Effective power in action is the true end of education, rather than the storing up of information.
Charles Eliot, president of Harvard, 1898
In spite of centuries of teaching biology, we biologists continue to be ensnared in attempts to define what parts of biology are essential for a literate nonscientist to know. Such discussions gain deeper urgency with the nearly annual reports of our students' shortcomings in tests that purportedly evaluate their understandings of math and science (e.g., see O'Sullivan, 2003). Are we failing our undergraduates? Will our shortcomings in teaching biology imperil their ability to live full lives? Will their misunderstandings or lack of knowledge about science imperil our democratic way of life and national security?
My own teaching of nonmajors has been both liberated and enriched by the realization that the answer to each of these questions is, “No.” As a result, I have abandoned the mistaken notion that unless I “cover” a particular list of “content,” my students will be unprepared for the future and I will have failed them as a teacher. I no longer agonize about losing valuable lecture time to in-class discussions, group problem solving, or other activities that take time away from covering content. Instead, I purposefully include such activities in order to offer a richer, more valuable learning experience. This change moves toward a “lived curriculum” (Hurd, 1998) that provides meaning well beyond the particular facts and even beyond biology. Rather than focusing on covering key facts or principles, a lived curriculum in nonmajors biology focuses on helping students learn to use scientific knowledge to solve relevant problems. Content mastery emerges naturally as students seek out, evaluate, and organize the information they need to develop an informed understanding about an issue such as the genetics of race, stem cell research, or invasive species. In the context of a lived curriculum, benchmarks for student learning become potential destinations for deep exploration rather than a roadmap for a 10- or 15-week hovercraft tour across the contours of biology. By focusing our efforts on developing intellectual skills rather than simply covering a list of facts, a lived curriculum will have a long-term positive impact on students' lives and their ability to function as informed citizens in a democratic society.
THE SCHOLARLY HISTORY OF SCIENCE LITERACY SHOULD INFORM OUR CURRENT EDUCATION EFFORTS
That men do not learn very much from the lessons of history is the most important of all the lessons that history has to teach.
Aldous Huxley, English novelist, 1959
The idea that knowledge about science has value beyond the practice of science is hardly modern, although we lose sight of this fact (for reviews, see DeBoer, 1991,2000; Hurd, 1998). Scientific literacy has been promoted as an important goal since at least 400 BC, when Plato was leading discussions at the Academy in Athens. The goals of biology literacy have similarly been a part of higher education in Western civilization for centuries (Rudolph, 1977). In keeping with this tradition, biology has been included in American college curricula since the founding of Harvard College in 1636 (Mather, 1907). Modeled on the curricula of Oxford and Cambridge at the time, the Harvard College curriculum required students to take botany courses in both sophomore and junior or senior years. In the forms of botany, zoology, and physiology, biology education has also been part of the formal elementary and high school curricula in the United States from its beginnings.
Beginning with Thomas Jefferson's calls for improved science education during his vice presidency (Shamos, 1995), dozens of national studies, reports, and recommendations have offered potential solutions to science literacy in the United States. In fact, about every 20 years since the late 1800s, an influential national report has called attention to the failures of the past and offered solutions for the future. The sophistication of these reports is surprising, at least to one who discovered them only recently. For example, recommendations made in the “Committee of Ten” report, authored more than 100 years ago, reflect advocacy for what we would call today “inquiry-based learning.” Twenty years later, another report called for making science education relevant to students' lives, promoting what we would call today “problem-based learning.” Calls for a relevant science education continued until the 1940s and World War II.
