Abstract
1. Glutamate oxidation in brain and liver mitochondrial systems proceeds mainly through transamination with oxaloacetate followed by oxidation of the α-oxoglutarate formed. Both in the presence and absence of dinitrophenol in liver mitochondria this pathway accounted for almost 80% of the uptake of glutamate. In brain preparations the transamination pathway accounted for about 90% of the glutamate uptake. 2. The oxidation of [1-14C]- and [5-14C]-glutamate in brain preparations is compatible with utilization through the tricarboxylic acid cycle, either after the formation of α-oxoglutarate or after decarboxylation to form γ-aminobutyrate. There is no indication of γ-decarboxylation of glutamate. 3. The high respiratory control ratio obtained with glutamate as substrate in brain mitochondrial preparations is due to the low respiration rate in the absence of ADP: this results from the low rate of formation of oxaloacetate under these conditions. When oxaloacetate is made available by the addition of malate or of NAD+, the respiration rate is increased to the level obtained with other substrates. 4. When the transamination pathway of glutamate oxidation was blocked with malonate, the uptake of glutamate was inhibited in the presence of ADP or ADP plus dinitrophenol by about 70 and 80% respectively in brain mitochondrial systems, whereas the inhibition was only about 50% in dinitrophenol-stimulated liver preparations. In unstimulated liver mitochondria in the presence of malonate there was a sixfold increase in the oxidation of glutamate by the glutamate-dehydrogenase pathway. Thus the operating activity of glutamate dehydrogenase is much less than the `free' (non-latent) activity. 5. The following explanation is put forward for the control of glutamate metabolism