Abstract
Free amphipathic peptides and peptides bound to dimyristoylphosphatidylcholine (DMPC) were studied directly at the air/water interface using polarization modulation infrared reflection absorption spectroscopy (PMIRRAS). Such differential reflectivity measurements proved to be a sensitive and efficient technique to investigate in situ the respective conformations and orientations of lipid and peptide molecules in pure and mixed films. Data obtained for melittin, a natural hemolytic peptide, are compared to those of L15K7, an ideally amphipathic synthetic peptide constituted by only apolar Leu and polar Lys residues. For pure peptidic films, the intensity, shape, and position of the amide I and II bands indicate that the L15K7 peptide adopts a totally alpha-helical structure, whereas the structure of melittin is mainly alpha-helical and presents some unordered domains. The L15K7 alpha-helix axis is oriented essentially parallel to the air-water interface plane; it differs for melittin. When injected into the subphase, L15K7 and melittin insert into preformed expanded DMPC monolayers and can be detected by PMIRRAS, even at low peptide content (> 50 DMPC molecules per peptide). In such conditions, peptides have the same secondary structure and orientation as in pure peptidic films.
Full text
PDF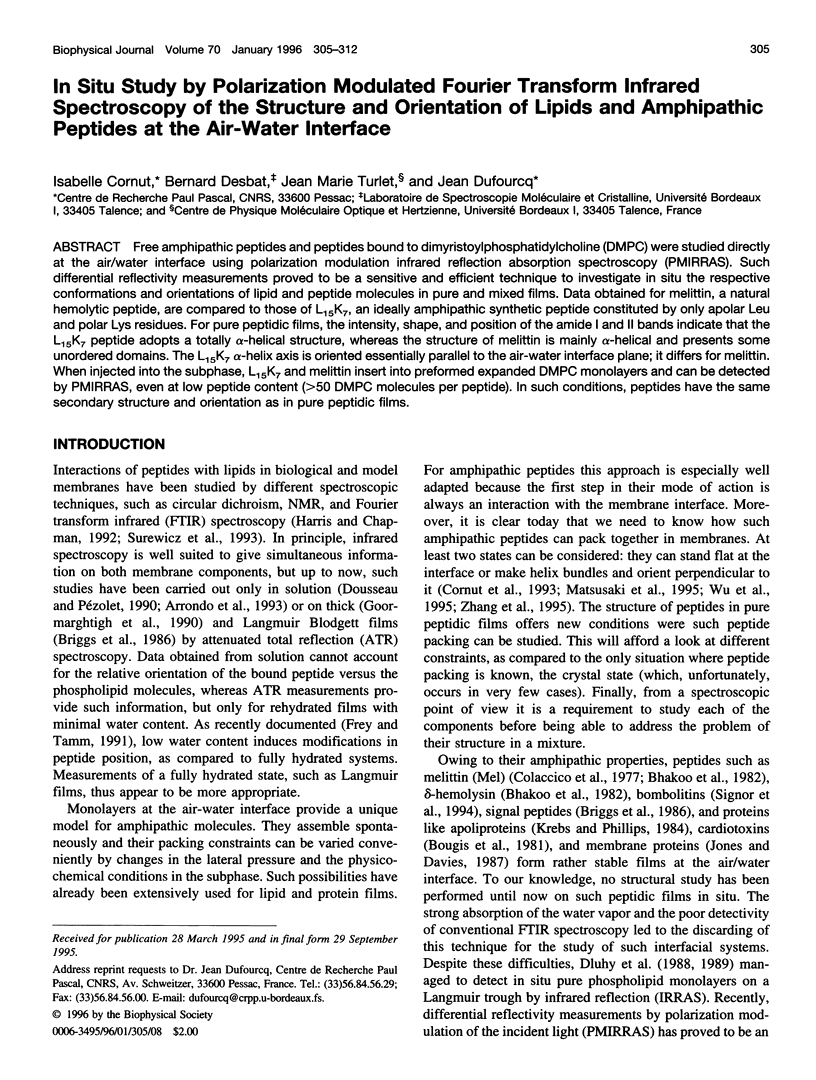
