Abstract
The photoprotein aequorin was injected into cells of ferret papillary muscles to monitor the resting intracellular free Ca concentration [( Ca2+]i). Increasing the external Ca concentration [( Ca2+]o) increased both resting [Ca2+]i and resting tension. The tension and [Ca2+]i both rose to a peak and then declined to a steady-state level which was higher than the control. Qualitatively similar, but larger, effects were observed if [Ca2+]i was first elevated with strophanthidin. The increase of [Ca2+]i was accompanied by the development of spontaneous oscillations of [Ca2+]i. When a steady level of [Ca2+]i had been reached in high [Ca2+]o, [Ca2+]o was reduced back to the control level for a brief period. A subsequent increase of [Ca2+]o produced a rise of [Ca2+]i to the same steady level as that previously found in the high [Ca2+]o but the initial peak and subsequent decline were absent. It is suggested that the decline of [Ca2+]i from the initial peak is mediated by a fall of intracellular Na concentration [( Na+]i) limiting Ca entry on a Na-Ca exchange. Increasing external K concentration [( K+]o) from 5 to 30 mmol/l had no detectable effect on [Ca2+]i under control conditions. However, if [Ca2+]i was first increased either by applying strophanthidin or by increasing [Ca2+]o, increasing [K+]o produced a transient rise of [Ca2+]i and tension. This rise was unaffected by D600. It is suggested that the secondary decline of [Ca2+]i after the initial rise may, again, be produced by a fall of [Na+]i acting on an Na-Ca exchange. Acidification produced by increasing [CO2] had no detectable effect on [Ca2+]i under control conditions. However, if [Ca2+]i was increased by strophanthidin, acidification produced a rise of [Ca2+]i. This rise of [Ca2+]i was partly transient even when the intracellular acidification was presumably maintained (raising CO2 at constant [HCO3-]). Acidification in Na-free solutions had qualitatively similar effects to those in Na-containing solutions. In Na-free solutions (Na replaced by K) the [Ca2+]i could be maintained at a low level for at least several hours. Increases of [Ca2+]o in Na-free solutions led to a decrease of [Ca2+]i, and similarly decreasing [Ca2+]o led to an increase in [Ca2+]i. These anomalous effects of [Ca2+]o on [Ca2+]i could be abolished by Mn ions or D600. It is suggested that changes in [Ca2+]o may have reciprocal effects on Ca permeability and hence on [Ca2+]i. The application of the mitochondrial uncoupler FCCP in Na-free solutions led to an increase of resting tension followed, after a substantial delay, by an increase of [Ca2+]i.(ABSTRACT TRUNCATED AT 400 WORDS)
