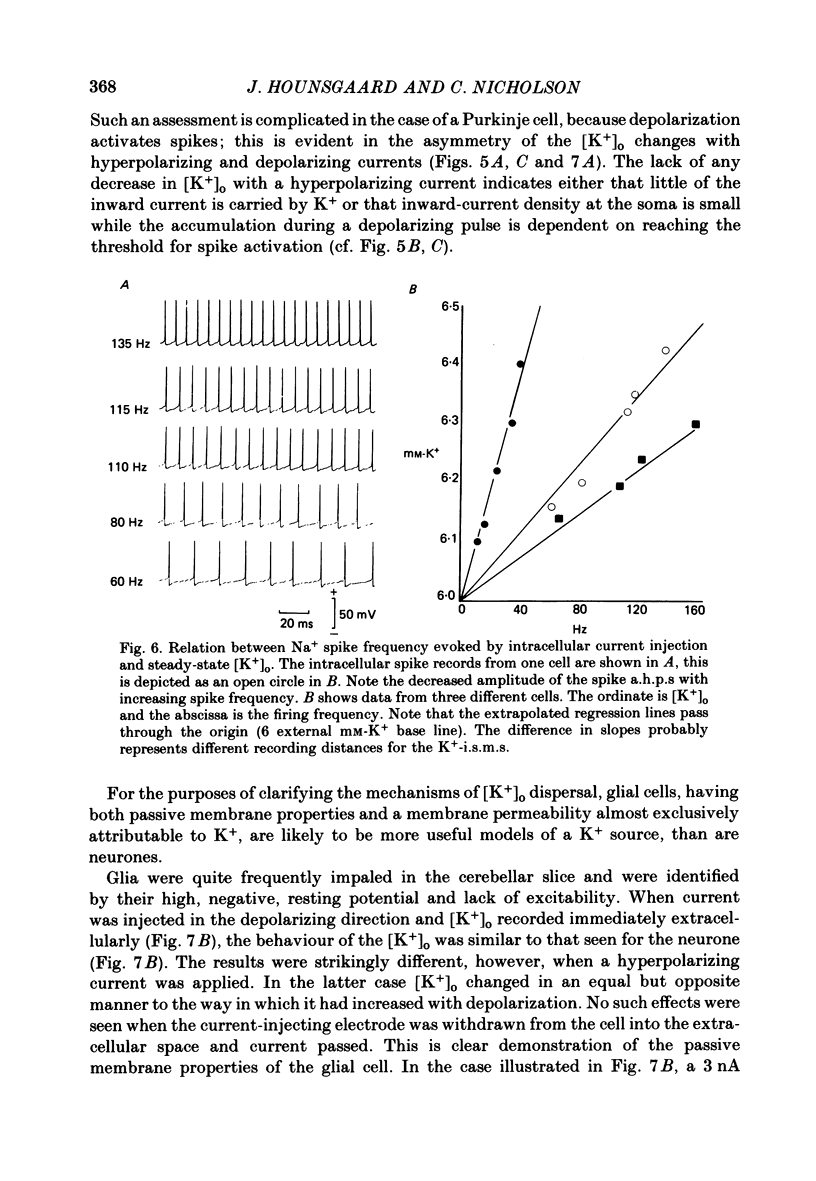
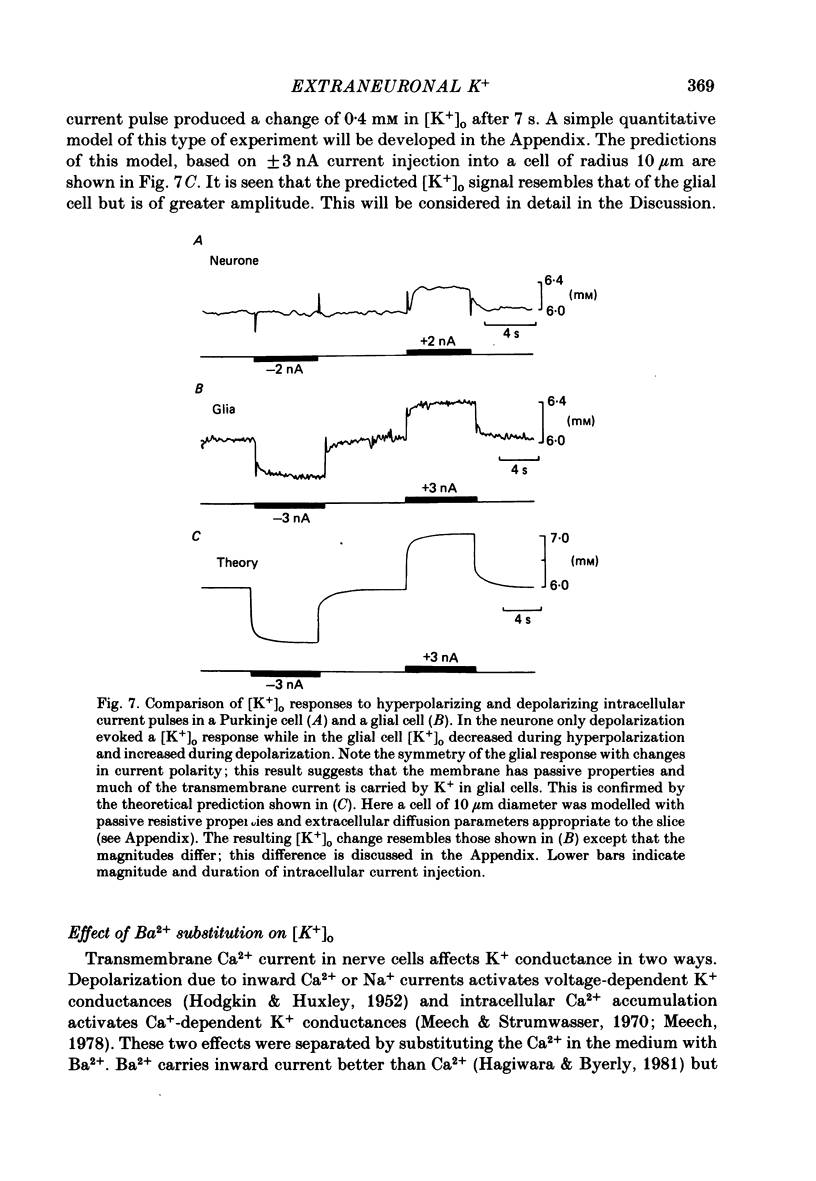
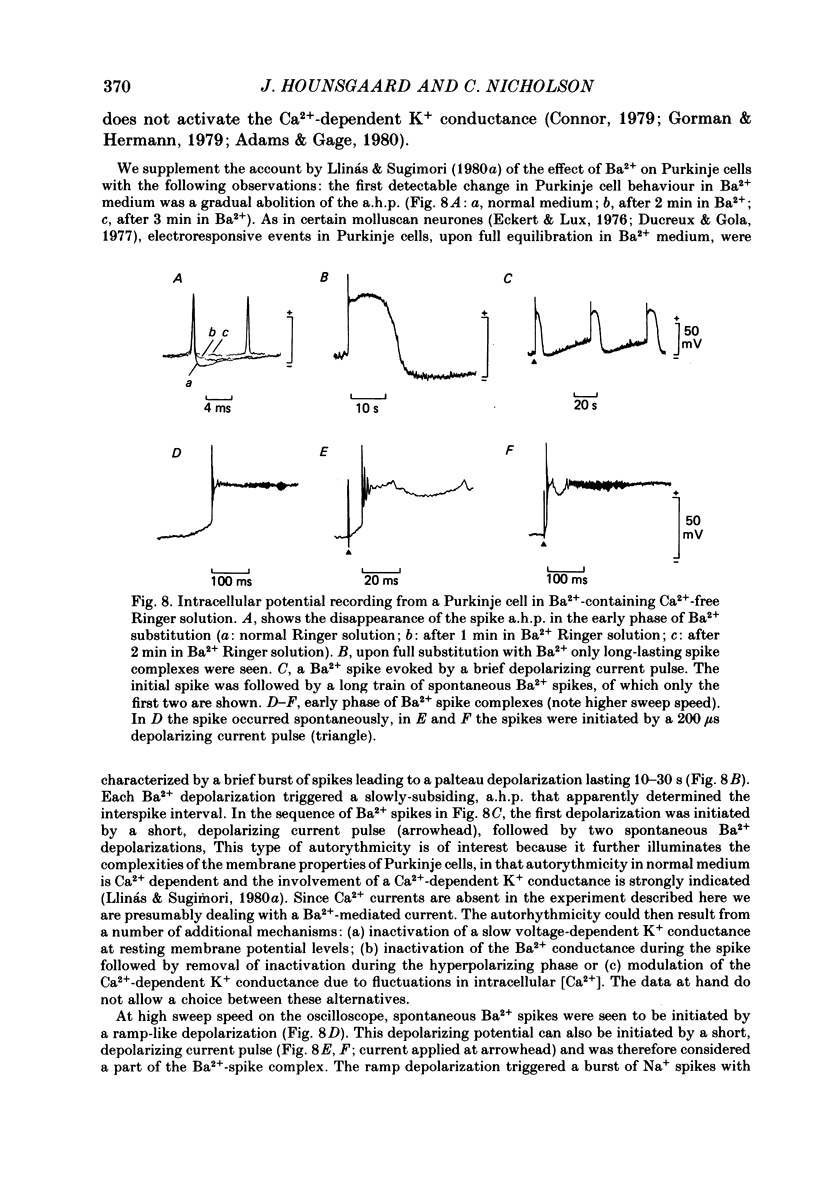
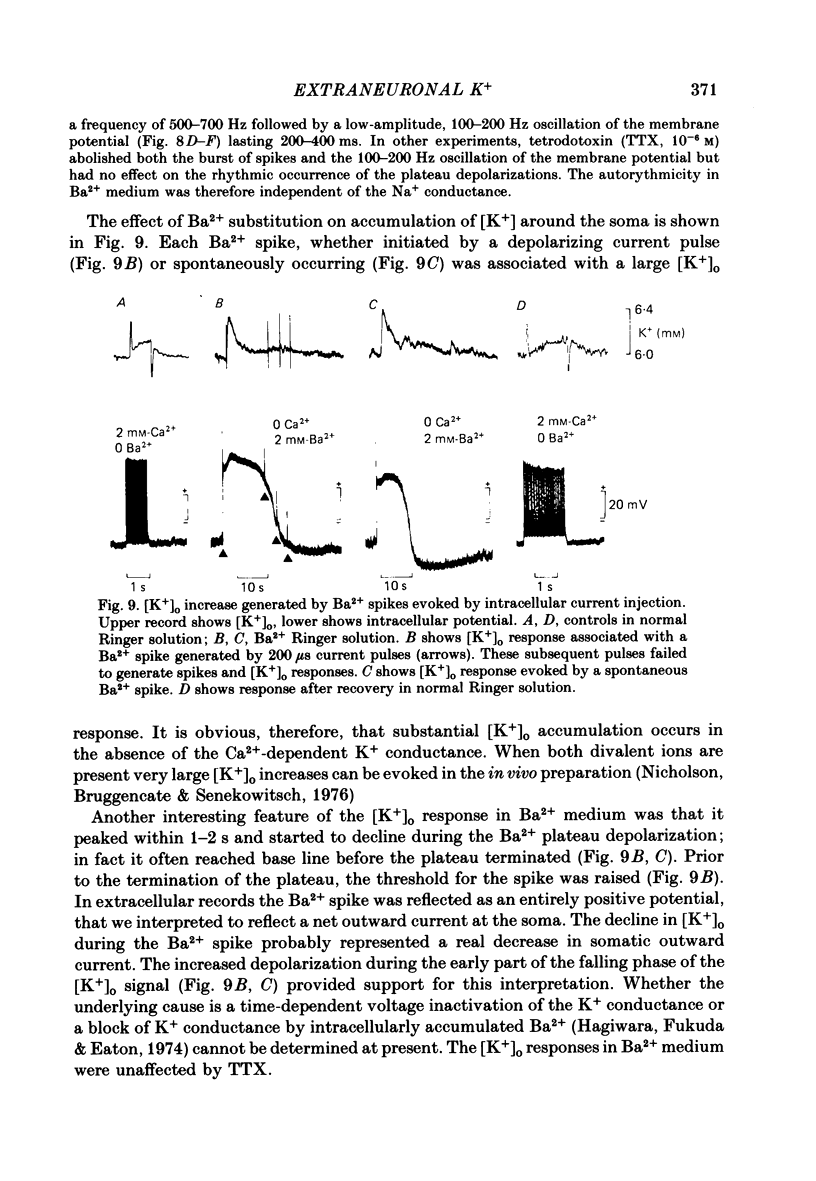
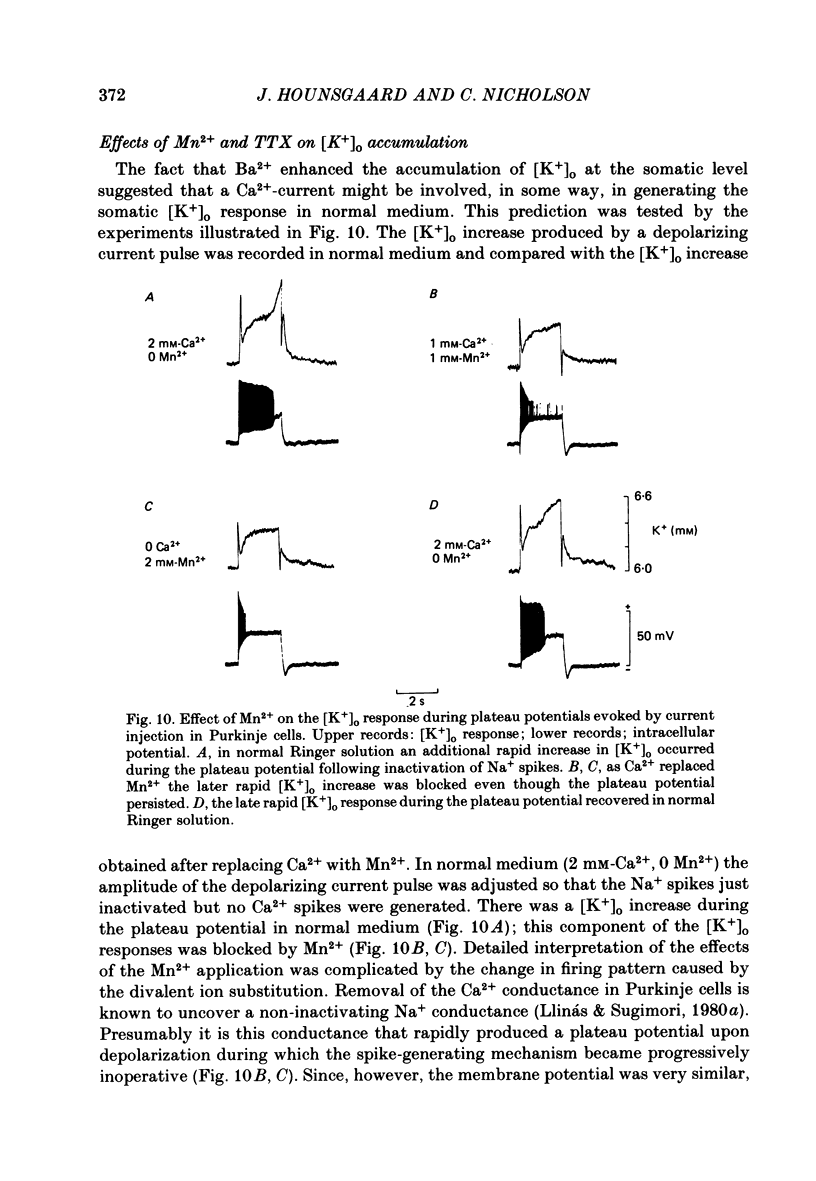
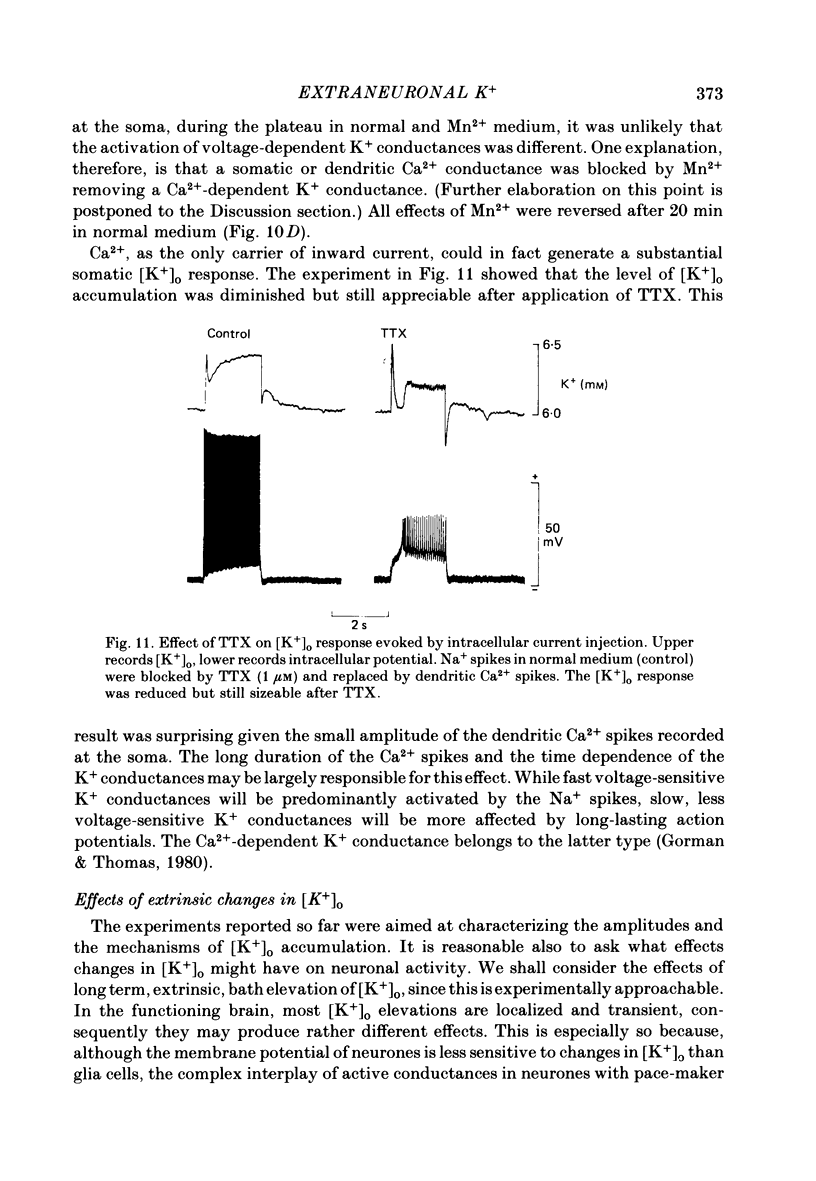
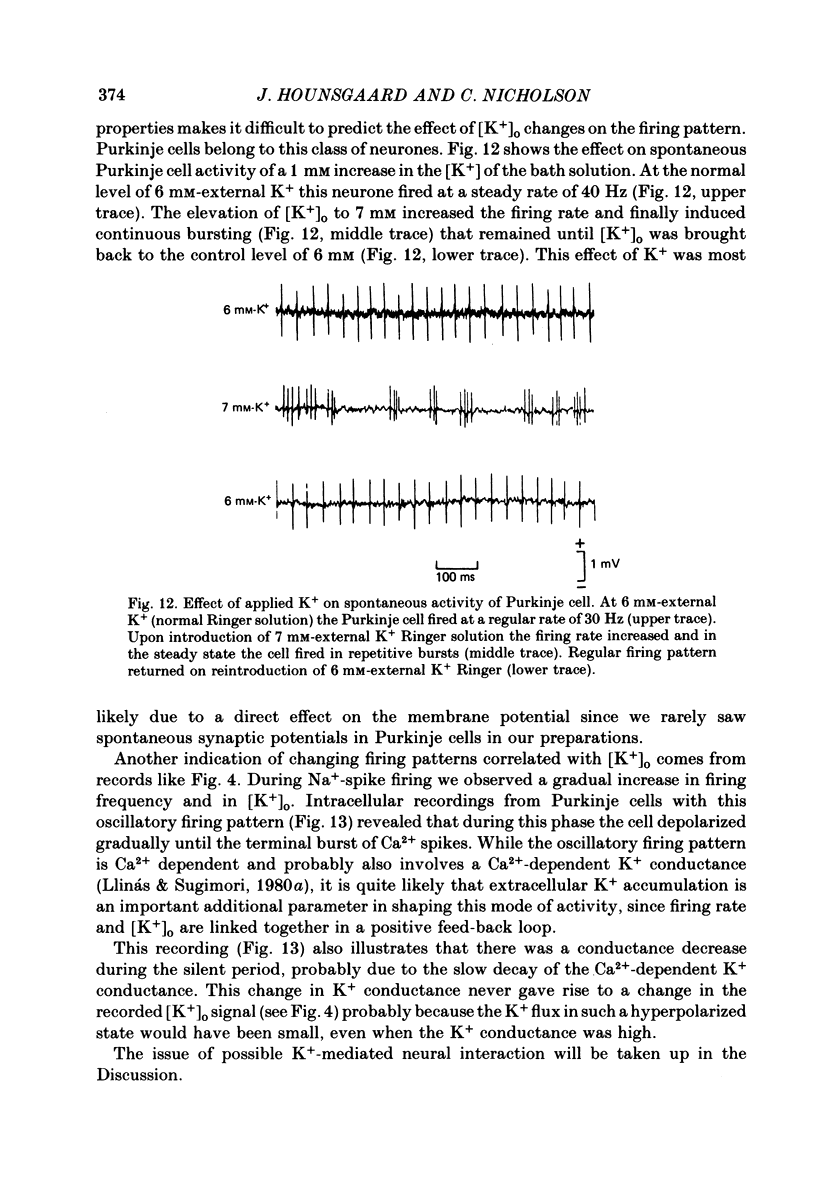
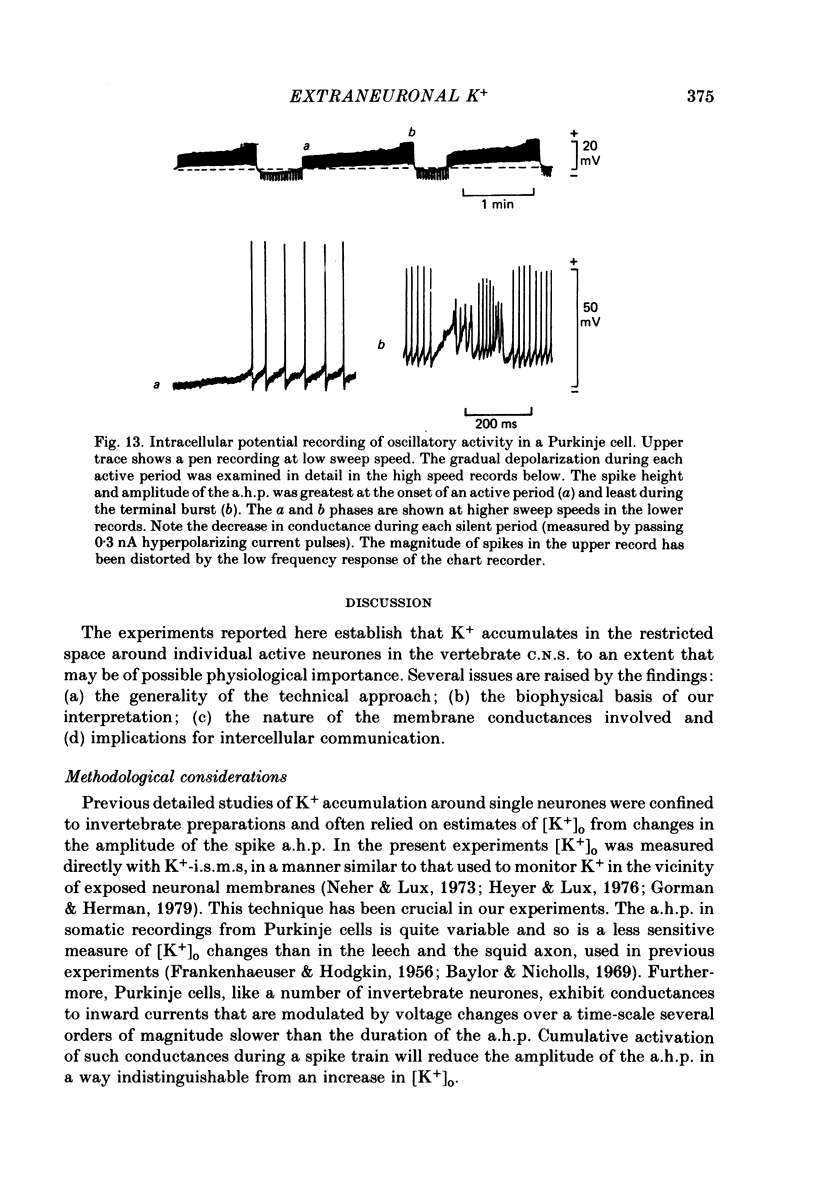
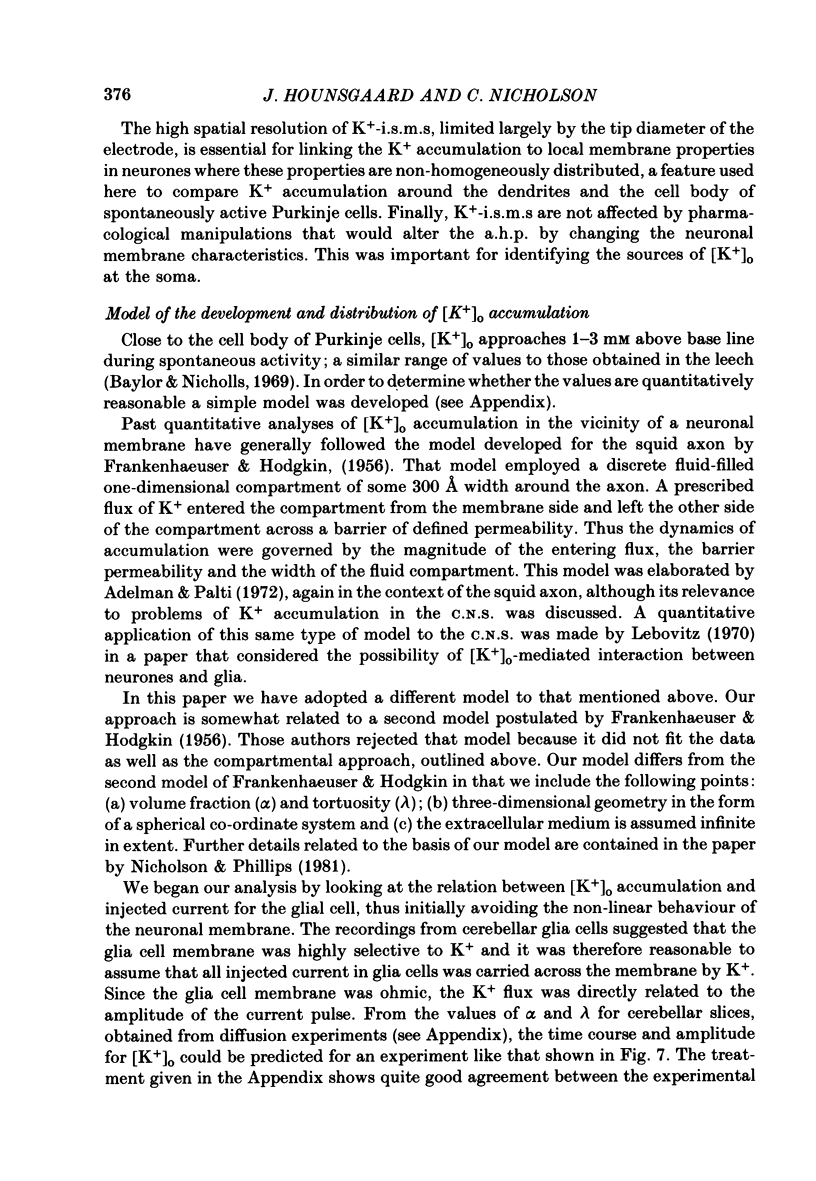
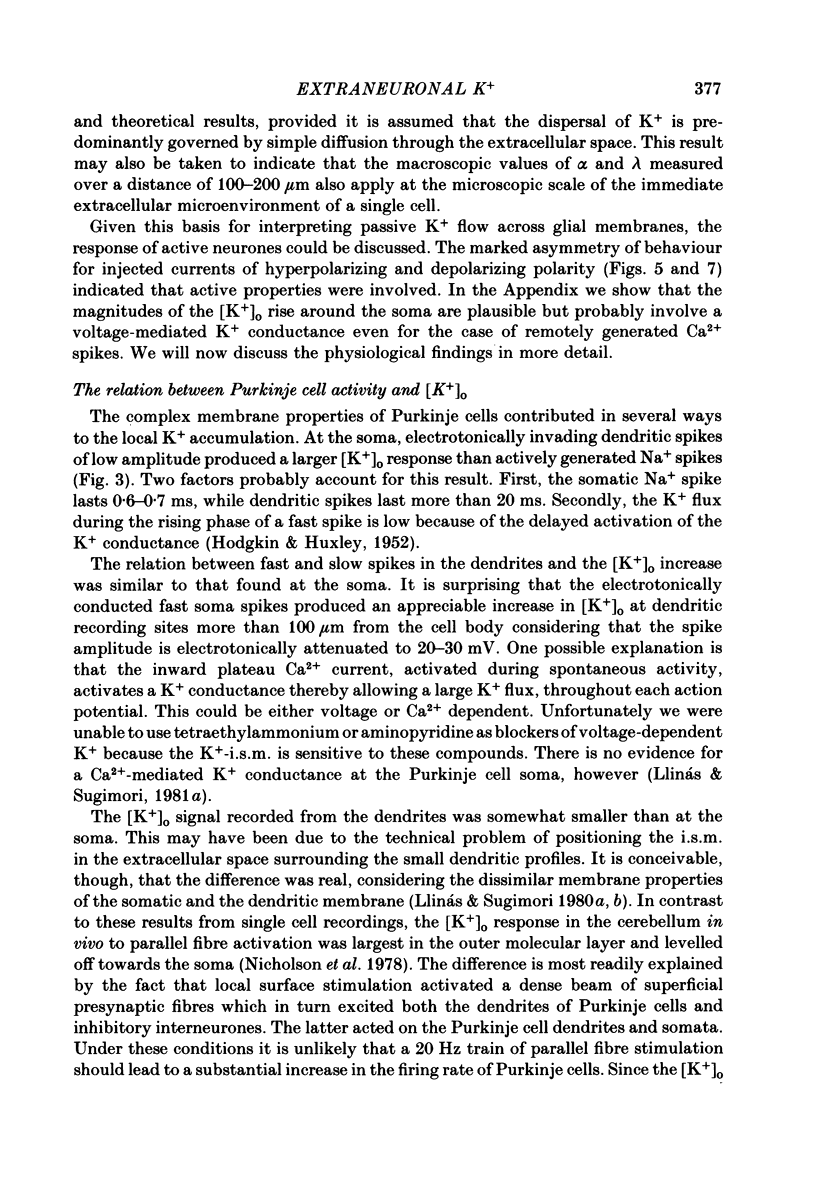
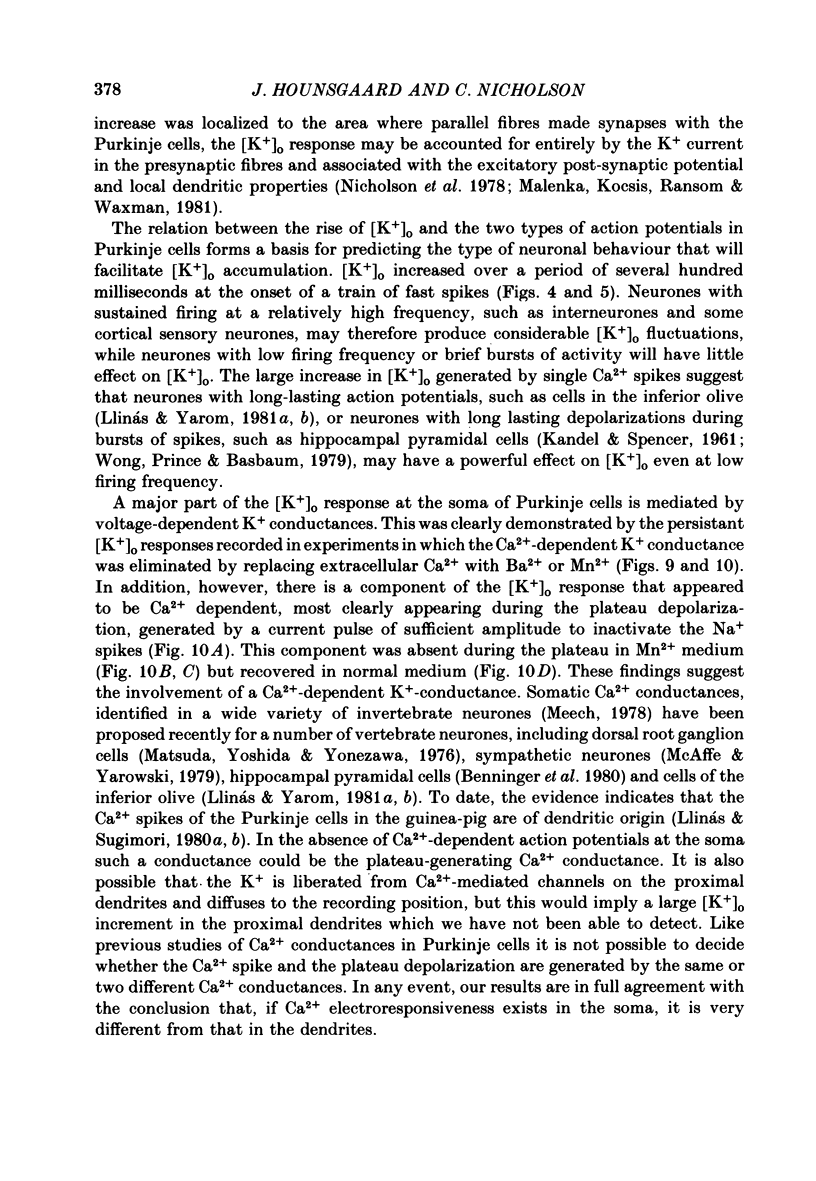
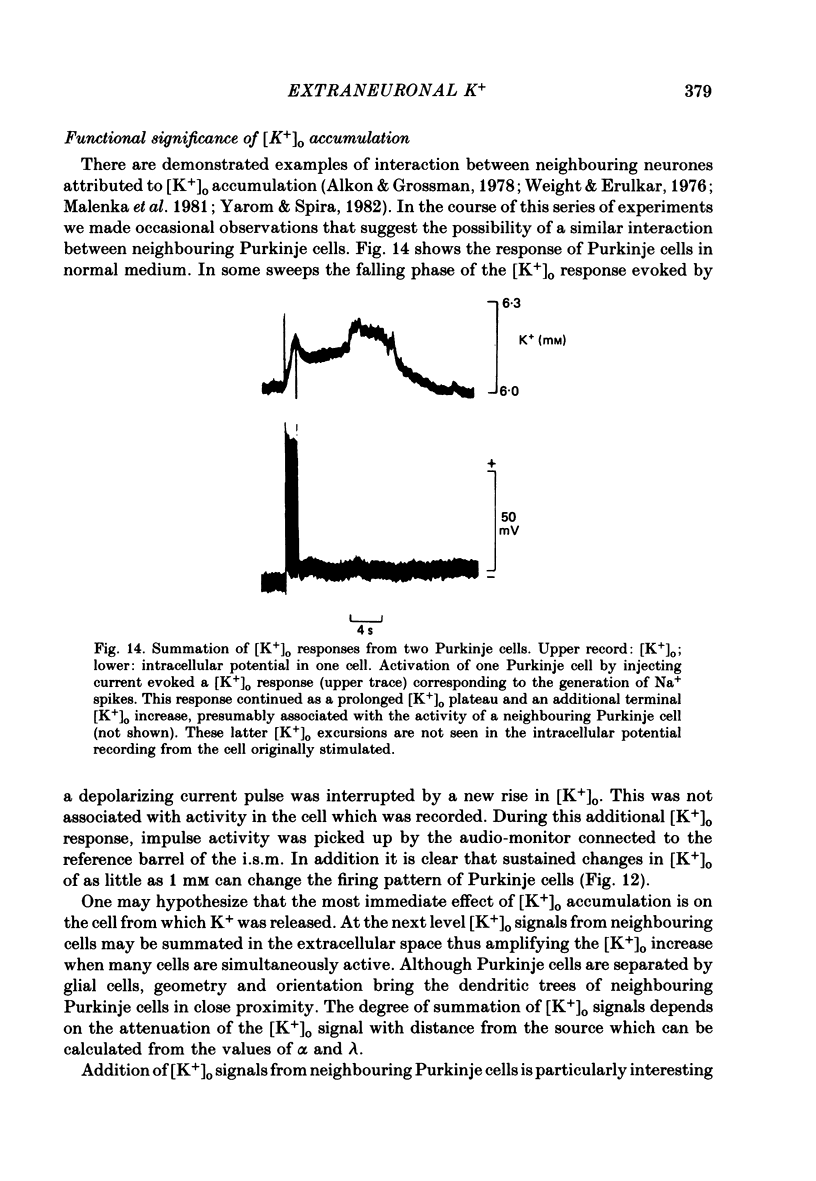
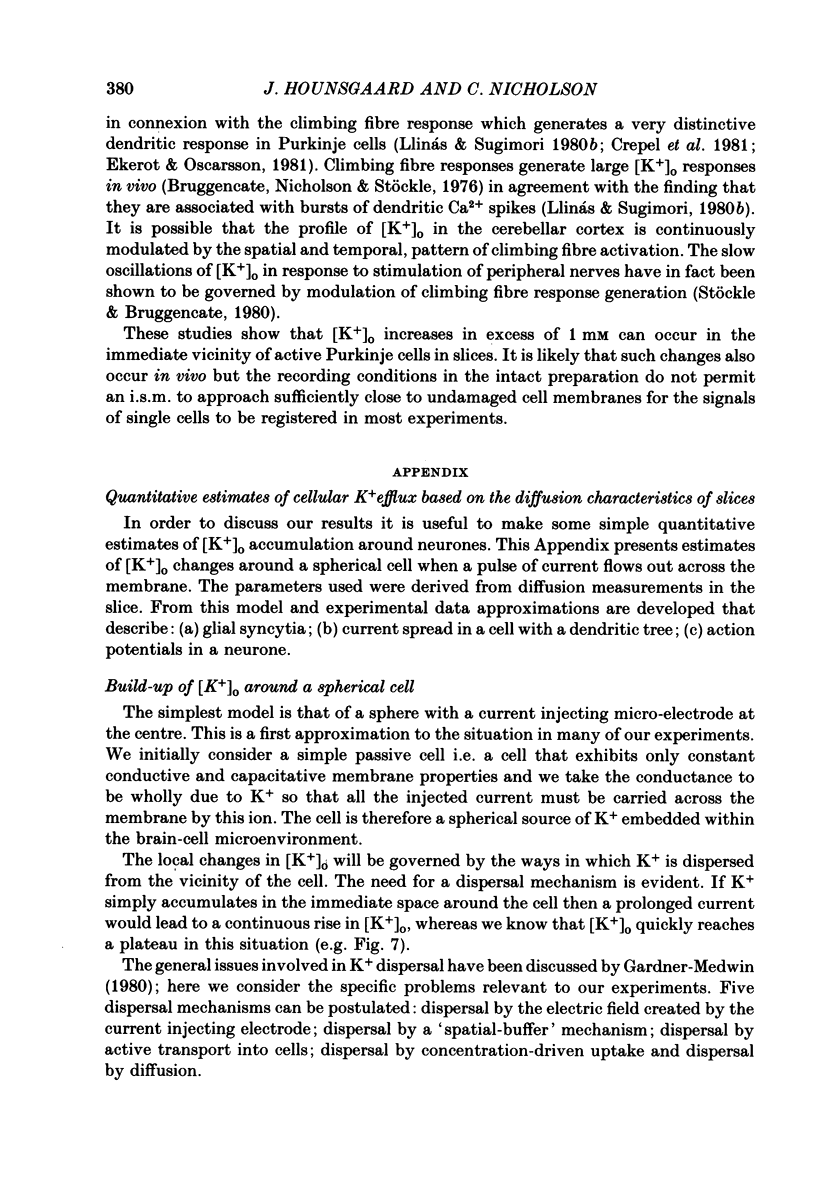
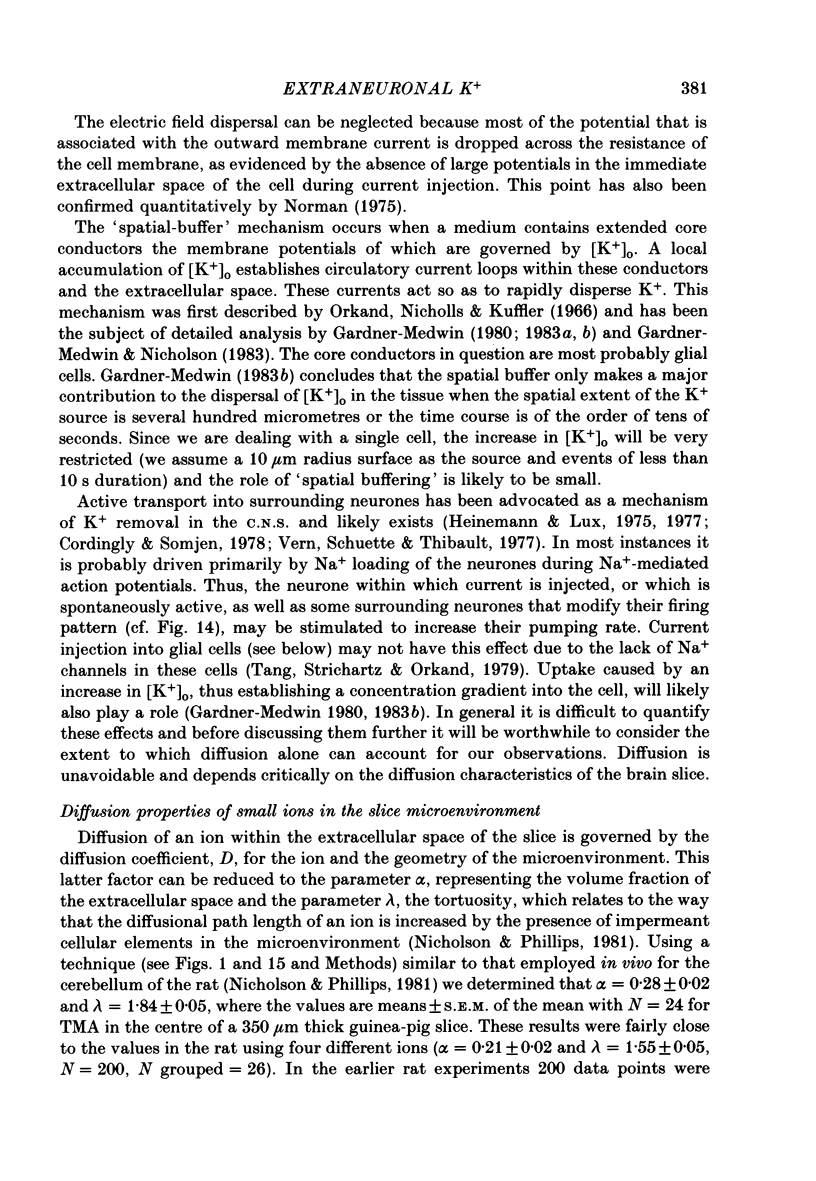
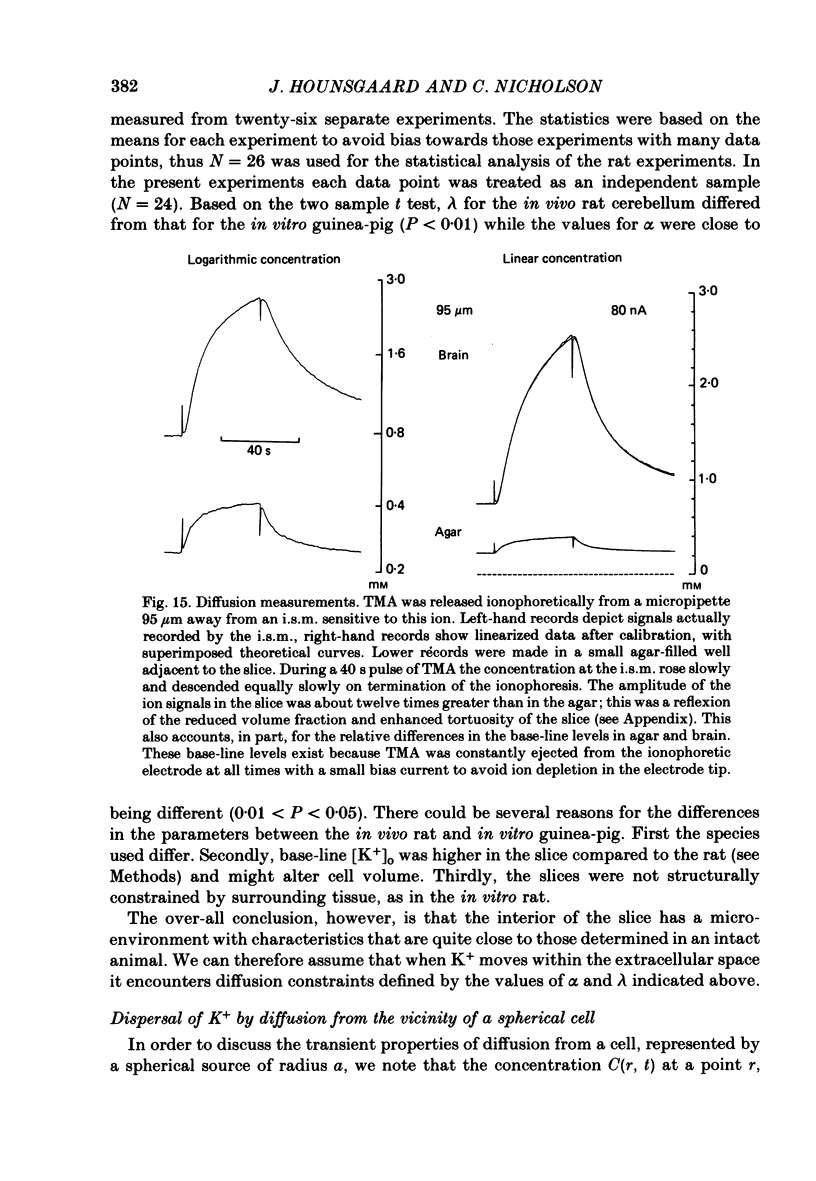
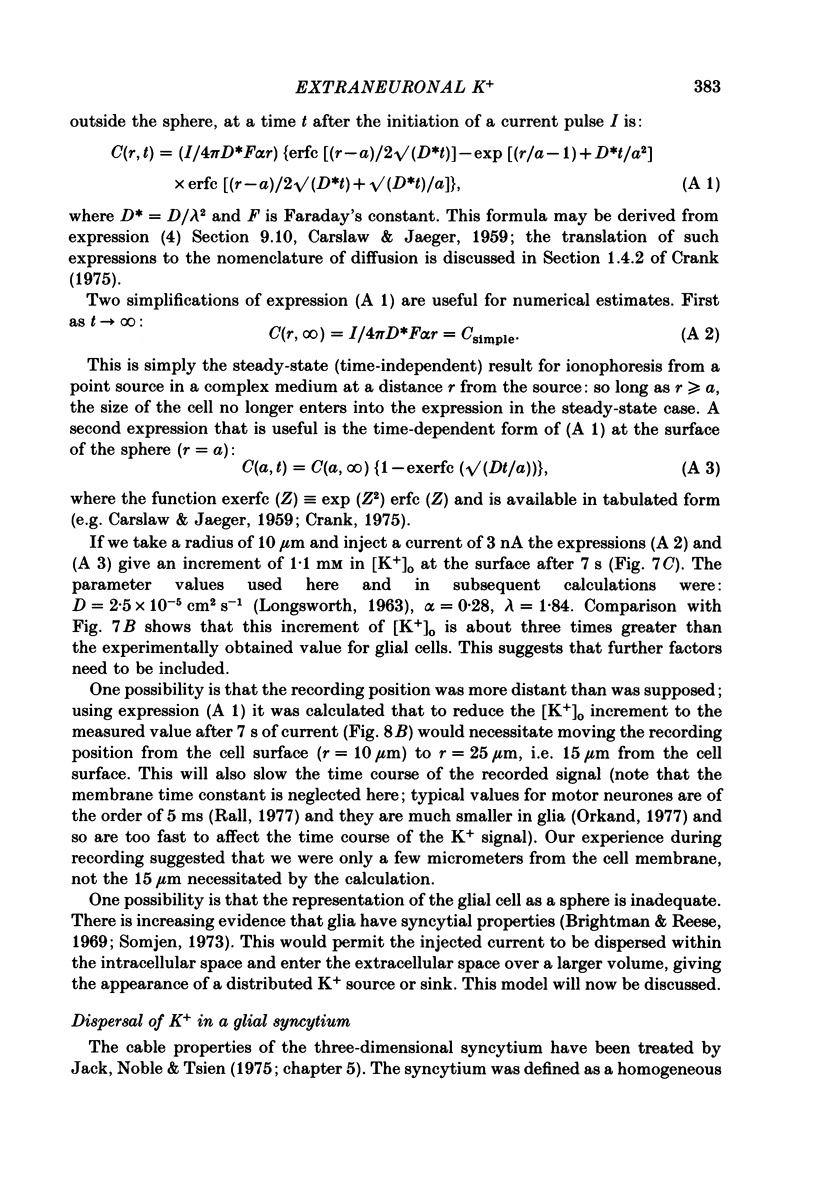
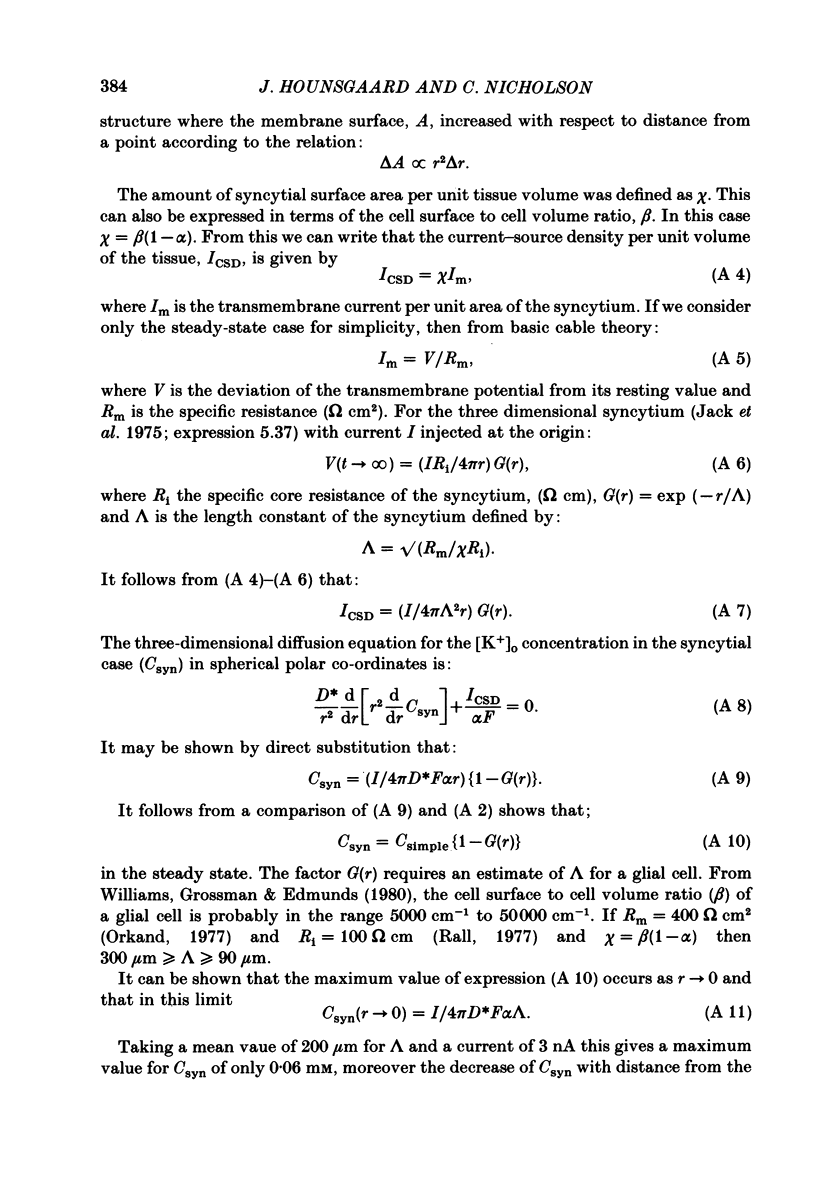
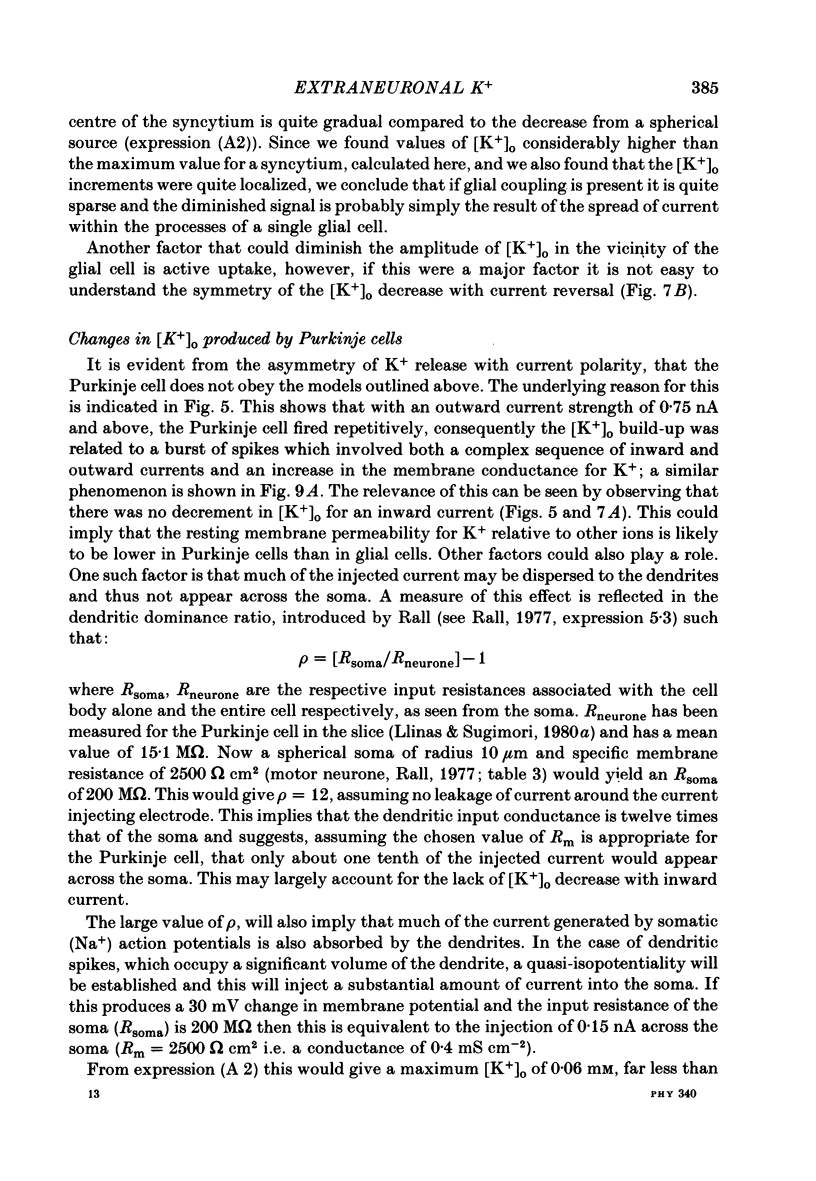
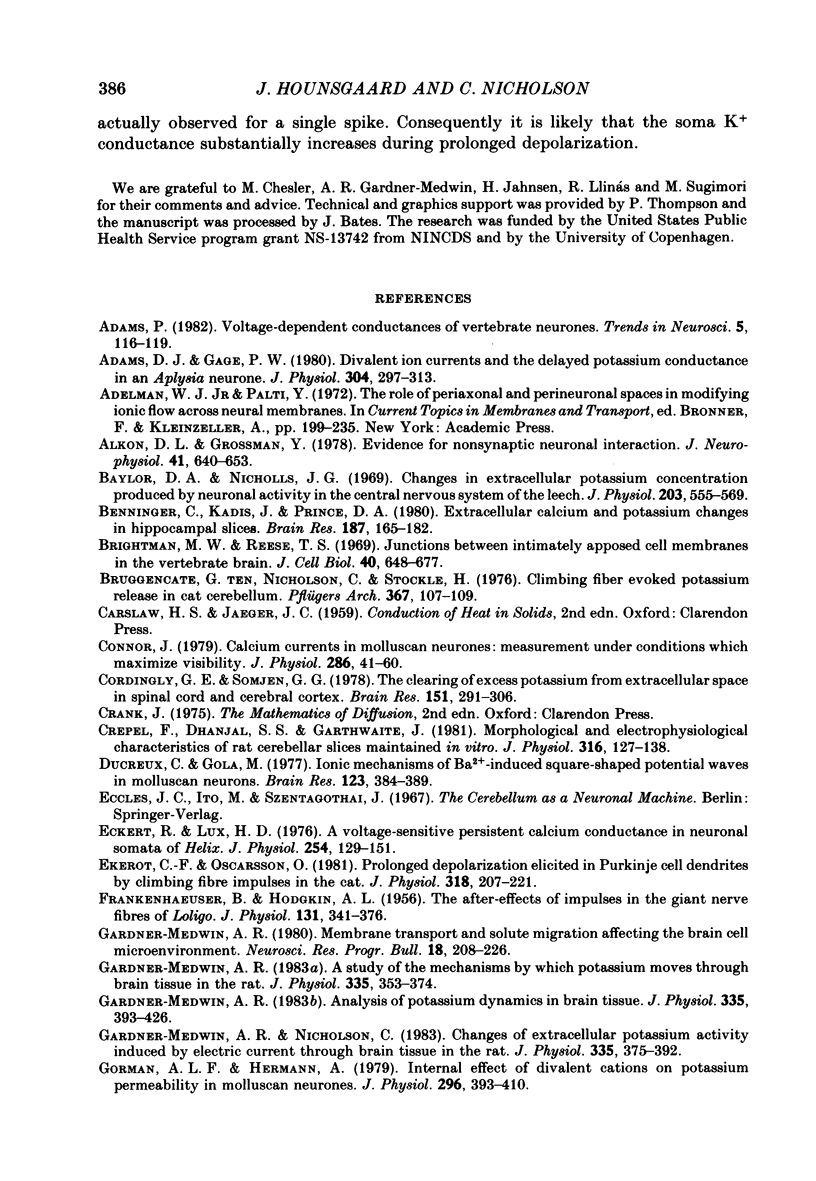
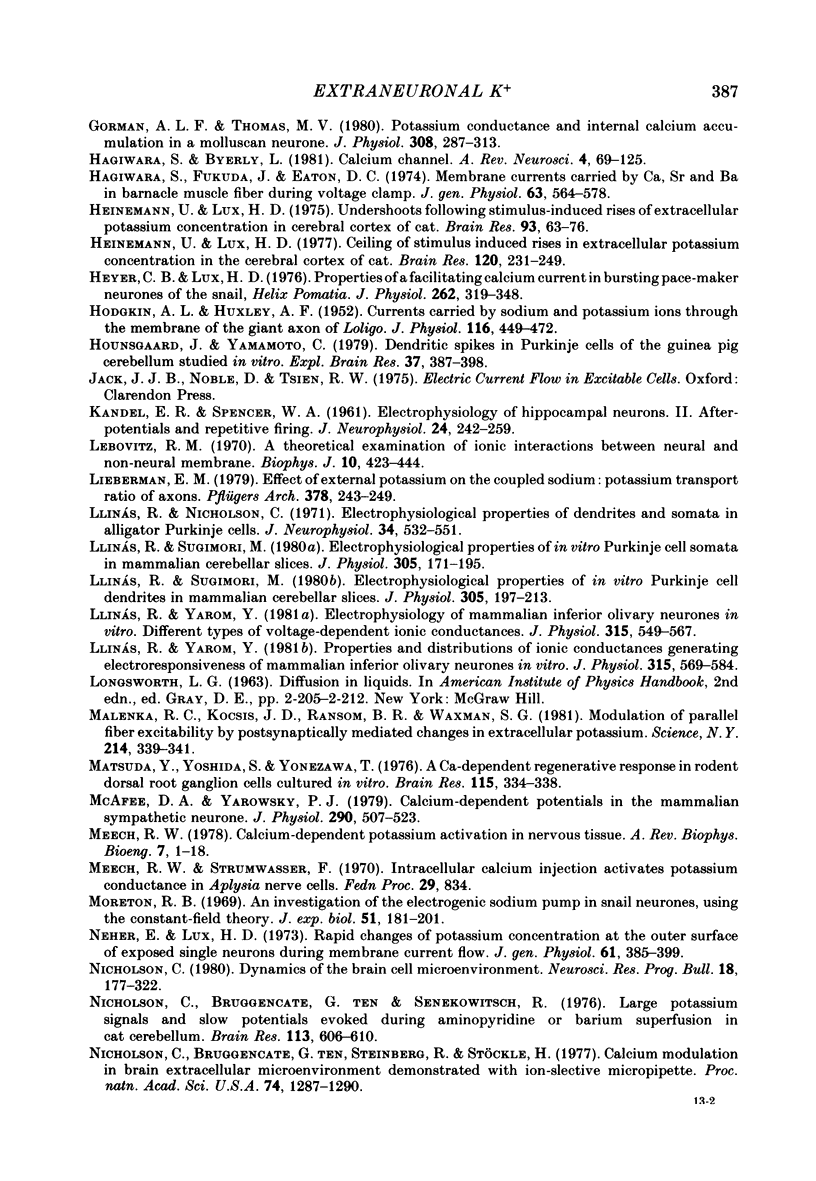
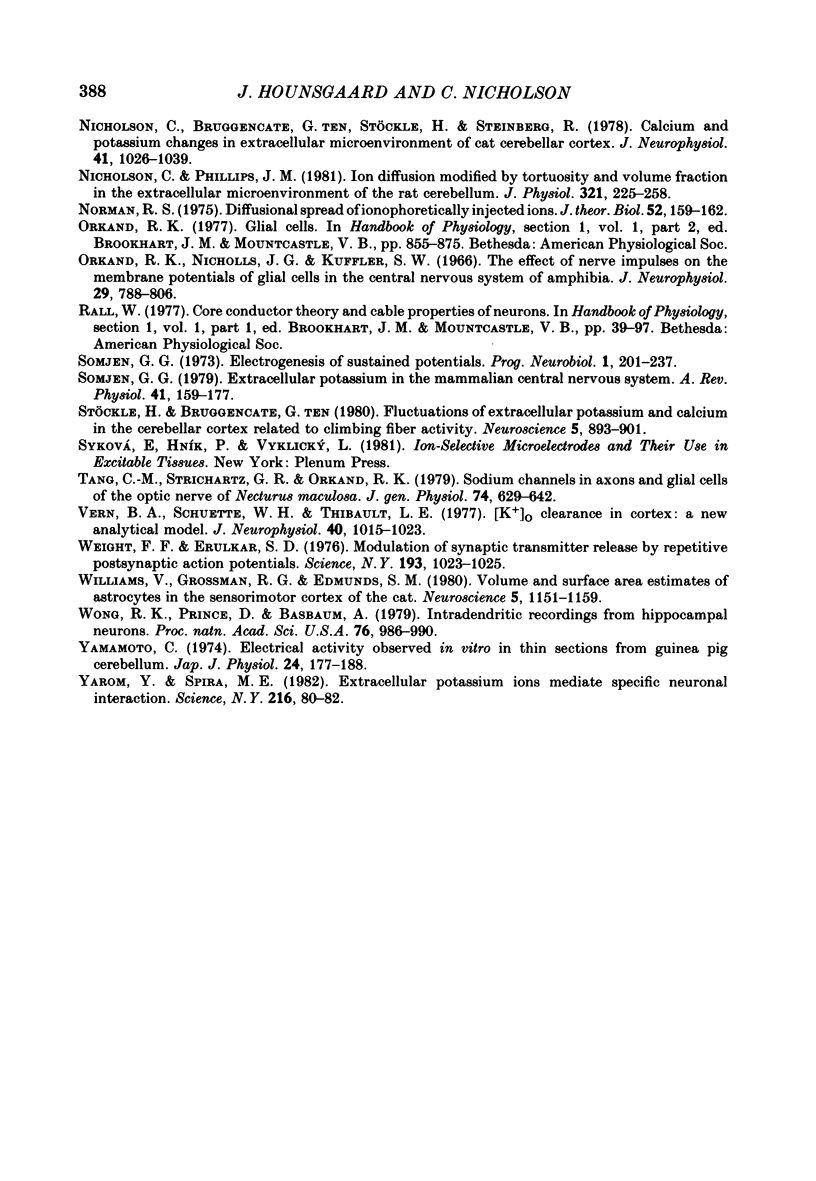
Selected References
These references are in PubMed. This may not be the complete list of references from this article.
- Adams D. J., Gage P. W. Divalent ion currents and the delayed potassium conductance in an Aplysia neurone. J Physiol. 1980 Jul;304:297–313. doi: 10.1113/jphysiol.1980.sp013325. [DOI] [PMC free article] [PubMed] [Google Scholar]
- Alkon D. L., Grossman Y. Evidence for nonsynaptic neuronal interaction. J Neurophysiol. 1978 May;41(3):640–653. doi: 10.1152/jn.1978.41.3.640. [DOI] [PubMed] [Google Scholar]
- Baylor D. A., Nicholls J. G. Changes in extracellular potassium concentration produced by neuronal activity in the central nervous system of the leech. J Physiol. 1969 Aug;203(3):555–569. doi: 10.1113/jphysiol.1969.sp008879. [DOI] [PMC free article] [PubMed] [Google Scholar]