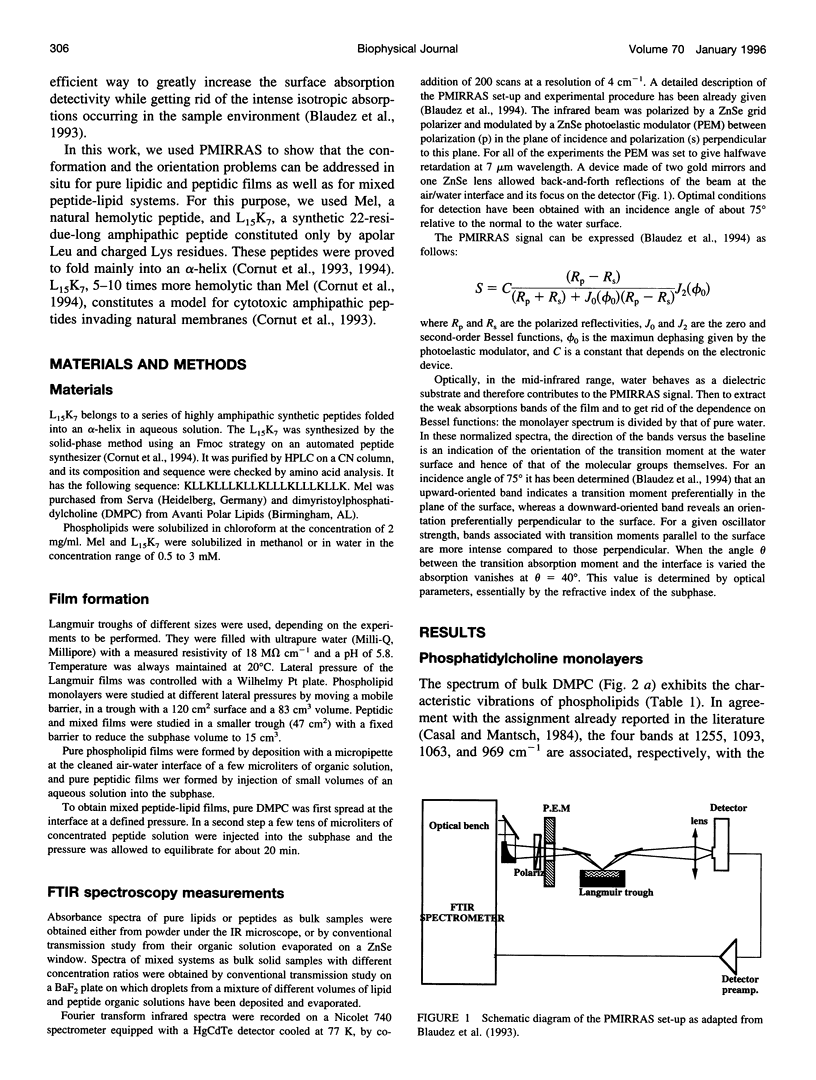
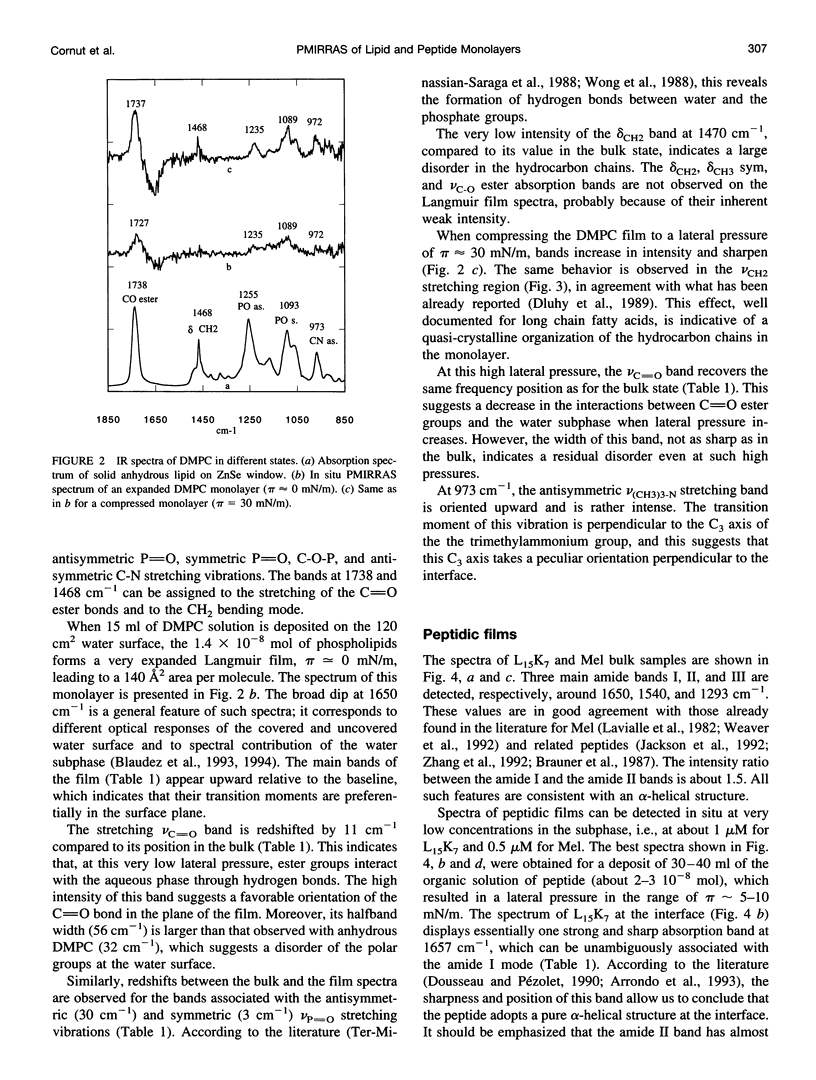
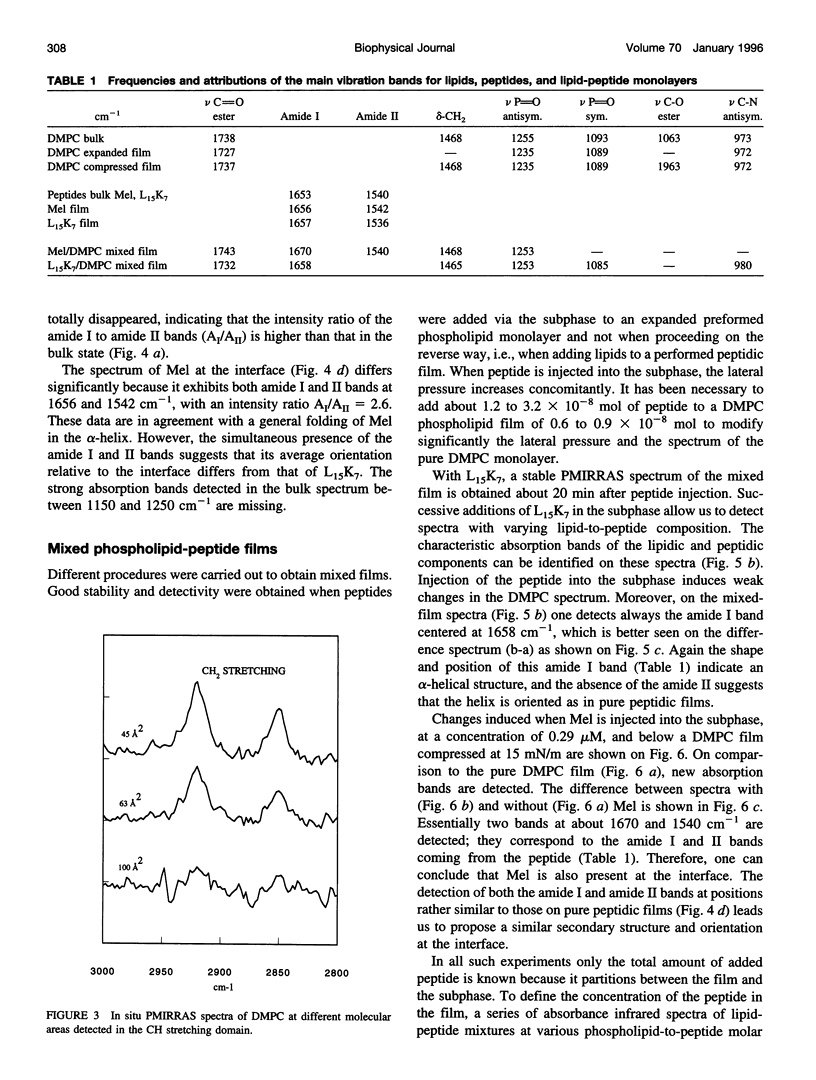
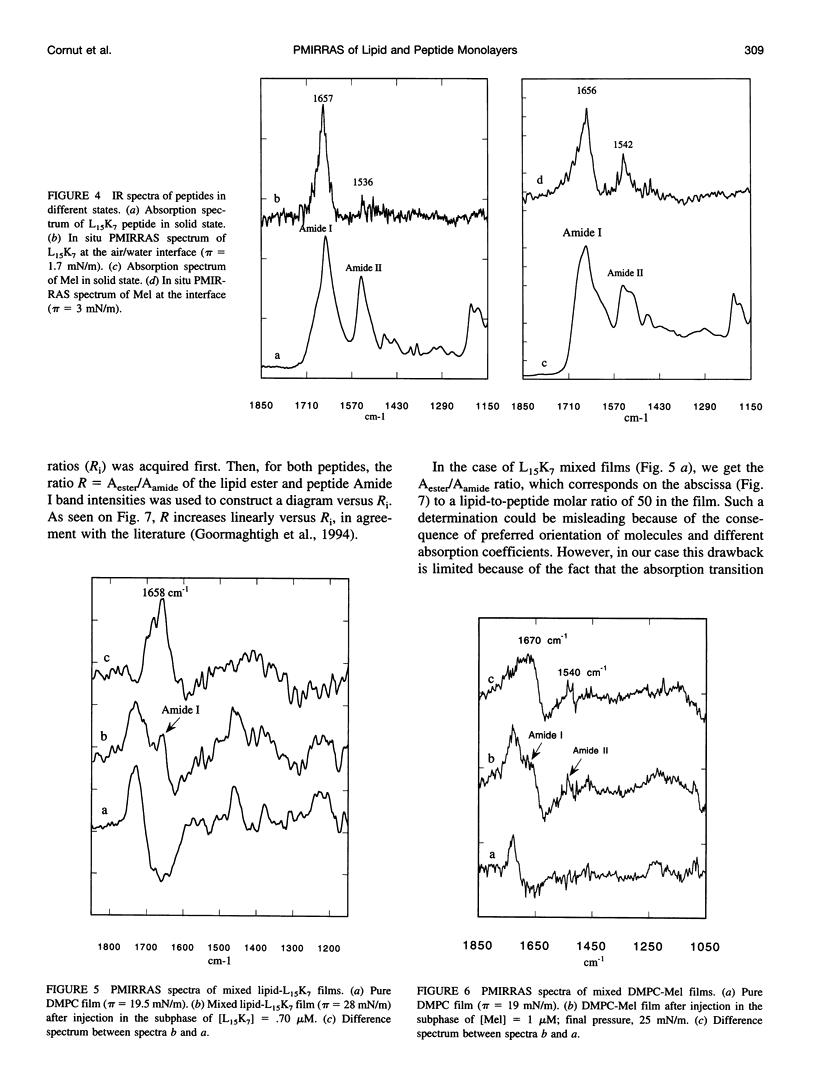
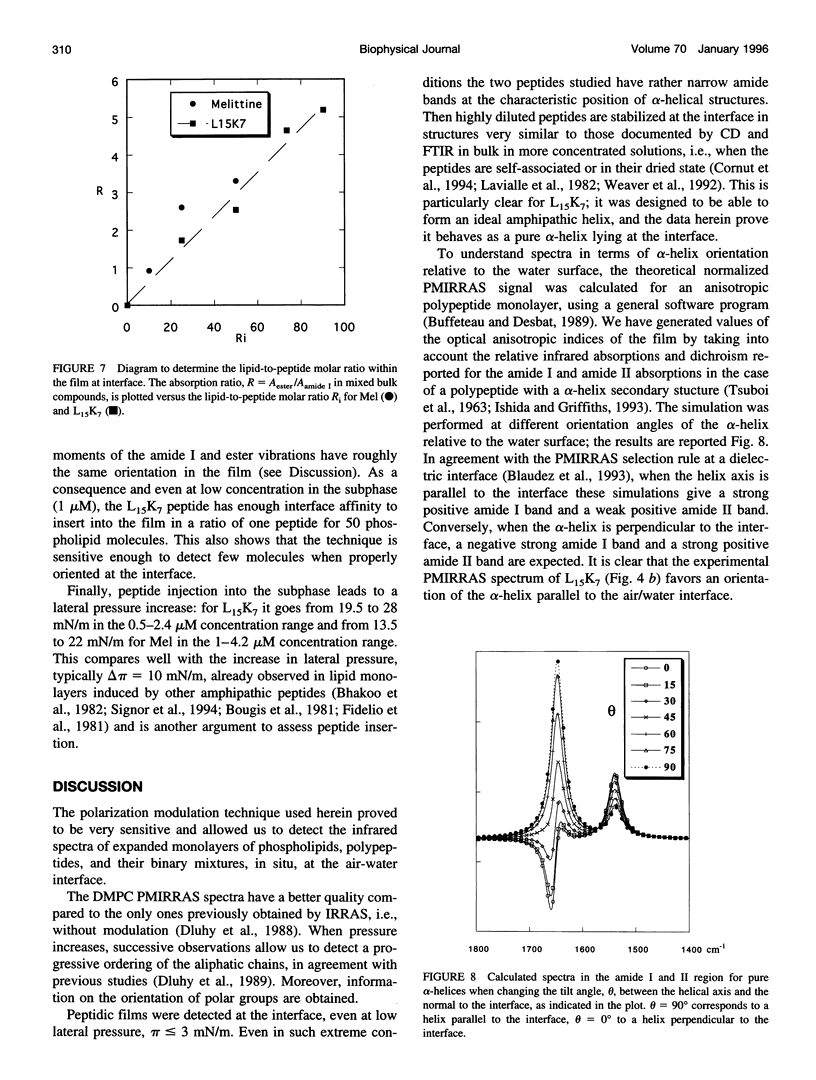
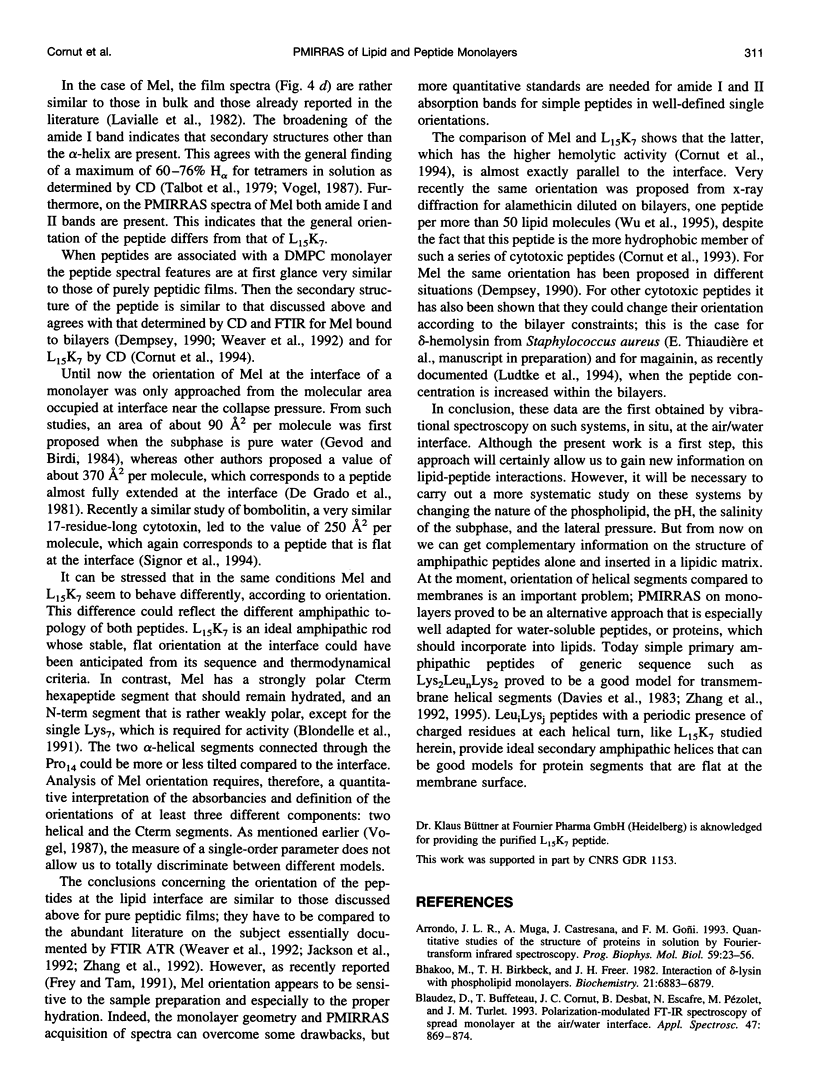

Selected References
These references are in PubMed. This may not be the complete list of references from this article.
