Abstract
A double-barrelled, pH-sensitive micro-electrode suitable for use in mammalian smooth muscle has been developed. It was shown to be unaffected by alteration of Na, K, Ca, Mg, Cl or CO2, and yielded the same results in mouse soleus muscle as had been obtained previously with the recessed-tip pH-sensitive glass electrode ( Aickin & Thomas, 1977 a). Intracellular pH (pHi) of the surface cells of guinea-pig vas deferens was 7.06 +/- 0.09 (n = 52, S.D. of an observation) in Krebs solution equilibrated with 3% CO2 at pH 7.35. The membrane potential (Em) was -65.5 +/- 6.7 mV. Thus pHi is about 0.8 units more alkaline than that predicted if H+ ions were passively distributed across the cell membrane. Alteration of extracellular pH (pHo) at constant CO2 caused a smaller change in pHi, by about 40% of that in pHo. The change was complete in 6-12 min and was of a similar magnitude whether the alteration was made in the continual presence or absence of CO2. Alteration of the CO2 level at constant pHo caused a rapid change in pHi followed by a slower, complete recovery. Thus the same stabilized pHi was recorded in different CO2-containing solutions. When CO2 was removed, the expected intracellular alkalinization was reduced or even obscured by a considerable acidification. pHi then stabilized at a mean value of 6.81 +/- 0.11 (n = 18) with an Em of -60.8 +/- 8.2 mV. The acidification expected on readmission of CO2 was minimized or obscured by a rapid recovery of pHi to the value previously recorded in CO2-containing solutions. A simultaneous increase in CO2 and decrease in pHo caused a rapid fall in pHi which increased in magnitude with decreasing pHo. This fall was followed by