- Benninger C., Kadis J., Prince D. A. Extracellular calcium and potassium changes in hippocampal slices. Brain Res. 1980 Apr 7;187(1):165–182. doi: 10.1016/0006-8993(80)90502-8. [DOI] [PubMed] [Google Scholar]
- Brightman M. W., Reese T. S. Junctions between intimately apposed cell membranes in the vertebrate brain. J Cell Biol. 1969 Mar;40(3):648–677. doi: 10.1083/jcb.40.3.648. [DOI] [PMC free article] [PubMed] [Google Scholar]
- Bruggencate G. T., Nicholson C., Stöckle H. Climbing fiber evoked potassium release in cat cerebellum. Pflugers Arch. 1976 Nov 30;367(1):107–109. doi: 10.1007/BF00583664. [DOI] [PubMed] [Google Scholar]
- Connor J. A. Calcium current in molluscan neurones: measurement under conditions which maximize its visibility. J Physiol. 1979 Jan;286:41–60. doi: 10.1113/jphysiol.1979.sp012606. [DOI] [PMC free article] [PubMed] [Google Scholar]
- Cordingley G. E., Somjen G. G. The clearing of excess potassium from extracellular space in spinal cord and cerebral cortex. Brain Res. 1978 Aug 4;151(2):291–306. doi: 10.1016/0006-8993(78)90886-7. [DOI] [PubMed] [Google Scholar]
- Crepel F., Dhanjal S. S., Garthwaite J. Morphological and electrophysiological characteristics of rat cerebellar slices maintained in vitro. J Physiol. 1981 Jul;316:127–138. doi: 10.1113/jphysiol.1981.sp013777. [DOI] [PMC free article] [PubMed] [Google Scholar]
- Ducreux C., Gola M. Ionic mechanism of Ba2+-induced square-shaped potential waves in molluscan neurons. Brain Res. 1977 Mar 11;123(2):384–389. doi: 10.1016/0006-8993(77)90491-7. [DOI] [PubMed] [Google Scholar]
- Eckert R., Lux H. D. A voltage-sensitive persistent calcium conductance in neuronal somata of Helix. J Physiol. 1976 Jan;254(1):129–151. doi: 10.1113/jphysiol.1976.sp011225. [DOI] [PMC free article] [PubMed] [Google Scholar]