Full text
PDF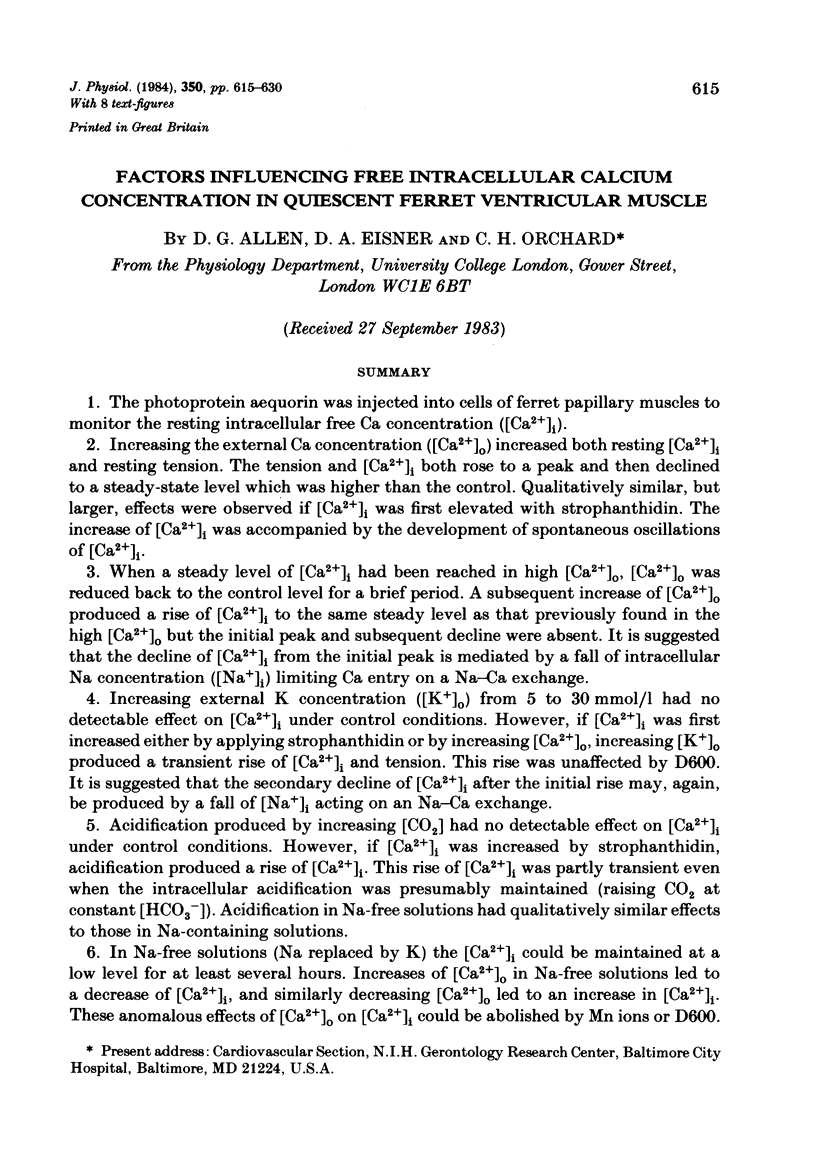
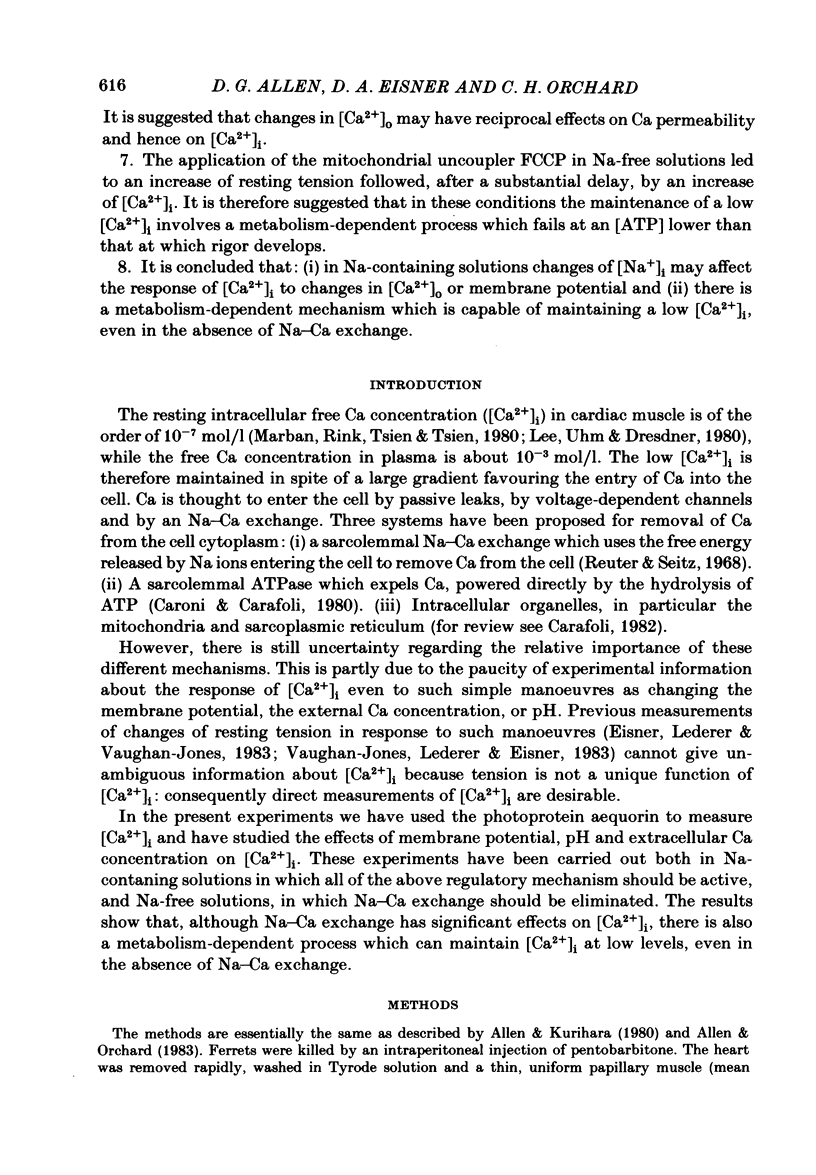
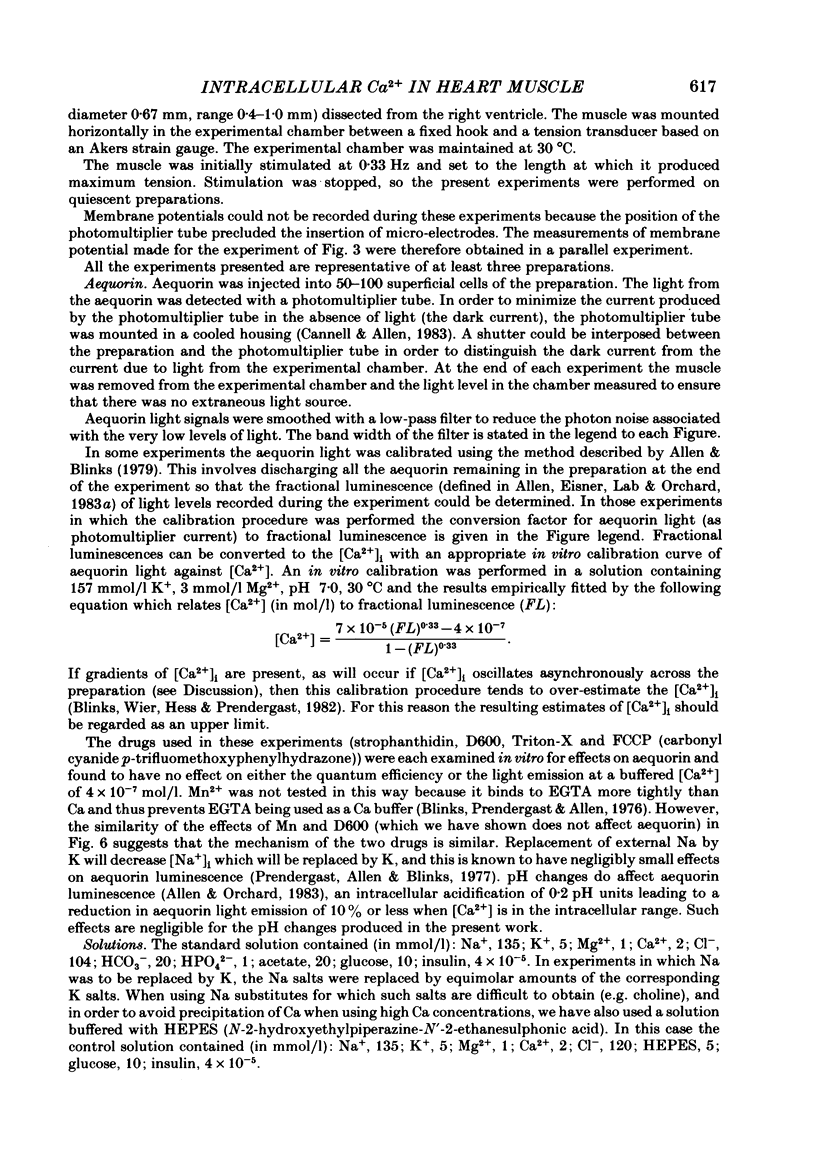
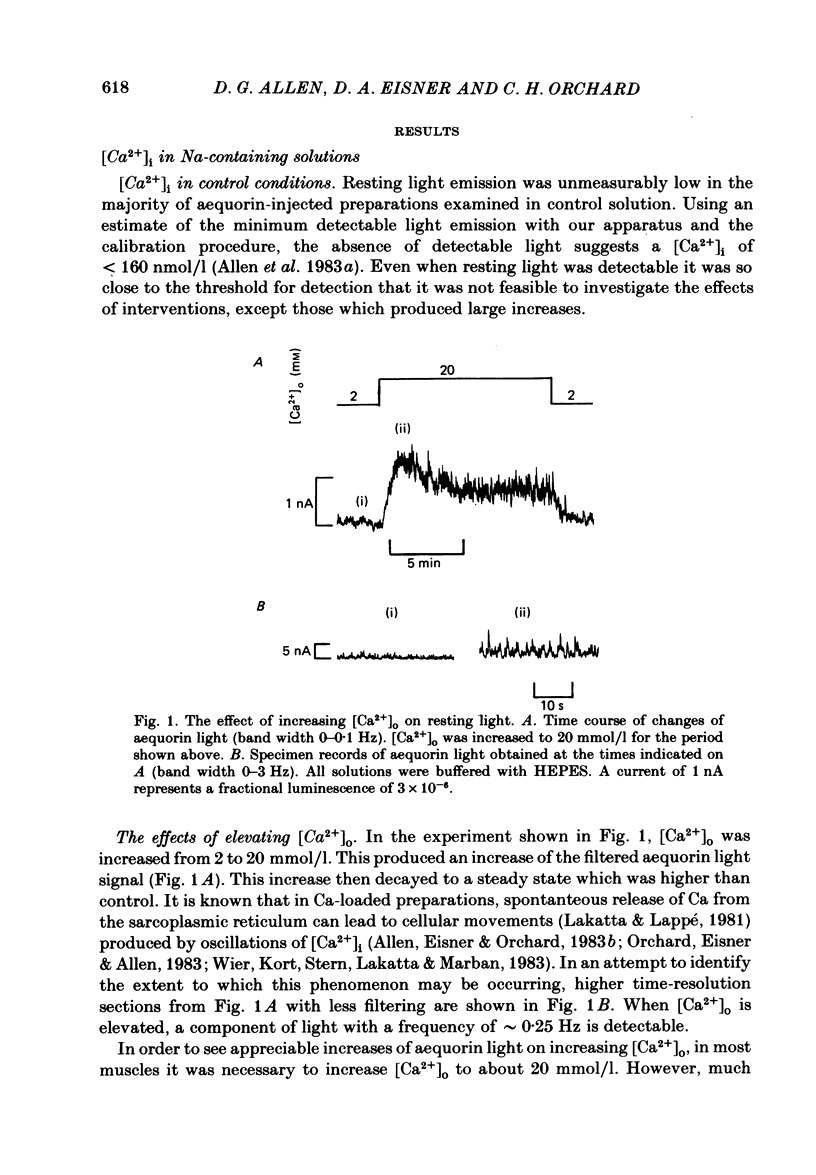
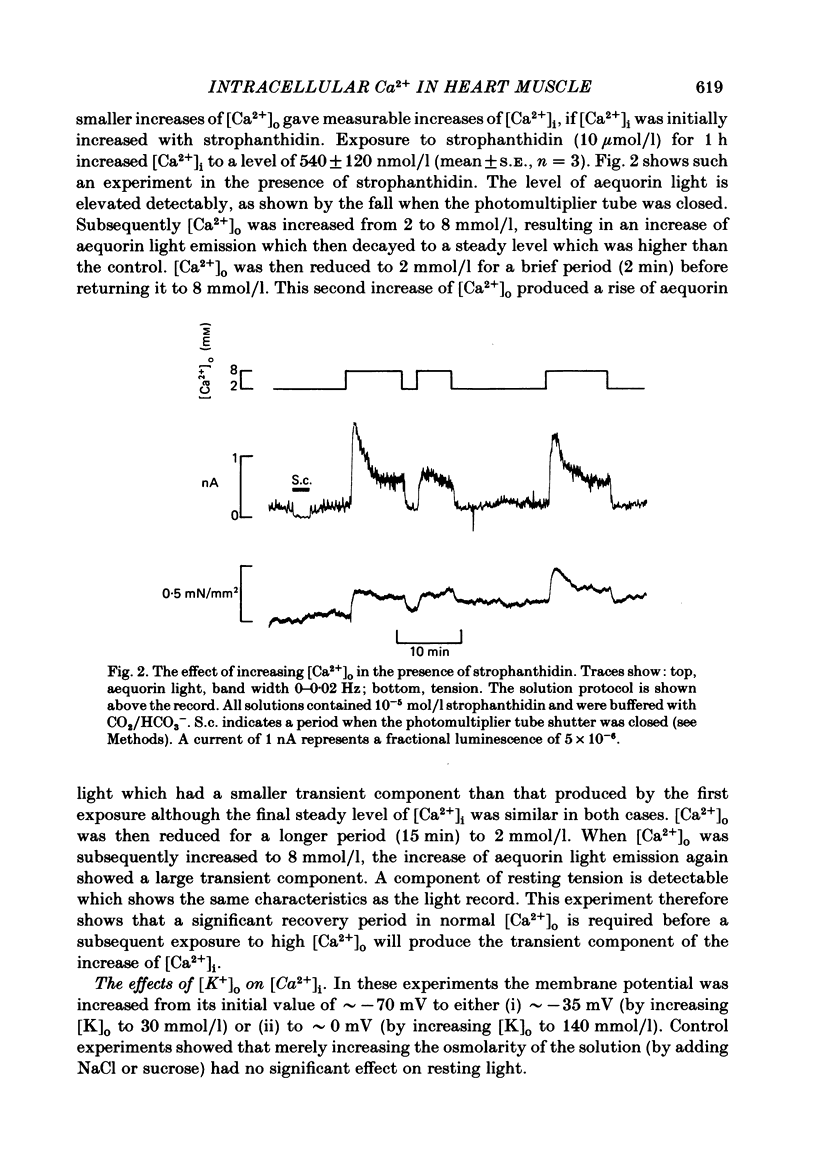
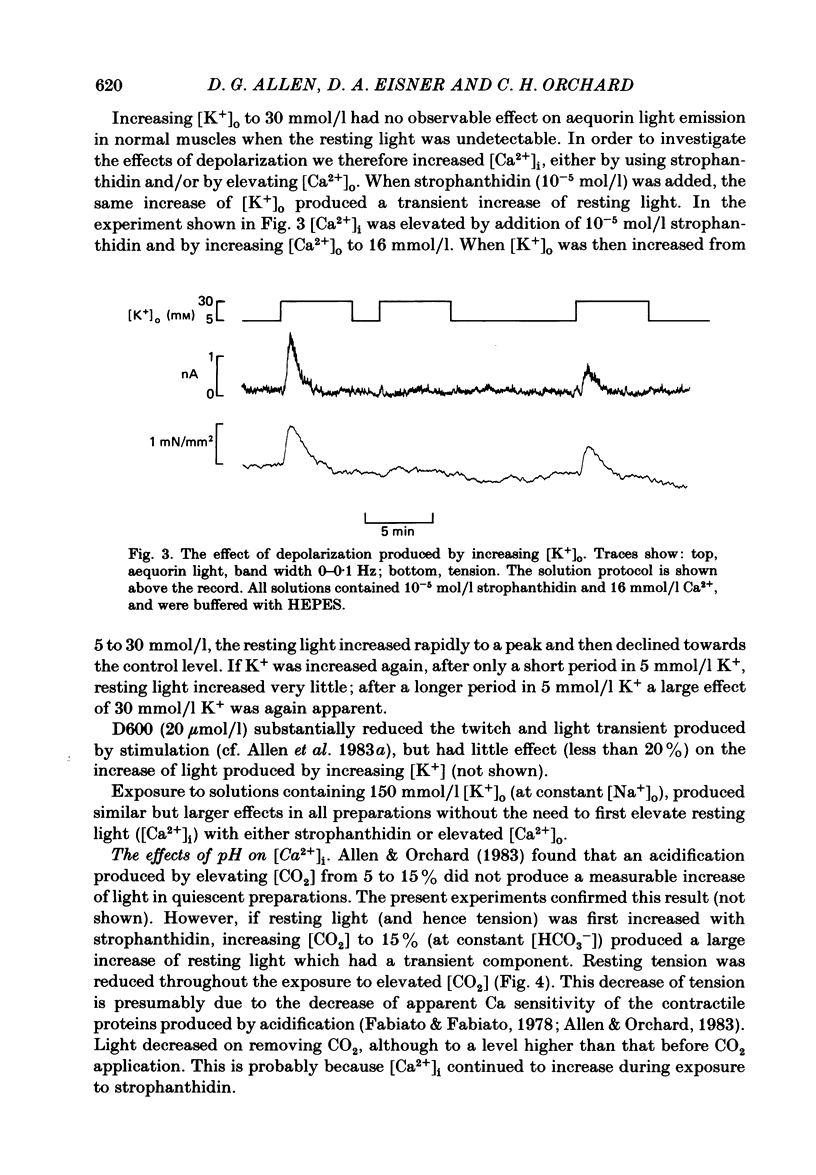
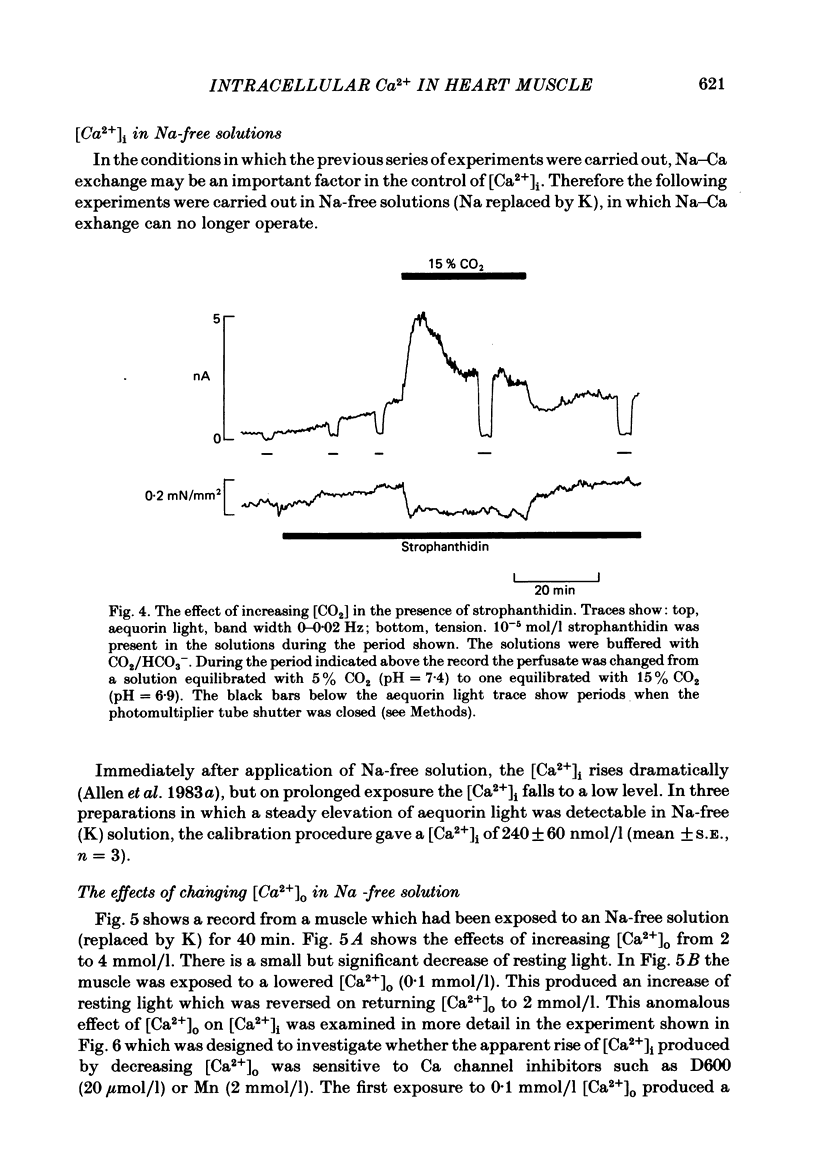
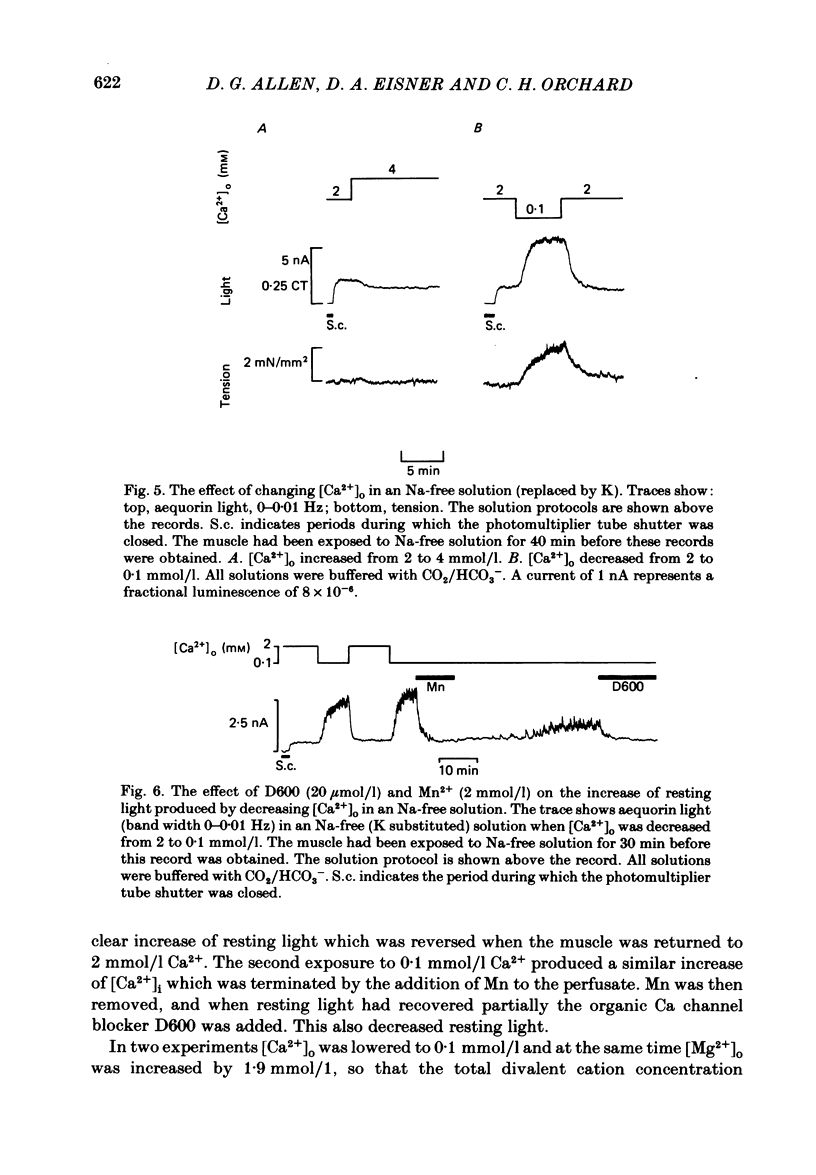

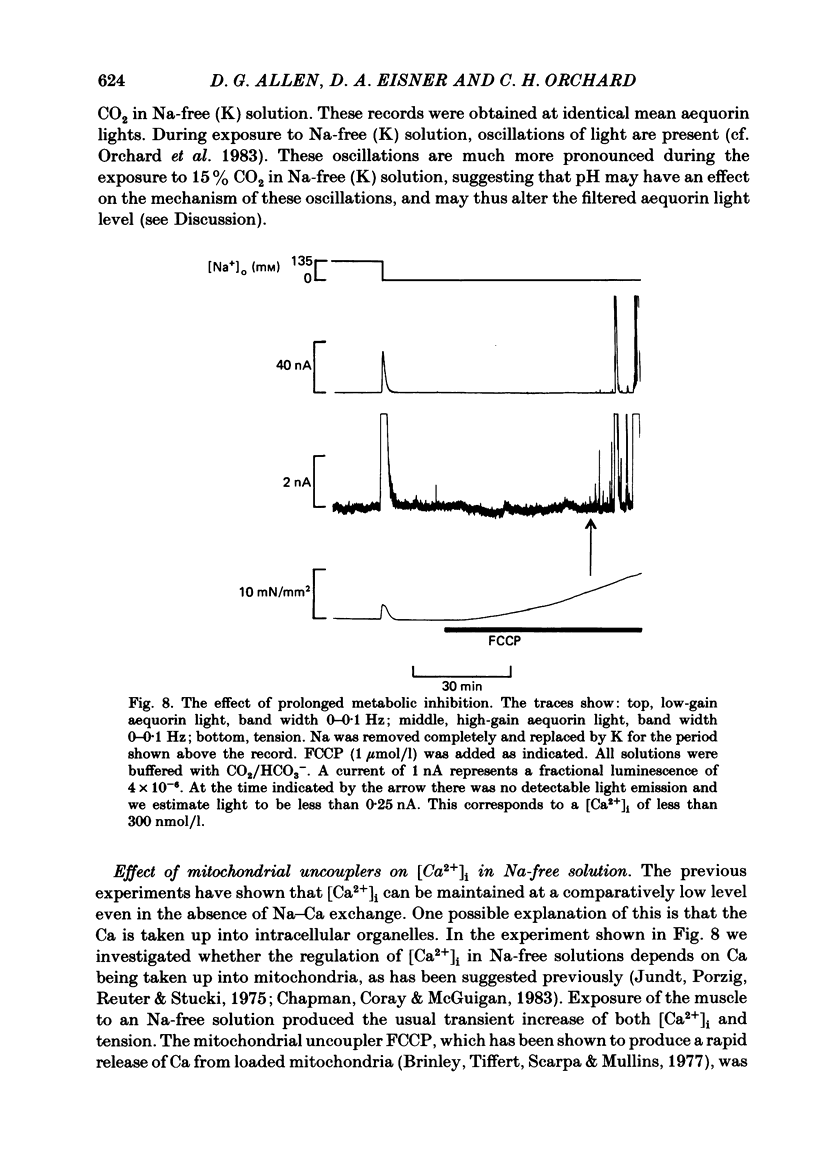
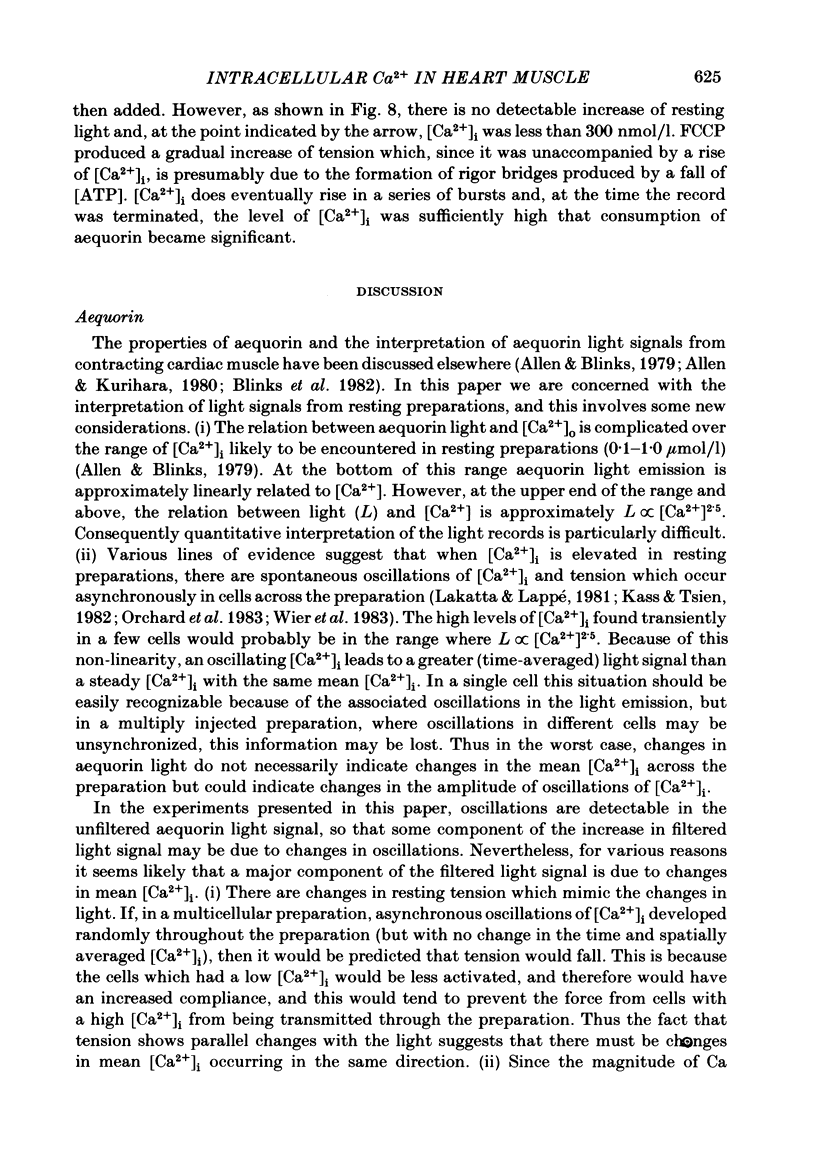
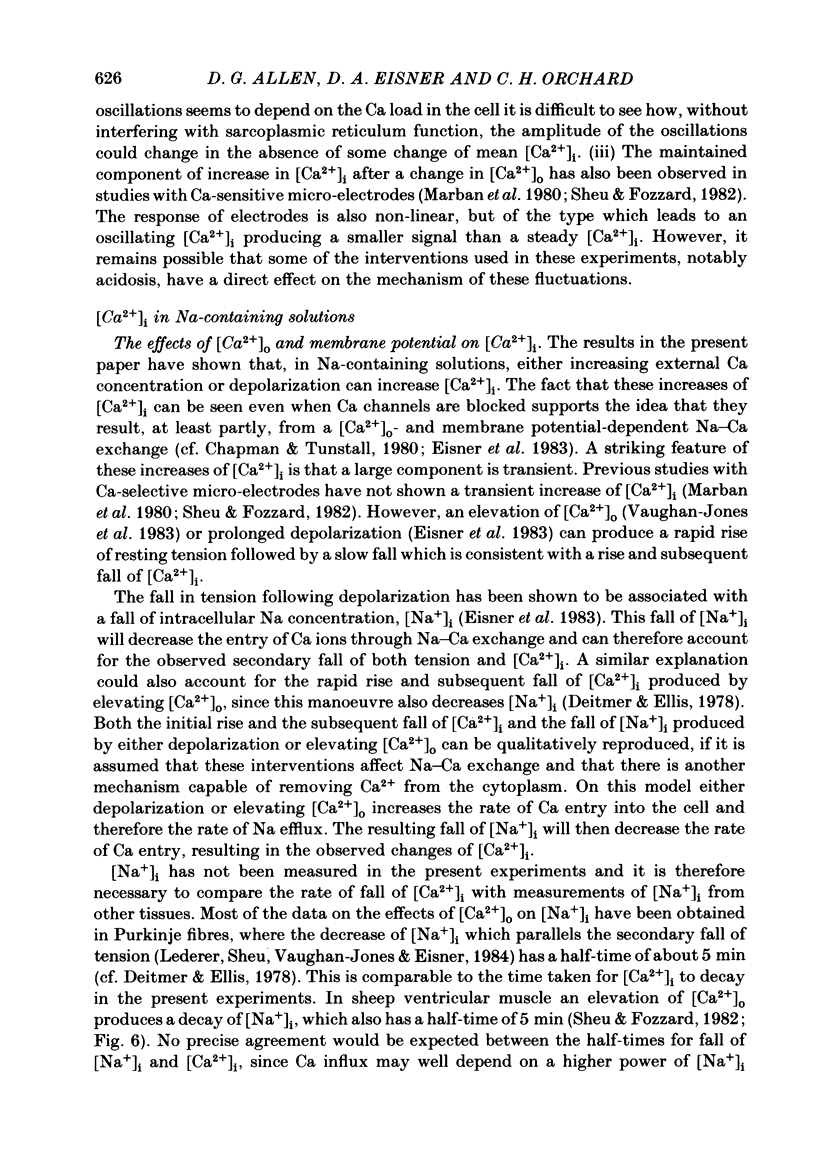

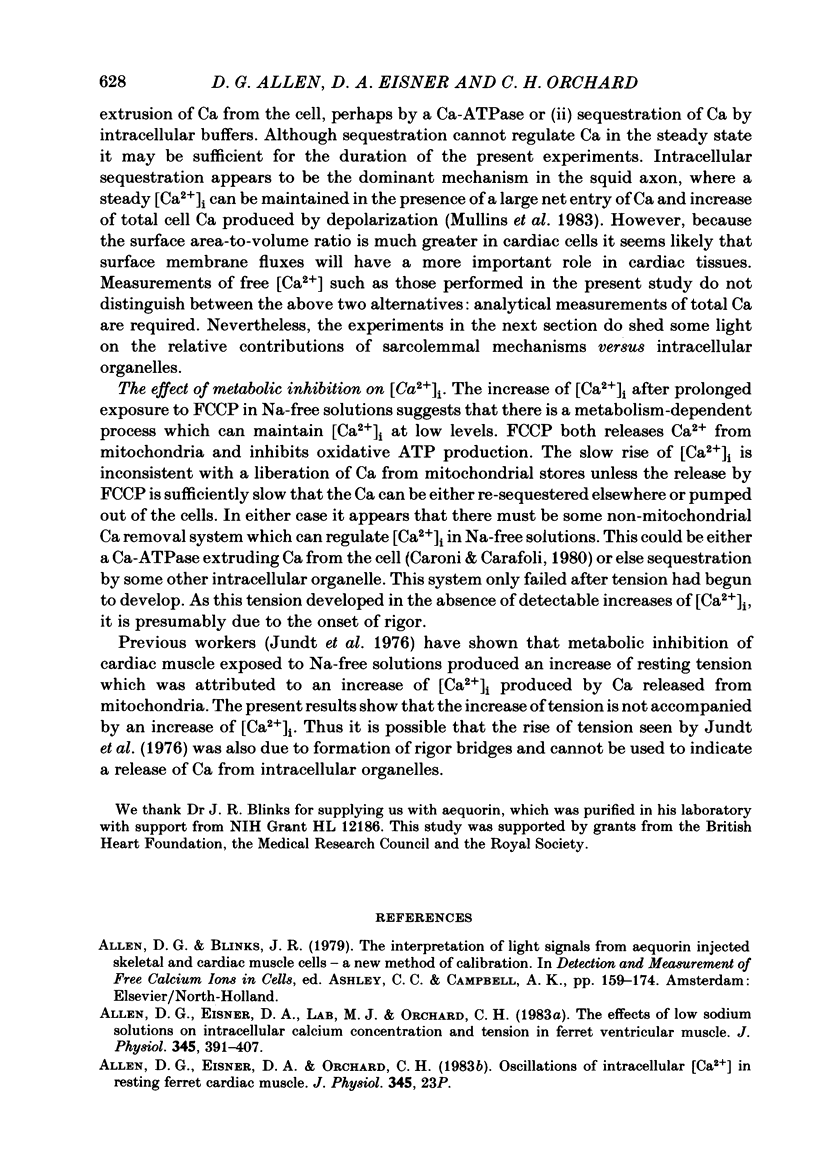
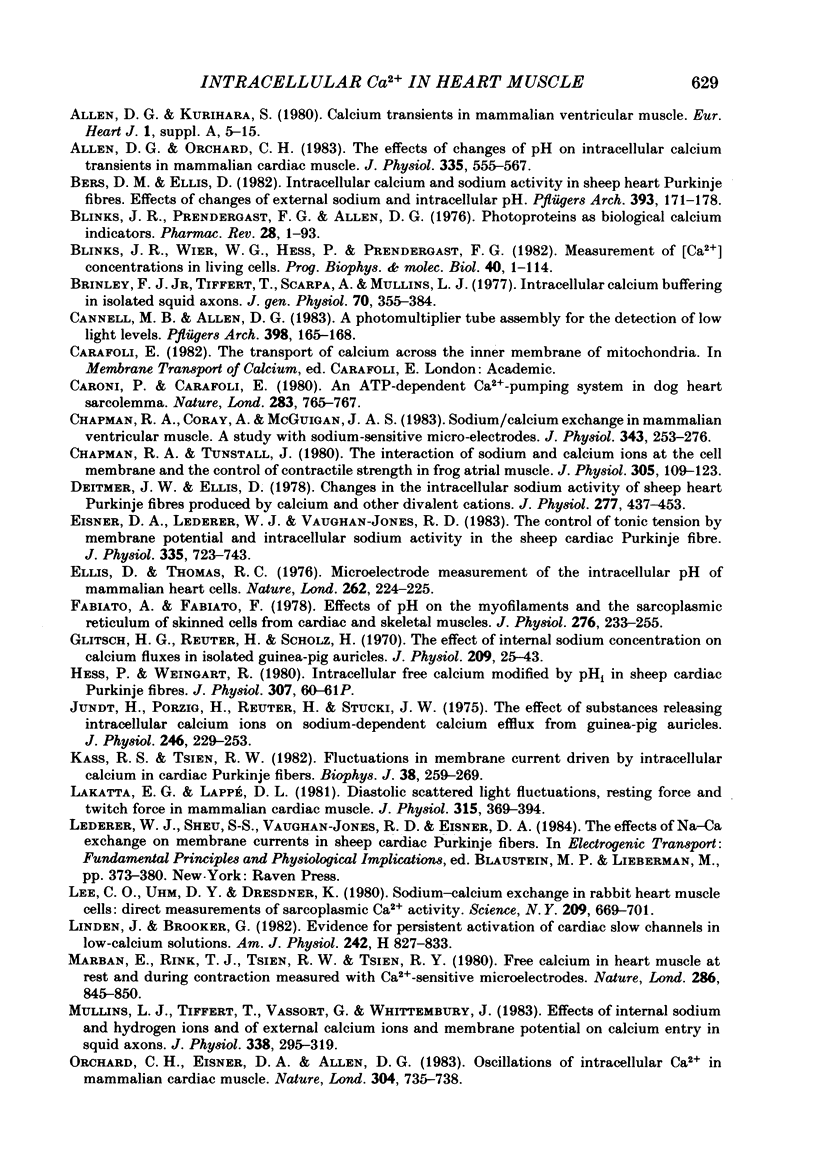

Selected References
These references are in PubMed. This may not be the complete list of references from this article.
- Allen D. G., Eisner D. A., Lab M. J., Orchard C. H. The effects of low sodium solutions on intracellular calcium concentration and tension in ferret ventricular muscle. J Physiol. 1983 Dec;345:391–407. doi: 10.1113/jphysiol.1983.sp014984. [DOI] [PMC free article] [PubMed] [Google Scholar]
- Allen D. G., Kurihara S. Calcium transients in mammalian ventricular muscle. Eur Heart J. 1980;Suppl A:5–15. doi: 10.1093/eurheartj/1.suppl_1.5. [DOI] [PubMed] [Google Scholar]