- Arrondo J. L., Muga A., Castresana J., Goñi F. M. Quantitative studies of the structure of proteins in solution by Fourier-transform infrared spectroscopy. Prog Biophys Mol Biol. 1993;59(1):23–56. doi: 10.1016/0079-6107(93)90006-6. [DOI] [PubMed] [Google Scholar]
- Bhakoo M., Birkbeck T. H., Freer J. H. Interaction of Staphylococcus aureus delta-lysin with phospholipid monolayers. Biochemistry. 1982 Dec 21;21(26):6879–6883. doi: 10.1021/bi00269a039. [DOI] [PubMed] [Google Scholar]
- Blondelle S. E., Houghten R. A. Hemolytic and antimicrobial activities of the twenty-four individual omission analogues of melittin. Biochemistry. 1991 May 14;30(19):4671–4678. doi: 10.1021/bi00233a006. [DOI] [PubMed] [Google Scholar]
- Bougis P., Rochat H., Piéroni G., Verger R. Penetration of phospholipid monolayers by cardiotoxins. Biochemistry. 1981 Aug 18;20(17):4915–4920. doi: 10.1021/bi00520a017. [DOI] [PubMed] [Google Scholar]
- Brauner J. W., Mendelsohn R., Prendergast F. G. Attenuated total reflectance Fourier transform infrared studies of the interaction of melittin, two fragments of melittin, and delta-hemolysin with phosphatidylcholines. Biochemistry. 1987 Dec 15;26(25):8151–8158. doi: 10.1021/bi00399a020. [DOI] [PubMed] [Google Scholar]
- Briggs M. S., Cornell D. G., Dluhy R. A., Gierasch L. M. Conformations of signal peptides induced by lipids suggest initial steps in protein export. Science. 1986 Jul 11;233(4760):206–208. doi: 10.1126/science.2941862. [DOI] [PubMed] [Google Scholar]
- Casal H. L., Mantsch H. H. Polymorphic phase behaviour of phospholipid membranes studied by infrared spectroscopy. Biochim Biophys Acta. 1984 Dec 4;779(4):381–401. doi: 10.1016/0304-4157(84)90017-0. [DOI] [PubMed] [Google Scholar]
- Colacicco G., Basu M. K., Buckelew A. R., Jr, Bernheimer A. W. Surface properties of membrane systems. Transport of staphylococcal delta-toxin from aqueous to membrane phase. Biochim Biophys Acta. 1977 Mar 1;465(2):378–390. doi: 10.1016/0005-2736(77)90087-6. [DOI] [PubMed] [Google Scholar]
- Cornut I., Büttner K., Dasseux J. L., Dufourcq J. The amphipathic alpha-helix concept. Application to the de novo design of ideally amphipathic Leu, Lys peptides with hemolytic activity higher than that of melittin. FEBS Lett. 1994 Jul 25;349(1):29–33. doi: 10.1016/0014-5793(94)00621-0. [DOI] [PubMed] [Google Scholar]
- Dempsey C. E. The actions of melittin on membranes. Biochim Biophys Acta. 1990 May 7;1031(2):143–161. doi: 10.1016/0304-4157(90)90006-x. [DOI] [PubMed] [Google Scholar]
- Dluhy R. A., Reilly K. E., Hunt R. D., Mitchell M. L., Mautone A. J., Mendelsohn R. Infrared spectroscopic investigations of pulmonary surfactant. Surface film transitions at the air-water interface and bulk phase thermotropism. Biophys J. 1989 Dec;56(6):1173–1181. doi: 10.1016/S0006-3495(89)82764-X. [DOI] [PMC free article] [PubMed] [Google Scholar]
- Dousseau F., Pézolet M. Determination of the secondary structure content of proteins in aqueous solutions from their amide I and amide II infrared bands. Comparison between classical and partial least-squares methods. Biochemistry. 1990 Sep 18;29(37):8771–8779. doi: 10.1021/bi00489a038. [DOI] [PubMed] [Google Scholar]
