Abstract
Monte Carlo simulations were used to describe the interaction of peripheral and integral proteins with lipids in terms of heat capacity profiles and protein distribution. The simulations were based on a two-state model for the lipid, representing the lipid state as being either gel or fluid. The interaction between neighboring lipids has been taken into account through an unlike nearest neighbor free energy term delta omega, which is a measure of the cooperativity of the lipid transition. Lipid/protein interaction was considered using the experimental observation that the transition midpoints of lipid membranes are shifted upon protein binding, a thermodynamic consequence of different binding constants of protein with fluid or gel lipids. The difference of the binding free energies was used as an additional parameter to describe lipid-protein interaction. The heat capacity profiles of lipid/protein complexes could be well described for both peripheral and integral proteins. Binding of proteins results in a shift and an asymmetric broadening of the melting profile. The model results in a coexistence of gel and fluid lipid domains in the proximity of the thermotropic transition. As a consequence, bound peripheral proteins aggregate in the temperature range of the lipid transition. Integral proteins induce calorimetric melting curves that are qualitatively different from that of peripheral proteins and aggregate in either gel or liquid crystalline lipid phase. The results presented here are in good agreement with calorimetric experiments on lipid-protein complexes and have implementations for the functional control of proteins.
Full text
PDF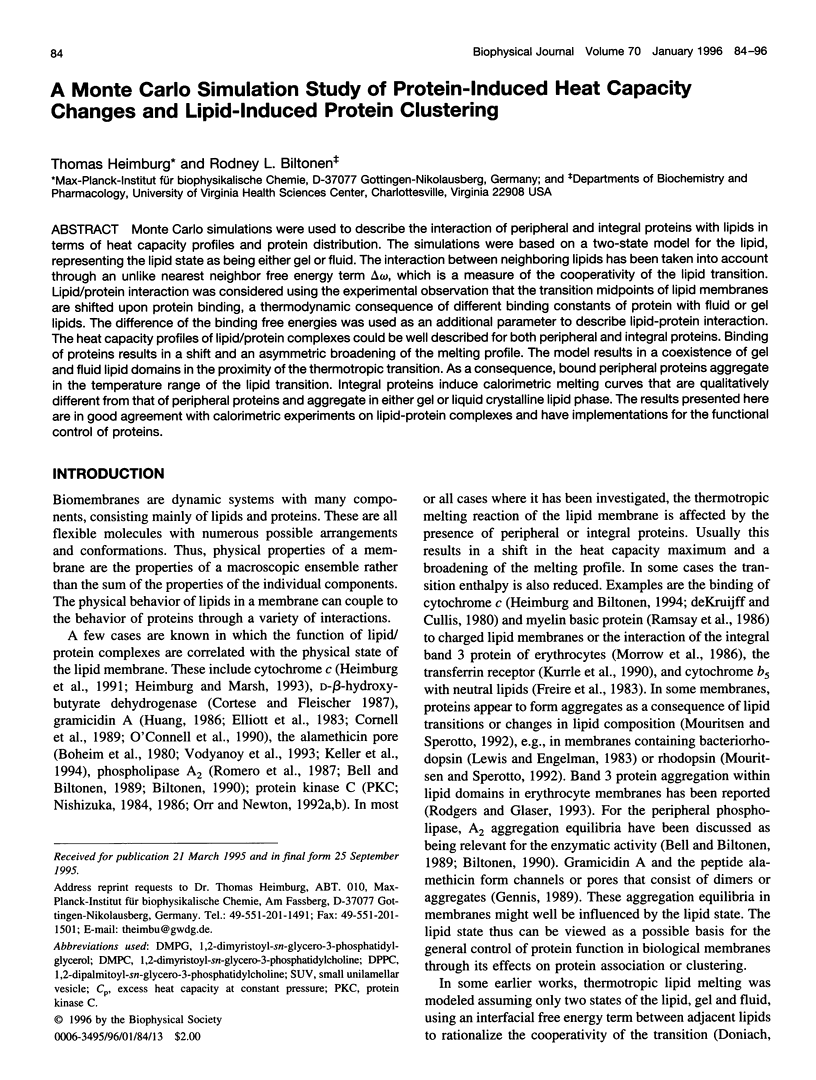
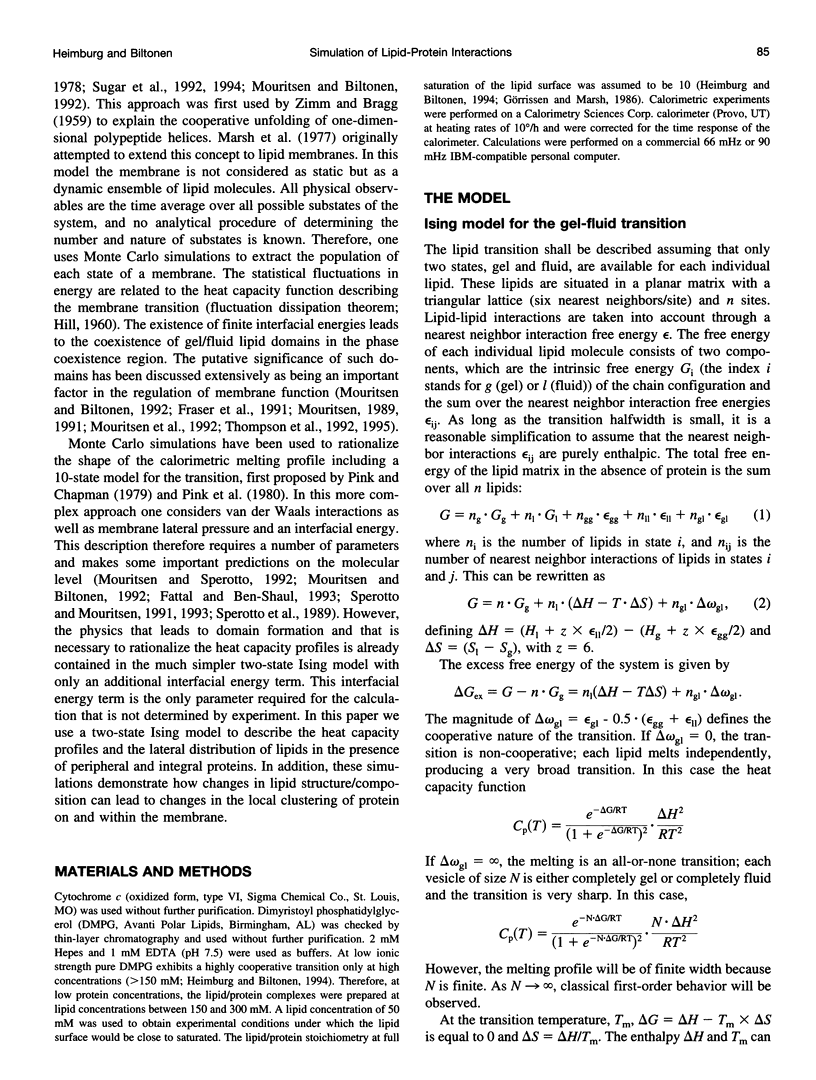
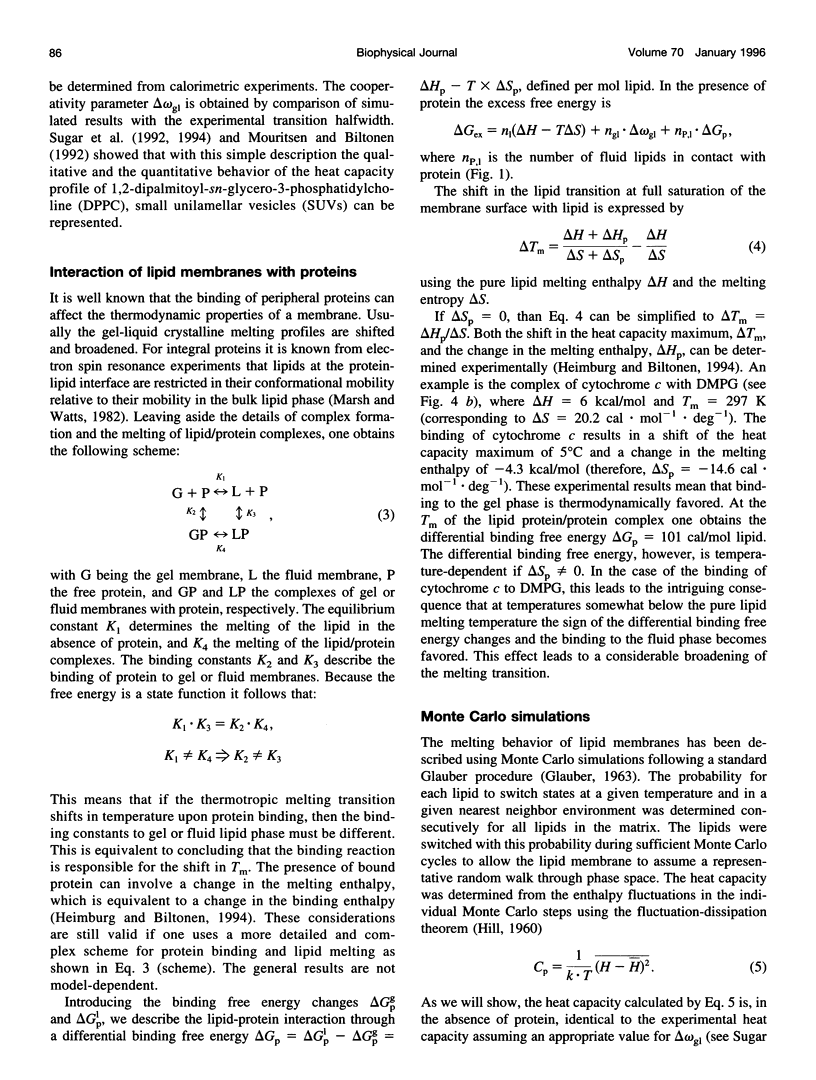
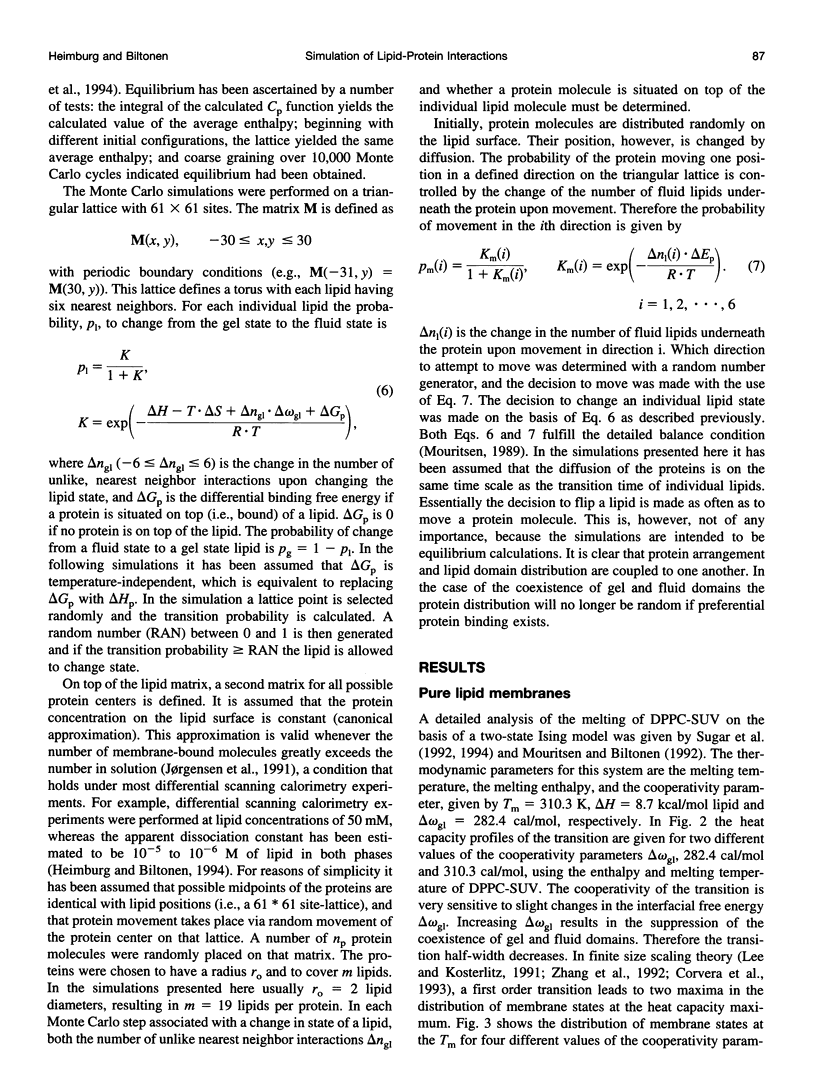
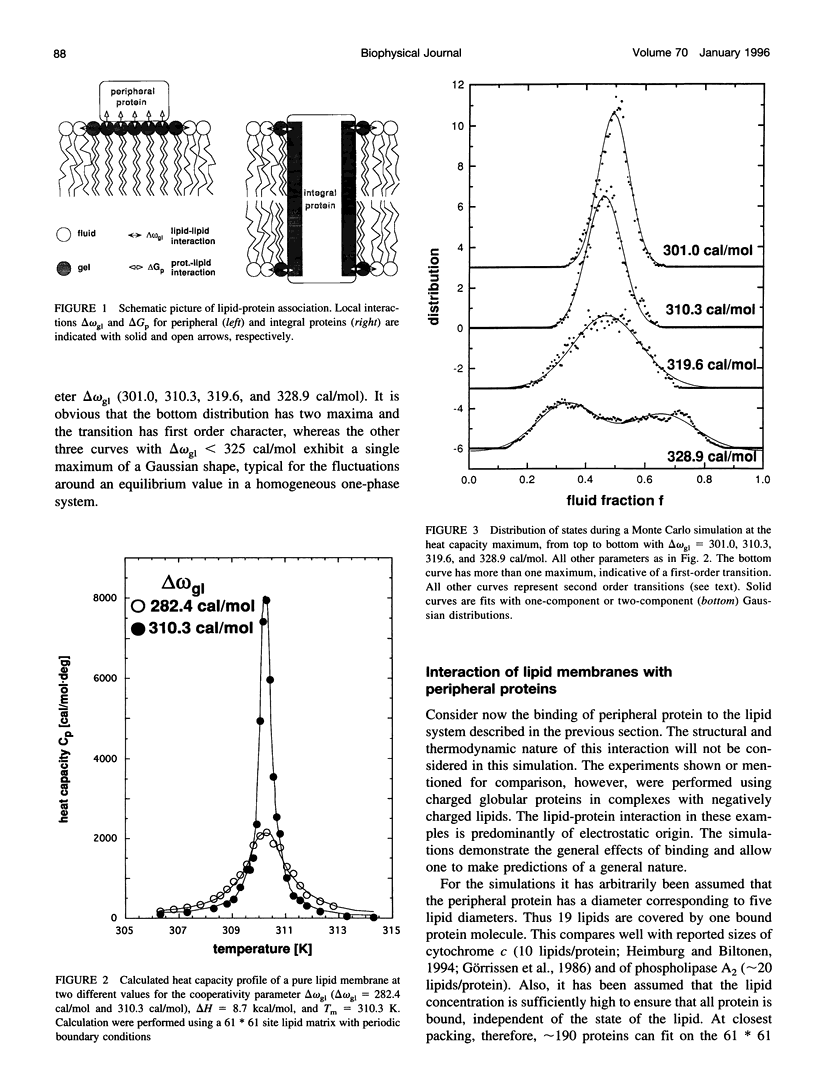
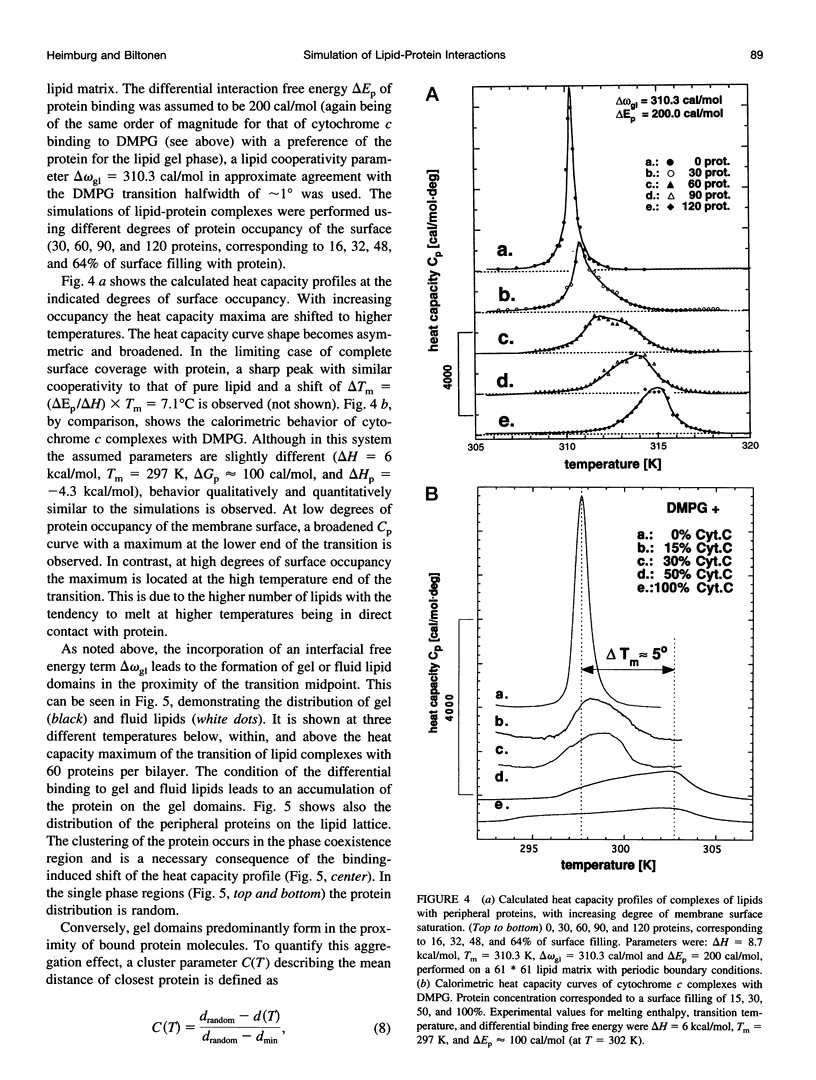