an incomplete recovery when pHo was above about 6.8 (but below 7.35), by no further change in pHi when pHo was about 6.7 and by a slow, continued fall in pHi when pHo was below 6.7. The intrinsic buffering power was calculated from the pHi changes observed on alteration of CO2. The values obtained increased in parallel with the extent to which pHi recovered following the imposed change, probably explained by an inseparable contribution to the minimization of the change by transport processes.(ABSTRACT TRUNCATED AT 400 WORDS)
Full text
PDF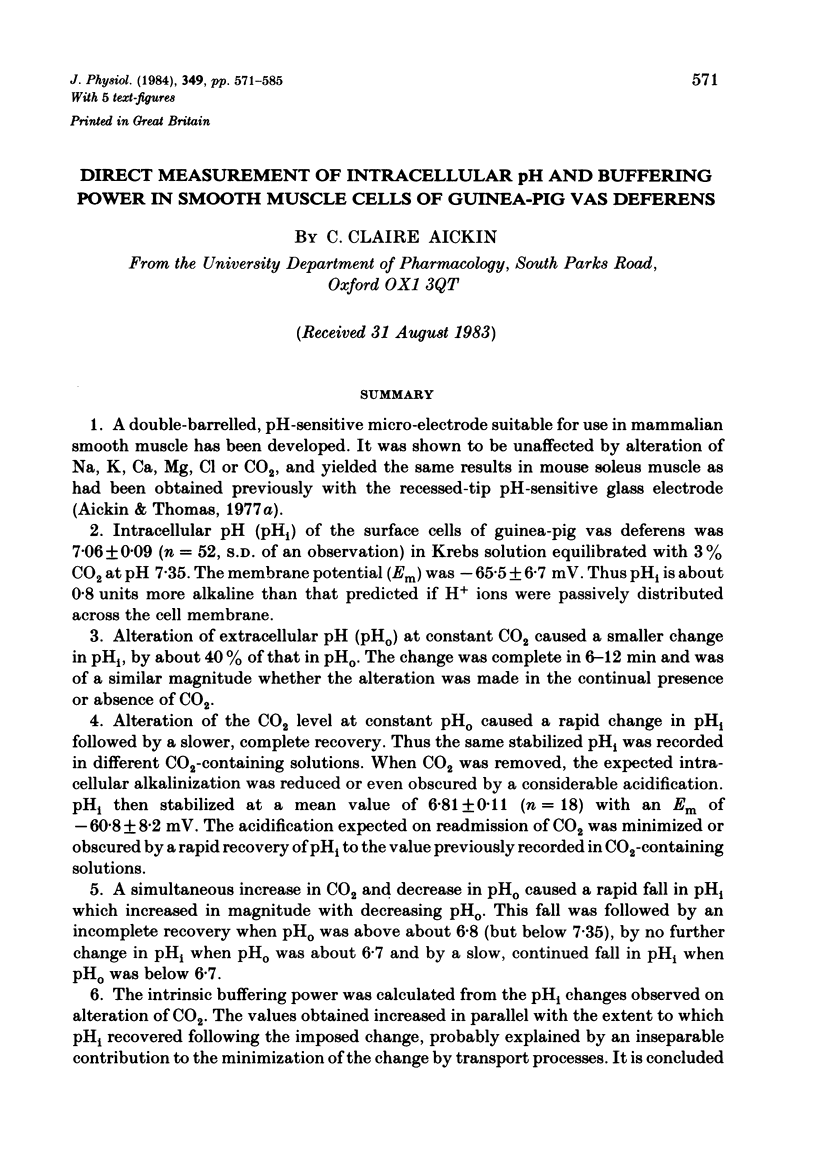
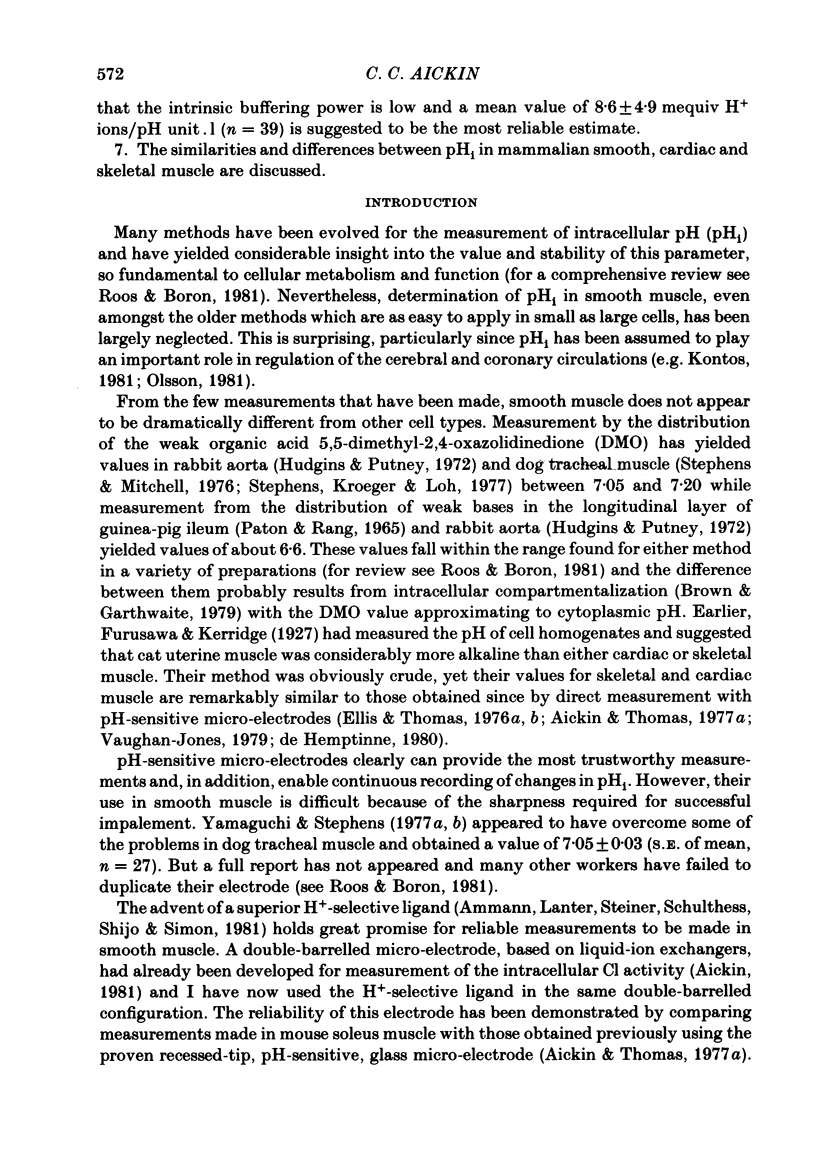
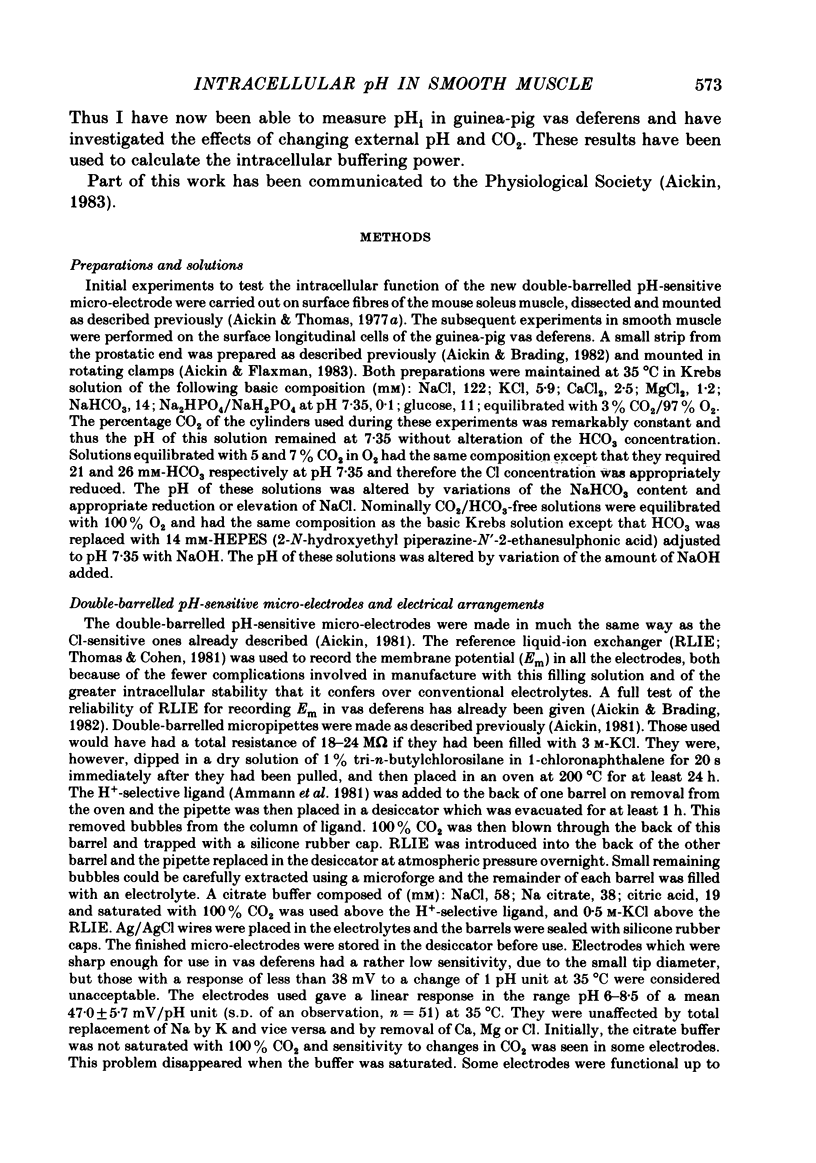
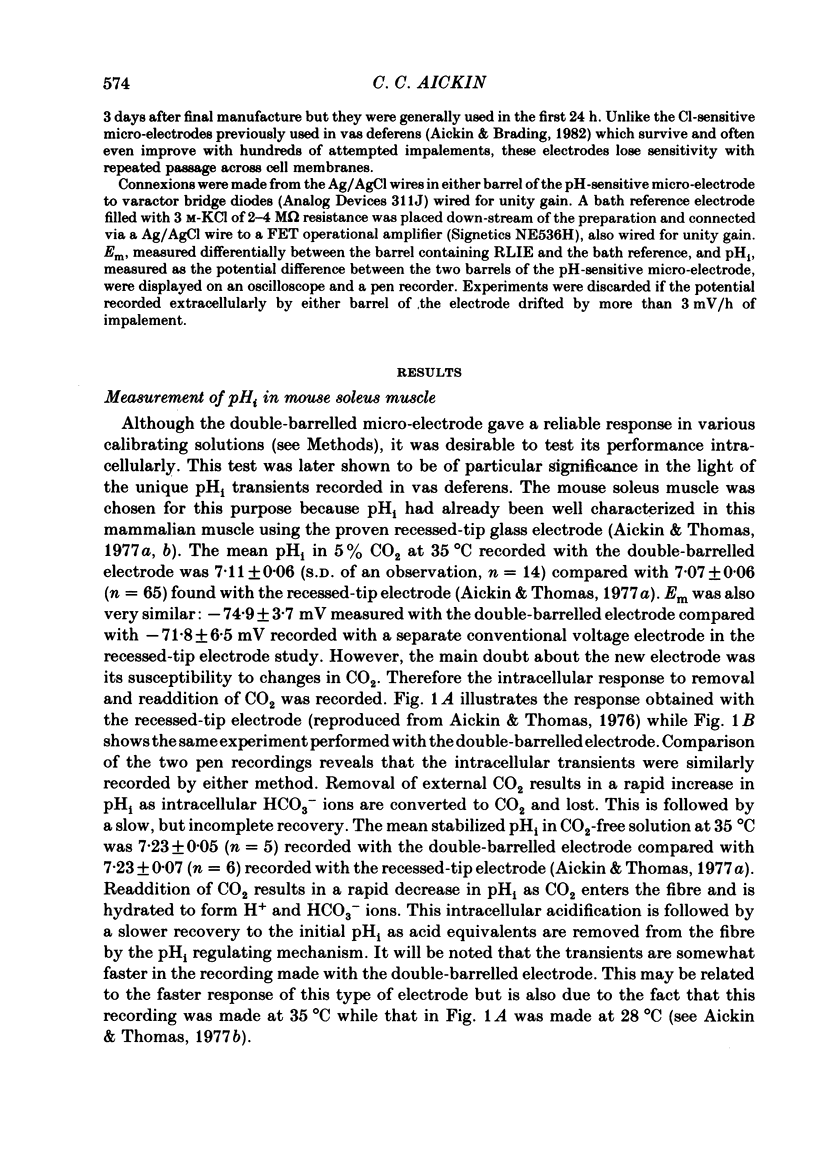
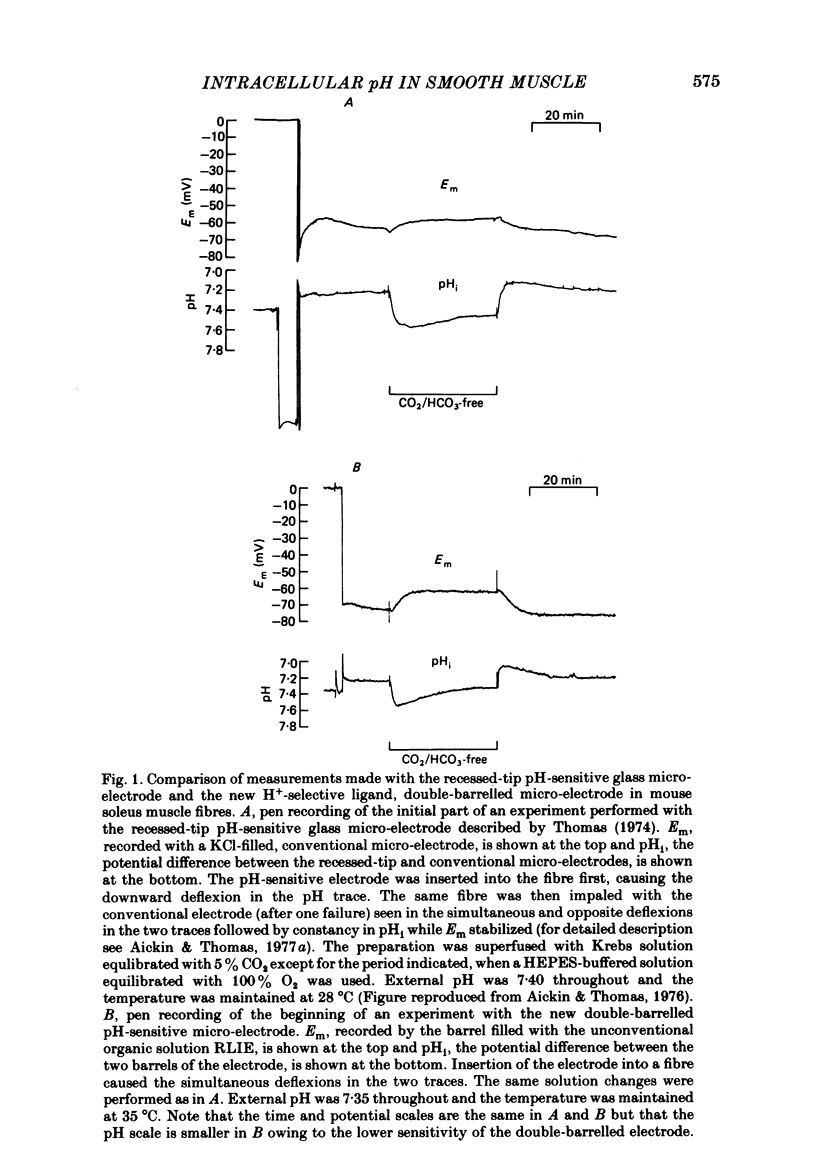
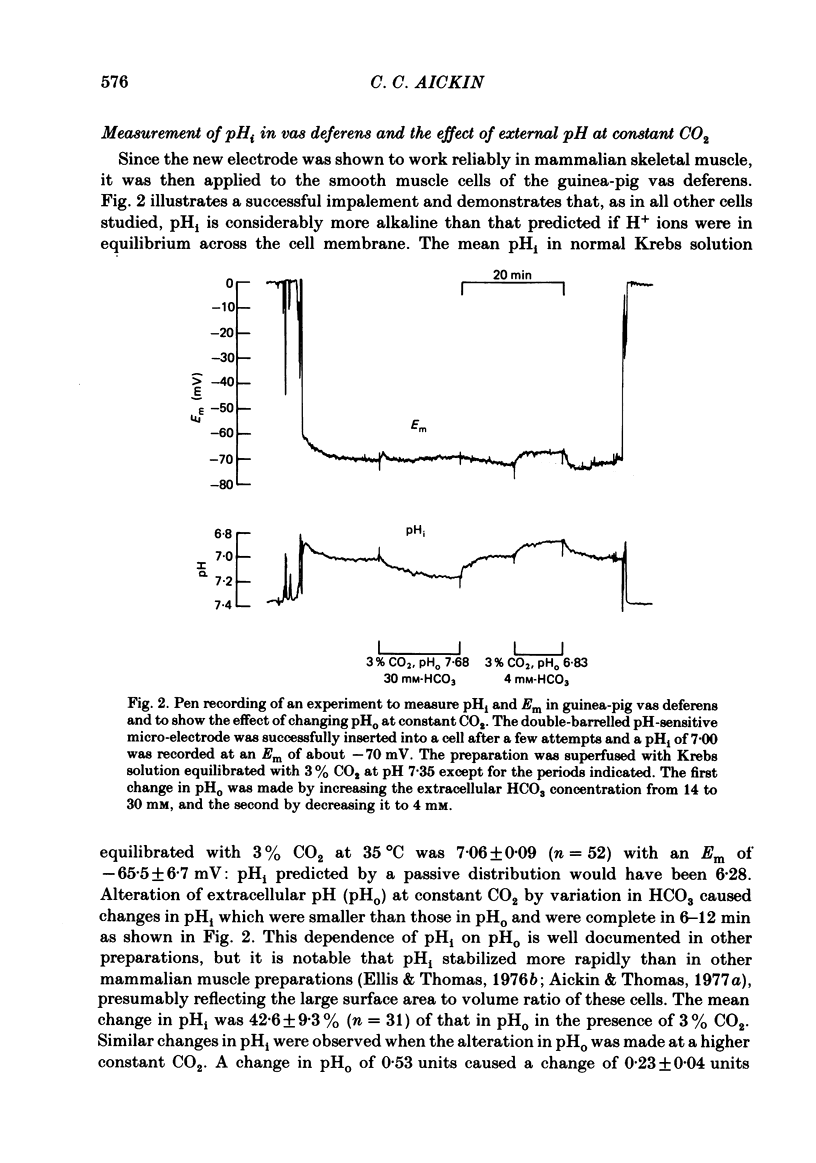
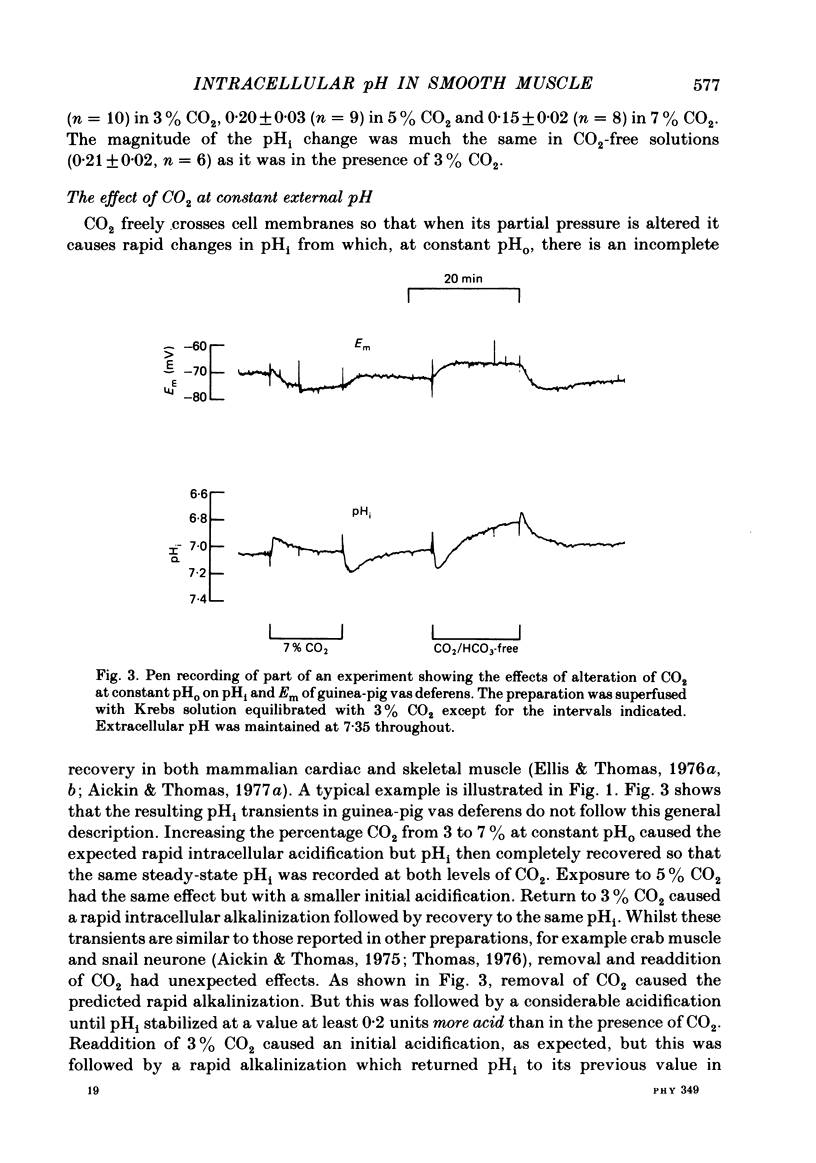
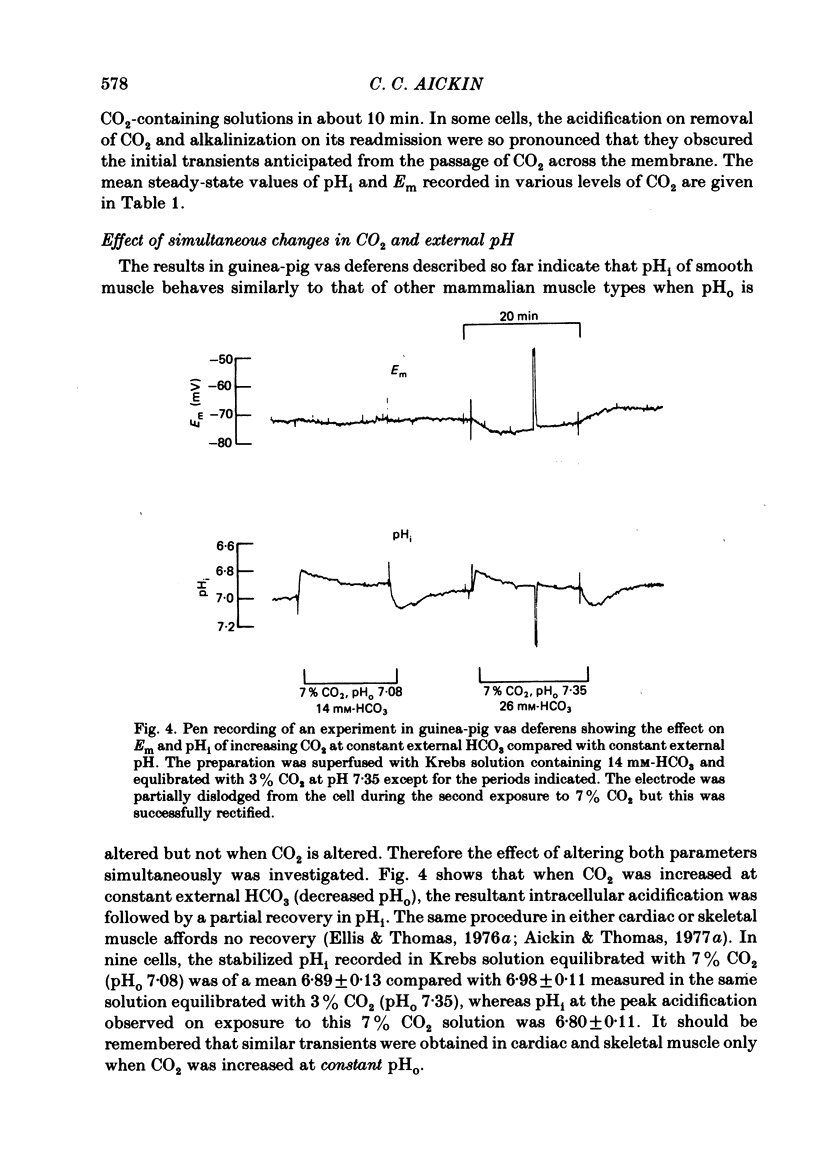