After World War II, the failures of our education system to meet the rising need for scientists generated “back to the basics” curriculum reform movements that began in the 1960s (Hoopes and Oakland University, 1963). These movements, which advocated standardized courses of study with prescribed content, resulted in large monetary investments in science education by the National Science Foundation (NSF) and others. In spite of this infusion of resources, the failures in science literacy continued to be described by a myriad of influential reports (Boyer, 1983; Mullis et al., 1988; National Science Board Commission on Precollege Education in Mathematics, Science, and Technology, 1982, 1983; Project 2061 [American Association for the Advancement of Science], 1989; U.S. National Commission on Excellence in Education, 1983, 1984a, b; Venezky et al., 1987). Once again, as at the turn of the twentieth century, educators at the turn of the twenty-first are called upon to develop relevant, inquiry-based approaches to teaching science (Bybee, 1997; Center for Science, Mathematics, and Engineering Education, 1998; Fox et al., 2003; Handelsman et al., 2004; Hazen and Trefil, 1991; Hurd, 1998; McCray et al., 2003; National Research Council, 1996; National Research Council Committee on Undergraduate Biology Education, 2003; National Research Council Committee on Undergraduate Science Education, 1997; Nelson, 1999; Project 2061 [American Association for the Advancement of Science], 1989, 1993; Tobias, 1992).
Even this brief overview illustrates the incredible amount of effort and resources we have invested over the past 200 years to define and deliver an excellent science education. However, this history also illustrates that none of these efforts has led to systemic, enduring changes in how we teach science or even what we expect students to learn. The problems we wrestle with today are basically the same as those faced at the founding of the United States, and before. Solutions have been proposed for decades, with little actual impact on our classroom practice. Such inertia might be a source of despair, if not for the remarkable progress we have made in science research. We have sent machines beyond the solar system and humans to the moon. We have developed medicines and therapies that can postpone death, sometimes for decades. We have deciphered the genetic heritage of hundreds of species and stand on the threshold of creating life de novo. Thus, achieving whatever it is we mean by “a scientifically literate population,” however worthy and important, does not appear to be essential for making progress in science. As a result, some scholars conclude that the goal of scientific literacy is a Quixotic venture at best, a myth at worst (Shamos, 1995).
As we consider nonmajors college biology classes today, what should we take away from the history of science literacy? We should be aware that, in our efforts to improve science education, we tread well-worn paths. If we treated this area of investigation as a worthy scholarly effort and simply read this literature, we might not be doomed to reinvent wheels, axles, or wagons, but might truly build on the scholarship of the past, as we naturally do in our research. We should be aware of the many attempts to define science literacy in terms of content lists (e.g., see the Office of Education circular, Martin, 1948). These lists have evolved (somewhat) as our understanding of life deepens, but they have not provided long-term solutions to science literacy. Finally, we should take heart that relying on scientists to bring their expertise and passion to nonscientists has great and long-lasting value. It is the passion of the teacher for her subject that changes students, who in turn change the world. Whatever modes and mechanisms we construct to support science literacy should not dilute the opportunity for individual biologists to share themselves with their students.
LITERACY IS NOT CONTENT KNOWLEDGE
Science is built up with facts, as a house is with stones. But a collection of facts is no more a science than a heap of stones is a house.
Jules Poincaré, mathematician, 1908
All knowledge has some value. Some knowledge has great value. However, no knowledge exists in any field, including biology, that is so vital or essential that every literate person must know it. In reply to this assertion, I can hear my esteemed colleagues argue, “But what about evolution?” or “But what about how DNA works?” or “But what about the richness of species diversity on the earth?” Each of us will have a “What about…” (or several) that captures our own biases and expertise as practicing biologists. The answer to every one is “No, that knowledge isn't essential.” All of it has value, but none is essential for all nonbiologists to know. Can a person live a worthwhile life, even a scholarly life, without knowing the mechanisms of evolution, or the function of DNA, or what a coelom is? Of course, they can. In fact, most people (even college professors) do. However, to be literate about biology, an educated person has to have the skills to ask and answer questions about biology in a scientifically valid way.
Thus, rather than mastery of content, literacy also requires the development of intellectual skills. Content is not irrelevant, since it provides the raw materials, Poincaré's “stones,” that students can manipulate to develop these skills. However, creation of synapses that store biology content is neither the goal nor the measure of biological literacy. Moving away from biology may help us gain perspective. Consider mathematics as an example of the importance of intellectual skill over mere content. Memorizing the multiplication tables is not essential for using math, although it would speed up the process of problem solving. However, knowing when and how to use multiplication to solve a problem is an essential intellectual skill of a mathematically literate person. Memorization of multiplication tables would become essential only if it were essential to using multiplication as a tool. It is not. Interestingly, memorizing the multiplication tables is likely to evolve naturally through continued opportunities to solve problems that require multiplication.