- Allen D. G., Orchard C. H. The effects of changes of pH on intracellular calcium transients in mammalian cardiac muscle. J Physiol. 1983 Feb;335:555–567. doi: 10.1113/jphysiol.1983.sp014550. [DOI] [PMC free article] [PubMed] [Google Scholar]
- Bers D. M., Ellis D. Intracellular calcium and sodium activity in sheep heart Purkinje fibres. Effect of changes of external sodium and intracellular pH. Pflugers Arch. 1982 Apr;393(2):171–178. doi: 10.1007/BF00582941. [DOI] [PubMed] [Google Scholar]
- Blinks J. R., Prendergast F. G., Allen D. G. Photoproteins as biological calcium indicators. Pharmacol Rev. 1976 Mar;28(1):1–93. [PubMed] [Google Scholar]
- Blinks J. R., Wier W. G., Hess P., Prendergast F. G. Measurement of Ca2+ concentrations in living cells. Prog Biophys Mol Biol. 1982;40(1-2):1–114. doi: 10.1016/0079-6107(82)90011-6. [DOI] [PubMed] [Google Scholar]
- Brinley F. J., Jr, Tiffert T., Scarpa A., Mullins L. J. Intracellular calcium buffering capacity in isolated squid axons. J Gen Physiol. 1977 Sep;70(3):355–384. doi: 10.1085/jgp.70.3.355. [DOI] [PMC free article] [PubMed] [Google Scholar]
- Cannell M. B., Allen D. G. A photomultiplier tube assembly for the detection of low light levels. Pflugers Arch. 1983 Jul;398(2):165–168. doi: 10.1007/BF00581066. [DOI] [PubMed] [Google Scholar]