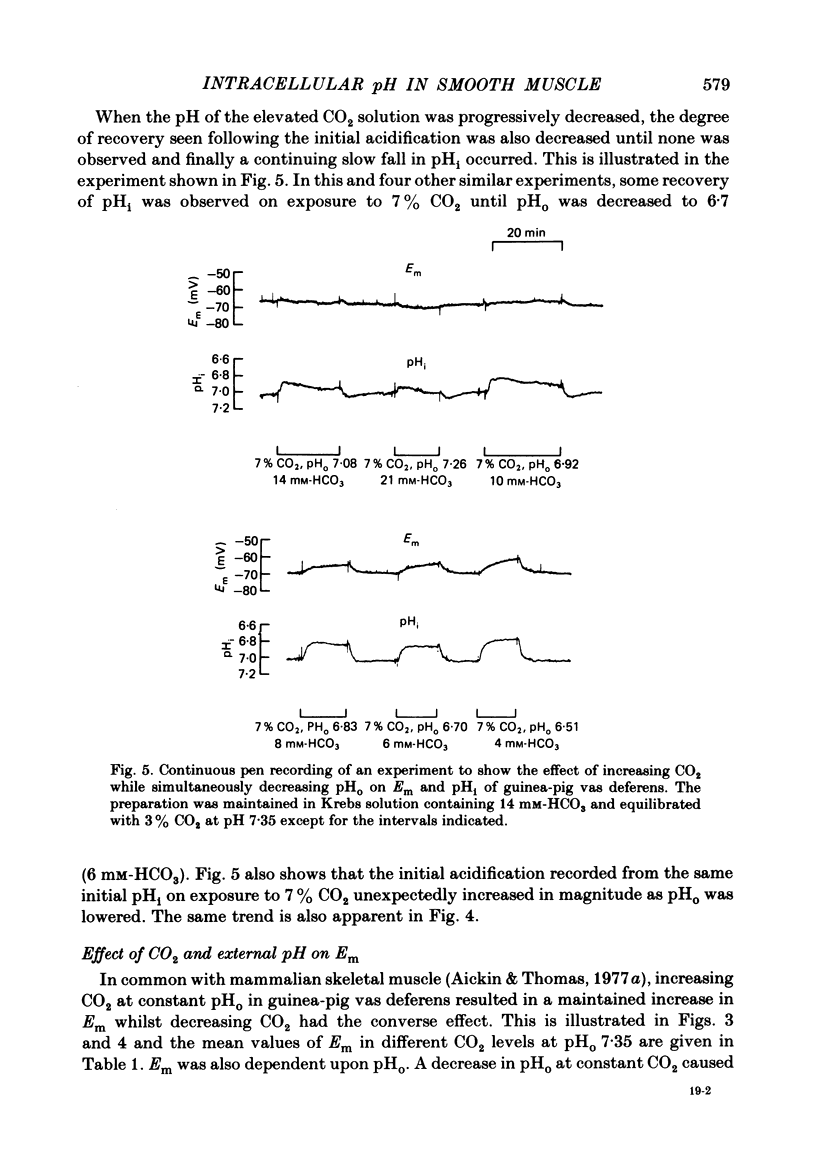
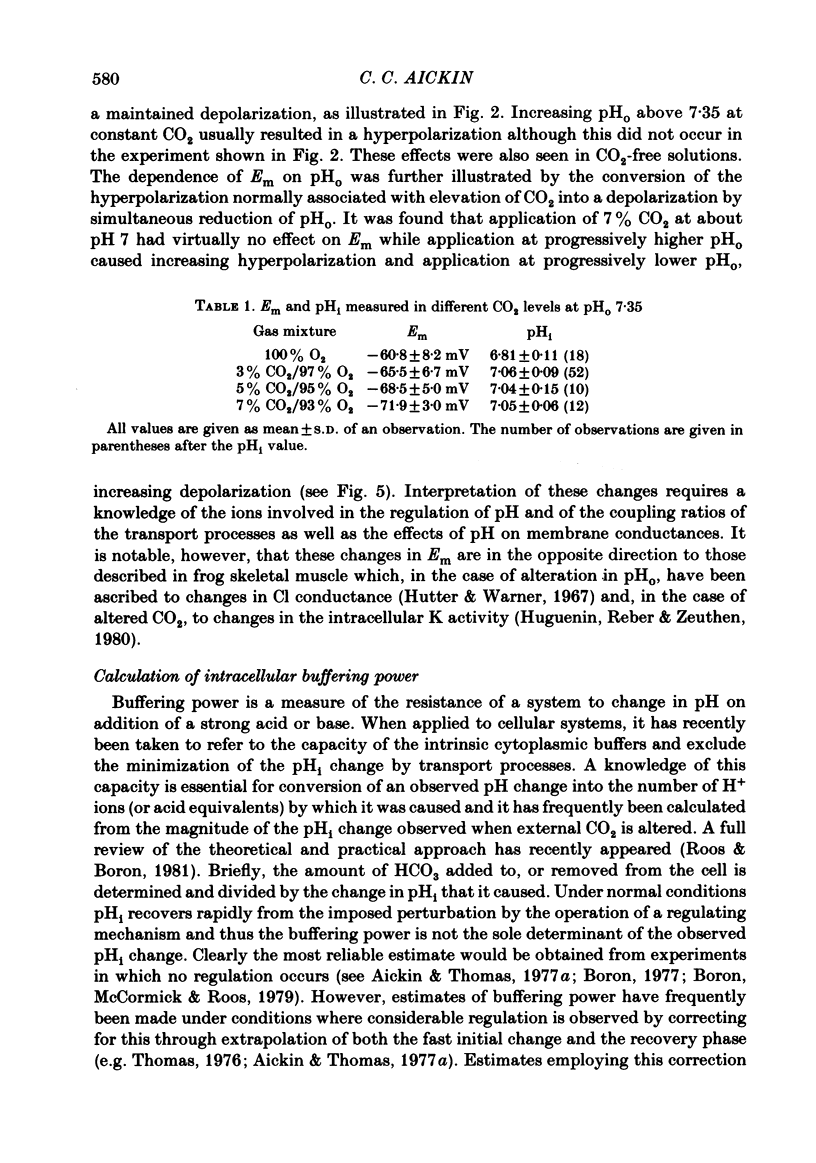
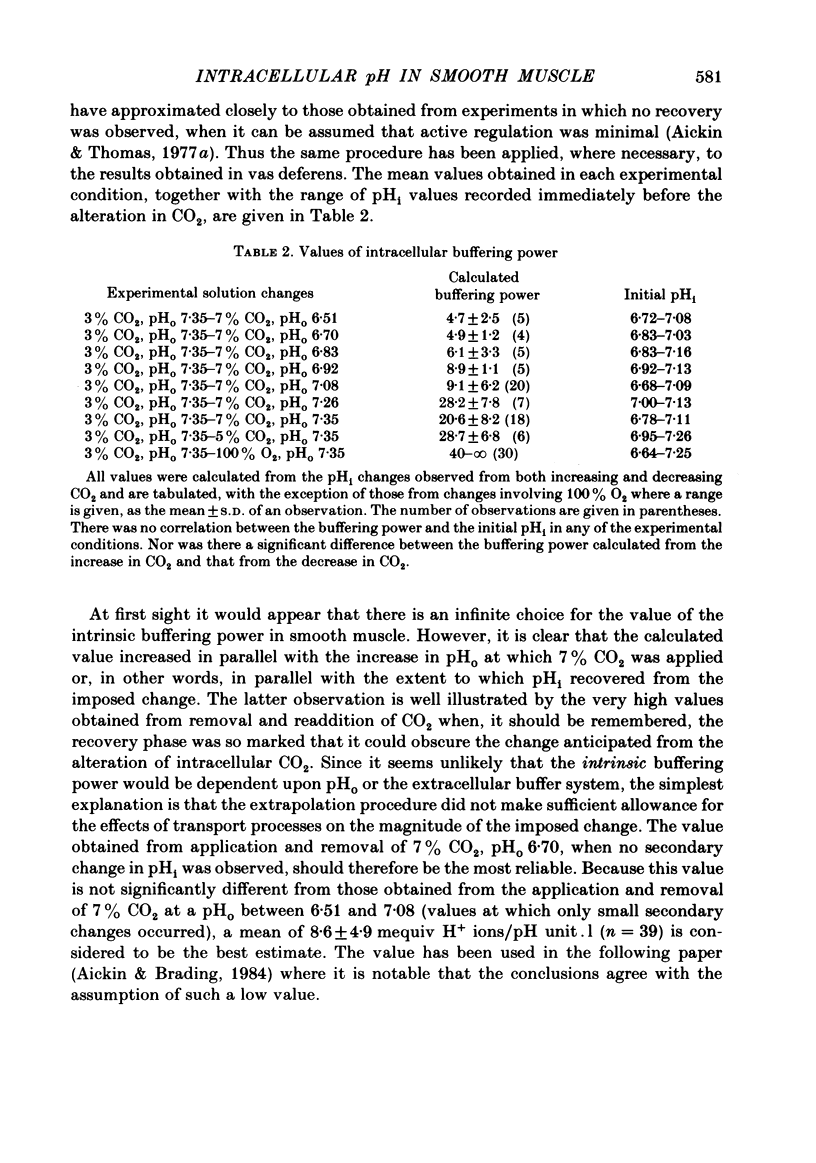
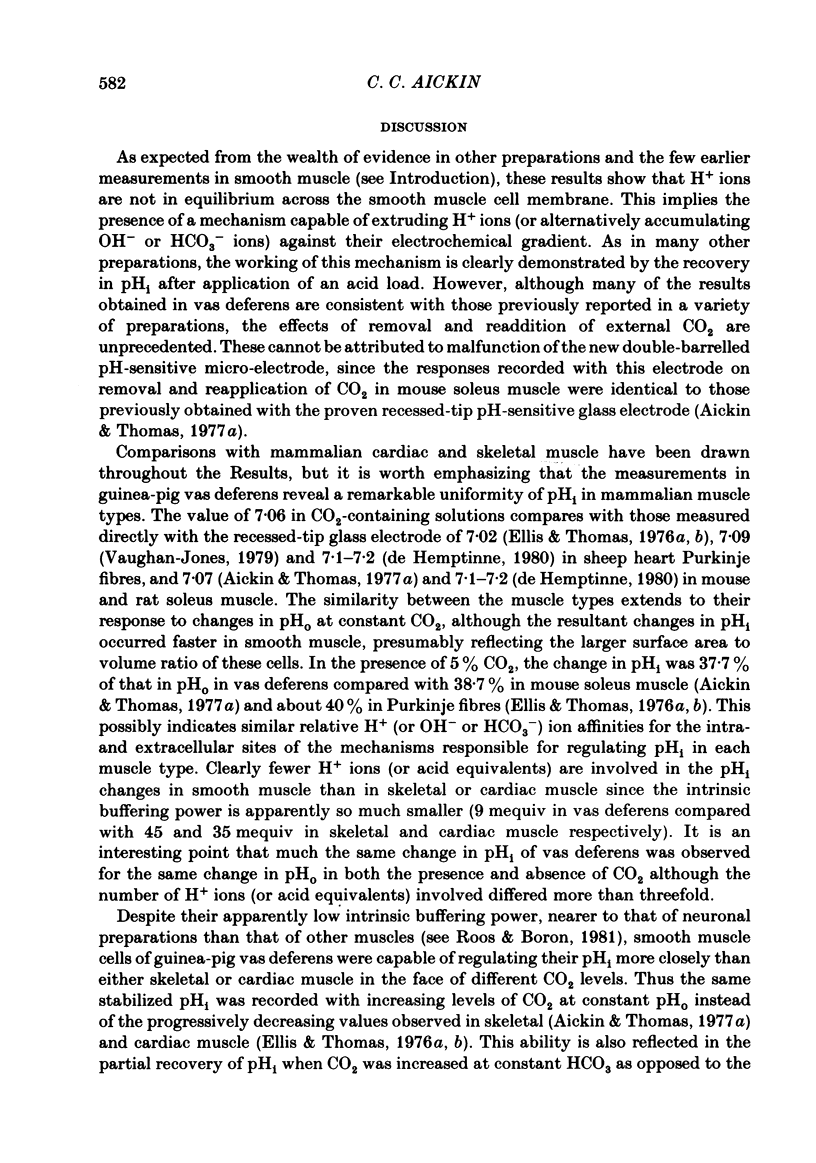
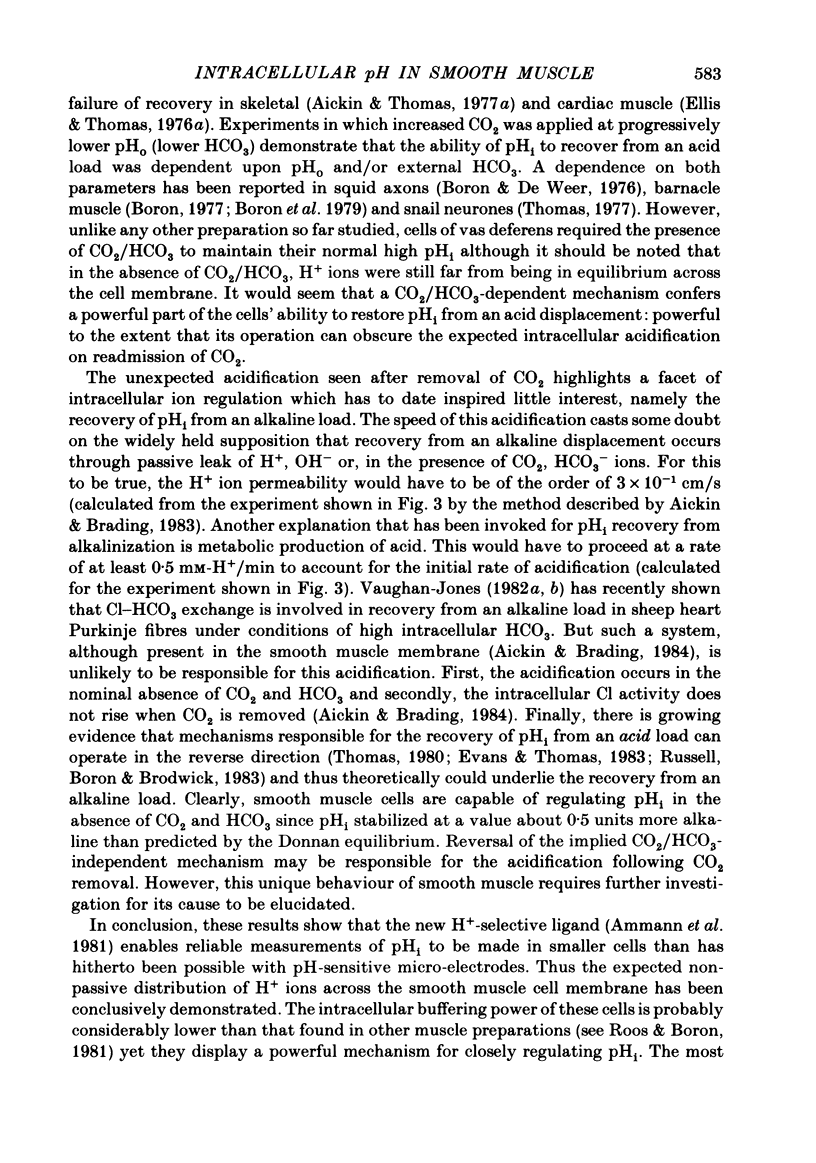
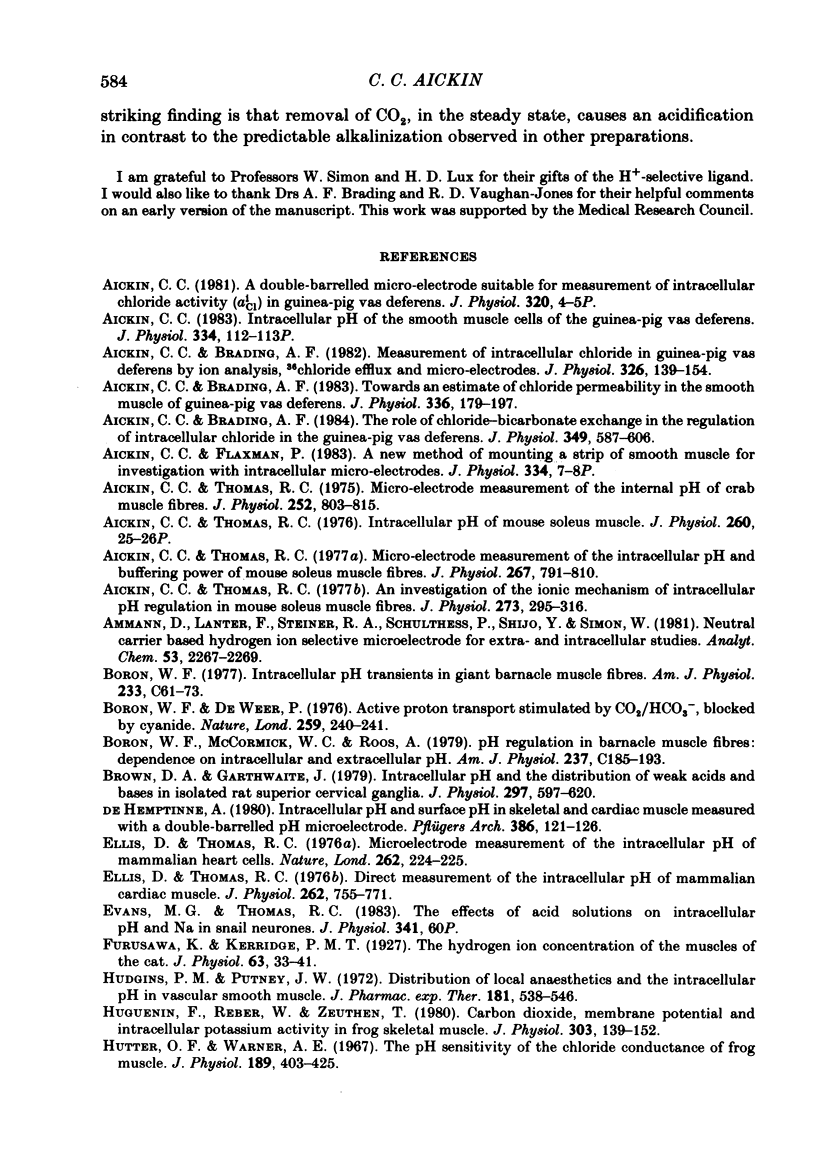
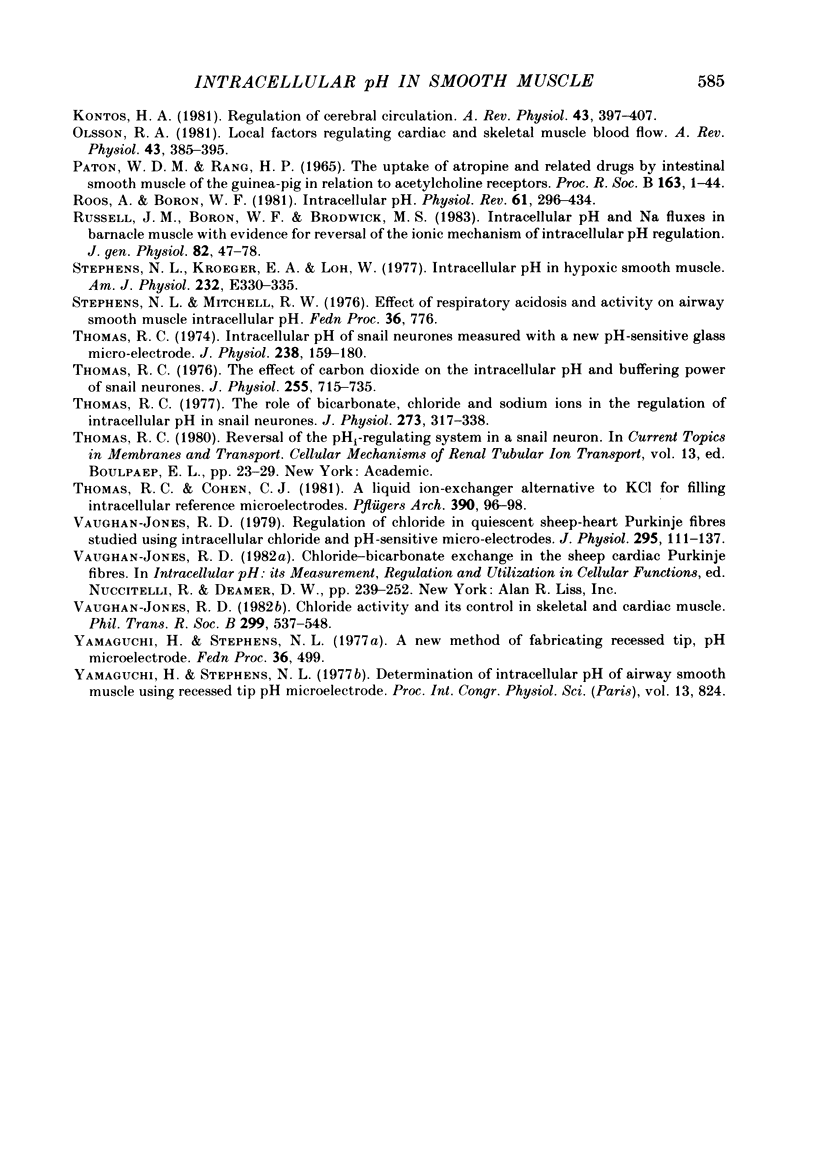
Selected References
These references are in PubMed. This may not be the complete list of references from this article.