- Ekerot C. F., Oscarsson O. Prolonged depolarization elicited in Purkinje cell dendrites by climbing fibre impulses in the cat. J Physiol. 1981 Sep;318:207–221. doi: 10.1113/jphysiol.1981.sp013859. [DOI] [PMC free article] [PubMed] [Google Scholar]
- FRANKENHAEUSER B., HODGKIN A. L. The after-effects of impulses in the giant nerve fibres of Loligo. J Physiol. 1956 Feb 28;131(2):341–376. doi: 10.1113/jphysiol.1956.sp005467. [DOI] [PMC free article] [PubMed] [Google Scholar]
- Gardner-Medwin A. R. A study of the mechanisms by which potassium moves through brain tissue in the rat. J Physiol. 1983 Feb;335:353–374. doi: 10.1113/jphysiol.1983.sp014539. [DOI] [PMC free article] [PubMed] [Google Scholar]
- Gardner-Medwin A. R. Analysis of potassium dynamics in mammalian brain tissue. J Physiol. 1983 Feb;335:393–426. doi: 10.1113/jphysiol.1983.sp014541. [DOI] [PMC free article] [PubMed] [Google Scholar]
- Gardner-Medwin A. R., Nicholson C. Changes of extracellular potassium activity induced by electric current through brain tissue in the rat. J Physiol. 1983 Feb;335:375–392. doi: 10.1113/jphysiol.1983.sp014540. [DOI] [PMC free article] [PubMed] [Google Scholar]
- Gorman A. L., Hermann A. Internal effects of divalent cations on potassium permeability in molluscan neurones. J Physiol. 1979 Nov;296:393–410. doi: 10.1113/jphysiol.1979.sp013012. [DOI] [PMC free article] [PubMed] [Google Scholar]
- Gorman A. L., Thomas M. V. Potassium conductance and internal calcium accumulation in a molluscan neurone. J Physiol. 1980 Nov;308:287–313. doi: 10.1113/jphysiol.1980.sp013472. [DOI] [PMC free article] [PubMed] [Google Scholar]