- Caroni P., Carafoli E. An ATP-dependent Ca2+-pumping system in dog heart sarcolemma. Nature. 1980 Feb 21;283(5749):765–767. doi: 10.1038/283765a0. [DOI] [PubMed] [Google Scholar]
- Chapman R. A., Coray A., McGuigan J. A. Sodium/calcium exchange in mammalian ventricular muscle: a study with sodium-sensitive micro-electrodes. J Physiol. 1983 Oct;343:253–276. doi: 10.1113/jphysiol.1983.sp014891. [DOI] [PMC free article] [PubMed] [Google Scholar]
- Chapman R. A., Tunstall J. The interaction of sodium and calcium ions at the cell membrane and the control of contractile strength in frog atrial muscle. J Physiol. 1980 Aug;305:109–123. doi: 10.1113/jphysiol.1980.sp013353. [DOI] [PMC free article] [PubMed] [Google Scholar]
- Deitmer J. W., Ellis D. Changes in the intracellular sodium activity of sheep heart Purkinje fibres produced by calcium and other divalent cations. J Physiol. 1978 Apr;277:437–453. doi: 10.1113/jphysiol.1978.sp012283. [DOI] [PMC free article] [PubMed] [Google Scholar]
- Eisner D. A., Lederer W. J., Vaughan-Jones R. D. The control of tonic tension by membrane potential and intracellular sodium activity in the sheep cardiac Purkinje fibre. J Physiol. 1983 Feb;335:723–743. doi: 10.1113/jphysiol.1983.sp014560. [DOI] [PMC free article] [PubMed] [Google Scholar]