in liver and brain mitochondrial preparations. The oxidation of glutamate by either pathway yields α-oxoglutarate, which is further metabolized. Since aspartate aminotransferase is present in great excess compared with the respiration rate, the oxaloacetate formed is continuously removed by the transamination reaction. Thus α-oxoglutarate is formed independently of glutamate dehydrogenation, and the question is how the dehydrogenation of glutamate is influenced by the continuous formation of α-oxoglutarate. The results indicate that a competition takes place between the α-oxoglutarate-dehydrogenase complex and glutamate dehydrogenase, probably for NAD+, resulting in preferential oxidation of α-oxoglutarate.
Full text
PDF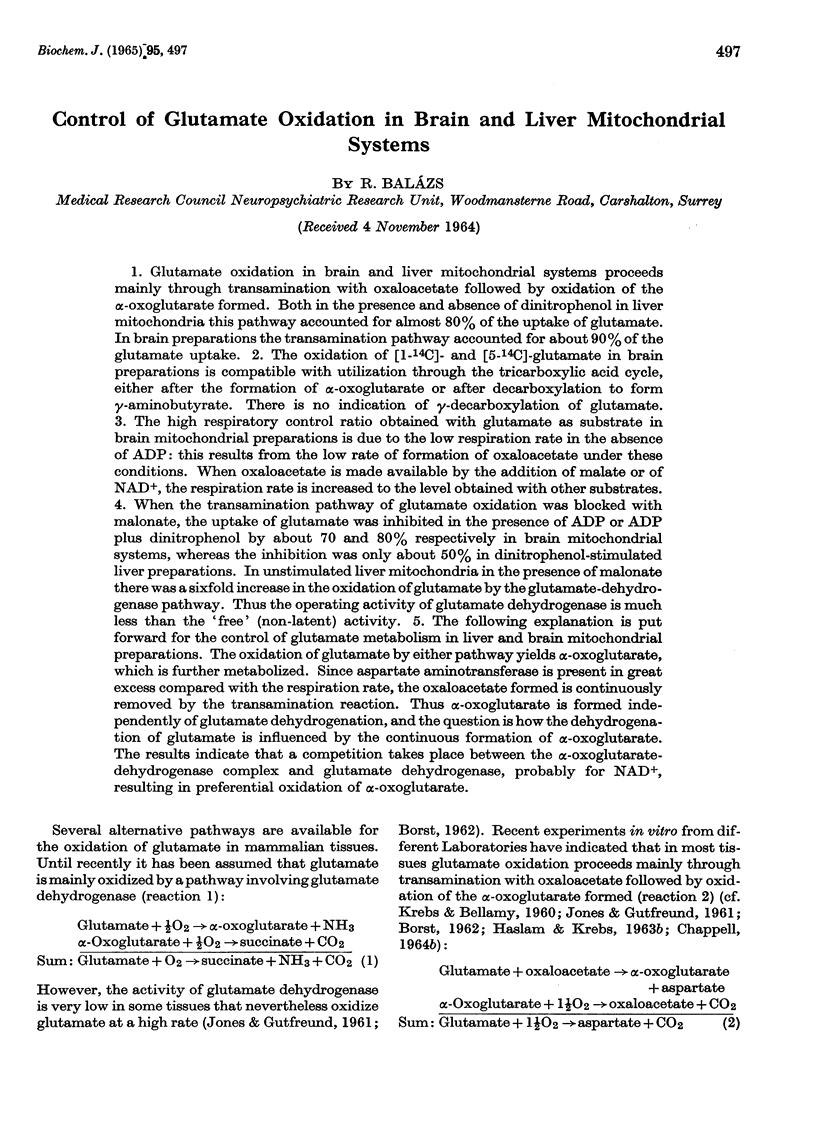
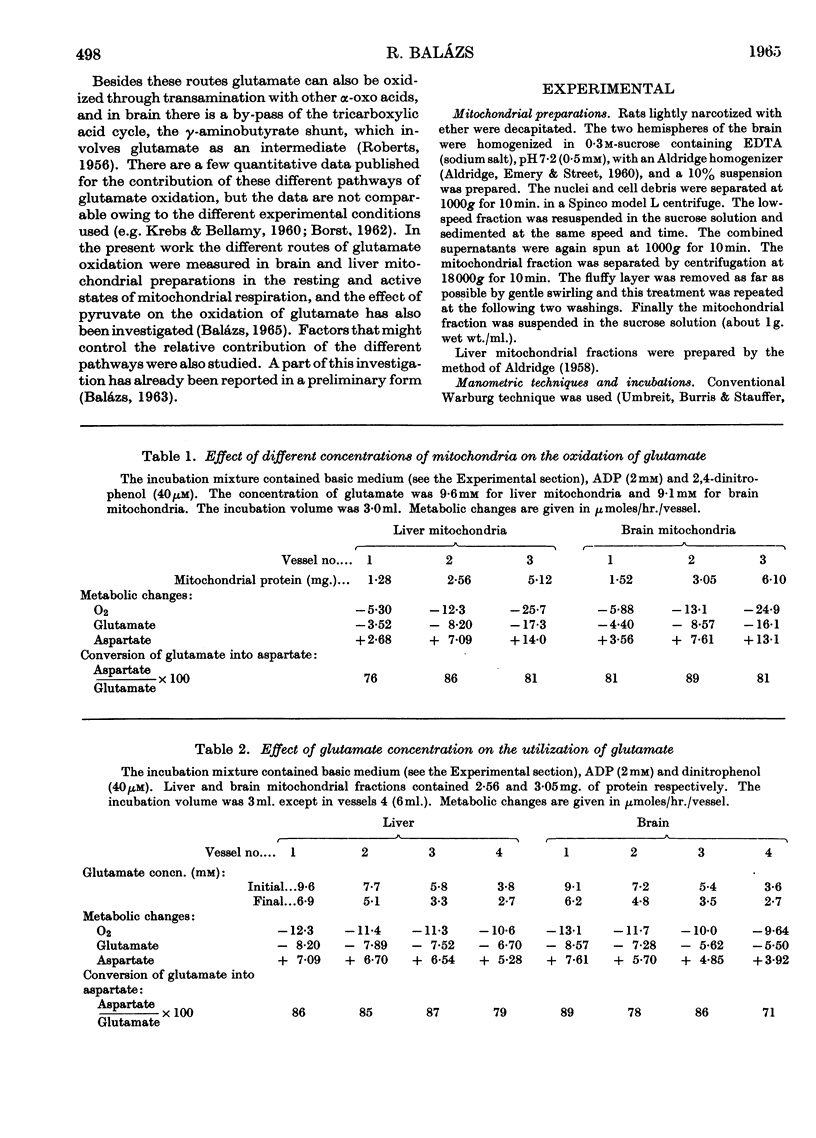
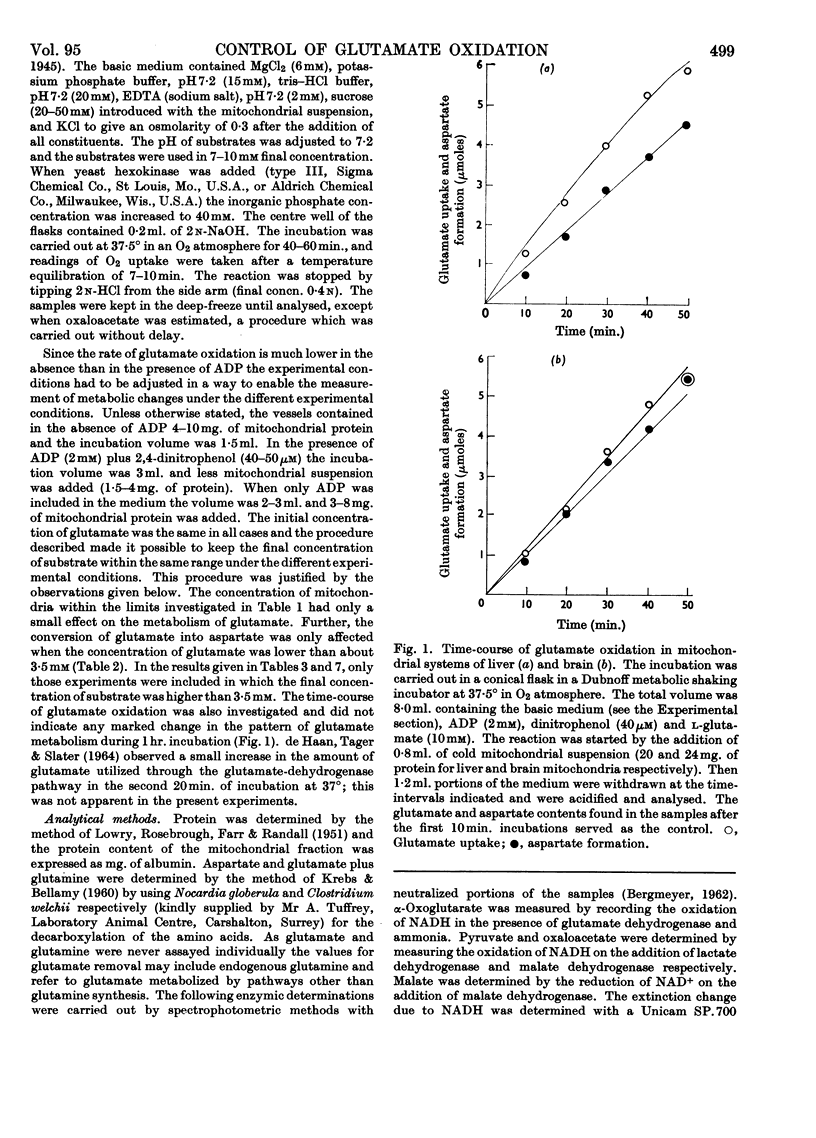
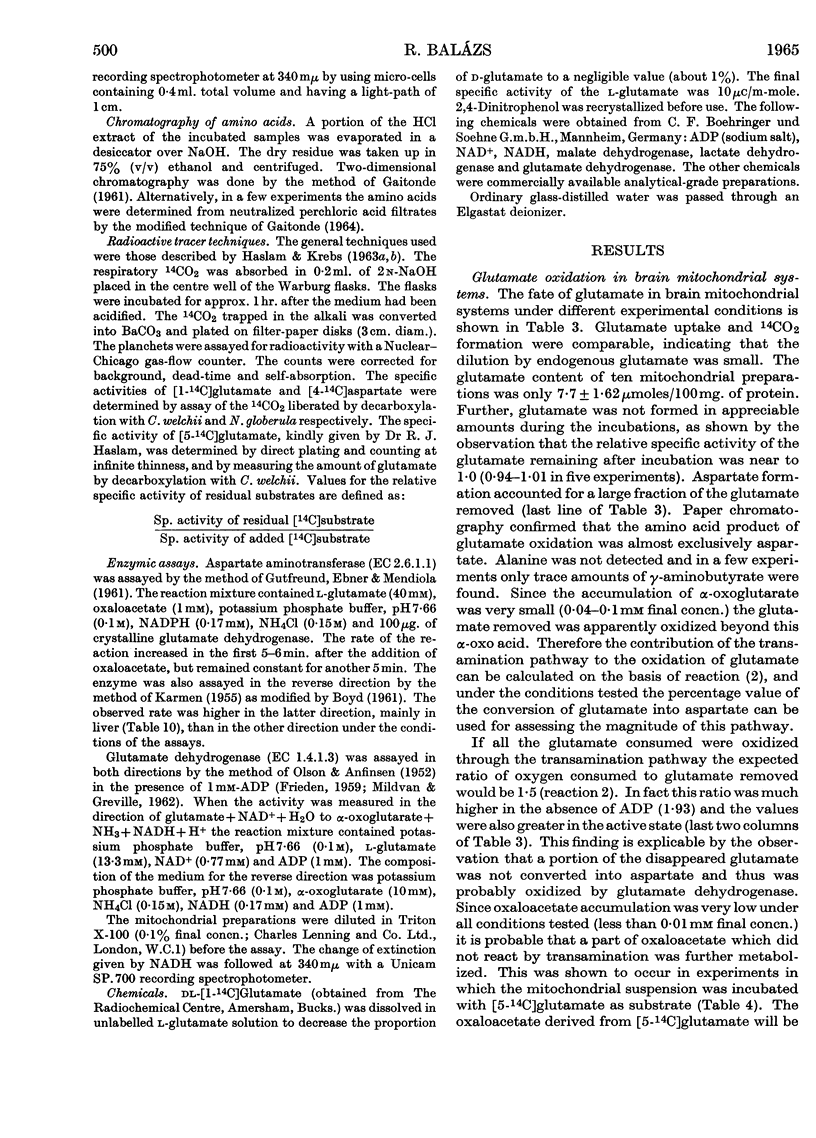