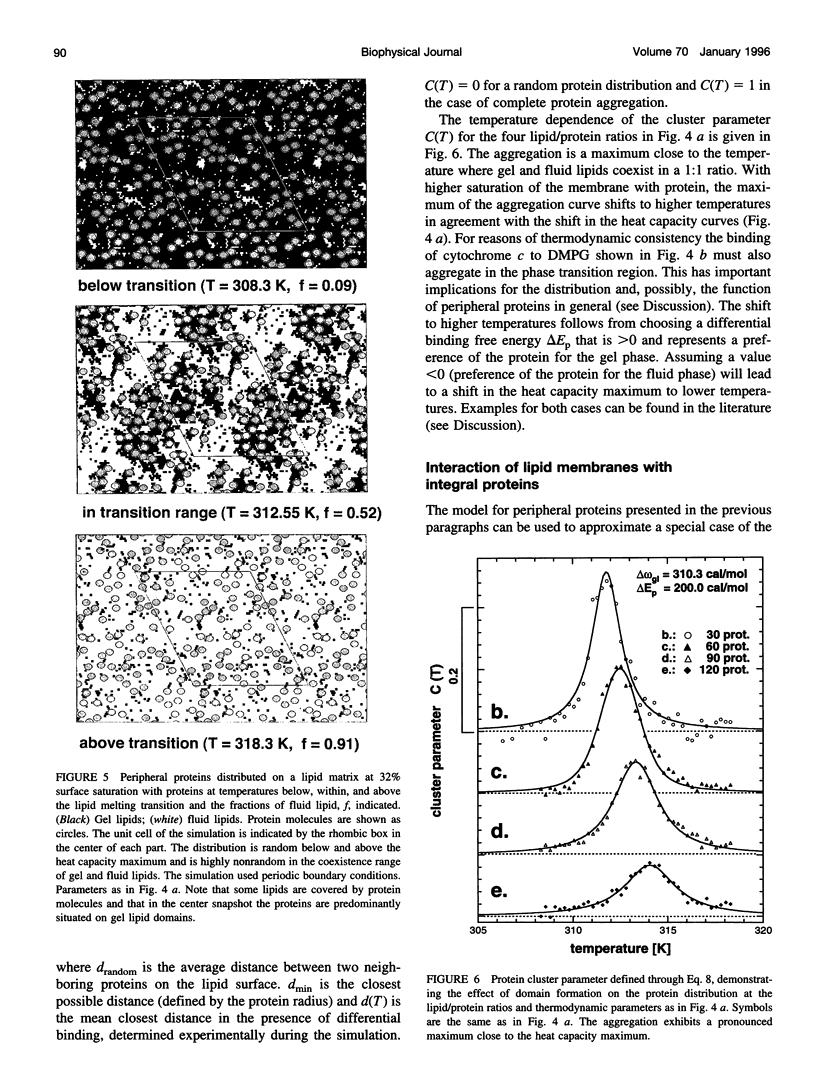
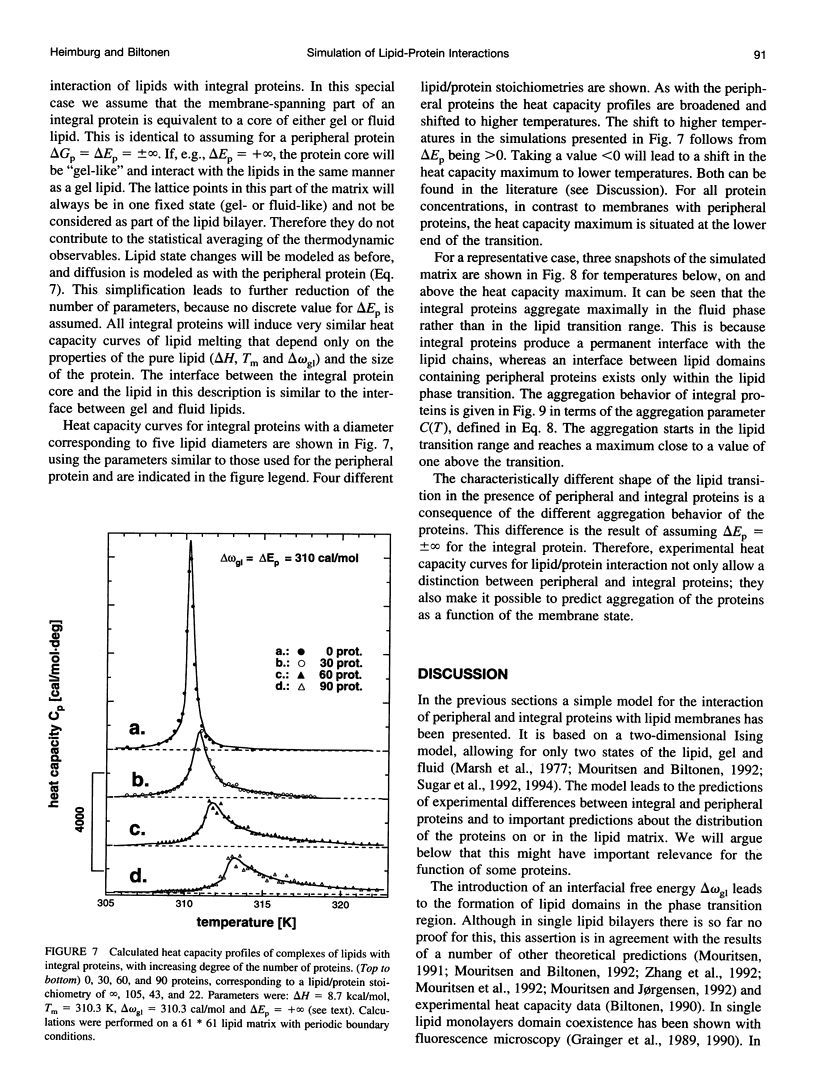
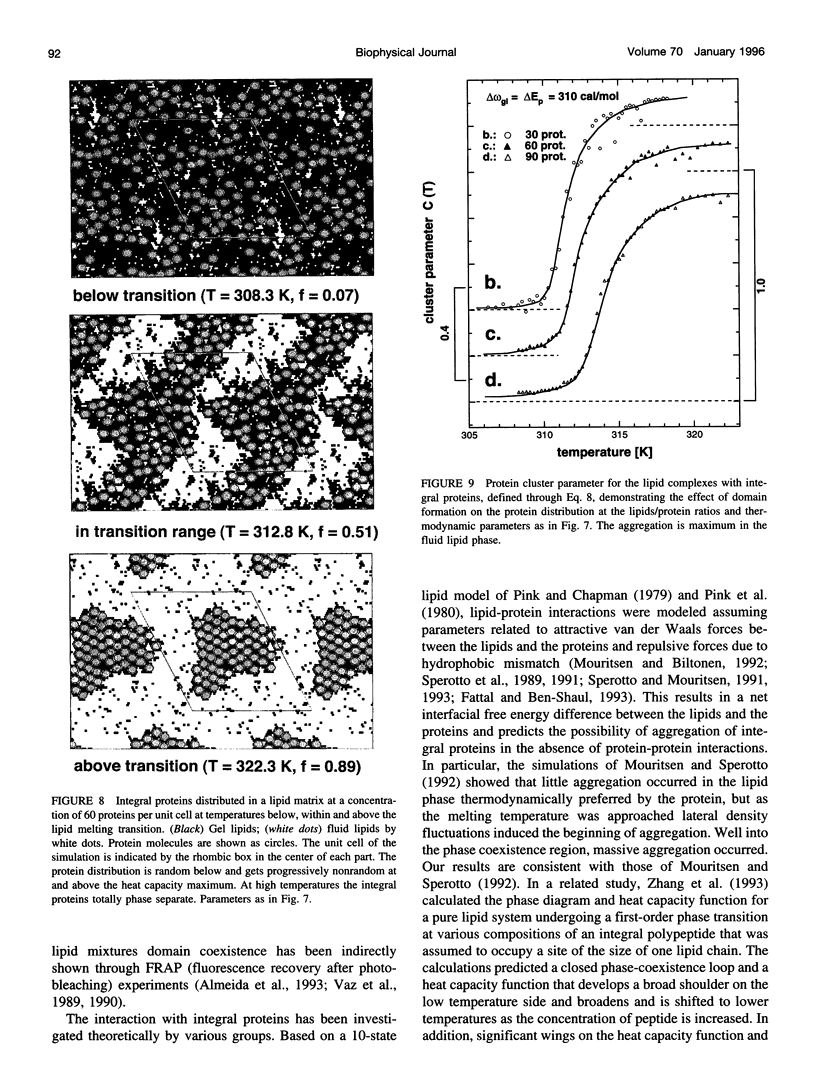
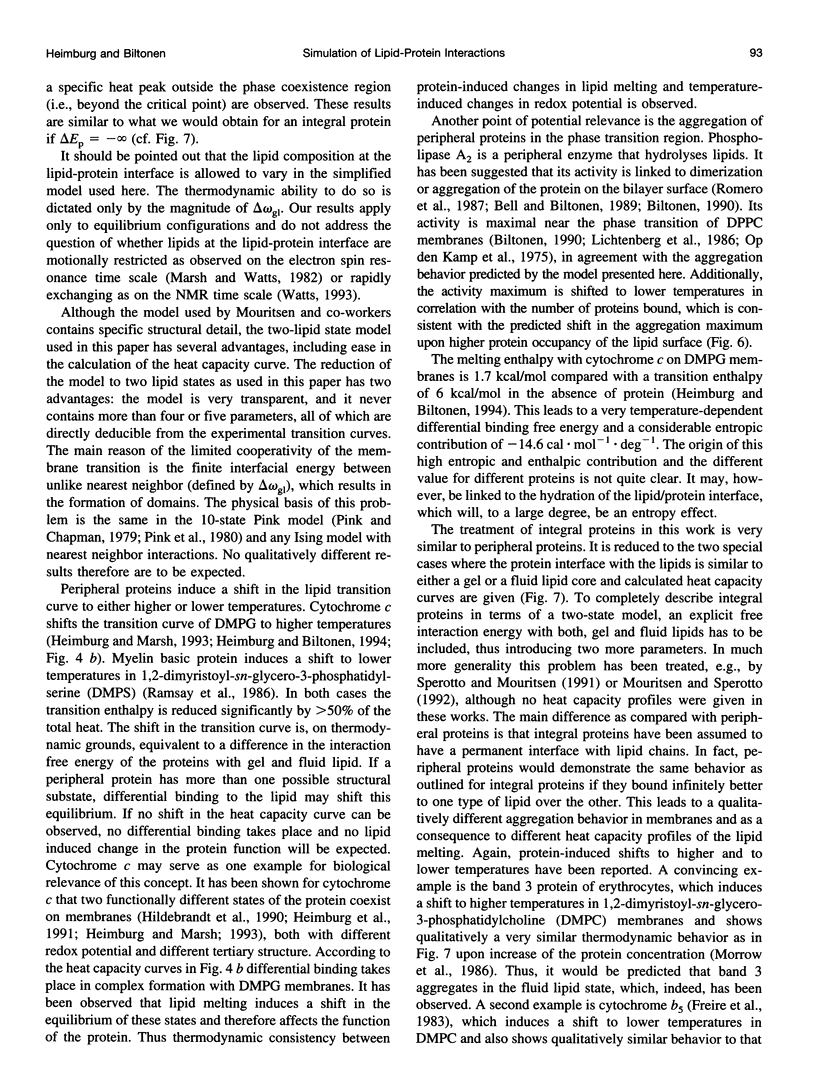
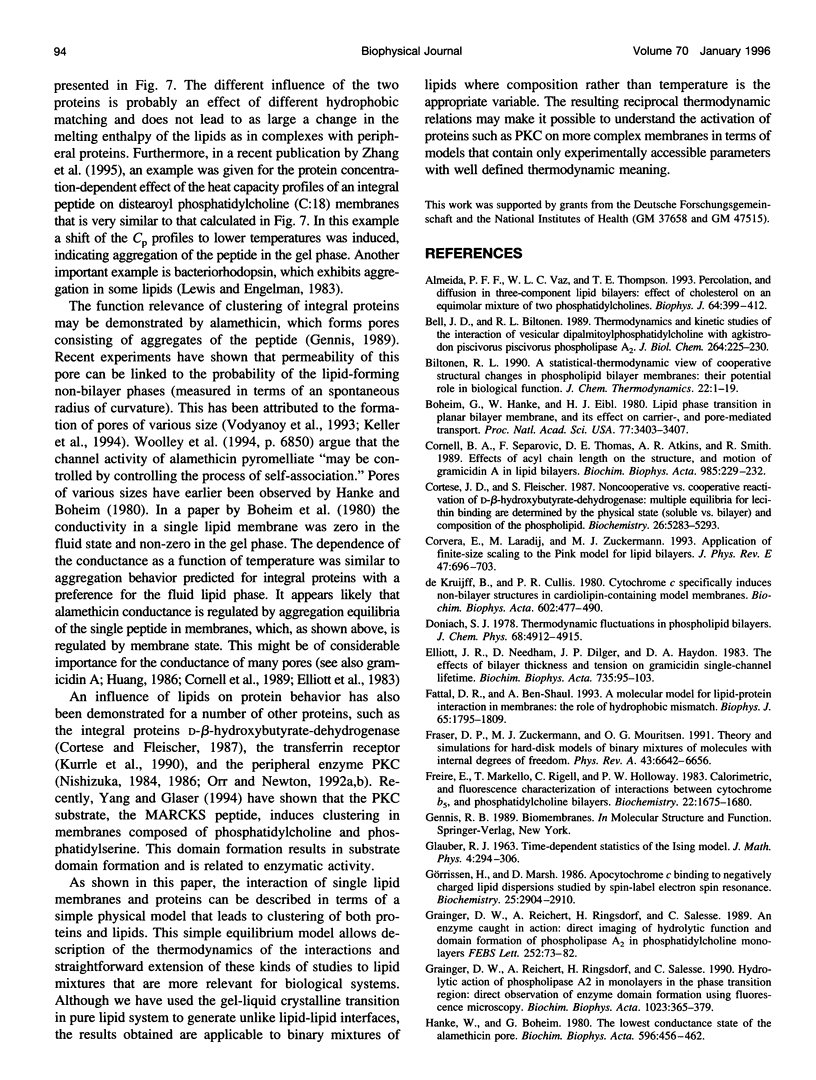
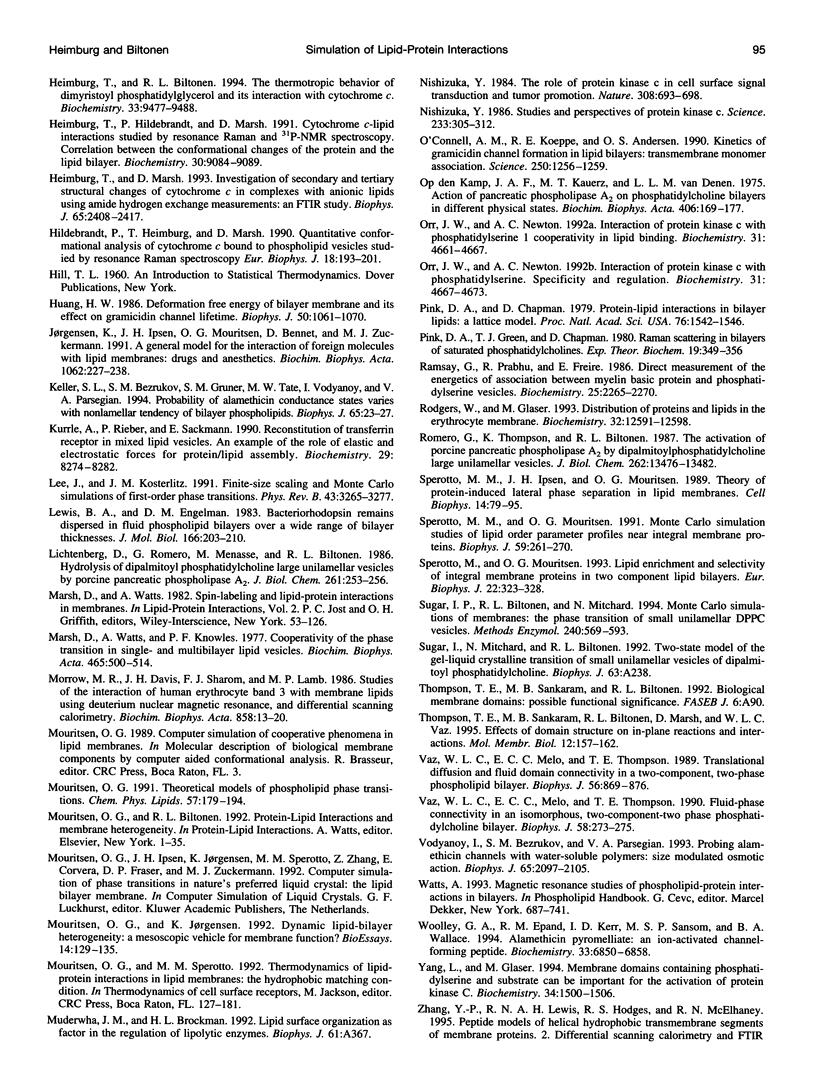
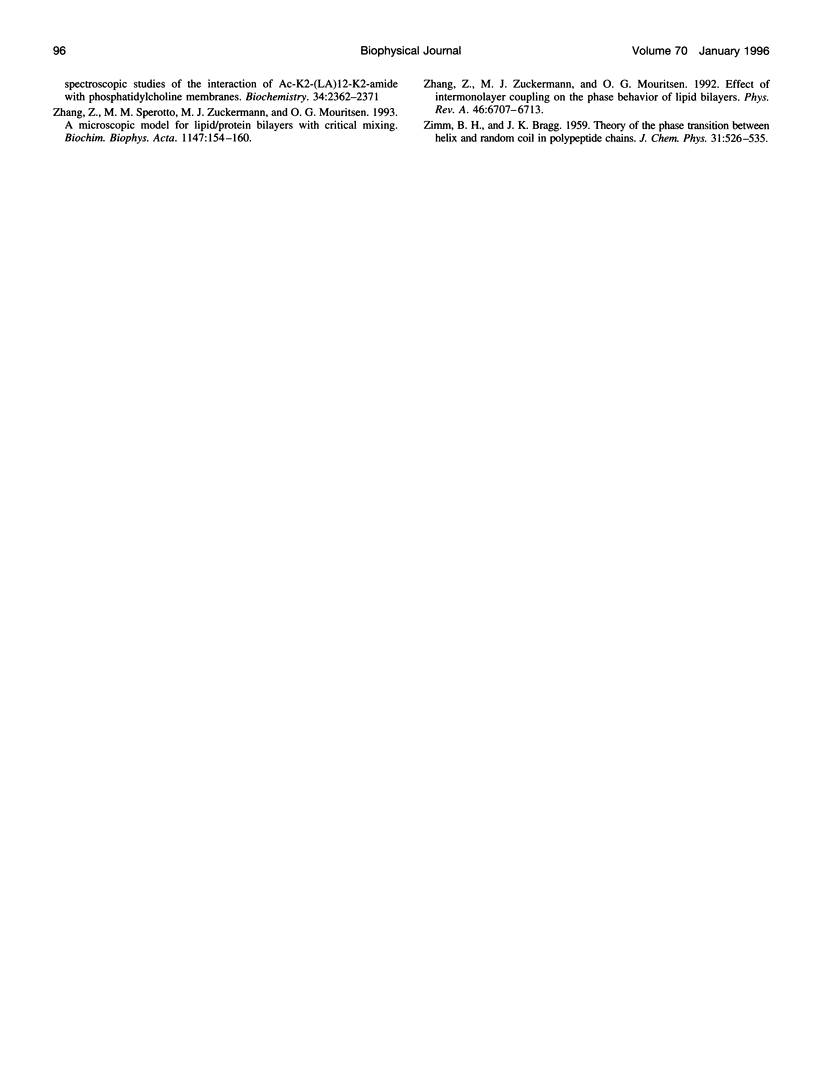
Images in this article
Selected References
These references are in PubMed. This may not be the complete list of references from this article.
- Almeida P. F., Vaz W. L., Thompson T. E. Percolation and diffusion in three-component lipid bilayers: effect of cholesterol on an equimolar mixture of two phosphatidylcholines. Biophys J. 1993 Feb;64(2):399–412. doi: 10.1016/S0006-3495(93)81381-X. [DOI] [PMC free article] [PubMed] [Google Scholar]
- Bell J. D., Biltonen R. L. Thermodynamic and kinetic studies of the interaction of vesicular dipalmitoylphosphatidylcholine with Agkistrodon piscivorus piscivorus phospholipase A2. J Biol Chem. 1989 Jan 5;264(1):225–230. [PubMed] [Google Scholar]
- Boheim G., Hanke W., Eibl H. Lipid phase transition in planar bilayer membrane and its effect on carrier- and pore-mediated ion transport. Proc Natl Acad Sci U S A. 1980 Jun;77(6):3403–3407. doi: 10.1073/pnas.77.6.3403. [DOI] [PMC free article] [PubMed] [Google Scholar]
- Burke M., Purvis S. F., Sivaramakrishnan M. Isolation of heavy chain isoenzymes of myosin subfragment 1 by high performance ion exchange chromatography. J Biol Chem. 1986 Jan 5;261(1):253–256. [PubMed] [Google Scholar]
- Cornell B. A., Separovic F., Thomas D. E., Atkins A. R., Smith R. Effect of acyl chain length on the structure and motion of gramicidin A in lipid bilayers. Biochim Biophys Acta. 1989 Oct 16;985(2):229–232. doi: 10.1016/0005-2736(89)90368-4. [DOI] [PubMed] [Google Scholar]
- Cortese J. D., Fleischer S. Noncooperative vs. cooperative reactivation of D-beta-hydroxybutyrate dehydrogenase: multiple equilibria for lecithin binding are determined by the physical state (soluble vs. bilayer) and composition of the phospholipids. Biochemistry. 1987 Aug 25;26(17):5283–5293. doi: 10.1021/bi00391a011. [DOI] [PubMed] [Google Scholar]