- HODGKIN A. L., HUXLEY A. F. Currents carried by sodium and potassium ions through the membrane of the giant axon of Loligo. J Physiol. 1952 Apr;116(4):449–472. doi: 10.1113/jphysiol.1952.sp004717. [DOI] [PMC free article] [PubMed] [Google Scholar]
- Hagiwara S., Byerly L. Calcium channel. Annu Rev Neurosci. 1981;4:69–125. doi: 10.1146/annurev.ne.04.030181.000441. [DOI] [PubMed] [Google Scholar]
- Hagiwara S., Fukuda J., Eaton D. C. Membrane currents carried by Ca, Sr, and Ba in barnacle muscle fiber during voltage clamp. J Gen Physiol. 1974 May;63(5):564–578. doi: 10.1085/jgp.63.5.564. [DOI] [PMC free article] [PubMed] [Google Scholar]
- Heinemann U., Lux H. D. Ceiling of stimulus induced rises in extracellular potassium concentration in the cerebral cortex of cat. Brain Res. 1977 Jan 21;120(2):231–249. doi: 10.1016/0006-8993(77)90903-9. [DOI] [PubMed] [Google Scholar]
- Heinemann U., Lux H. D. Undershoots following stimulus-induced rises of extracellular potassium concentration in cerebral cortex of cat. Brain Res. 1975 Jul 25;93(1):63–76. doi: 10.1016/0006-8993(75)90286-3. [DOI] [PubMed] [Google Scholar]
- Heyer C. B., Lux H. D. Properties of a facilitating calcium current in pace-maker neurones of the snail, Helix pomatia. J Physiol. 1976 Nov;262(2):319–348. doi: 10.1113/jphysiol.1976.sp011598. [DOI] [PMC free article] [PubMed] [Google Scholar]
- Hounsgaard J., Yamamoto C. Dendritic spikes in Purkinje cells of the guinea pig cerebellum studied in vitro. Exp Brain Res. 1979 Oct;37(2):387–398. doi: 10.1007/BF00237721. [DOI] [PubMed] [Google Scholar]
- KANDEL E. R., SPENCER W. A. Electrophysiology of hippocampal neurons. II. After-potentials and repetitive firing. J Neurophysiol. 1961 May;24:243–259. doi: 10.1152/jn.1961.24.3.243. [DOI] [PubMed] [Google Scholar]