Perhaps math is a poor example for the argument of the goal of skill over content, since math is a discipline that is inherently and obviously devoted to the skill of problem solving. Consider, then, American literature. I have never read Catcher in the Rye and would venture to guess that many of the readers of this article would acknowledge the same deficiency. However, because I am literate, I could certainly get a copy and read it with understanding. This understanding would undoubtedly be richer if I had access to discussions with teacher-scholars about the meaning and context of this work, as in a college literature class. However, if I am sufficiently interested, I could also seek out the writings of scholars and even pursue the issue to the point that I could provide new insights into this work from my own experience. Perhaps, then, the theoretical potential to contribute new ideas or raise new questions is what literacy, in any field, ultimately means.
When considering how to help students become biology literate, we would be best served to think about ourselves in the context of a field distant from biology, in my case, literature. How did I come to have the ability to read literature with understanding and insight? Certainly, not through reading particular works that experts regard as seminal, but simply through reading, mostly books or articles that I chose based on my own interests and that I read outside the context of a class, frequently for pleasure. It was not by mastering particular American Literature content. It was by mastering the skill of reading, of knowing how and when to look up words I didn't know, of learning which sources provided information that was likely to be trustworthy and valuable. I could have gained this skill by reading (almost) anything.
The foundation of my argument is that literacy in any field implies that an individual has the potential for deeper learning in that field. Literacy isn't committing a particular set of facts to memory, but the ability to use resources to find, evaluate, and use information in a manner that reflects that field. If my premise is correct, the definition of biological literacy is straightforward: biologically literate individuals can ask and answer their own biologically relevant questions. Unfortunately, my definition is not the goal of most of our undergraduate biology classes, whether for biology majors or not.
Based on dozens of biology course syllabi from diverse institutions, it is clear that we emphasize content over skill in our classes, including those for nonmajors. A typical syllabus describes the course as a succession of topics (cell structure, metabolism, mitosis/meiosis, the central dogma, populations, natural selection, etc.) along with the appropriate text chapters to be read and perhaps lab exercises to conduct. In many ways, our syllabi give the impression that biology is a foreign language and that all students really need to know about biology are the definitions.
Our content-centric syllabi, and the courses they describe, contrast sharply with what we actually want our students to learn in our classes. I have asked dozens of my colleagues what they hope students who successfully complete their class will have learned. They don't list the content of the class. Instead, they want their students to make connections between the content and their lives, to be able to critically evaluate information in the future, to see the world in a new way, to be more interested in biology than before. The disconnect between spoken goals and written syllabus is staggering. Unfortunately, because there is so much content to cover, we don't have time to help our students learn to use the content we teach with any sophistication or understanding. We expect students will gain these intellectual skills on their own, simply as a result of learning the content listed in the syllabus. We expect these intellectual skills to emerge as students learn the vocabulary of biology. We are disappointed when they do not. Like Poincaré's stones, such unconstructed, disconnected knowledge is the antithesis of literacy.
A LIVED CURRICULUM IN BIOLOGY: FIRST, DO NO HARM
From the viewpoint of general education the principal criticism to be leveled at much of present college instruction in science is that it consists of courses in special fields, directed toward training the future specialist and making few concessions to the general student. Most of the time in such courses is devoted to developing a technical vocabulary and technical skills and to a systematic presentation of the accumulated fact and theory which science has inherited from the past.
James Conant, president of Harvard, 1945
The basic skill that defines biology literacy is arguably the same as for any other discipline: the ability to pose a question and then find, evaluate, and use information to answer it in a manner that is consistent with the mode of inquiry of that discipline. What kinds of college biology courses enable nonscientists to attain a lifelong skill in asking and answering biological questions? Even more challenging, because a single course is typically all of the biology that a nonscientist will take during college, how can this goal be accomplished in 10 to 15 weeks?