- Fidelio G. D., Maggio B., Cumar F. A., Caputto R. Interaction of glycosphingolipids with melittin and myelin basis protein in monolayers. Biochem J. 1981 Feb 1;193(2):643–646. doi: 10.1042/bj1930643. [DOI] [PMC free article] [PubMed] [Google Scholar]
- Frey S., Tamm L. K. Orientation of melittin in phospholipid bilayers. A polarized attenuated total reflection infrared study. Biophys J. 1991 Oct;60(4):922–930. doi: 10.1016/S0006-3495(91)82126-9. [DOI] [PMC free article] [PubMed] [Google Scholar]
- Gevod V. S., Birdi K. S. Melittin and the 8-26 fragment. Differences in ionophoric properties as measured by monolayer method. Biophys J. 1984 Jun;45(6):1079–1083. doi: 10.1016/S0006-3495(84)84255-1. [DOI] [PMC free article] [PubMed] [Google Scholar]
- Goormaghtigh E., Cabiaux V., Ruysschaert J. M. Determination of soluble and membrane protein structure by Fourier transform infrared spectroscopy. II. Experimental aspects, side chain structure, and H/D exchange. Subcell Biochem. 1994;23:363–403. doi: 10.1007/978-1-4615-1863-1_9. [DOI] [PubMed] [Google Scholar]
- Goormaghtigh E., Cabiaux V., Ruysschaert J. M. Secondary structure and dosage of soluble and membrane proteins by attenuated total reflection Fourier-transform infrared spectroscopy on hydrated films. Eur J Biochem. 1990 Oct 24;193(2):409–420. doi: 10.1111/j.1432-1033.1990.tb19354.x. [DOI] [PubMed] [Google Scholar]
- Haris P. I., Chapman D. Does Fourier-transform infrared spectroscopy provide useful information on protein structures? Trends Biochem Sci. 1992 Sep;17(9):328–333. doi: 10.1016/0968-0004(92)90305-s. [DOI] [PubMed] [Google Scholar]
- Ishiguro R., Kimura N., Takahashi S. Orientation of fusion-active synthetic peptides in phospholipid bilayers: determination by Fourier transform infrared spectroscopy. Biochemistry. 1993 Sep 21;32(37):9792–9797. doi: 10.1021/bi00088a034. [DOI] [PubMed] [Google Scholar]
- Jackson M., Mantsch H. H., Spencer J. H. Conformation of magainin-2 and related peptides in aqueous solution and membrane environments probed by Fourier transform infrared spectroscopy. Biochemistry. 1992 Aug 18;31(32):7289–7293. doi: 10.1021/bi00147a012. [DOI] [PubMed] [Google Scholar]
- Krebs K. E., Phillips M. C. The contribution of alpha-helices to the surface activities of proteins. FEBS Lett. 1984 Oct 1;175(2):263–266. doi: 10.1016/0014-5793(84)80748-6. [DOI] [PubMed] [Google Scholar]
- Lavialle F., Adams R. G., Levin I. W. Infrared spectroscopic study of the secondary structure of melittin in water, 2-chloroethanol, and phospholipid bilayer dispersions. Biochemistry. 1982 May 11;21(10):2305–2312. doi: 10.1021/bi00539a006. [DOI] [PubMed] [Google Scholar]
- Ludtke S. J., He K., Wu Y., Huang H. W. Cooperative membrane insertion of magainin correlated with its cytolytic activity. Biochim Biophys Acta. 1994 Feb 23;1190(1):181–184. doi: 10.1016/0005-2736(94)90050-7. [DOI] [PubMed] [Google Scholar]
- Matsuzaki K., Murase O., Fujii N., Miyajima K. Translocation of a channel-forming antimicrobial peptide, magainin 2, across lipid bilayers by forming a pore. Biochemistry. 1995 May 16;34(19):6521–6526. doi: 10.1021/bi00019a033. [DOI] [PubMed] [Google Scholar]