- Lebovitz R. M. A theoretical examination of ionic interactions between neural and non-neural membranes. Biophys J. 1970 May;10(5):423–444. doi: 10.1016/S0006-3495(70)86310-X. [DOI] [PMC free article] [PubMed] [Google Scholar]
- Lieberman E. M. Effect of external potassium on the coupled sodium: potassium transport ratio of axons. Pflugers Arch. 1979 Jan 31;378(3):243–249. doi: 10.1007/BF00592742. [DOI] [PubMed] [Google Scholar]
- Llinas R., Nicholson C. Electrophysiological properties of dendrites and somata in alligator Purkinje cells. J Neurophysiol. 1971 Jul;34(4):532–551. doi: 10.1152/jn.1971.34.4.532. [DOI] [PubMed] [Google Scholar]
- Llinás R., Sugimori M. Electrophysiological properties of in vitro Purkinje cell dendrites in mammalian cerebellar slices. J Physiol. 1980 Aug;305:197–213. doi: 10.1113/jphysiol.1980.sp013358. [DOI] [PMC free article] [PubMed] [Google Scholar]
- Llinás R., Sugimori M. Electrophysiological properties of in vitro Purkinje cell somata in mammalian cerebellar slices. J Physiol. 1980 Aug;305:171–195. doi: 10.1113/jphysiol.1980.sp013357. [DOI] [PMC free article] [PubMed] [Google Scholar]
- Llinás R., Yarom Y. Electrophysiology of mammalian inferior olivary neurones in vitro. Different types of voltage-dependent ionic conductances. J Physiol. 1981 Jun;315:549–567. doi: 10.1113/jphysiol.1981.sp013763. [DOI] [PMC free article] [PubMed] [Google Scholar]
- Llinás R., Yarom Y. Properties and distribution of ionic conductances generating electroresponsiveness of mammalian inferior olivary neurones in vitro. J Physiol. 1981 Jun;315:569–584. doi: 10.1113/jphysiol.1981.sp013764. [DOI] [PMC free article] [PubMed] [Google Scholar]