Above all other aims for a nonmajors biology course, the most important is to do no harm. If students leave their single required biology class convinced that the material is too dull, too difficult, or too irrelevant to merit their attention in the future, that class has failed to promote biology literacy, regardless of its definition. Biologically literate students will have experienced both the vibrancy and utility of biology. Rather than snuffing out their curiosity, a successful biology course for nonmajors will encourage students to recapture or develop a curiosity about the living world around them and their place in it. Literate students will be more likely to read an article in the paper about a newly discovered gene or take their kids to a science museum. They will be more likely to consult their biology textbook (or, more realistically, the Internet) to find out more about birth control pills or flu shots or trans fats. And they will be able to evaluate the information to distinguish reliable sources from the junk. In short, they will be more likely to ask questions whose answers are based in the science of biology.
Now, I hear my esteemed colleagues argue, “You haven't faced students like mine. They have already lost the spark of curiosity, if they ever had it. They have already decided that biology is uninteresting and irrelevant. They have already learned that it is too hard for them to master. They would not even be here unless they had to meet their Gen Ed requirements.” I've had those students, too. We should, at least, do no harm. Our classes should not validate their preconceptions. Our classes shouldn't make them even less likely to read a biologically relevant news article than they were when they entered our class. They should leave the class thinking, at the minimum, “Maybe this stuff isn't so bad, after all.”
It is important to understand that the exhortation to “do no harm” does not mean “give everyone an A.” Nonmajors courses should have high academic standards and be as rigorous as those offered to biology majors. To require less would not provide an authentic understanding of the practice of biology. “No harm” does not mean “no failures.” However, I have found faculty consistently underestimate what students are willing and capable of doing when motivated. Nonmajors, even those on athletic scholarships, can learn to read primary research literature; to devise and evaluate experiments; to write high-quality essays in a scientific style; and to understand the most intricate, detailed aspect of any biological system they care to know. It just requires more modeling of those skills by the professor and more time for students to practice these skills and receive feedback on their performance. And it requires the professor to give up the (mistaken) idea that she must cover a critical list of content.
A LIVED CURRICULUM IN BIOLOGY: CONSTRUCT A CONTEXT FOR CONTENT
We must teach our science for the sake of the student and not for the sake of the subject.
Thomas Smyth, 1940
Does it matter what specific biology content we teach an individual who will not become a practicing biologist? Clearly, my answer to this question is, “No.” In contrast, as future practitioners of biology, biology majors need to master a body of content knowledge, as well as to hone a variety of technical and intellectual skills that will prepare them for their future careers. Thus, the learning outcomes of biology classes for biology majors are vastly different from the learning outcomes for nonmajors. As we consider exemplary practices in nonmajors biology, we should recognize that those universities in which teaching of biology majors is separate from nonscience majors have made an important step in the right direction. Fortunately, this separation appears to be more the rule rather than the exception in U.S. colleges and universities at present.
Given this freedom from content coverage in nonmajors biology, we should not encumber ourselves or our students with a dumbed-down version of a biology survey course. In fact, a traditional survey course is least likely to meet the primary learning goal of helping nonscientists learn to pose and answer biological questions and most likely to “do harm.” Instead, the best nonmajors courses are constructed around compelling problems about which students are inherently interested and with which the individual professor is passionately engaged. Within this context, we demonstrate how biologists analyze a particular problem, what methods are used to provide relevant data, how to critically evaluate these data, how to deal with complexity and ambiguity, and how to distinguish science from nonscience. Within this context, we exploit the principles of human learning to help students move toward being independent learners of biology (Table 1). Within this context, we help students learn a lot of biology content. (Think how much one would need to know to think critically about synthetic life, or genetically modified foods, or human evolution, or emerging diseases!) Within this context, we model within the course structure itself how biologists ask and answer questions.
Table 1.