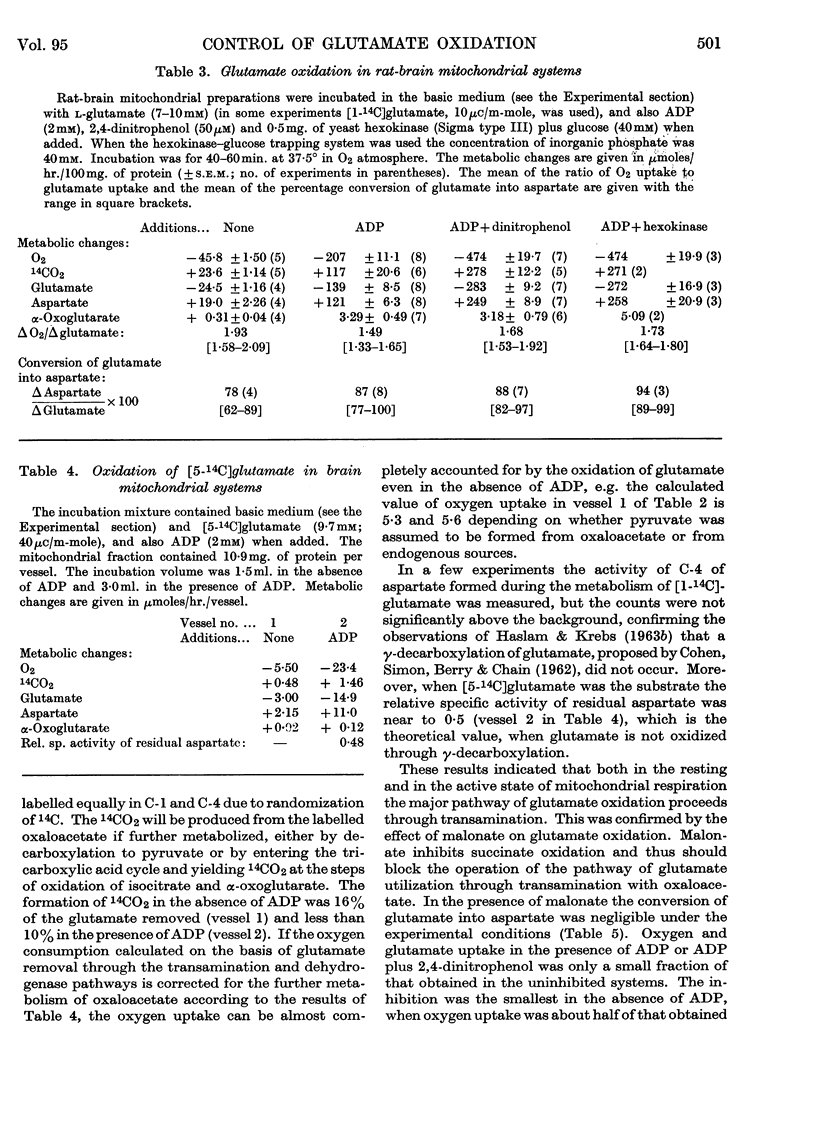
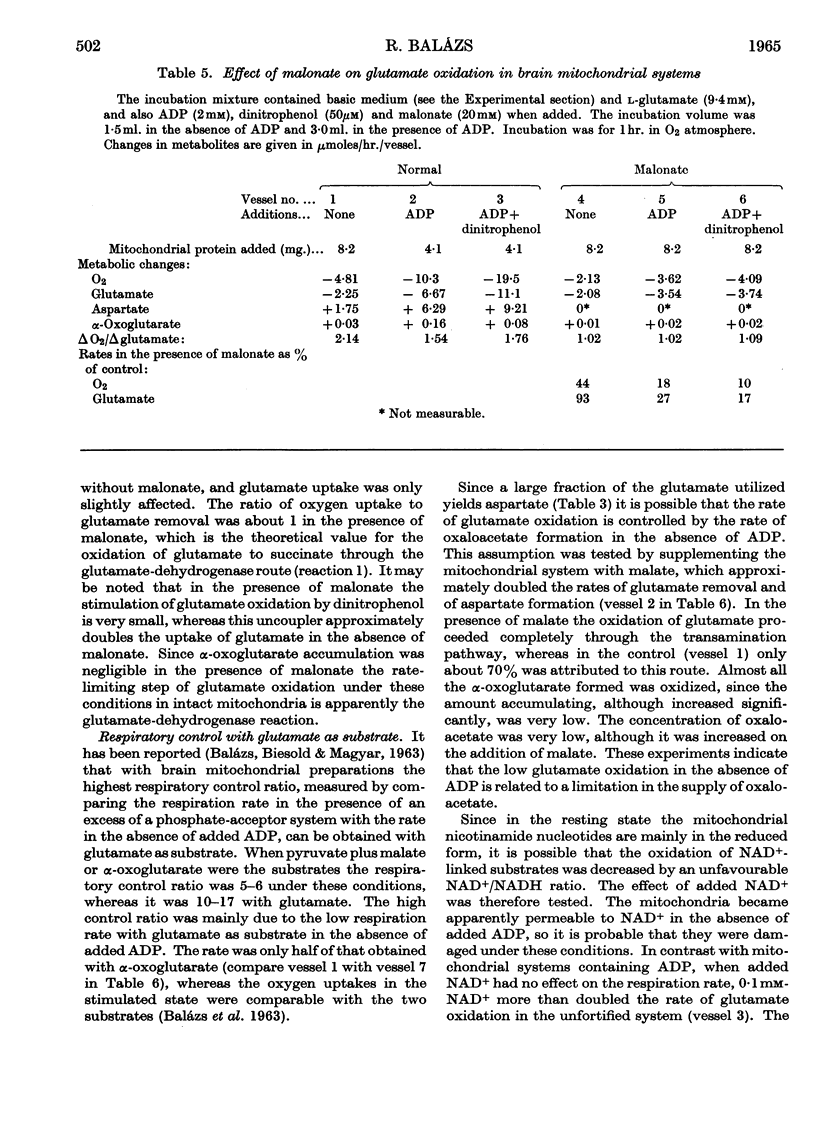
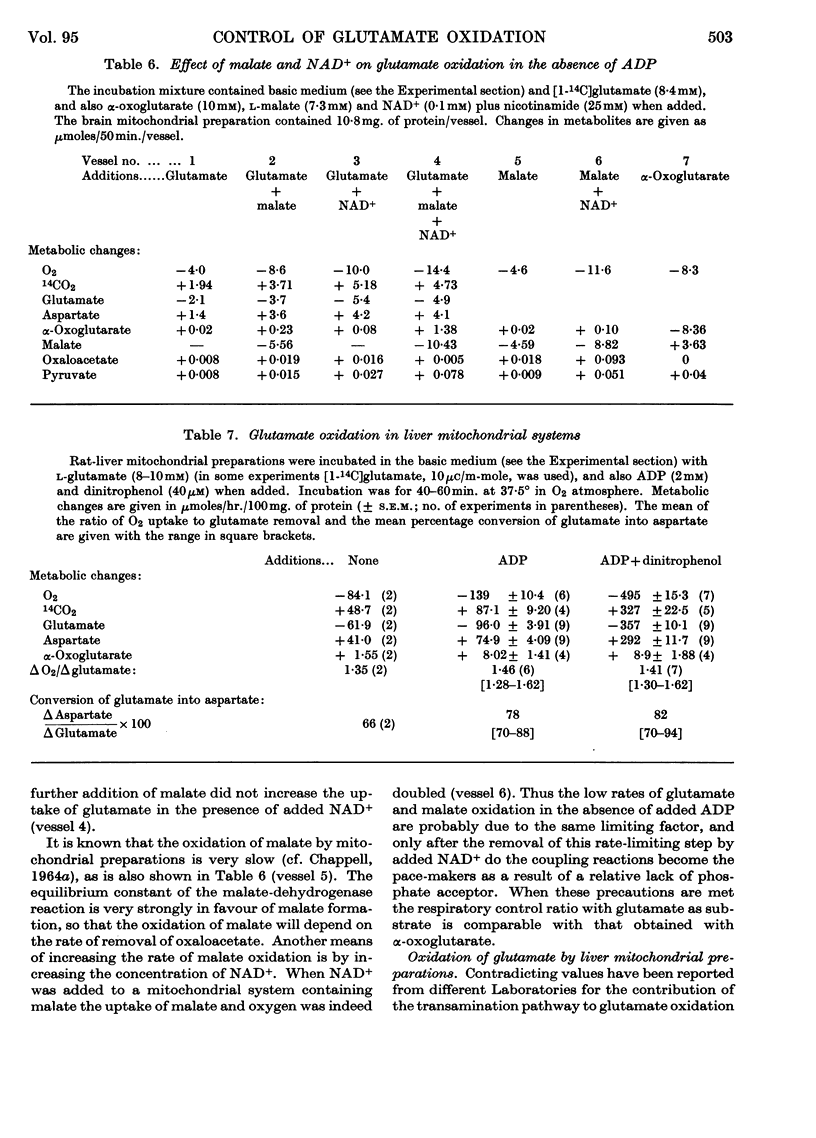
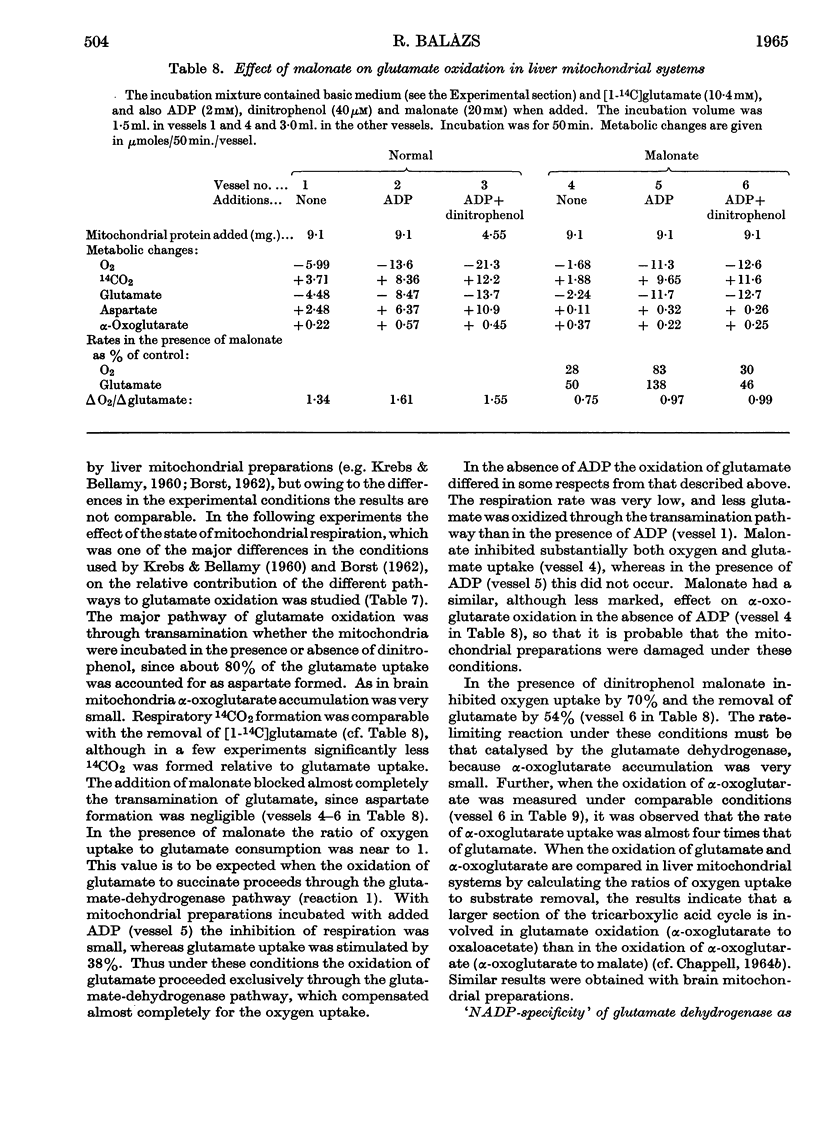
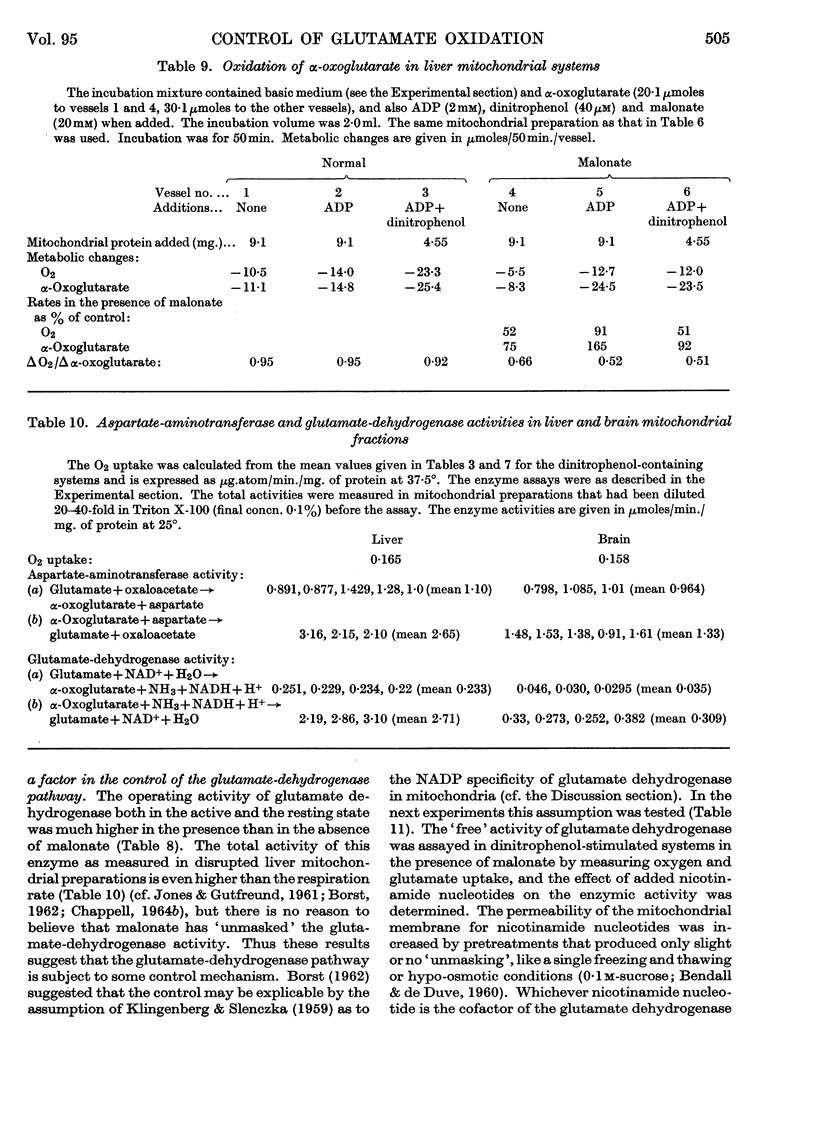
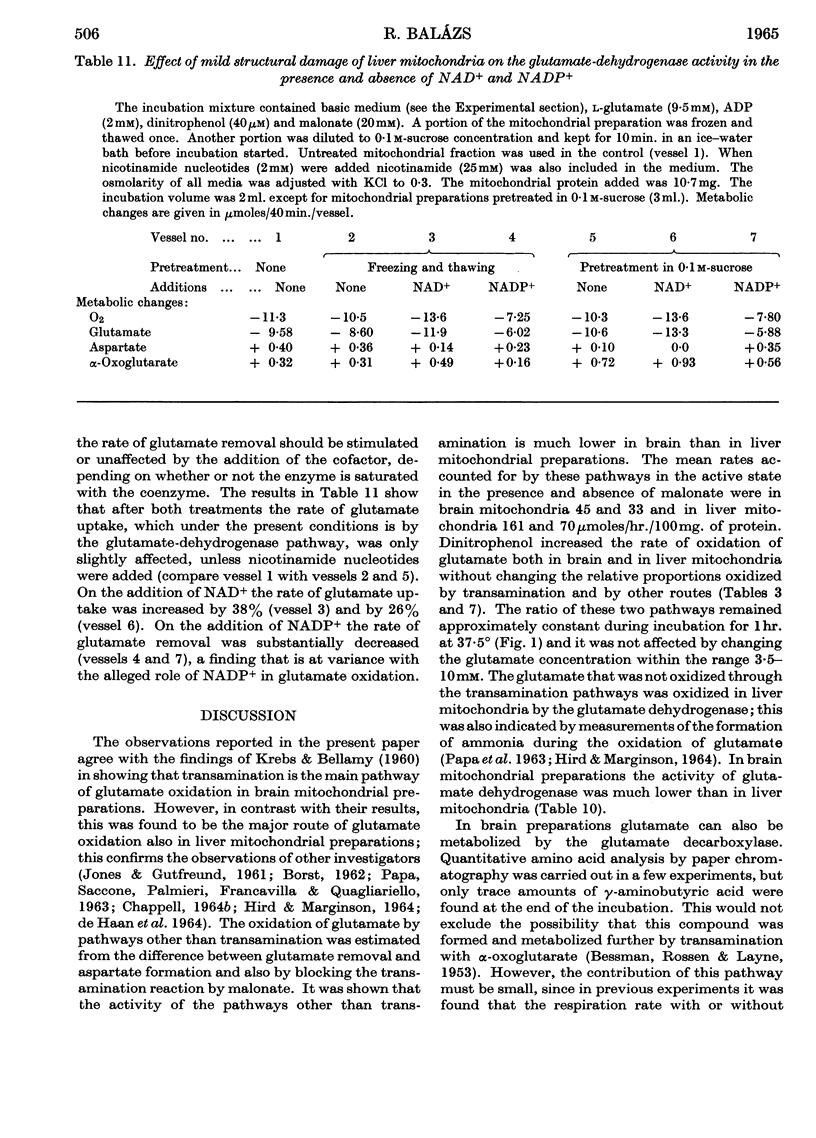
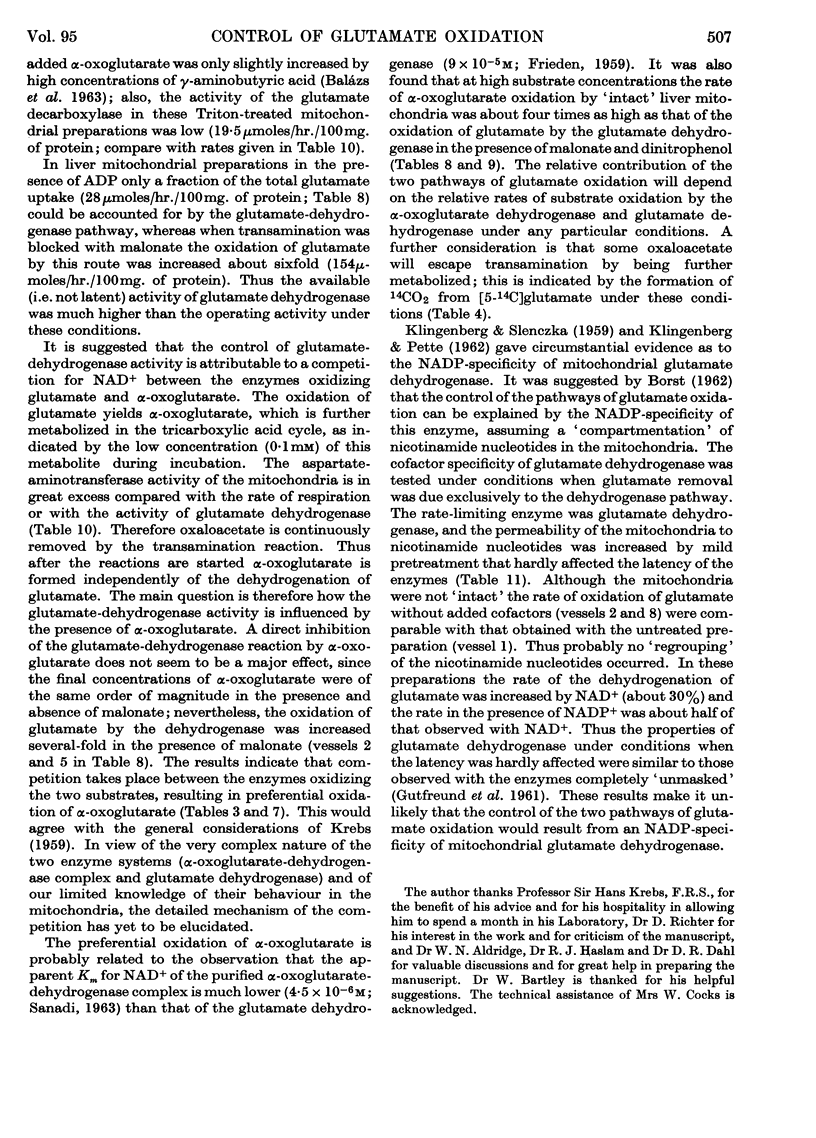
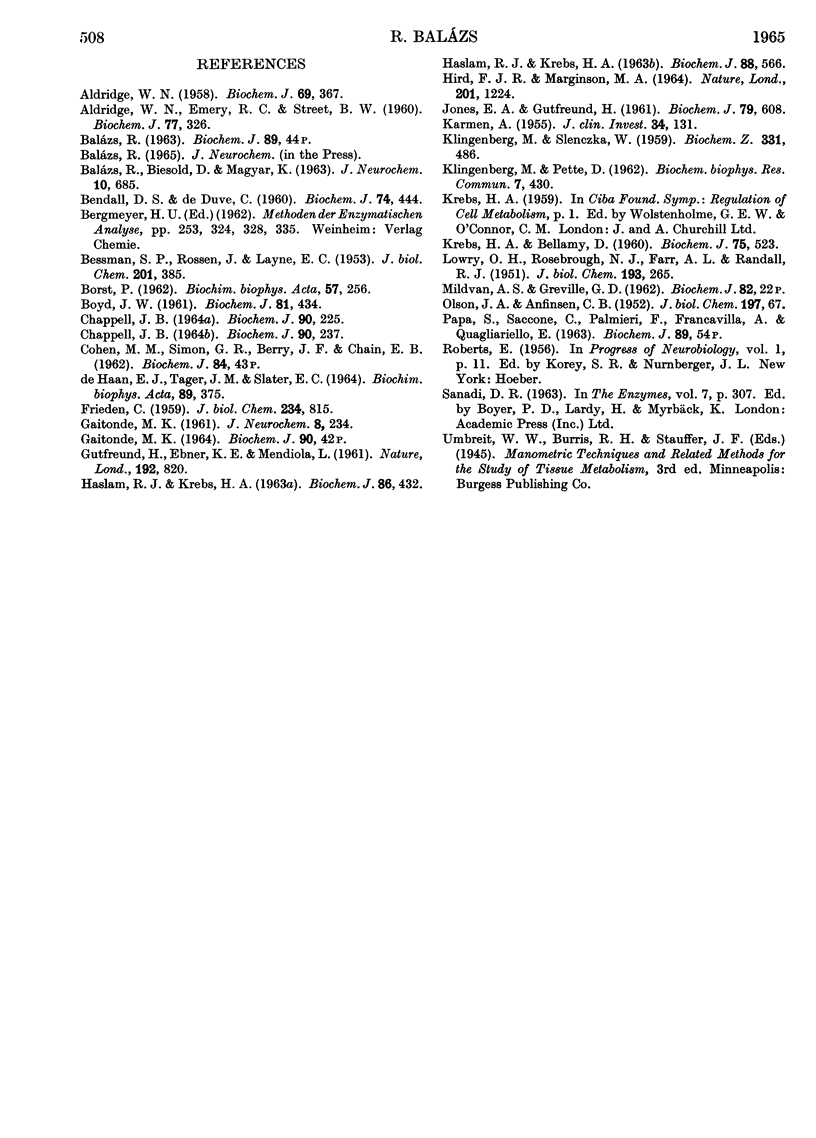
Selected References
These references are in PubMed. This may not be the complete list of references from this article.
