Abstract
Single ventricular cells were enzymatically isolated from adult guinea-pig hearts (Isenberg & Klöckner, 1982). The patch-clamp technique (Hamill, Marty, Neher, Sakmann & Sigworth, 1981) was used to examine the conductance properties of an inward-rectifying K+ channel present in their sarcolemmal membrane. When the K+ concentration on the extracellular side of the patch was between 10.8 and 300 mM, inward current steps were observed at potentials more negative than the K+ equilibrium potential (EK). At more positive potentials no current steps were detectable, demonstrating the strong rectification of the channel. The zero-current potential extrapolated from the voltage dependence of the inward currents depends on the external K4 concentration [K+]o in a fashion expected for a predominantly K+-selective ion channel. It is shifted by 49 mV for a tenfold change in [K+]o. The conductance of the channel depends on the square root of [K+]o. In approximately symmetrical transmembrane K+ concentrations (145 mM-external K+), the single-channel conductance is 27 pS (at 19-23 degrees C). In normal Tyrode solution (5.4 mM-external K+) we calculate a single-channel conductance of 3.6 pS. The size of inward current steps at a fixed negative membrane potential V increases with [K+]o. The relation between step size and [K+]o shows saturation. Assuming a Michaelis-Menten scheme for binding of permeating K+ to the