The implications of the seven principles of learning for nonmajors biology courses
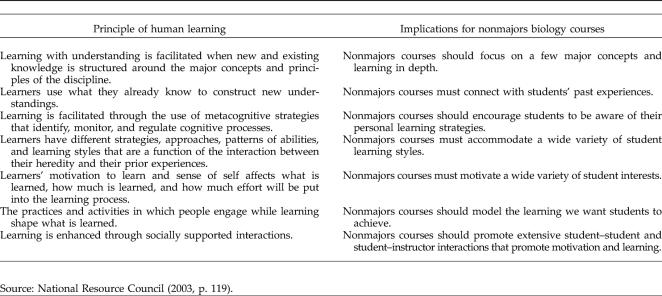
What universities currently offer nonmajors biology courses that focus on developing problem-solving skills? To my knowledge, the best example comes from the University of Oregon's Workshop Biology Project (http://yucca.uoregon.edu/wb/), which aims to help students “make informed, critical decisions about important biological issues.” Workshop Biology classes meet each week in two 90-minute “assemblies,” which replace traditional lectures, and one 80-minute lab. In both venues, students participate in concept activities, investigative activities, and issues activities. Detailed descriptions of this exemplary program are available at http://yucca.uoregon.edu/wb/Materials/WB_Handbook.pdf, although the courses taught using the Workshop Biology paradigm are apparently no longer offered to nonmajors at the University of Oregon. Another example, still in its infancy, is the multi-institutional effort of the Associated Colleges of the South Reform of Introductory Science Courses for Non-science Majors. However, this effort largely supports individual course changes rather than promoting organized, systemic change.
In contrast to these limited examples of systemic approaches, many examples exist of individual nonmajors biology classes that take a contextual approach (see Table 2 for several). The richness of individual course examples and paucity of institutionalized efforts is consistent with the idea of empowering individual faculty to develop courses for nonmajors that reflect key issues in their research disciplines. Consequently, the absence of obvious systematic efforts to develop contextualized biology courses for nonmajors may be a positive rather than negative sign. However, it is distressing that the creativity and scholarship behind these individual courses are usually not publicly available. As a result, instructors are doomed to re-create courses de novo, rather than build on previous classroom scholarship. To help communicate this cryptic scholarship, I encourage those of you who are engaged in nonmajors biology to share your experiences via the discussion forum associated with this article.
Table 2.
Examples of nonmajors biology systemic efforts and individual courses
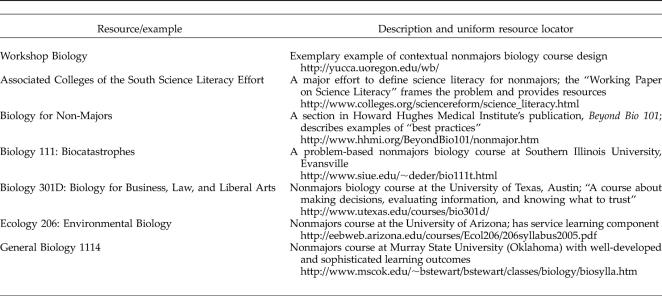
Another excellent model of a problem-based approach that bears highlighting is in chemistry, rather than biology. Chem Connections (http://mc2.cchem.berkeley.edu/; http://chemlinks.beloit.edu/), an NSF-funded effort supporting systematic change in chemistry education, provides a modular, contextual approach to the first year of college chemistry for majors and nonmajors. The courses have specific content mastery goals, but content is embedded in the context of interesting problems, such as “What should we do about global warming?” and “How could life have arisen on earth?” The goals of the project include helping students develop increased interest in chemistry, greater conceptual understanding, and improved problem-solving skills. Assessment of this modular, problem-oriented approach shows that students' content mastery is as good or better in the modular class as in traditional courses, at both University of California—Berkeley and Grinnell College. Interestingly, students at Grinnell College appear to prefer the modular course, whereas students at Berkeley appear to prefer the traditional course (Gutwill-Wise, 2001).
A LIVED CURRICULUM: DEVELOP NEW ASSESSMENT STRATEGIES
We measure the success of schools not by the kinds of human beings they promote but by whatever increases in reading scores they chalk up. We have allowed quantitative standards, so central to the adult economic system, to become the principal yardstick for our definition of our children's worth.