- Corvera E, Laradji M, Zuckermann MJ. Application of finite-size scaling to the Pink model for lipid bilayers. Phys Rev E Stat Phys Plasmas Fluids Relat Interdiscip Topics. 1993 Jan;47(1):696–703. doi: 10.1103/physreve.47.696. [DOI] [PubMed] [Google Scholar]
- Elliott J. R., Needham D., Dilger J. P., Haydon D. A. The effects of bilayer thickness and tension on gramicidin single-channel lifetime. Biochim Biophys Acta. 1983 Oct 26;735(1):95–103. doi: 10.1016/0005-2736(83)90264-x. [DOI] [PubMed] [Google Scholar]
- Fattal D. R., Ben-Shaul A. A molecular model for lipid-protein interaction in membranes: the role of hydrophobic mismatch. Biophys J. 1993 Nov;65(5):1795–1809. doi: 10.1016/S0006-3495(93)81249-9. [DOI] [PMC free article] [PubMed] [Google Scholar]
- Fraser DP, Zuckermann MJ, Mouritsen OG. Theory and simulations for hard-disk models of binary mixtures of molecules with internal degrees of freedom. Phys Rev A. 1991 Jun 15;43(12):6642–6656. doi: 10.1103/physreva.43.6642. [DOI] [PubMed] [Google Scholar]
- Freire E., Markello T., Rigell C., Holloway P. W. Calorimetric and fluorescence characterization of interactions between cytochrome b5 and phosphatidylcholine bilayers. Biochemistry. 1983 Mar 29;22(7):1675–1680. doi: 10.1021/bi00276a024. [DOI] [PubMed] [Google Scholar]
- Grainger D. W., Reichert A., Ringsdorf H., Salesse C. Hydrolytic action of phospholipase A2 in monolayers in the phase transition region: direct observation of enzyme domain formation using fluorescence microscopy. Biochim Biophys Acta. 1990 Apr 30;1023(3):365–379. doi: 10.1016/0005-2736(90)90128-b. [DOI] [PubMed] [Google Scholar]
- Görrissen H., Marsh D., Rietveld A., de Kruijff B. Apocytochrome c binding to negatively charged lipid dispersions studied by spin-label electron spin resonance. Biochemistry. 1986 May 20;25(10):2904–2910. doi: 10.1021/bi00358a025. [DOI] [PubMed] [Google Scholar]