channel, an apparent binding constant of 210 mM is calculated for a membrane potential of -100 mV. For this potential the current at saturating [K+]o is estimated as 6.5 pA. The rectification of the single-channel conductance at membrane potentials positive to EK occurs within 1.5 ms of stepping the membrane potential from a potential of high conductance to one of low conductance. In addition to the main conductance state, the channel can adopt several substates of conductance. The main state could be the result of the simultaneous opening of four conducting subunits, each of which has a conductance of about 7 pS in 145 mM-external K+. The density of the inward-rectifying K+ channels in the ventricular sarcolemma is 0-10 channel/10 micron2 of surface membrane; the average of twenty-eight patches was 1 channel/1.8 micron2. It is concluded that the inward-rectifying K+ channels mediate the resting K+ conductance of ventricular heart muscle and the current termed IK1 in conventional voltage-clamp experiments.
Full text
PDF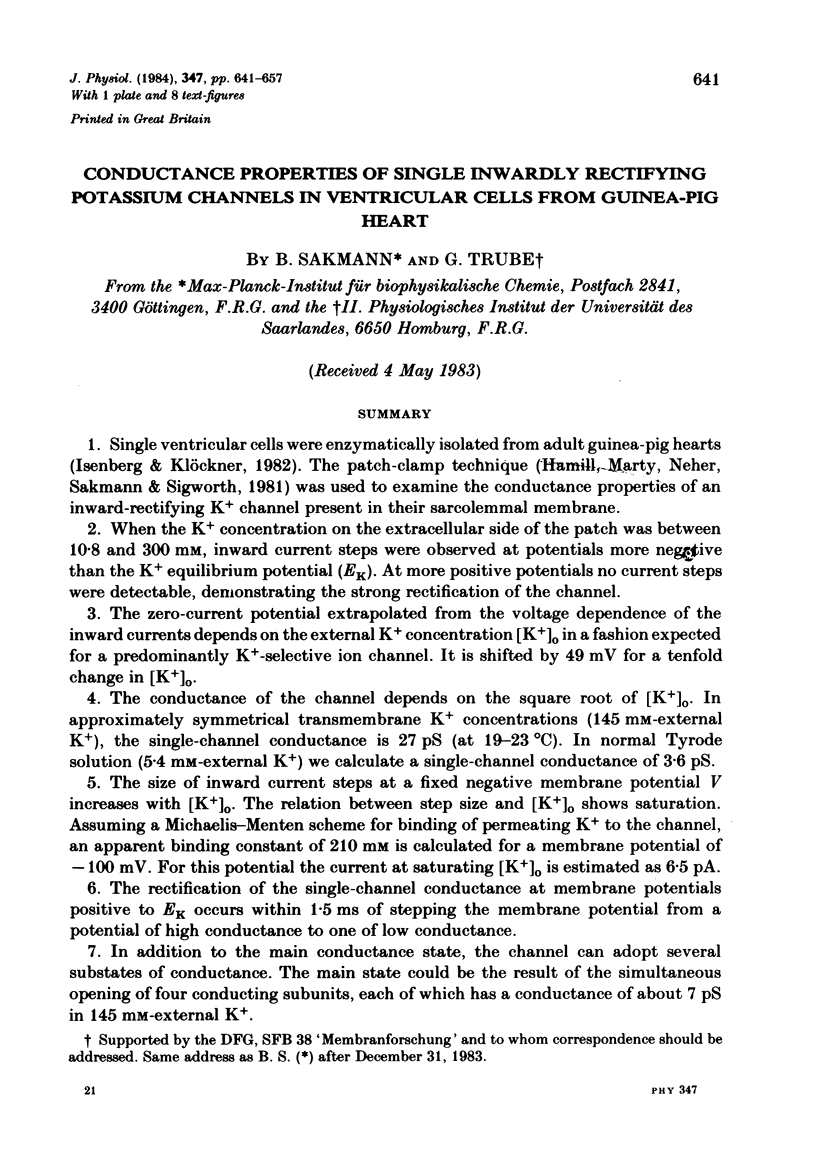
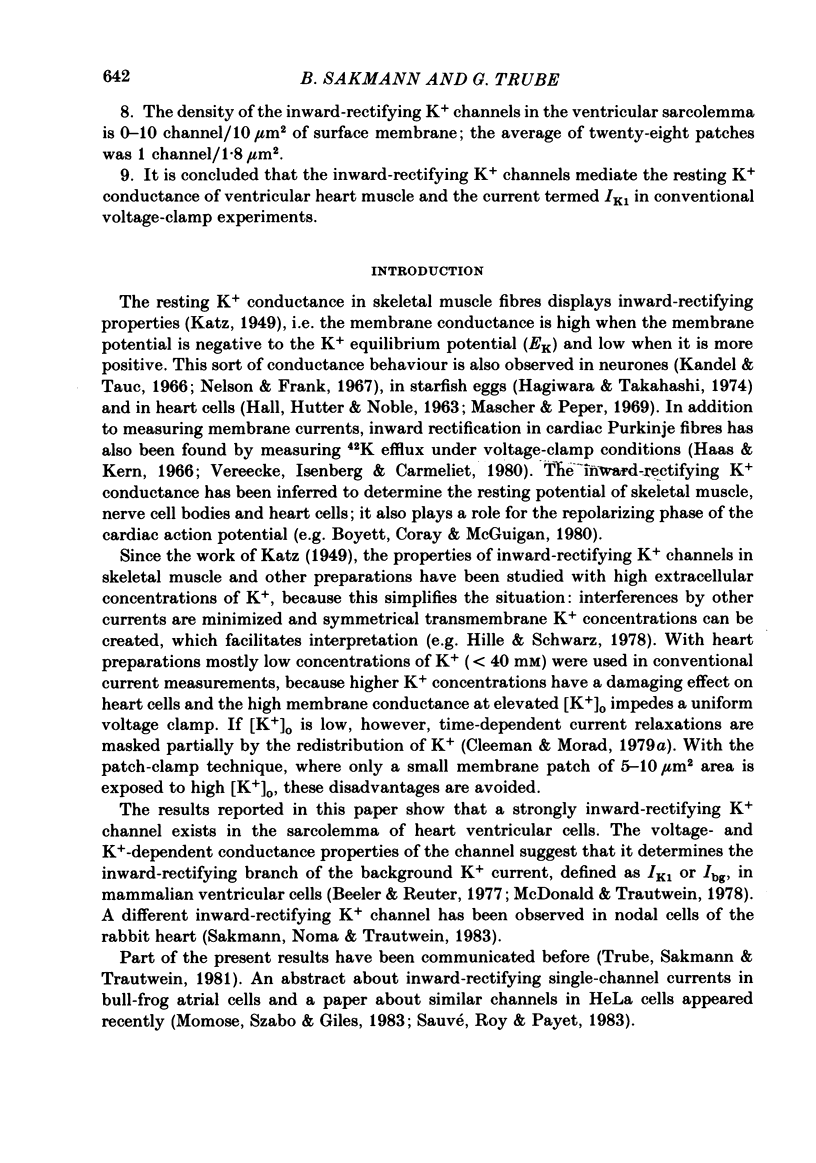
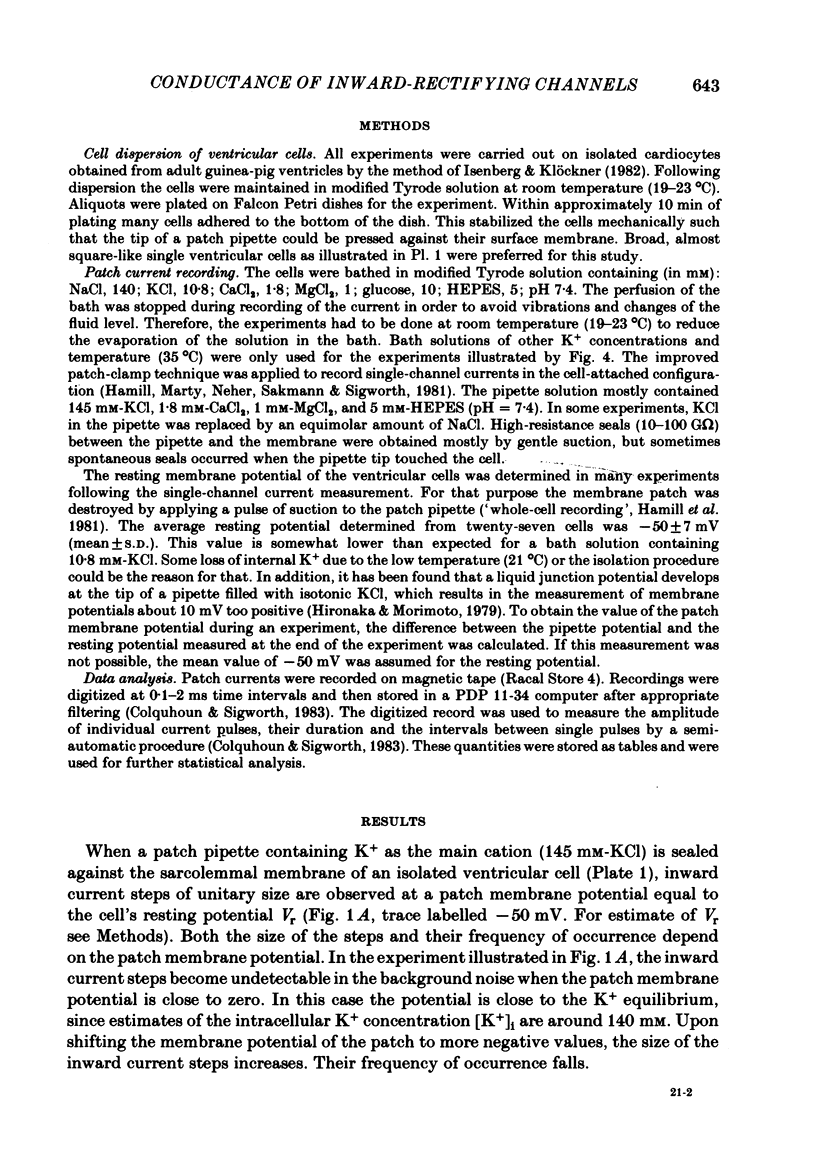
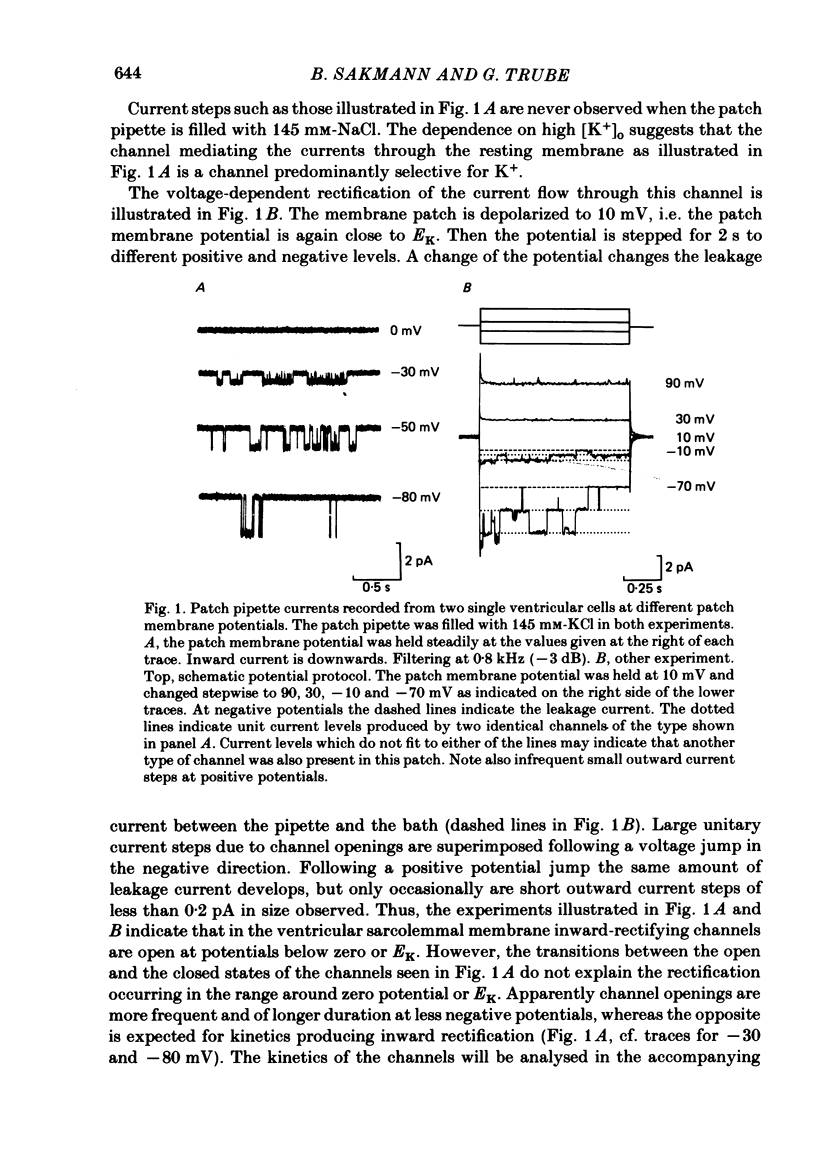
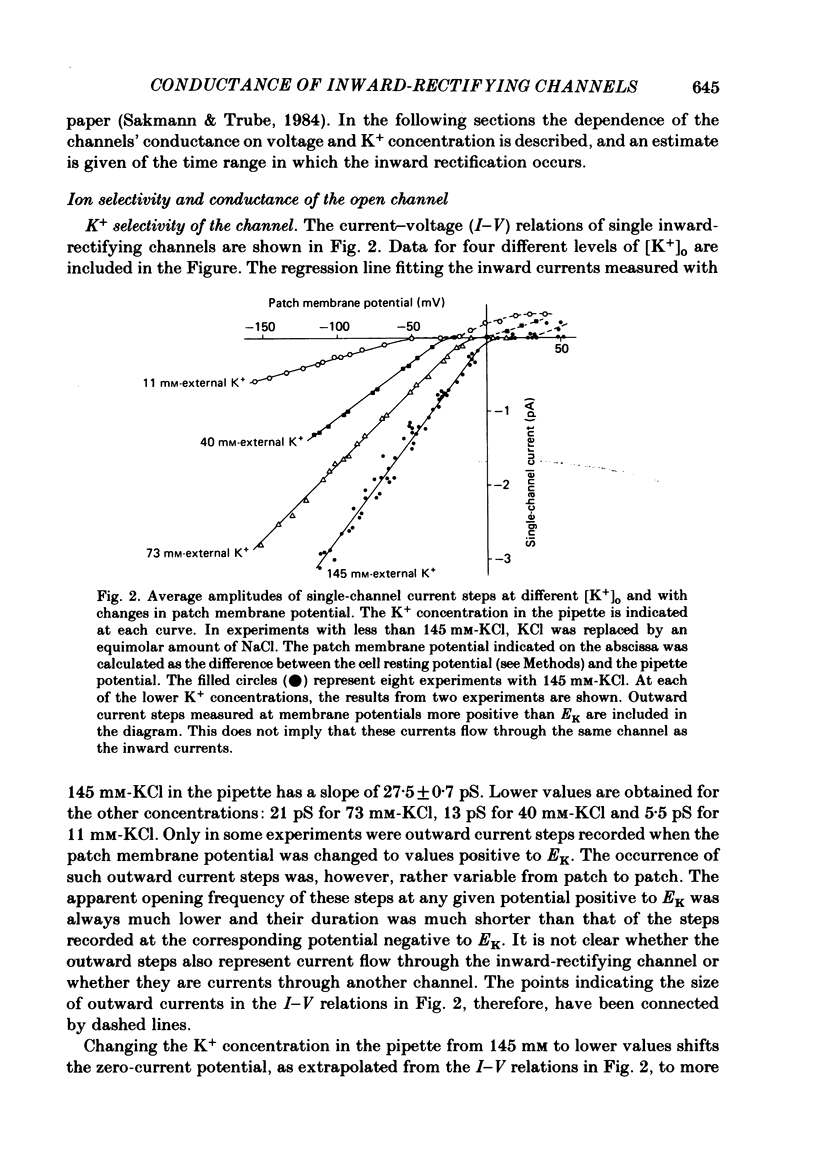
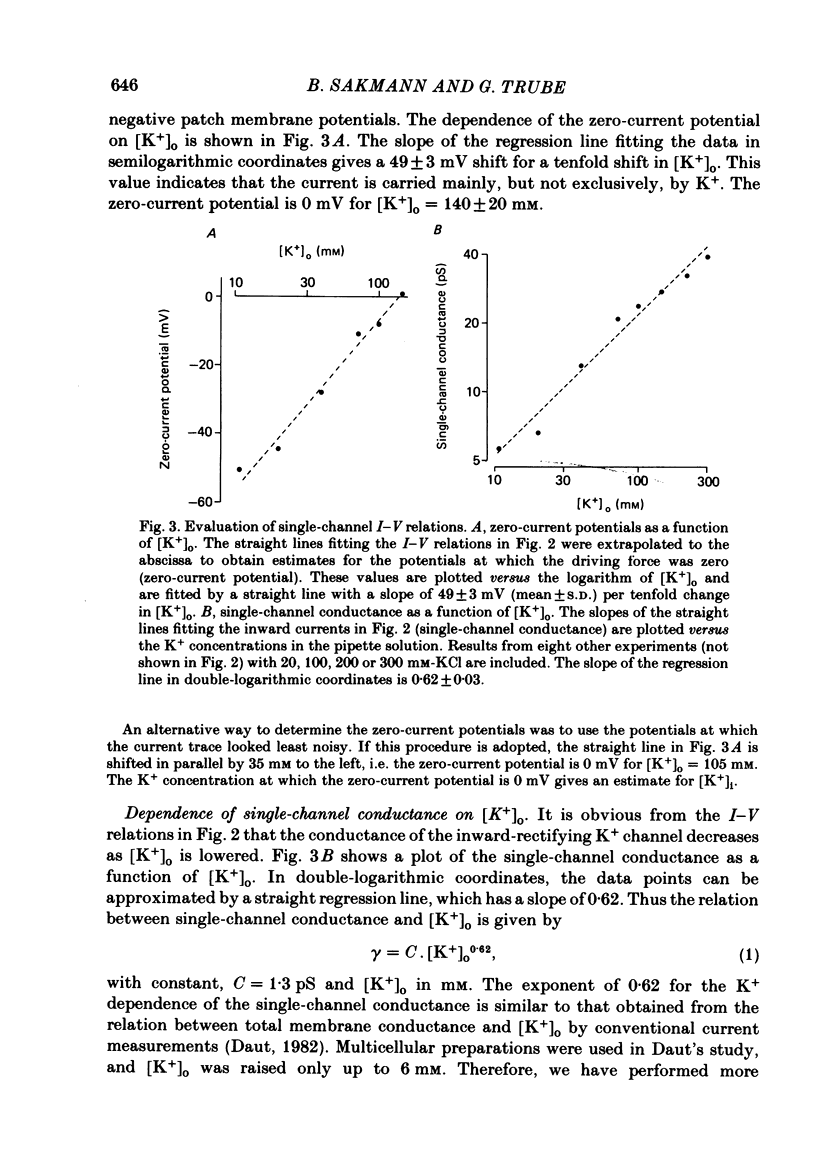
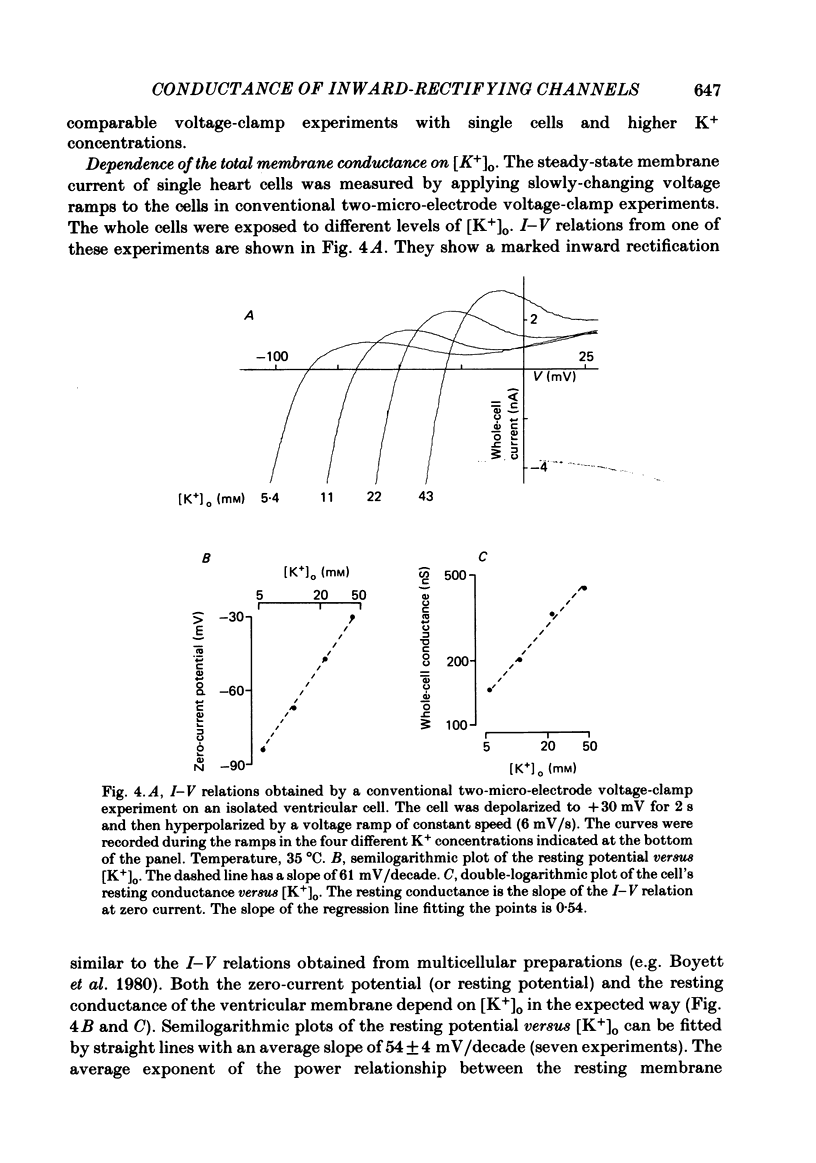
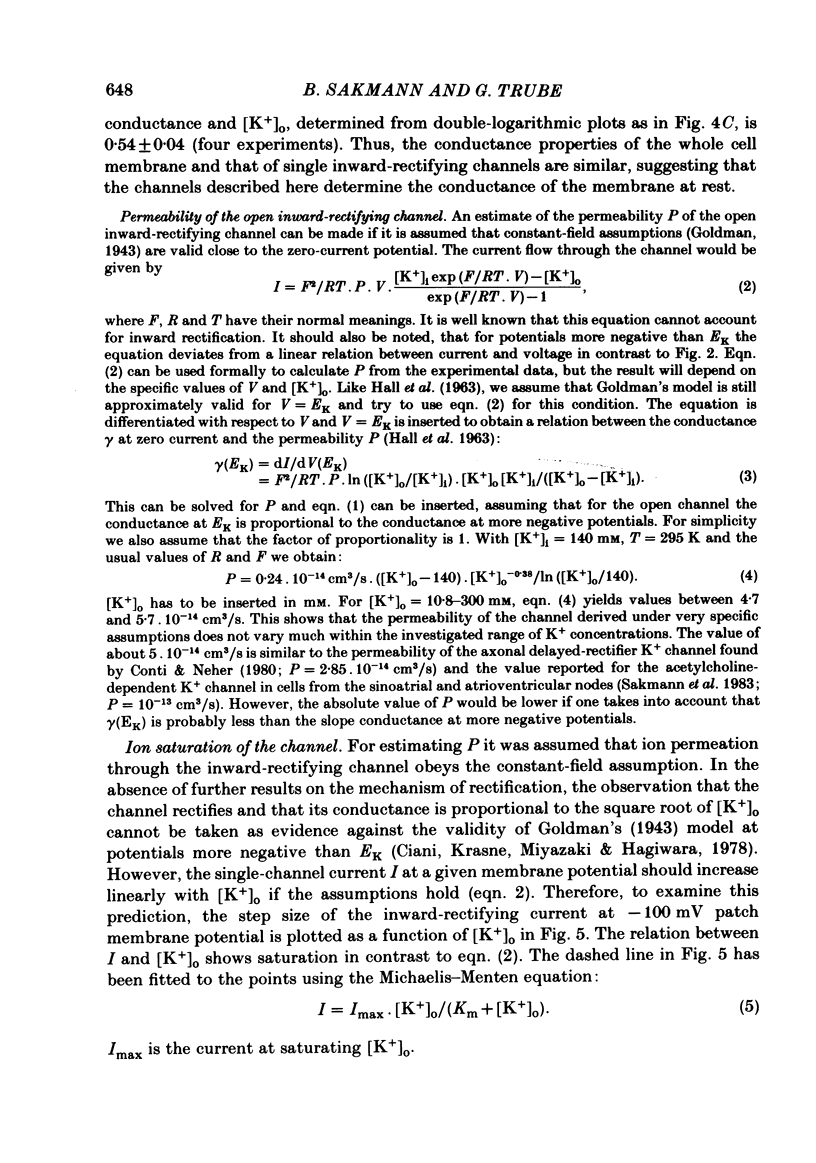
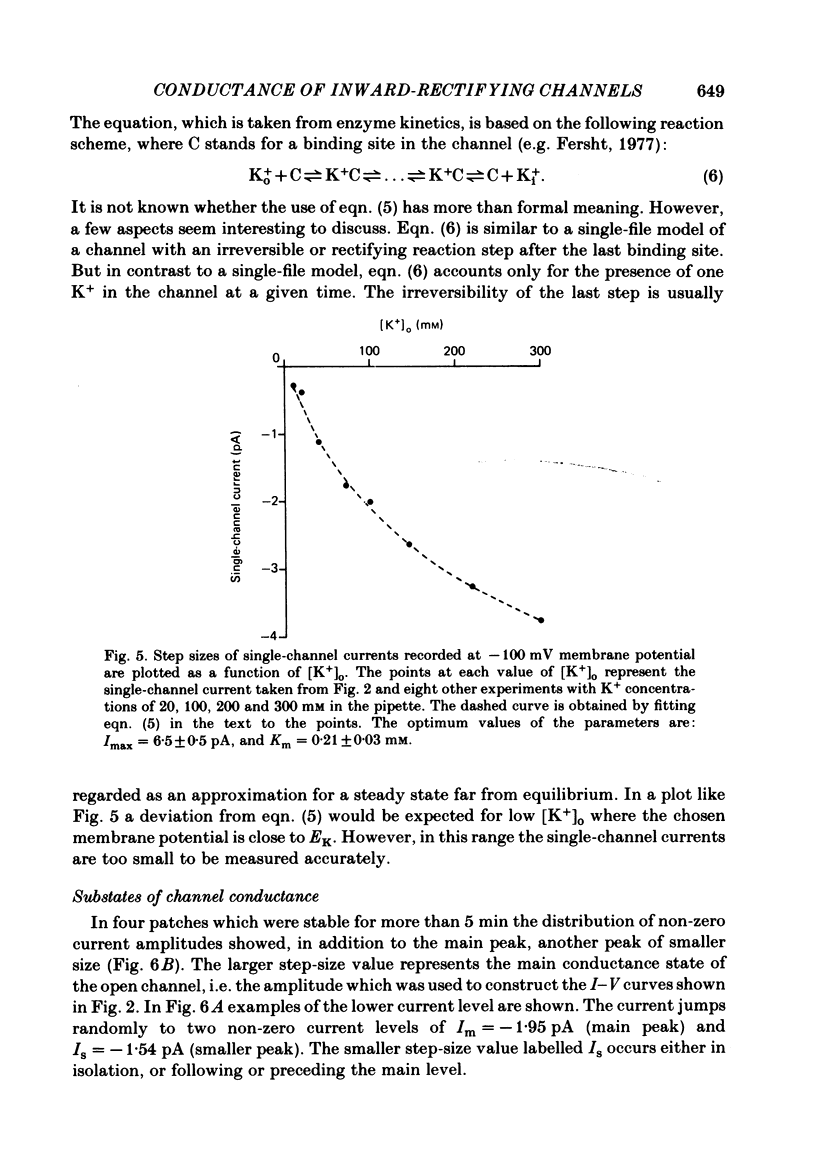
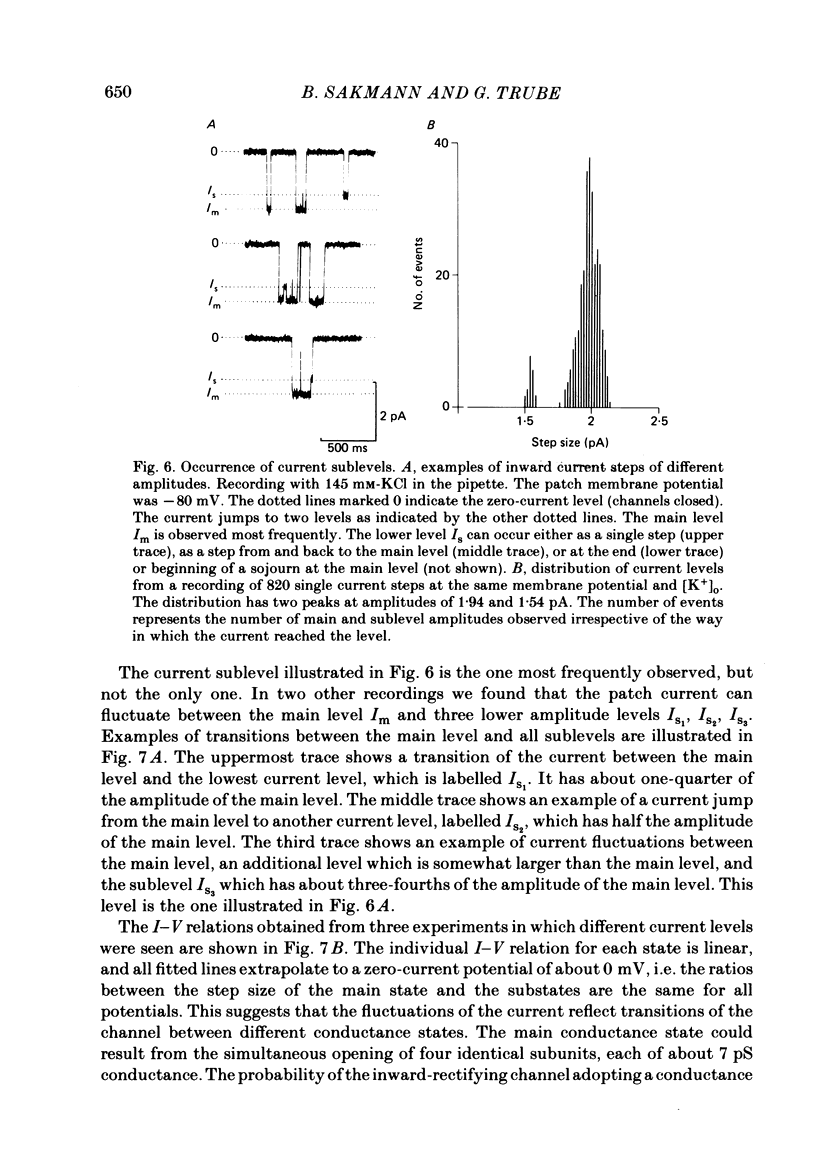