Kenneth Keniston, Massachusetts Institute of Technology, professor of Human Development, 1976
Even if we can build an ideal list of content, as Michael Klymkowsky argues in his “Point of View” essay, even if we knew which parts of this list students learned well and which parts they did not, teaching this list is the wrong approach if our aim is authentic biology literacy. Instead, biology literacy requires that our students learn to think and communicate in biology, not simply recite a list of facts back to us. Teaching for mastery of even an ideal content list risks converting science literacy into a checklist for standardized testing. I have argued, instead, that biology literacy is the ability to ask a biologically relevant question and answer it in a way that reflects the science of biology. If this premise is valid, we face a serious challenge. How do we measure the ability of our students to pose biologically relevant questions and to find, evaluate, and use the information needed to answer them? What is a good question, and how can we assign it a grade? What are the standards by which we judge a students' facility to find, evaluate, and use information? How can we distinguish “A”-quality work from “C”-quality work?
This insidious assessment challenge may be the root of our centuries-long failure to effect systemic changes in science education. Our persistent focus on content transfer in our classes may reflect the ease with which we can measure content knowledge in large classes, with relatively little effort. We are facile at the use of selected-response (multiple choice, matching, true/false) or short-answer questions to determine whether or not a student remembers the definition of mitosis, or the DNA basepairing rules, or the nitrogen cycle. For classes with large enrollment, selected/short-answer exams are frequently the major (or only) assessment tools we use to assign grades. Assigning grades based on these assessments is similarly straightforward: simply map the average score to a curve or standard and mark the appropriate box on the grade reporting sheet.
Measuring how well students pose and solve biological problems is much more difficult and time consuming. It requires substantive individual evaluation of student work presented as written reports, oral presentations, posters, Web sites, portfolios, and the like. Even if we were willing and able to devote the necessary time to evaluating individual student work, we would be challenged to devise assignments and corresponding assessment strategies that fairly and reproducibly measure the student's ability to ask and answer questions. Consider reproducibility as one example of the challenge. Authentic assessment rubrics produce the same grade regardless of who scores the assignment. Where will we find the time or develop the expertise needed to devise and test our rubrics, as well as calibrate the scoring to ensure reproducibility among faculty teaching different courses?
A variety of resources concerning assessment are available, many of which can only be described as intimidating (Table 3). Thus, one of the most important things biology educators might do to promote biology literacy would be to develop assessment strategies that enable us to measure the skills that underlie the ability to ask and answer biological questions, including information literacy, critical thinking, data analysis, effective communication, and logic. Again, I invite the readers to share their experience in the discussion forum for this article.
Table 3.
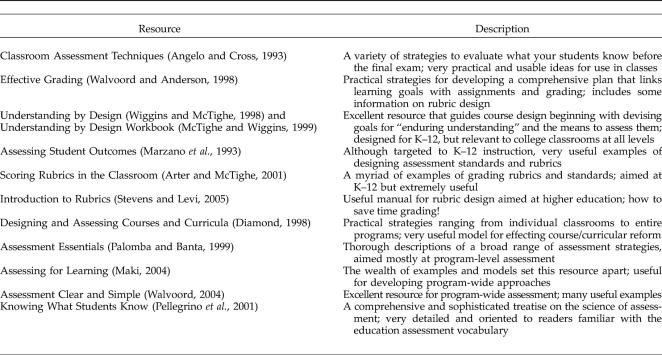
A LIVED CURRICULUM: SHARE THE GRANDEUR
There is grandeur in this view of life, with its several powers, having been breathed into a few forms or into one; and that, whilst this planet has gone cycling on according to the fixed law of gravity, from so simple a beginning endless forms most beautiful and most wonderful have been, and are being, evolved.
Charles Darwin, naturalist, 1859
The biology I want to teach my students changes their lives. It is the biology of my grade school class where I first saw tiny paramecia in a drop of water and realized that a single cell behaved in complex ways. At that moment, I felt there was some hope that I might eventually understand a single cell. It is the biology of my college invertebrate zoology class, where I learned to really look at things. I felt that everything I saw afterwards had a new clarity and brilliance and meaning. It is the biology of the late-night observation, when the experiment failed to support the hypothesis but revealed a new truth and I was the first to discover it. I felt both humility and exaltation in that moment of knowing, as new ideas and understandings took shape.
The biology we want our students to learn is the same biology that captured us, that distilled our interests into our careers as biologists, and that continues to inspire us today. As we wrestle with the details and argue about definitions, let us not lose sight of the importance of sharing the grandeur of biology with our students, as it was shared with us.