- Hanke W., Boheim G. The lowest conductance state of the alamethicin pore. Biochim Biophys Acta. 1980 Mar 13;596(3):456–462. doi: 10.1016/0005-2736(80)90134-0. [DOI] [PubMed] [Google Scholar]
- Heimburg T., Biltonen R. L. Thermotropic behavior of dimyristoylphosphatidylglycerol and its interaction with cytochrome c. Biochemistry. 1994 Aug 16;33(32):9477–9488. doi: 10.1021/bi00198a013. [DOI] [PubMed] [Google Scholar]
- Heimburg T., Hildebrandt P., Marsh D. Cytochrome c-lipid interactions studied by resonance Raman and 31P NMR spectroscopy. Correlation between the conformational changes of the protein and the lipid bilayer. Biochemistry. 1991 Sep 17;30(37):9084–9089. doi: 10.1021/bi00101a025. [DOI] [PubMed] [Google Scholar]
- Heimburg T., Marsh D. Investigation of secondary and tertiary structural changes of cytochrome c in complexes with anionic lipids using amide hydrogen exchange measurements: an FTIR study. Biophys J. 1993 Dec;65(6):2408–2417. doi: 10.1016/S0006-3495(93)81299-2. [DOI] [PMC free article] [PubMed] [Google Scholar]
- Hildebrandt P., Heimburg T., Marsh D. Quantitative conformational analysis of cytochrome c bound to phospholipid vesicles studied by resonance Raman spectroscopy. Eur Biophys J. 1990;18(3):193–201. doi: 10.1007/BF02427378. [DOI] [PubMed] [Google Scholar]
- Huang H. W. Deformation free energy of bilayer membrane and its effect on gramicidin channel lifetime. Biophys J. 1986 Dec;50(6):1061–1070. doi: 10.1016/S0006-3495(86)83550-0. [DOI] [PMC free article] [PubMed] [Google Scholar]
- Jørgensen K., Ipsen J. H., Mouritsen O. G., Bennett D., Zuckermann M. J. A general model for the interaction of foreign molecules with lipid membranes: drugs and anaesthetics. Biochim Biophys Acta. 1991 Feb 25;1062(2):227–238. doi: 10.1016/0005-2736(91)90397-q. [DOI] [PubMed] [Google Scholar]