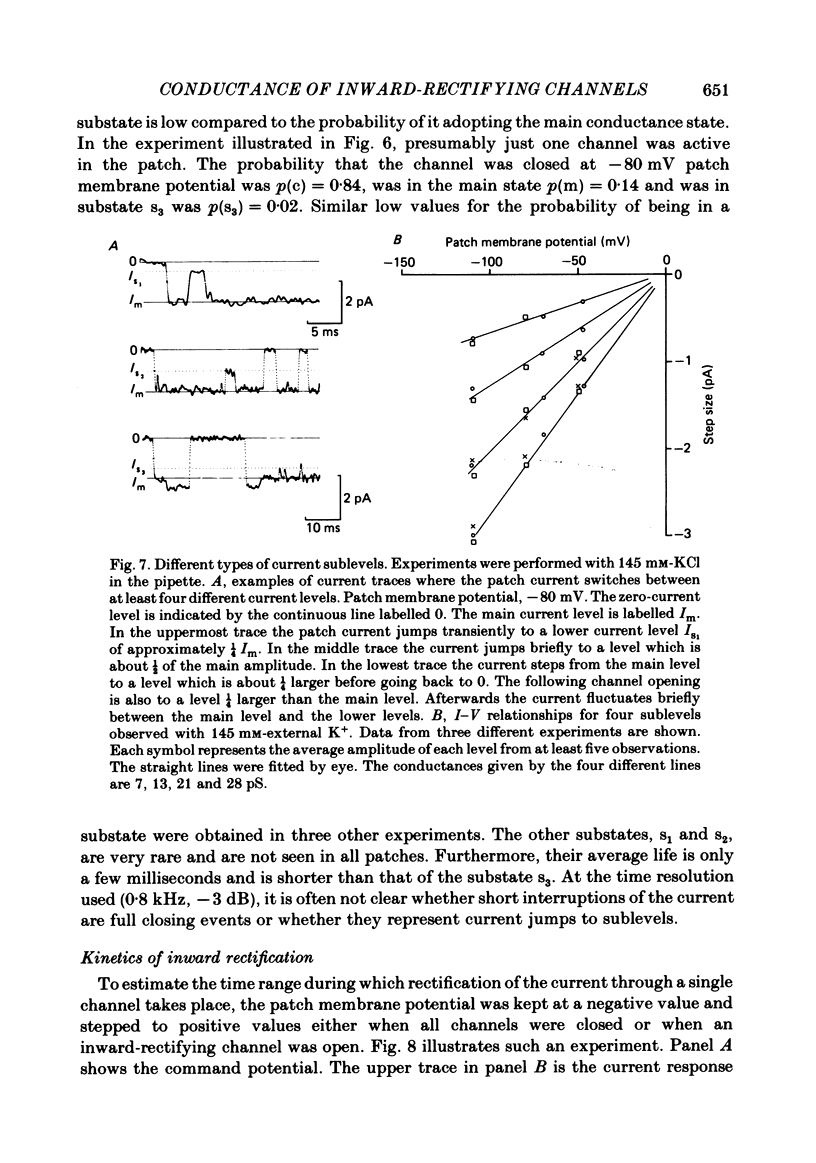
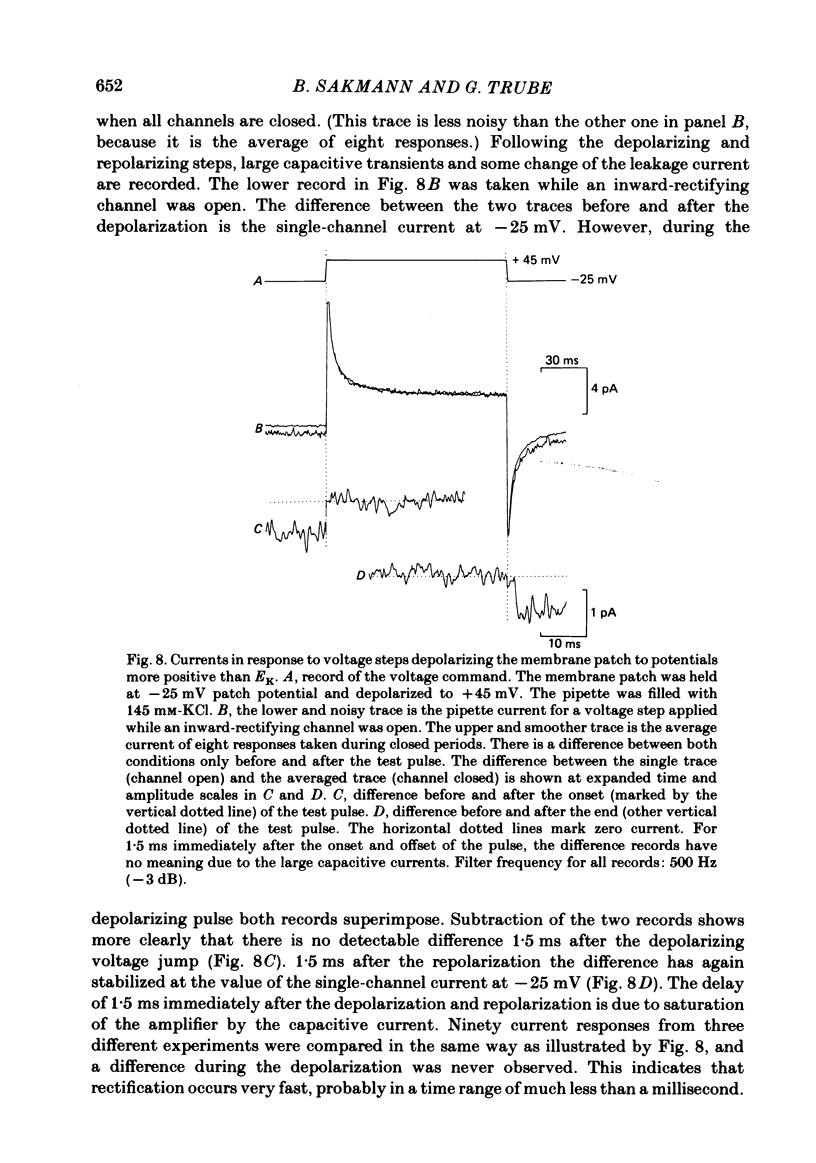
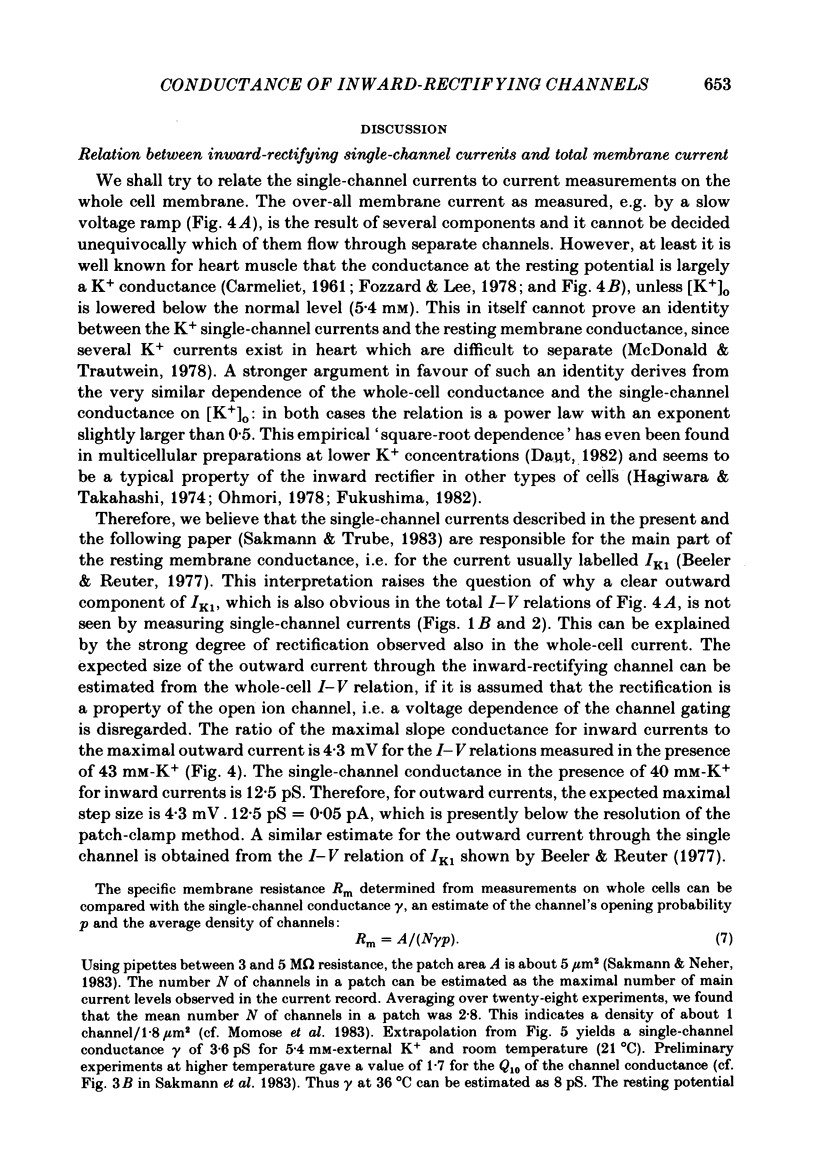
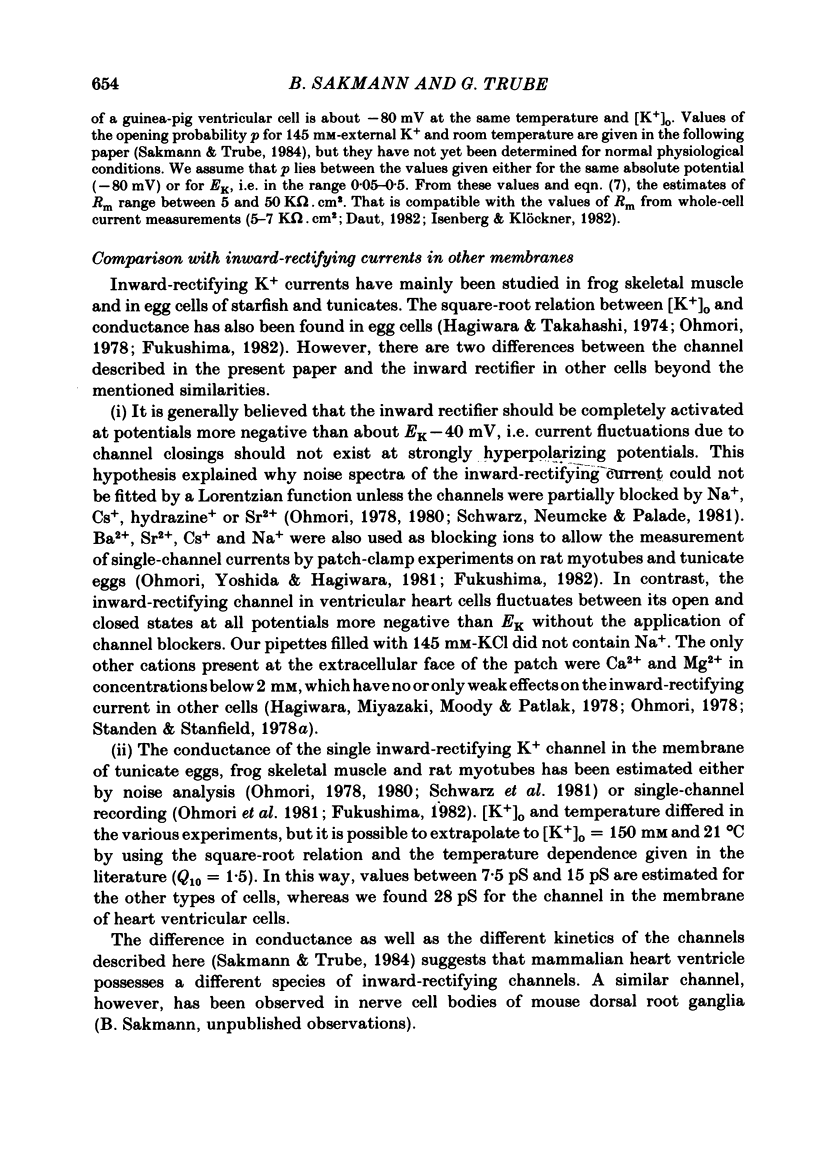
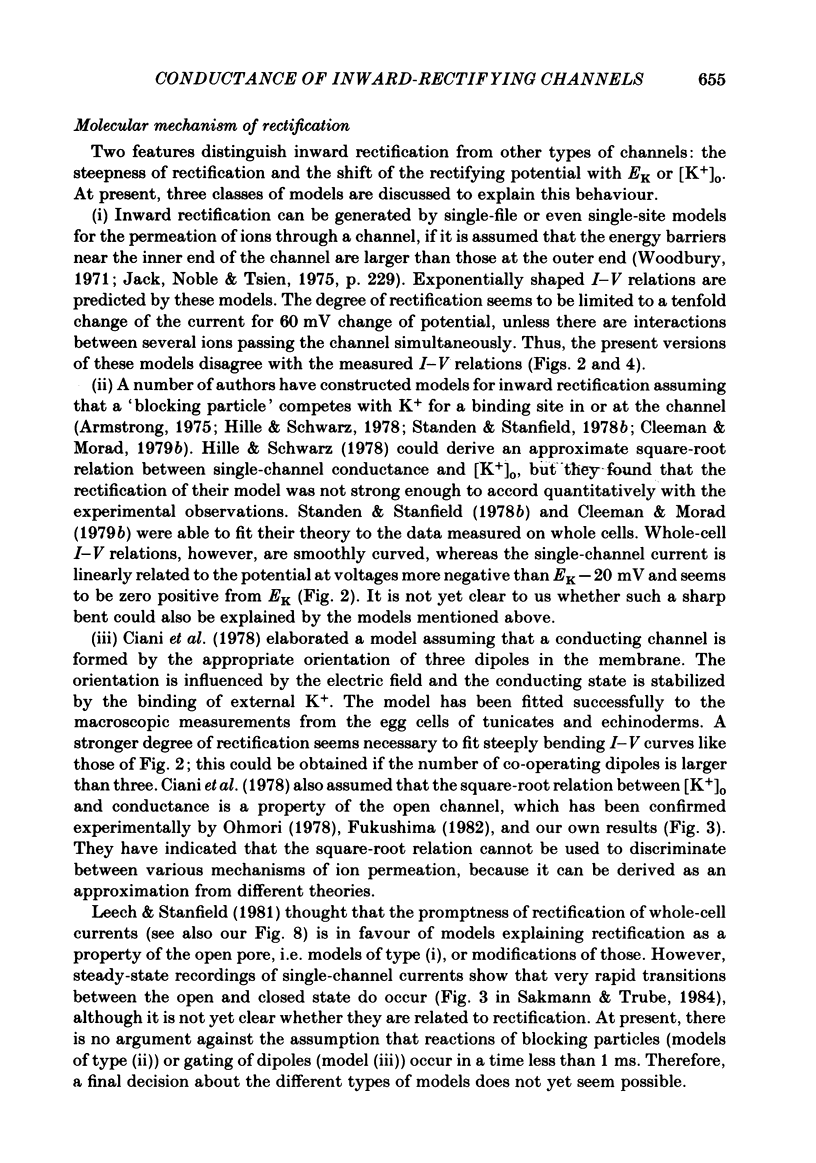
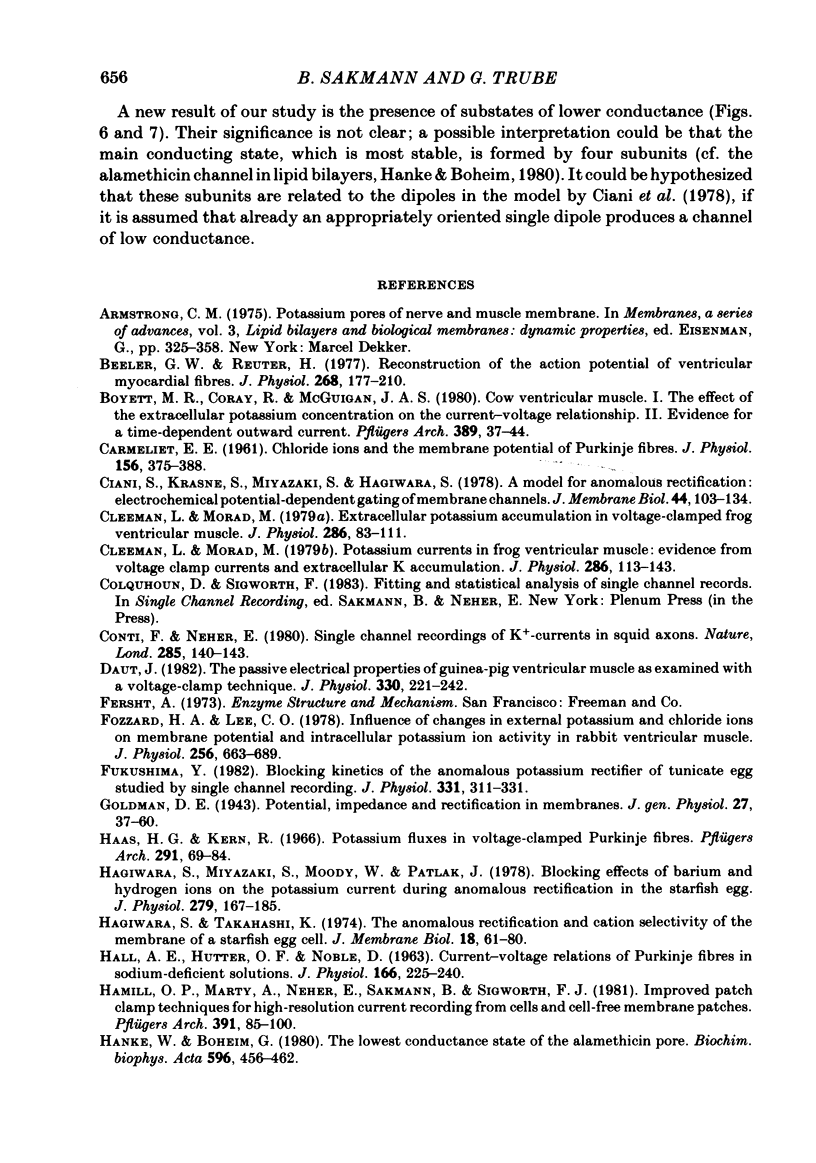
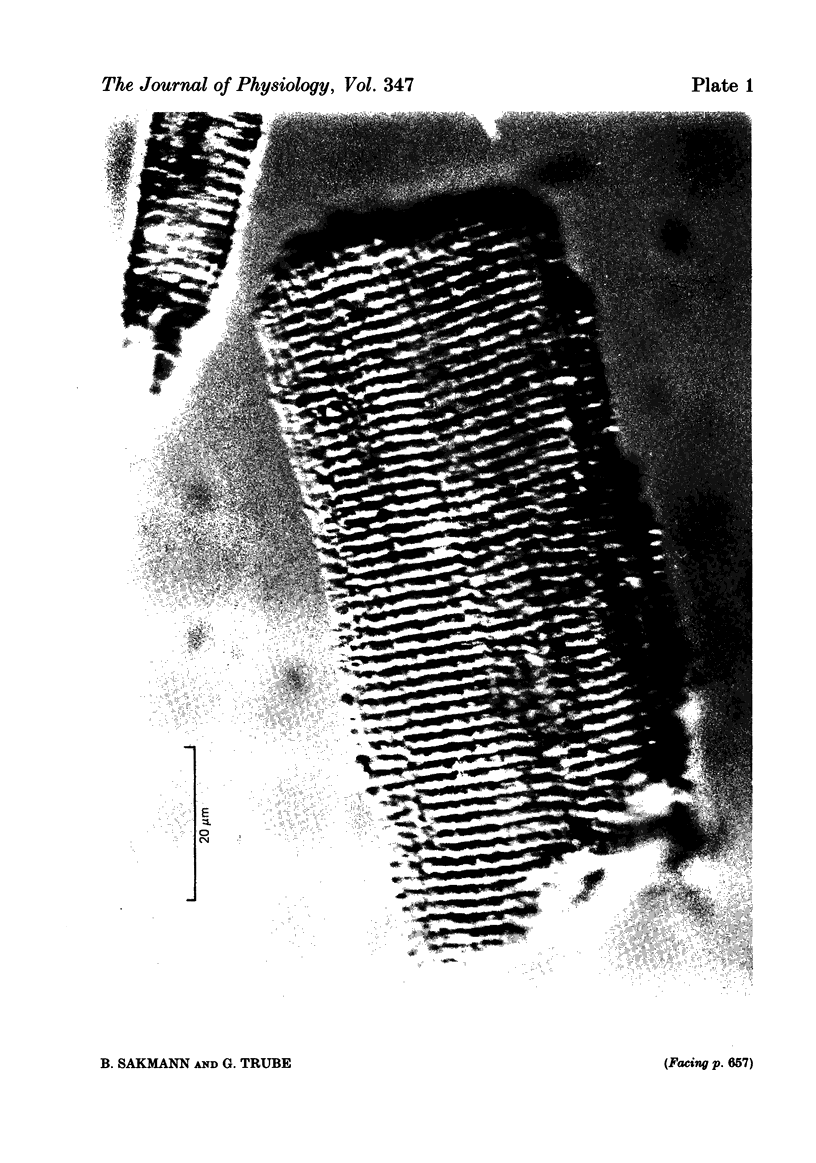
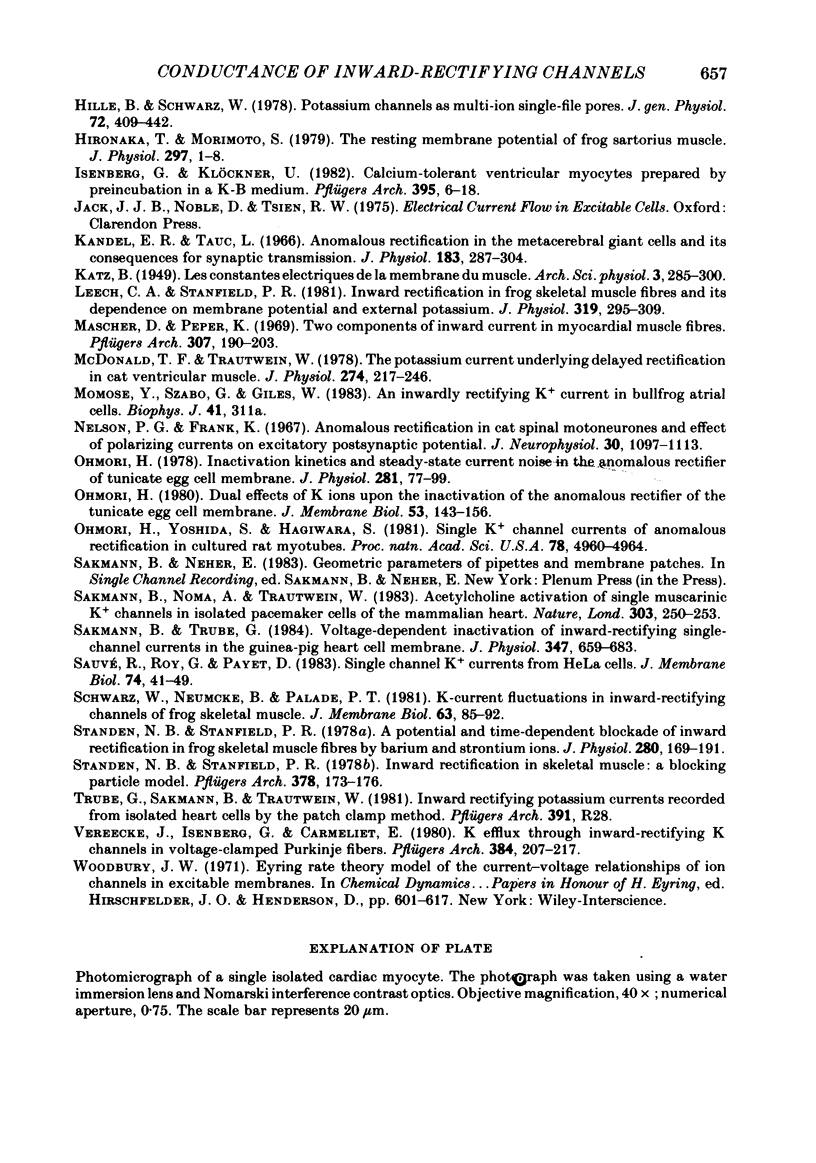
Images in this article
Selected References
These references are in PubMed. This may not be the complete list of references from this article.