REFERENCES
-
Angelo T.A, Cross K.P. Classroom Assessment Techniques: A Handbook for College Teachers. San Francisco: Jossey-Bass Publishers; (1993).. [Google Scholar]
-
Arter J.A, McTighe J. Scoring Rubrics in the Classroom: Using Performance Criteria for Assessing and Improving Student Performance. Thousand Oaks, CA: Corwin Press; (2001).. [Google Scholar]
-
Boyer E.L. High School: A Report on Secondary Education in America. New York: Harper & Row; (1983).. [Google Scholar]
-
Bybee R.W. Achieving Scientific Literacy: From Purposes to Practices. Portsmouth, NH: Heinemann; (1997).. [Google Scholar]
-
Center for Science Mathematics and Engineering Education. Every Child a Scientist: Achieving Scientific Literacy for All. Washington, DC: National Academy Press; (1998).. [Google Scholar]
-
DeBoer G.E. A History of Ideas in Science Education: Implications for Practice. New York: Teachers College Press; (1991).. [Google Scholar]
-
DeBoer G.E. Scientific literacy: another look at its historical and contemporary meanings and its relationship to science education reform. J. Res. Sci. Teach. (2000).;37:582–601. [Google Scholar]
-
Diamond R.M. Designing and Assessing Courses and Curricula: A Practical Guide. San Francisco: Jossey-Bass Publishers; (1998).. [Google Scholar]
-
Fox M.A, Hackerman N. Evaluating and improving undergraduate teaching in science, technology, engineering, and mathematics. Washington, DC: National Academy Press; (2003).. eds. [Google Scholar]
-
Gutwill-Wise J.P. The impact of active and context-based learning in introductory chemistry courses: an early evaluation of the modular approach. J. Chem. Educ. (2001).;78:684–690. [Google Scholar]
-
Handelsman J, Ebert-May D, Beichner R, Bruns P, Chang A, DeHaan R, Gentile J, Lauffer S, Stewart J, Tilghman S.M, Wood W.B. Education: scientific teaching. Science. (2004).;304:521–522. doi: 10.1126/science.1096022. [DOI] [PubMed] [Google Scholar]
-
Hazen R.M, Trefil J.S. Science Matters: Achieving Scientific Literacy. New York: Doubleday; (1991).. [Google Scholar]
-
Hoopes R. Science in the College Curriculum: A Report of a Conference. Rochester, MI: Oakland University; (1963).. [Google Scholar]
-
Hurd P.D. Scientific literacy: new minds for a changing world. Sci. Educ. (1998).;82:407–416. [Google Scholar]
-
Maki P. Assessing for Learning: Building a Sustainable Commitment across the Institution. Sterling, VA: Stylus; (2004).. [Google Scholar]
- Martin W.E. The Major Principles of the Biological Sciences of Importance for General Education. Washington, DC: U.S. Office of Education, Circular No. (1948).. 308.
-
Marzano R.J, Pickering D, McTighe J. Assessing Student Outcomes: Performance Assessment Using the Dimensions of Learning Model. Alexandria, VA: Association for Supervision and Curriculum Development; (1993).. [Google Scholar]
-
Mather C. The History of Harvard College. Boston: Directors of the Old South Work; (1907).. [Google Scholar]
-
McCray R, DeHaan R.L, Schuck J.A. Improving Undergraduate Instruction in Science, Technology, Engineering, and Mathematics: Report of a Workshop. Washington, DC: National Academies Press; (2003).. eds. [Google Scholar]
-
McTighe J, Wiggins G.P. Understanding by Design Handbook. Alexandria, VA: Association for Supervision and Curriculum Development; (1999).. [Google Scholar]
-
Mullis I.V.S, Jenkins L. The Science Report Card: Elements of Risk and Recovery: Trends and Achievement Based on the 1986 National Assessment. Princeton, NJ: Educational Testing Service; (1988).. [Google Scholar]
-
National Research Council. National Science Education Standards: Observe, Interact, Change, Learn. Washington, DC: National Academy Press; (1996).. [Google Scholar]
- National Research Council. Learning and Understanding: Improving Advanced Study of Mathematics and Science in U.S. High Schools. (2003).. http://books.nap.edu/books/0309074401/html/index.html (accessed 2 May 2005).