- Ellis D., Thomas R. C. Microelectrode measurement of the intracellular pH of mammalian heart cells. Nature. 1976 Jul 15;262(5565):224–225. doi: 10.1038/262224a0. [DOI] [PubMed] [Google Scholar]
- Fabiato A., Fabiato F. Effects of pH on the myofilaments and the sarcoplasmic reticulum of skinned cells from cardiace and skeletal muscles. J Physiol. 1978 Mar;276:233–255. doi: 10.1113/jphysiol.1978.sp012231. [DOI] [PMC free article] [PubMed] [Google Scholar]
- Glitsch H. G., Reuter H., Scholz H. The effect of the internal sodium concentration on calcium fluxes in isolated guinea-pig auricles. J Physiol. 1970 Jul;209(1):25–43. doi: 10.1113/jphysiol.1970.sp009153. [DOI] [PMC free article] [PubMed] [Google Scholar]
- Jundt H., Porzig H., Reuter H., Stucki J. W. The effect of substances releasing intracellular calcium ions on sodium-dependent calcium efflux from guinea-pig auricles. J Physiol. 1975 Mar;246(1):229–253. doi: 10.1113/jphysiol.1975.sp010888. [DOI] [PMC free article] [PubMed] [Google Scholar]
- Kass R. S., Tsien R. W. Fluctuations in membrane current driven by intracellular calcium in cardiac Purkinje fibers. Biophys J. 1982 Jun;38(3):259–269. doi: 10.1016/S0006-3495(82)84557-8. [DOI] [PMC free article] [PubMed] [Google Scholar]