- Keller S. L., Bezrukov S. M., Gruner S. M., Tate M. W., Vodyanoy I., Parsegian V. A. Probability of alamethicin conductance states varies with nonlamellar tendency of bilayer phospholipids. Biophys J. 1993 Jul;65(1):23–27. doi: 10.1016/S0006-3495(93)81040-3. [DOI] [PMC free article] [PubMed] [Google Scholar]
- Kurrle A., Rieber P., Sackmann E. Reconstitution of transferrin receptor in mixed lipid vesicles. An example of the role of elastic and electrostatic forces for protein/lipid assembly. Biochemistry. 1990 Sep 11;29(36):8274–8282. doi: 10.1021/bi00488a011. [DOI] [PubMed] [Google Scholar]
- Lee J, Kosterlitz JM. Finite-size scaling and Monte Carlo simulations of first-order phase transitions. Phys Rev B Condens Matter. 1991 Feb 1;43(4):3265–3277. doi: 10.1103/physrevb.43.3265. [DOI] [PubMed] [Google Scholar]
- Lewis B. A., Engelman D. M. Bacteriorhodopsin remains dispersed in fluid phospholipid bilayers over a wide range of bilayer thicknesses. J Mol Biol. 1983 May 15;166(2):203–210. doi: 10.1016/s0022-2836(83)80006-0. [DOI] [PubMed] [Google Scholar]
- Marsh D., Watts A., Knowles P. F. Cooperativity of the phase transition in single- and multibilayer lipid vesicles. Biochim Biophys Acta. 1977 Mar 17;465(3):500–514. doi: 10.1016/0005-2736(77)90268-1. [DOI] [PubMed] [Google Scholar]
- Morrow M. R., Davis J. H., Sharom F. J., Lamb M. P. Studies on the interaction of human erythrocyte band 3 with membrane lipids using deuterium nuclear magnetic resonance and differential scanning calorimetry. Biochim Biophys Acta. 1986 Jun 13;858(1):13–20. doi: 10.1016/0005-2736(86)90286-5. [DOI] [PubMed] [Google Scholar]
- Mouritsen O. G., Jørgensen K. Dynamic lipid-bilayer heterogeneity: a mesoscopic vehicle for membrane function? Bioessays. 1992 Feb;14(2):129–136. doi: 10.1002/bies.950140211. [DOI] [PubMed] [Google Scholar]