-
National Research Council Committee on Undergraduate Biology Education. Bio 2010: Transforming Undergraduate Education for Future Research Biologists. Washington, DC: National Academies Press; (2003).. [Google Scholar]
-
National Research Council Committee on Undergraduate Science Education. Science Teaching Reconsidered: A Handbook. Washington, DC: National Academy Press; (1997).. [Google Scholar]
-
National Science Board Commission on Precollege Education in Mathematics, Science, and Technology. Today's Problems, Tomorrow's Crises. Washington, DC: National Science Foundation; (1982).. [Google Scholar]
-
National Science Board Commission on Precollege Education in Mathematics, Science, and Technology. Educating Americans for the 21st Century: A Plan of Action for Improving Mathematics, Science, and Technology Education for All American Elementary and Secondary Students So That Their Achievement Is the Best in the World by 1995: A Report to the American People and the National Science Board. Washington, DC: (1983).. [Google Scholar]
-
Nelson G.D. Science literacy for all in the 21st century. Educ. Leadership. (1999).;57:14–17. [Google Scholar]
- O'Sullivan C.Y. The nation's report card: Science 2000. Washington, DC: U.S. Dept. (2003).. of Education Institute of Education Science.
-
Palomba C.A, Banta T.W. Assessment Essentials: Planning, Implementing, and Improving Assessment in Higher Education. San Francisco: Jossey-Bass Publishers; (1999).. [Google Scholar]
-
Pellegrino J.W, Chudowsky N, Glaser R. Knowing What Students Know: The Science and Design of Educational Assessment. Washington, DC: National Academy Press; (2001).. [Google Scholar]
-
Project 2061. Science for All Americans: A Project 2061 Report on Literacy Goals in Science, Mathematics, and Technology. Washington, DC: American Association for the Advancement of Science; (1989).. [Google Scholar]
-
Project 2061. Benchmarks for Science Literacy. New York: Oxford University Press; (1993).. [Google Scholar]
-
Rudolph F. Curriculum: A History of the American Undergraduate Course of Study Since 1636. San Francisco: Jossey-Bass Publishers; (1977).. [Google Scholar]
-
Shamos M.H. The Myth of Scientific Literacy. New Brunswick, NJ: Rutgers University Press; (1995).. [Google Scholar]
-
Stevens D.D, Levi A. Introduction to Rubrics: An Assessment Tool To Save Grading Time, Convey Effective Feedback, and Promote Student Learning. Sterling, VA: Stylus Publishing; (2005).. [Google Scholar]
-
Tobias S. Revitalizing Undergraduate Science: Why Some Things Work and Most Don't. Tucson, AZ: Research Corp; (1992).. [Google Scholar]
-
U.S. National Commission on Excellence in Education. A Nation at Risk: The Imperative for Educational Reform: A Report to the Nation and the Secretary of Education, United States Department of Education. Washington, DC: (1983).. [Google Scholar]
-
U.S. National Commission on Excellence in Education. Meeting the Challenge of A Nation at Risk. Cambridge, MA: USA Research; (1984a).. [Google Scholar]
-
U.S. National Commission on Excellence in Education. A Nation at Risk: The Full Account. Cambridge, MA: USA Research; (1984b).. [Google Scholar]
-
Venezky R.L, Kaestle C.F, Sum A.M. The Subtle Danger: Reflections on the Literacy Abilities of America's Young Adults. Princeton, NJ: Center for the Assessment of Educational Progress, Educational Testing Service; (1987).. [Google Scholar]
-
Walvoord B.E.F. Assessment Clear and Simple: A Practical Guide for Institutions, Departments, and General Education. San Francisco: Jossey-Bass Publishers; (2004).. [Google Scholar]
-
Walvoord B.E.F, Anderson V.J. Effective Grading: A Tool for Learning and Assessment. San Francisco: Jossey-Bass Publishers; (1998).. [Google Scholar]
-
Wiggins G.P, McTighe J. Understanding by Design. Alexandria, VA: Association for Supervision and Curriculum Development; (1998).. [Google Scholar]