- Lakatta E. G., Lappé D. L. Diastolic scattered light fluctuation, resting force and twitch force in mammalian cardiac muscle. J Physiol. 1981 Jun;315:369–394. doi: 10.1113/jphysiol.1981.sp013753. [DOI] [PMC free article] [PubMed] [Google Scholar]
- Lederer W. J., Sheu S. S., Vaughan-Jones R. D., Eisner D. A. The effects of Na-Ca exchange on membrane currents in sheep cardiac Purkinje fibers. Soc Gen Physiol Ser. 1984;38:373–380. [PubMed] [Google Scholar]
- Lee C. O., Uhm D. Y., Dresdner K. Sodium-calcium exchange in rabbit heart muscle cells: direct measurement of sarcoplasmic Ca2+ activity. Science. 1980 Aug 8;209(4457):699–701. doi: 10.1126/science.7394527. [DOI] [PubMed] [Google Scholar]
- Marban E., Rink T. J., Tsien R. W., Tsien R. Y. Free calcium in heart muscle at rest and during contraction measured with Ca2+ -sensitive microelectrodes. Nature. 1980 Aug 28;286(5776):845–850. doi: 10.1038/286845a0. [DOI] [PubMed] [Google Scholar]
- Mullins L. J., Tiffert T., Vassort G., Whittembury J. Effects of internal sodium and hydrogen ions and of external calcium ions and membrane potential on calcium entry in squid axons. J Physiol. 1983 May;338:295–319. doi: 10.1113/jphysiol.1983.sp014674. [DOI] [PMC free article] [PubMed] [Google Scholar]