- Malenka R. C., Kocsis J. D., Ransom B. R., Waxman S. G. Modulation of parallel fiber excitability by postsynaptically mediated changes in extracellular potassium. Science. 1981 Oct 16;214(4518):339–341. doi: 10.1126/science.7280695. [DOI] [PubMed] [Google Scholar]
- Matsuda Y., Yoshida S., Yonezawa T. A Ca- dependent regenerative response in rodent dorsal root ganglion cells cultured in vitro. Brain Res. 1976 Oct 15;115(2):334–338. doi: 10.1016/0006-8993(76)90519-9. [DOI] [PubMed] [Google Scholar]
- McAfee D. A., Yarowsky P. J. Calcium-dependent potentials in the mammalian sympathetic neurone. J Physiol. 1979 May;290(2):507–523. doi: 10.1113/jphysiol.1979.sp012787. [DOI] [PMC free article] [PubMed] [Google Scholar]
- Meech R. W. Calcium-dependent potassium activation in nervous tissues. Annu Rev Biophys Bioeng. 1978;7:1–18. doi: 10.1146/annurev.bb.07.060178.000245. [DOI] [PubMed] [Google Scholar]
- Moreton R. B. An investigation of the electrogenic sodium pump in snail neurones, using the constant-field theory. J Exp Biol. 1969 Aug;51(1):181–201. doi: 10.1242/jeb.51.1.181. [DOI] [PubMed] [Google Scholar]
- Neher E., Lux H. D. Rapid changes of potassium concentration at the outer surface of exposed single neurons during membrane current flow. J Gen Physiol. 1973 Mar;61(3):385–399. doi: 10.1085/jgp.61.3.385. [DOI] [PMC free article] [PubMed] [Google Scholar]
- Nicholson C., Bruggencate G. T., Senekowitsch R. Large potassium signals and slow potentials evoked during aminopyridine or barium superfusion in cat cerebellum. Brain Res. 1976 Sep 3;113(3):606–610. doi: 10.1016/0006-8993(76)90063-9. [DOI] [PubMed] [Google Scholar]