- Signor G., Mammi S., Peggion E., Ringsdorf H., Wagenknecht A. Interaction of bombolitin III with phospholipid monolayers and liposomes and effect on the activity of phospholipase A2. Biochemistry. 1994 May 31;33(21):6659–6670. doi: 10.1021/bi00187a036. [DOI] [PubMed] [Google Scholar]
- Surewicz W. K., Mantsch H. H., Chapman D. Determination of protein secondary structure by Fourier transform infrared spectroscopy: a critical assessment. Biochemistry. 1993 Jan 19;32(2):389–394. doi: 10.1021/bi00053a001. [DOI] [PubMed] [Google Scholar]
- Talbot J. C., Dufourcq J., de Bony J., Faucon J. F., Lussan C. Conformational change and self association of monomeric melittin. FEBS Lett. 1979 Jun 1;102(1):191–193. doi: 10.1016/0014-5793(79)80957-6. [DOI] [PubMed] [Google Scholar]
- Ter-Minassian-Saraga L., Okamura E., Umemura J., Takenaka T. Fourier transform infrared-attenuated total reflection spectroscopy of hydration of dimyristoylphosphatidylcholine multibilayers. Biochim Biophys Acta. 1988 Dec 22;946(2):417–423. doi: 10.1016/0005-2736(88)90417-8. [DOI] [PubMed] [Google Scholar]
- Vogel H. Comparison of the conformation and orientation of alamethicin and melittin in lipid membranes. Biochemistry. 1987 Jul 14;26(14):4562–4572. doi: 10.1021/bi00388a060. [DOI] [PubMed] [Google Scholar]
- Vogel H., Jähnig F., Hoffmann V., Stümpel J. The orientation of melittin in lipid membranes. A polarized infrared spectroscopy study. Biochim Biophys Acta. 1983 Sep 7;733(2):201–209. doi: 10.1016/0005-2736(83)90523-0. [DOI] [PubMed] [Google Scholar]
- Weaver A. J., Kemple M. D., Brauner J. W., Mendelsohn R., Prendergast F. G. Fluorescence, CD, attenuated total reflectance (ATR) FTIR, and 13C NMR characterization of the structure and dynamics of synthetic melittin and melittin analogues in lipid environments. Biochemistry. 1992 Feb 11;31(5):1301–1313. doi: 10.1021/bi00120a005. [DOI] [PubMed] [Google Scholar]
- Wong P. T., Capes S. E., Mantsch H. H. Hydrogen bonding between anhydrous cholesterol and phosphatidylcholines: an infrared spectroscopic study. Biochim Biophys Acta. 1989 Mar 27;980(1):37–41. doi: 10.1016/0005-2736(89)90197-1. [DOI] [PubMed] [Google Scholar]
- Wu Y., He K., Ludtke S. J., Huang H. W. X-ray diffraction study of lipid bilayer membranes interacting with amphiphilic helical peptides: diphytanoyl phosphatidylcholine with alamethicin at low concentrations. Biophys J. 1995 Jun;68(6):2361–2369. doi: 10.1016/S0006-3495(95)80418-2. [DOI] [PMC free article] [PubMed] [Google Scholar]
- Zhang Y. P., Lewis R. N., Henry G. D., Sykes B. D., Hodges R. S., McElhaney R. N. Peptide models of helical hydrophobic transmembrane segments of membrane proteins. 1. Studies of the conformation, intrabilayer orientation, and amide hydrogen exchangeability of Ac-K2-(LA)12-K2-amide. Biochemistry. 1995 Feb 21;34(7):2348–2361. doi: 10.1021/bi00007a031. [DOI] [PubMed] [Google Scholar]
- Zhang Y. P., Lewis R. N., Hodges R. S., McElhaney R. N. FTIR spectroscopic studies of the conformation and amide hydrogen exchange of a peptide model of the hydrophobic transmembrane alpha-helices of membrane proteins. Biochemistry. 1992 Nov 24;31(46):11572–11578. doi: 10.1021/bi00161a041. [DOI] [PubMed] [Google Scholar]