- Orchard C. H., Eisner D. A., Allen D. G. Oscillations of intracellular Ca2+ in mammalian cardiac muscle. Nature. 1983 Aug 25;304(5928):735–738. doi: 10.1038/304735a0. [DOI] [PubMed] [Google Scholar]
- Reuter H., Seitz N. The dependence of calcium efflux from cardiac muscle on temperature and external ion composition. J Physiol. 1968 Mar;195(2):451–470. doi: 10.1113/jphysiol.1968.sp008467. [DOI] [PMC free article] [PubMed] [Google Scholar]
- Sheu S. S., Fozzard H. A. Transmembrane Na+ and Ca2+ electrochemical gradients in cardiac muscle and their relationship to force development. J Gen Physiol. 1982 Sep;80(3):325–351. doi: 10.1085/jgp.80.3.325. [DOI] [PMC free article] [PubMed] [Google Scholar]
- Vaughan-Jones R. D., Lederer W. J., Eisner D. A. Ca2+ ions can affect intracellular pH in mammalian cardiac muscle. Nature. 1983 Feb 10;301(5900):522–524. doi: 10.1038/301522a0. [DOI] [PubMed] [Google Scholar]
- Wier W. G., Kort A. A., Stern M. D., Lakatta E. G., Marban E. Cellular calcium fluctuations in mammalian heart: direct evidence from noise analysis of aequorin signals in Purkinje fibers. Proc Natl Acad Sci U S A. 1983 Dec;80(23):7367–7371. doi: 10.1073/pnas.80.23.7367. [DOI] [PMC free article] [PubMed] [Google Scholar]