- Mouritsen O. G. Theoretical models of phospholipid phase transitions. Chem Phys Lipids. 1991 Mar;57(2-3):179–194. doi: 10.1016/0009-3084(91)90075-m. [DOI] [PubMed] [Google Scholar]
- Nishizuka Y. Studies and perspectives of protein kinase C. Science. 1986 Jul 18;233(4761):305–312. doi: 10.1126/science.3014651. [DOI] [PubMed] [Google Scholar]
- Nishizuka Y. The role of protein kinase C in cell surface signal transduction and tumour promotion. Nature. 1984 Apr 19;308(5961):693–698. doi: 10.1038/308693a0. [DOI] [PubMed] [Google Scholar]
- O'Connell A. M., Koeppe R. E., 2nd, Andersen O. S. Kinetics of gramicidin channel formation in lipid bilayers: transmembrane monomer association. Science. 1990 Nov 30;250(4985):1256–1259. doi: 10.1126/science.1700867. [DOI] [PubMed] [Google Scholar]
- Op den Kamp J. A., Kauerz M. T., van Deenen L. L. Action of pancreatic phospholipase A2 on phosphatidylcholine bilayers in different physical states. Biochim Biophys Acta. 1975 Oct 6;406(2):169–177. doi: 10.1016/0005-2736(75)90001-2. [DOI] [PubMed] [Google Scholar]
- Orr J. W., Newton A. C. Interaction of protein kinase C with phosphatidylserine. 1. Cooperativity in lipid binding. Biochemistry. 1992 May 19;31(19):4661–4667. doi: 10.1021/bi00134a018. [DOI] [PubMed] [Google Scholar]
- Orr J. W., Newton A. C. Interaction of protein kinase C with phosphatidylserine. 2. Specificity and regulation. Biochemistry. 1992 May 19;31(19):4667–4673. doi: 10.1021/bi00134a019. [DOI] [PubMed] [Google Scholar]
- Pink D. A., Chapman D. Protein-lipid interactions in bilayer membranes: a lattice model. Proc Natl Acad Sci U S A. 1979 Apr;76(4):1542–1546. doi: 10.1073/pnas.76.4.1542. [DOI] [PMC free article] [PubMed] [Google Scholar]
- Pink D. A., Green T. J., Chapman D. Raman scattering in bilayers of saturated phosphatidylcholines. Experiment and theory. Biochemistry. 1980 Jan 22;19(2):349–356. doi: 10.1021/bi00543a016. [DOI] [PubMed] [Google Scholar]