- Nicholson C., Bruggencate G. T., Steinberg R., Stöckle H. Calcium modulation in brain extracellular microenvironment demonstrated with ion-selective micropipette. Proc Natl Acad Sci U S A. 1977 Mar;74(3):1287–1290. doi: 10.1073/pnas.74.3.1287. [DOI] [PMC free article] [PubMed] [Google Scholar]
- Nicholson C. Dynamics of the brain cell microenvironment. Neurosci Res Program Bull. 1980 Apr;18(2):175–322. [PubMed] [Google Scholar]
- Nicholson C., Phillips J. M. Ion diffusion modified by tortuosity and volume fraction in the extracellular microenvironment of the rat cerebellum. J Physiol. 1981 Dec;321:225–257. doi: 10.1113/jphysiol.1981.sp013981. [DOI] [PMC free article] [PubMed] [Google Scholar]
- Nicholson C., ten Bruggencate G., Stöckle H., Steinberg R. Calcium and potassium changes in extracellular microenvironment of cat cerebellar cortex. J Neurophysiol. 1978 Jul;41(4):1026–1039. doi: 10.1152/jn.1978.41.4.1026. [DOI] [PubMed] [Google Scholar]
- Norman R. S. Diffusional spread of iontophoretically injected ions. J Theor Biol. 1975 Jul;52(1):159–162. doi: 10.1016/0022-5193(75)90047-8. [DOI] [PubMed] [Google Scholar]
- Orkand R. K., Nicholls J. G., Kuffler S. W. Effect of nerve impulses on the membrane potential of glial cells in the central nervous system of amphibia. J Neurophysiol. 1966 Jul;29(4):788–806. doi: 10.1152/jn.1966.29.4.788. [DOI] [PubMed] [Google Scholar]
- Somjen G. G. Electrogenesis of sustained potentials. Prog Neurobiol. 1973;1(3):201–237. [PubMed] [Google Scholar]
- Somjen G. G. Extracellular potassium in the mammalian central nervous system. Annu Rev Physiol. 1979;41:159–177. doi: 10.1146/annurev.ph.41.030179.001111. [DOI] [PubMed] [Google Scholar]