- ALDRIDGE W. N., EMERY R. C., STREET B. W. A tissue homogenizer. Biochem J. 1960 Nov;77:326–327. doi: 10.1042/bj0770326. [DOI] [PMC free article] [PubMed] [Google Scholar]
- ALDRIDGE W. N. The biochemistry of organotin compounds: trialkyltins and oxidative phosphorylation. Biochem J. 1958 Jul;69(3):367–376. doi: 10.1042/bj0690367. [DOI] [PMC free article] [PubMed] [Google Scholar]
- BALAZS R., BIESOLD D., MAGYAR K. SOME PROPERTIES OF RAT BRAIN MITOCHONDRIAL PREPARATIONS: RESPIRATORY CONTROL. J Neurochem. 1963 Oct;10:685–708. doi: 10.1111/j.1471-4159.1963.tb08926.x. [DOI] [PubMed] [Google Scholar]
- BENDALL D. S., DE DUVE C. Tissue-fractionation studies. 14. The activation of latent dehydrogenases in mitochondria from rat liver. Biochem J. 1960 Mar;74:444–450. doi: 10.1042/bj0740444. [DOI] [PMC free article] [PubMed] [Google Scholar]
- BESSMAN S. P., ROSSEN J., LAYNE E. C. Gamma-Aminobutyric acid-glutamic acid transamination in brain. J Biol Chem. 1953 Mar;201(1):385–391. [PubMed] [Google Scholar]
- BORRELL S. URINARY STEROIDS OF CATS. 2. CORTICOSTEROID-LIKE SUBSTANCES. Biochem J. 1963 Oct;89:54–57. doi: 10.1042/bj0890054. [DOI] [PMC free article] [PubMed] [Google Scholar]
- BORST P. The pathway of glutamate oxidation by mitochondria isolated from different tissues. Biochim Biophys Acta. 1962 Feb 26;57:256–269. doi: 10.1016/0006-3002(62)91119-8. [DOI] [PubMed] [Google Scholar]
- BOYD J. W. The intracellular distribution, latency and electrophoretic mobility of L-glutamate-oxaloacetate transaminase from rat liver. Biochem J. 1961 Nov;81:434–441. doi: 10.1042/bj0810434. [DOI] [PMC free article] [PubMed] [Google Scholar]
- Chappell J. B. The effects of 2,4-dinitrophenol on mitochondrial oxidations. Biochem J. 1964 Feb;90(2):237–248. doi: 10.1042/bj0900237. [DOI] [PMC free article] [PubMed] [Google Scholar]
- Chappell J. B. The oxidation of citrate, isocitrate and cis-aconitate by isolated mitochondria. Biochem J. 1964 Feb;90(2):225–237. doi: 10.1042/bj0900225. [DOI] [PMC free article] [PubMed] [Google Scholar]
- DE HAAN E. J., TAGER J. M., SLATER E. C. PATHWAY OF GLUTAMATE OXIDATION IN RAT-LIVER MITOCHONDRIA. Biochim Biophys Acta. 1964 Aug 26;89:375–377. doi: 10.1016/0926-6569(64)90234-2. [DOI] [PubMed] [Google Scholar]
- FRIEDEN C. Glutamic dehydrogenase. II. The effect of various nucleotides on the association-dissociation and kinetic properties. J Biol Chem. 1959 Apr;234(4):815–820. [PubMed] [Google Scholar]
- GAITONDE M. K. The composition of brain lipoprotein fractions obtained by different procedures. J Neurochem. 1961 Dec;8:234–242. doi: 10.1111/j.1471-4159.1961.tb13548.x. [DOI] [PubMed] [Google Scholar]
- GUTFREUND H., EBNER K. E., MENDIOLA L. Transamination and the control of mitochondrial pathways. Nature. 1961 Dec 2;192:820–823. doi: 10.1038/192820a0. [DOI] [PubMed] [Google Scholar]
- HASLAM R. J., KREBS H. A. Substrate competition in the respiration of animal tissues. The metabolic interactions of pyruvate and alpha-oxoglutarate in rat-liver homogenates. Biochem J. 1963 Mar;86:432–446. doi: 10.1042/bj0860432. [DOI] [PMC free article] [PubMed] [Google Scholar]
- HASLAM R. J., KREBS H. A. THE METABOLISM OF GLUTAMATE IN HOMOGENATES AND SLICES OF BRAIN CORTEX. Biochem J. 1963 Sep;88:566–578. doi: 10.1042/bj0880566. [DOI] [PMC free article] [PubMed] [Google Scholar]
- HIRD F. J., MARGINSON M. A. FORMATION OF AMMONIA FROM GLUTAMATE BY MITOCHONDRIA. Nature. 1964 Mar 21;201:1224–1225. doi: 10.1038/2011224a0. [DOI] [PubMed] [Google Scholar]
- JONES E. A., GUTFREUND H. The control of some oxidative pathways in guinea-pig mammary-gland mitochondria. Biochem J. 1961 Jun;79:608–614. doi: 10.1042/bj0790608. [DOI] [PMC free article] [PubMed] [Google Scholar]
- KARMEN A. A note on the spectrometric assay of glutamic-oxalacetic transaminase in human blood serum. J Clin Invest. 1955 Jan;34(1):131–133. [PubMed] [Google Scholar]
- KLINGENBERG M., PETTE D. Proportions of mitochondrial enzymes and pyridine nucleotides. Biochem Biophys Res Commun. 1962 Jun 4;7:430–432. doi: 10.1016/0006-291x(62)90329-7. [DOI] [PubMed] [Google Scholar]
- KREBS H. A., BELLAMY D. The interconversion of glutamic acid and aspartic acid in respiring tissues. Biochem J. 1960 Jun;75:523–529. doi: 10.1042/bj0750523. [DOI] [PMC free article] [PubMed] [Google Scholar]
- LEAT W. M. FATTY ACID COMPOSITION OF THE SERUM LIPIDS OF PIGS GIVEN DIFFERENT AMOUNTS OF LINOLEIC ACID. Biochem J. 1963 Oct;89:44–51. doi: 10.1042/bj0890044. [DOI] [PMC free article] [PubMed] [Google Scholar]
- LOWRY O. H., ROSEBROUGH N. J., FARR A. L., RANDALL R. J. Protein measurement with the Folin phenol reagent. J Biol Chem. 1951 Nov;193(1):265–275. [PubMed] [Google Scholar]
- OLSON J. A., ANFINSEN C. B. The crystallization and characterization of L-glutamic acid dehydrogenase. J Biol Chem. 1952 May;197(1):67–79. [PubMed] [Google Scholar]