- Ramsay G., Prabhu R., Freire E. Direct measurement of the energetics of association between myelin basic protein and phosphatidylserine vesicles. Biochemistry. 1986 Apr 22;25(8):2265–2270. doi: 10.1021/bi00356a062. [DOI] [PubMed] [Google Scholar]
- Rodgers W., Glaser M. Distributions of proteins and lipids in the erythrocyte membrane. Biochemistry. 1993 Nov 30;32(47):12591–12598. doi: 10.1021/bi00210a007. [DOI] [PubMed] [Google Scholar]
- Romero G., Thompson K., Biltonen R. L. The activation of porcine pancreatic phospholipase A2 by dipalmitoylphosphatidylcholine large unilamellar vesicles. Analysis of the state of aggregation of the activated enzyme. J Biol Chem. 1987 Oct 5;262(28):13476–13482. [PubMed] [Google Scholar]
- Sperotto M. M., Ipsen J. H., Mouritsen O. G. Theory of protein-induced lateral phase separation in lipid membranes. Cell Biophys. 1989 Feb;14(1):79–95. doi: 10.1007/BF02797393. [DOI] [PubMed] [Google Scholar]
- Sperotto M. M., Mouritsen O. G. Lipid enrichment and selectivity of integral membrane proteins in two-component lipid bilayers. Eur Biophys J. 1993;22(5):323–328. doi: 10.1007/BF00213555. [DOI] [PubMed] [Google Scholar]
- Sperotto M. M., Mouritsen O. G. Monte Carlo simulation studies of lipid order parameter profiles near integral membrane proteins. Biophys J. 1991 Feb;59(2):261–270. doi: 10.1016/S0006-3495(91)82219-6. [DOI] [PMC free article] [PubMed] [Google Scholar]
- Sugár I. P., Biltonen R. L., Mitchard N. Monte Carlo simulations of membranes: phase transition of small unilamellar dipalmitoylphosphatidylcholine vesicles. Methods Enzymol. 1994;240:569–593. doi: 10.1016/s0076-6879(94)40064-4. [DOI] [PubMed] [Google Scholar]
- Thompson T. E., Sankaram M. B., Biltonen R. L., Marsh D., Vaz W. L. Effects of domain structure on in-plane reactions and interactions. Mol Membr Biol. 1995 Jan-Mar;12(1):157–162. doi: 10.3109/09687689509038512. [DOI] [PubMed] [Google Scholar]