- Stöckle H., Ten Bruggencate G. Fluctuation of extracellular potassium and calcium in the cerebellar cortex related to climbing fiber activity. Neuroscience. 1980;5(5):893–901. doi: 10.1016/0306-4522(80)90158-x. [DOI] [PubMed] [Google Scholar]
- Tang C. M., Strichartz G. R., Orkand R. K. Sodium channels in axons and glial cells of the optic nerve of Necturus maculosa. J Gen Physiol. 1979 Nov;74(5):629–642. doi: 10.1085/jgp.74.5.629. [DOI] [PMC free article] [PubMed] [Google Scholar]
- Vern B. A., Schuette W. H., Thibault L. E. [K+]o clearance in cortex: a new analytical model. J Neurophysiol. 1977 Sep;40(5):1015–1023. doi: 10.1152/jn.1977.40.5.1015. [DOI] [PubMed] [Google Scholar]
- Weight F. F., Erulkar S. D. Modulation of synaptic transmitter release by repetitive postsynaptic action potentials. Science. 1976 Sep 10;193(4257):1023–1025. doi: 10.1126/science.7839. [DOI] [PubMed] [Google Scholar]
- Williams V., Grossman R. G., Edmunds S. M. Volume and surface area estimates of astrocytes in the sensorimotor cortex of the cat. Neuroscience. 1980;5(7):1151–1159. doi: 10.1016/0306-4522(80)90194-3. [DOI] [PubMed] [Google Scholar]
- Wong R. K., Prince D. A., Basbaum A. I. Intradendritic recordings from hippocampal neurons. Proc Natl Acad Sci U S A. 1979 Feb;76(2):986–990. doi: 10.1073/pnas.76.2.986. [DOI] [PMC free article] [PubMed] [Google Scholar]
- Yamamoto C. Electrical activity observed in vitro in thin sections from guinea-pig cerebellum. Jpn J Physiol. 1974 Apr;24(2):177–188. doi: 10.2170/jjphysiol.24.177. [DOI] [PubMed] [Google Scholar]
- Yarom Y., Spira M. E. Extracellular potassium ions mediate specific neuronal interaction. Science. 1982 Apr 2;216(4541):80–82. doi: 10.1126/science.6278595. [DOI] [PubMed] [Google Scholar]