- Beeler G. W., Reuter H. Reconstruction of the action potential of ventricular myocardial fibres. J Physiol. 1977 Jun;268(1):177–210. doi: 10.1113/jphysiol.1977.sp011853. [DOI] [PMC free article] [PubMed] [Google Scholar]
- Boyett M. R., Coray A., McGuigan J. A. Cow ventricular muscle. I. The effect of the extracellular potassium concentration on the current-voltage relationship. II. Evidence for a time-dependent outward current. Pflugers Arch. 1980 Dec;389(1):37–44. doi: 10.1007/BF00587926. [DOI] [PubMed] [Google Scholar]
- CARMELIET E. E. Chloride ions and the membrane potential of Purkinje fibres. J Physiol. 1961 Apr;156:375–388. doi: 10.1113/jphysiol.1961.sp006682. [DOI] [PMC free article] [PubMed] [Google Scholar]
- Ciani S., Krasne S., Miyazaki S., Hagiwara S. A model for anomalous rectification: electrochemical-potential-dependent gating of membrane channels. J Membr Biol. 1978 Dec 15;44(2):103–134. doi: 10.1007/BF01976035. [DOI] [PubMed] [Google Scholar]
- Cleemann L., Morad M. Extracellular potassium accumulation in voltage-clamped frog ventricular muscle. J Physiol. 1979 Jan;286:83–111. doi: 10.1113/jphysiol.1979.sp012608. [DOI] [PMC free article] [PubMed] [Google Scholar]
- Cleemann L., Morad M. Potassium currents in frog ventricular muscle: evidence from voltage clamp currents and extracellular K accumulation. J Physiol. 1979 Jan;286:113–143. doi: 10.1113/jphysiol.1979.sp012609. [DOI] [PMC free article] [PubMed] [Google Scholar]
- Conti F., Neher E. Single channel recordings of K+ currents in squid axons. Nature. 1980 May 15;285(5761):140–143. doi: 10.1038/285140a0. [DOI] [PubMed] [Google Scholar]
- Daut J. The passive electrical properties of guinea-pig ventricular muscle as examined with a voltage-clamp technique. J Physiol. 1982 Sep;330:221–242. doi: 10.1113/jphysiol.1982.sp014338. [DOI] [PMC free article] [PubMed] [Google Scholar]
- Fozzard H. A., Lee C. O. Influence of changes in external potassium and chloride ions on membrane potential and intracellular potassium ion activity in rabbit ventricular muscle. J Physiol. 1976 Apr;256(3):663–689. doi: 10.1113/jphysiol.1976.sp011345. [DOI] [PMC free article] [PubMed] [Google Scholar]
- Fukushima Y. Blocking kinetics of the anomalous potassium rectifier of tunicate egg studied by single channel recording. J Physiol. 1982 Oct;331:311–331. doi: 10.1113/jphysiol.1982.sp014374. [DOI] [PMC free article] [PubMed] [Google Scholar]
- Goldman D. E. POTENTIAL, IMPEDANCE, AND RECTIFICATION IN MEMBRANES. J Gen Physiol. 1943 Sep 20;27(1):37–60. doi: 10.1085/jgp.27.1.37. [DOI] [PMC free article] [PubMed] [Google Scholar]
- HALL A. E., HUTTER O. F., NOBLE D. Current-voltage relations of Purkinje fibres in sodium-deficient solutions. J Physiol. 1963 Apr;166:225–240. doi: 10.1113/jphysiol.1963.sp007102. [DOI] [PMC free article] [PubMed] [Google Scholar]
- Haas H. G., Kern R. Potassium fluxes in voltage clamped Purkinje fibres. Pflugers Arch Gesamte Physiol Menschen Tiere. 1966;291(1):69–84. doi: 10.1007/BF00362653. [DOI] [PubMed] [Google Scholar]
- Hagiwara S., Miyazaki S., Moody W., Patlak J. Blocking effects of barium and hydrogen ions on the potassium current during anomalous rectification in the starfish egg. J Physiol. 1978 Jun;279:167–185. doi: 10.1113/jphysiol.1978.sp012338. [DOI] [PMC free article] [PubMed] [Google Scholar]
- Hagiwara S., Takahashi K. The anomalous rectification and cation selectivity of the membrane of a starfish egg cell. J Membr Biol. 1974;18(1):61–80. doi: 10.1007/BF01870103. [DOI] [PubMed] [Google Scholar]
- Hamill O. P., Marty A., Neher E., Sakmann B., Sigworth F. J. Improved patch-clamp techniques for high-resolution current recording from cells and cell-free membrane patches. Pflugers Arch. 1981 Aug;391(2):85–100. doi: 10.1007/BF00656997. [DOI] [PubMed] [Google Scholar]
- Hanke W., Boheim G. The lowest conductance state of the alamethicin pore. Biochim Biophys Acta. 1980 Mar 13;596(3):456–462. doi: 10.1016/0005-2736(80)90134-0. [DOI] [PubMed] [Google Scholar]
- Hille B., Schwarz W. Potassium channels as multi-ion single-file pores. J Gen Physiol. 1978 Oct;72(4):409–442. doi: 10.1085/jgp.72.4.409. [DOI] [PMC free article] [PubMed] [Google Scholar]
- Hironaka T., Morimoto S. The resting membrane potential of frog sartorius muscle. J Physiol. 1979 Dec;297(0):1–8. doi: 10.1113/jphysiol.1979.sp013024. [DOI] [PMC free article] [PubMed] [Google Scholar]
- Isenberg G., Klockner U. Calcium tolerant ventricular myocytes prepared by preincubation in a "KB medium". Pflugers Arch. 1982 Oct;395(1):6–18. doi: 10.1007/BF00584963. [DOI] [PubMed] [Google Scholar]
- Kandel E. R., Tauc L. Anomalous rectification in the metacerebral giant cells and its consequences for synaptic transmission. J Physiol. 1966 Mar;183(2):287–304. doi: 10.1113/jphysiol.1966.sp007867. [DOI] [PMC free article] [PubMed] [Google Scholar]
- Leech C. A., Stanfield P. R. Inward rectification in frog skeletal muscle fibres and its dependence on membrane potential and external potassium. J Physiol. 1981;319:295–309. doi: 10.1113/jphysiol.1981.sp013909. [DOI] [PMC free article] [PubMed] [Google Scholar]
- Mascher D., Peper K. Two components of inward current in myocardial muscle fibers. Pflugers Arch. 1969;307(3):190–203. doi: 10.1007/BF00592084. [DOI] [PubMed] [Google Scholar]
- McDonald T. F., Trautwein W. The potassium current underlying delayed rectification in cat ventricular muscle. J Physiol. 1978 Jan;274:217–246. doi: 10.1113/jphysiol.1978.sp012144. [DOI] [PMC free article] [PubMed] [Google Scholar]
- Nelson P. G., Frank K. Anomalous rectification in cat spinal motoneurons and effect of polarizing currents on excitatory postsynaptic potential. J Neurophysiol. 1967 Sep;30(5):1097–1113. doi: 10.1152/jn.1967.30.5.1097. [DOI] [PubMed] [Google Scholar]
- Ohmori H. Inactivation kinetics and steady-state current noise in the anomalous rectifier of tunicate egg cell membranes. J Physiol. 1978 Aug;281:77–99. doi: 10.1113/jphysiol.1978.sp012410. [DOI] [PMC free article] [PubMed] [Google Scholar]
- Ohmori H., Yoshida S., Hagiwara S. Single K+ channel currents of anomalous rectification in cultured rat myotubes. Proc Natl Acad Sci U S A. 1981 Aug;78(8):4960–4964. doi: 10.1073/pnas.78.8.4960. [DOI] [PMC free article] [PubMed] [Google Scholar]
- Sakmann B., Noma A., Trautwein W. Acetylcholine activation of single muscarinic K+ channels in isolated pacemaker cells of the mammalian heart. Nature. 1983 May 19;303(5914):250–253. doi: 10.1038/303250a0. [DOI] [PubMed] [Google Scholar]
- Sakmann B., Trube G. Voltage-dependent inactivation of inward-rectifying single-channel currents in the guinea-pig heart cell membrane. J Physiol. 1984 Feb;347:659–683. doi: 10.1113/jphysiol.1984.sp015089. [DOI] [PMC free article] [PubMed] [Google Scholar]
- Sauvé R., Roy G., Payet D. Single channel K+ currents from HeLa cells. J Membr Biol. 1983;74(1):41–49. doi: 10.1007/BF01870593. [DOI] [PubMed] [Google Scholar]
- Schwarz W., Neumcke B., Palade P. T. K-current fluctuations in inward-rectifying channels of frog skeletal muscle. J Membr Biol. 1981;63(1-2):85–92. doi: 10.1007/BF01969449. [DOI] [PubMed] [Google Scholar]
- Standen N. B., Stanfield P. R. A potential- and time-dependent blockade of inward rectification in frog skeletal muscle fibres by barium and strontium ions. J Physiol. 1978 Jul;280:169–191. doi: 10.1113/jphysiol.1978.sp012379. [DOI] [PMC free article] [PubMed] [Google Scholar]
- Standen N. B., Stanfield P. R. Inward rectification in skeletal muscle: a blocking particle model. Pflugers Arch. 1978 Dec 28;378(2):173–176. doi: 10.1007/BF00584452. [DOI] [PubMed] [Google Scholar]
- Vereecke J., Isenberg G., Carmeliet E. K efflux through inward rectifying K channels in voltage clamped Purkinje fibers. Pflugers Arch. 1980 Apr;384(3):207–217. doi: 10.1007/BF00584555. [DOI] [PubMed] [Google Scholar]