- Aickin C. C., Brading A. F. Measurement of intracellular chloride in guinea-pig vas deferens by ion analysis, 36chloride efflux and micro-electrodes. J Physiol. 1982 May;326:139–154. doi: 10.1113/jphysiol.1982.sp014182. [DOI] [PMC free article] [PubMed] [Google Scholar]
- Aickin C. C., Brading A. F. The role of chloride-bicarbonate exchange in the regulation of intracellular chloride in guinea-pig vas deferens. J Physiol. 1984 Apr;349:587–606. doi: 10.1113/jphysiol.1984.sp015175. [DOI] [PMC free article] [PubMed] [Google Scholar]
- Aickin C. C., Brading A. F. Towards an estimate of chloride permeability in the smooth muscle of guinea-pig vas deferens. J Physiol. 1983 Mar;336:179–197. doi: 10.1113/jphysiol.1983.sp014575. [DOI] [PMC free article] [PubMed] [Google Scholar]
- Aickin C. C., Thomas R. C. An investigation of the ionic mechanism of intracellular pH regulation in mouse soleus muscle fibres. J Physiol. 1977 Dec;273(1):295–316. doi: 10.1113/jphysiol.1977.sp012095. [DOI] [PMC free article] [PubMed] [Google Scholar]
- Aickin C. C., Thomas R. C. Intracellular pH of mouse soleus muscle [proceedings]. J Physiol. 1976 Sep;260(2):25P–26P. [PMC free article] [PubMed] [Google Scholar]
- Aickin C. C., Thomas R. C. Micro-electrode measurement of the internal pH of crab muscle fibres. J Physiol. 1975 Nov;252(3):803–815. doi: 10.1113/jphysiol.1975.sp011171. [DOI] [PMC free article] [PubMed] [Google Scholar]
- Ammann D., Lanter F., Steiner R. A., Schulthess P., Shijo Y., Simon W. Neutral carrier based hydrogen ion selective microelectrode for extra- and intracellular studies. Anal Chem. 1981 Dec;53(14):2267–2269. doi: 10.1021/ac00237a031. [DOI] [PubMed] [Google Scholar]
- Boron W. F., De Weer P. Active proton transport stimulated by CO2/HCO3-, blocked by cyanide. Nature. 1976 Jan 22;259(5540):240–241. doi: 10.1038/259240a0. [DOI] [PubMed] [Google Scholar]
- Boron W. F. Intracellular pH transients in giant barnacle muscle fibers. Am J Physiol. 1977 Sep;233(3):C61–C73. doi: 10.1152/ajpcell.1977.233.3.C61. [DOI] [PubMed] [Google Scholar]
- Boron W. F., McCormick W. C., Roos A. pH regulation in barnacle muscle fibers: dependence on intracellular and extracellular pH. Am J Physiol. 1979 Sep;237(3):C185–C193. doi: 10.1152/ajpcell.1979.237.3.C185. [DOI] [PubMed] [Google Scholar]
- Brown D. A., Garthwaite J. Intracellular pH and the distribution of weak acids and bases in isolated rat superior cervical ganglia. J Physiol. 1979 Dec;297(0):597–620. doi: 10.1113/jphysiol.1979.sp013059. [DOI] [PMC free article] [PubMed] [Google Scholar]
- Ellis D., Thomas R. C. Direct measurement of the intracellular pH of mammalian cardiac muscle. J Physiol. 1976 Nov;262(3):755–771. doi: 10.1113/jphysiol.1976.sp011619. [DOI] [PMC free article] [PubMed] [Google Scholar]
- Ellis D., Thomas R. C. Microelectrode measurement of the intracellular pH of mammalian heart cells. Nature. 1976 Jul 15;262(5565):224–225. doi: 10.1038/262224a0. [DOI] [PubMed] [Google Scholar]
- Furusawa K., Kerridge P. M. The hydrogen ion concentration of the muscles of the cat. J Physiol. 1927 Jun 7;63(1):33–41. doi: 10.1113/jphysiol.1927.sp002378. [DOI] [PMC free article] [PubMed] [Google Scholar]
- Hudgins P. M., Putney J. W., Jr Distribution of local anesthetics and the intracellular pH in vascular smooth muscle. J Pharmacol Exp Ther. 1972 Jun;181(3):538–546. [PubMed] [Google Scholar]
- Huguenin F., Reber W., Zeuthen T. Carbon dioxide, membrane potential and intracellular potassium activity in frog skeletal muscle. J Physiol. 1980 Jun;303:139–152. doi: 10.1113/jphysiol.1980.sp013276. [DOI] [PMC free article] [PubMed] [Google Scholar]
- Hutter O. F., Warner A. E. The pH sensitivity of the chloride conductance of frog skeletal muscle. J Physiol. 1967 Apr;189(3):403–425. doi: 10.1113/jphysiol.1967.sp008176. [DOI] [PMC free article] [PubMed] [Google Scholar]
- Kontos H. A. Regulation of the cerebral circulation. Annu Rev Physiol. 1981;43:397–407. doi: 10.1146/annurev.ph.43.030181.002145. [DOI] [PubMed] [Google Scholar]
- Olsson R. A. Local factors regulating cardiac and skeletal muscle blood flow. Annu Rev Physiol. 1981;43:385–395. doi: 10.1146/annurev.ph.43.030181.002125. [DOI] [PubMed] [Google Scholar]
- PATON W. D., RANG H. P. THE UPTAKE OF ATROPINE AND RELATED DRUGS BY INTESTINAL SMOOTH MUSCLE OF THE GUINEA-PIG IN RELATION TO ACETYLCHOLINE RECEPTORS. Proc R Soc Lond B Biol Sci. 1965 Aug 24;163:1–44. doi: 10.1098/rspb.1965.0058. [DOI] [PubMed] [Google Scholar]
- Roos A., Boron W. F. Intracellular pH. Physiol Rev. 1981 Apr;61(2):296–434. doi: 10.1152/physrev.1981.61.2.296. [DOI] [PubMed] [Google Scholar]
- Russell J. M., Boron W. F., Brodwick M. S. Intracellular pH and Na fluxes in barnacle muscle with evidence for reversal of the ionic mechanism of intracellular pH regulation. J Gen Physiol. 1983 Jul;82(1):47–78. doi: 10.1085/jgp.82.1.47. [DOI] [PMC free article] [PubMed] [Google Scholar]
- Stephens N. L., Kroeger E. A., Loh W. Intracellular pH in hypoxic smooth muscle. Am J Physiol. 1977 Mar;232(3):E330–E335. doi: 10.1152/ajpendo.1977.232.3.E330. [DOI] [PubMed] [Google Scholar]
- Thomas R. C., Cohen C. J. A liquid ion-exchanger alternative to KCl for filling intracellular reference microelectrodes. Pflugers Arch. 1981 Apr;390(1):96–98. doi: 10.1007/BF00582719. [DOI] [PubMed] [Google Scholar]
- Thomas R. C. Intracellular pH of snail neurones measured with a new pH-sensitive glass mirco-electrode. J Physiol. 1974 Apr;238(1):159–180. doi: 10.1113/jphysiol.1974.sp010516. [DOI] [PMC free article] [PubMed] [Google Scholar]
- Thomas R. C. The effect of carbon dioxide on the intracellular pH and buffering power of snail neurones. J Physiol. 1976 Mar;255(3):715–735. doi: 10.1113/jphysiol.1976.sp011305. [DOI] [PMC free article] [PubMed] [Google Scholar]
- Thomas R. C. The role of bicarbonate, chloride and sodium ions in the regulation of intracellular pH in snail neurones. J Physiol. 1977 Dec;273(1):317–338. doi: 10.1113/jphysiol.1977.sp012096. [DOI] [PMC free article] [PubMed] [Google Scholar]
- Vaughan-Jones R. D. Chloride activity and its control in skeletal and cardiac muscle. Philos Trans R Soc Lond B Biol Sci. 1982 Dec 1;299(1097):537–548. doi: 10.1098/rstb.1982.0150. [DOI] [PubMed] [Google Scholar]
- Vaughan-Jones R. D. Regulation of chloride in quiescent sheep-heart Purkinje fibres studied using intracellular chloride and pH-sensitive micro-electrodes. J Physiol. 1979 Oct;295:111–137. doi: 10.1113/jphysiol.1979.sp012957. [DOI] [PMC free article] [PubMed] [Google Scholar]
- de Hemptinne A. Intracellular pH and surface pH in skeletal and cardiac muscle measured with a double-barrelled pH microelectrode. Pflugers Arch. 1980 Jul;386(2):121–126. doi: 10.1007/BF00584198. [DOI] [PubMed] [Google Scholar]