- Vaz W. L., Melo E. C., Thompson T. E. Fluid phase connectivity in an isomorphous, two-component, two-phase phosphatidylcholine bilayer. Biophys J. 1990 Jul;58(1):273–275. doi: 10.1016/S0006-3495(90)82373-0. [DOI] [PMC free article] [PubMed] [Google Scholar]
- Vaz W. L., Melo E. C., Thompson T. E. Translational diffusion and fluid domain connectivity in a two-component, two-phase phospholipid bilayer. Biophys J. 1989 Nov;56(5):869–876. doi: 10.1016/S0006-3495(89)82733-X. [DOI] [PMC free article] [PubMed] [Google Scholar]
- Vodyanoy I., Bezrukov S. M., Parsegian V. A. Probing alamethicin channels with water-soluble polymers. Size-modulated osmotic action. Biophys J. 1993 Nov;65(5):2097–2105. doi: 10.1016/S0006-3495(93)81245-1. [DOI] [PMC free article] [PubMed] [Google Scholar]
- Woolley G. A., Epand R. M., Kerr I. D., Sansom M. S., Wallace B. A. Alamethicin pyromellitate: an ion-activated channel-forming peptide. Biochemistry. 1994 Jun 7;33(22):6850–6858. doi: 10.1021/bi00188a014. [DOI] [PubMed] [Google Scholar]
- Yang L., Glaser M. Membrane domains containing phosphatidylserine and substrate can be important for the activation of protein kinase C. Biochemistry. 1995 Feb 7;34(5):1500–1506. doi: 10.1021/bi00005a005. [DOI] [PubMed] [Google Scholar]
- Zhang Z., Sperotto M. M., Zuckermann M. J., Mouritsen O. G. A microscopic model for lipid/protein bilayers with critical mixing. Biochim Biophys Acta. 1993 Apr 8;1147(1):154–160. doi: 10.1016/0005-2736(93)90326-u. [DOI] [PubMed] [Google Scholar]
- Zhang Z, Zuckermann MJ, Mouritsen OG. Effect of intermonolayer coupling on the phase behavior of lipid bilayers. Phys Rev A. 1992 Nov 15;46(10):6707–6713. doi: 10.1103/physreva.46.6707. [DOI] [PubMed] [Google Scholar]
- de Kruijff B., Cullis P. R. Cytochrome c specifically induces non-bilayer structures in cardiolipin-containing model membranes. Biochim Biophys Acta. 1980 Nov 18;602(3):477–490. doi: 10.1016/0005-2736(80)90327-2. [DOI] [PubMed] [Google Scholar]