Abstract
Ion-coupled transporters are simulated by a model that differs from contemporary alternating-access schemes. Beginning with concepts derived from multi-ion pores, the model assumes that substrates (both inorganic ions and small organic molecules) hop a) between the solutions and binding sites and b) between binding sites within a single-file pore. No two substrates can simultaneously occupy the same site. Rate constants for hopping can be increased both a) when substrates in two sites attract each other into a vacant site between them and b) when substrates in adjacent sites repel each other. Hopping rate constants for charged substrates are also modified by the membrane field. For a three-site model, simulated annealing yields parameters to fit steady-state measurements of flux coupling, transport-associated currents, and charge movements for the GABA transporter GAT1. The model then accounts for some GAT1 kinetic data as well. The model also yields parameters that describe the available data for the rat 5-HT transporter and for the rabbit Na(+)-glucose transporter. The simulations show that coupled fluxes and other aspects of ion transport can be explained by a model that includes local substrate-substrate interactions but no explicit global conformational changes.
Full text
PDF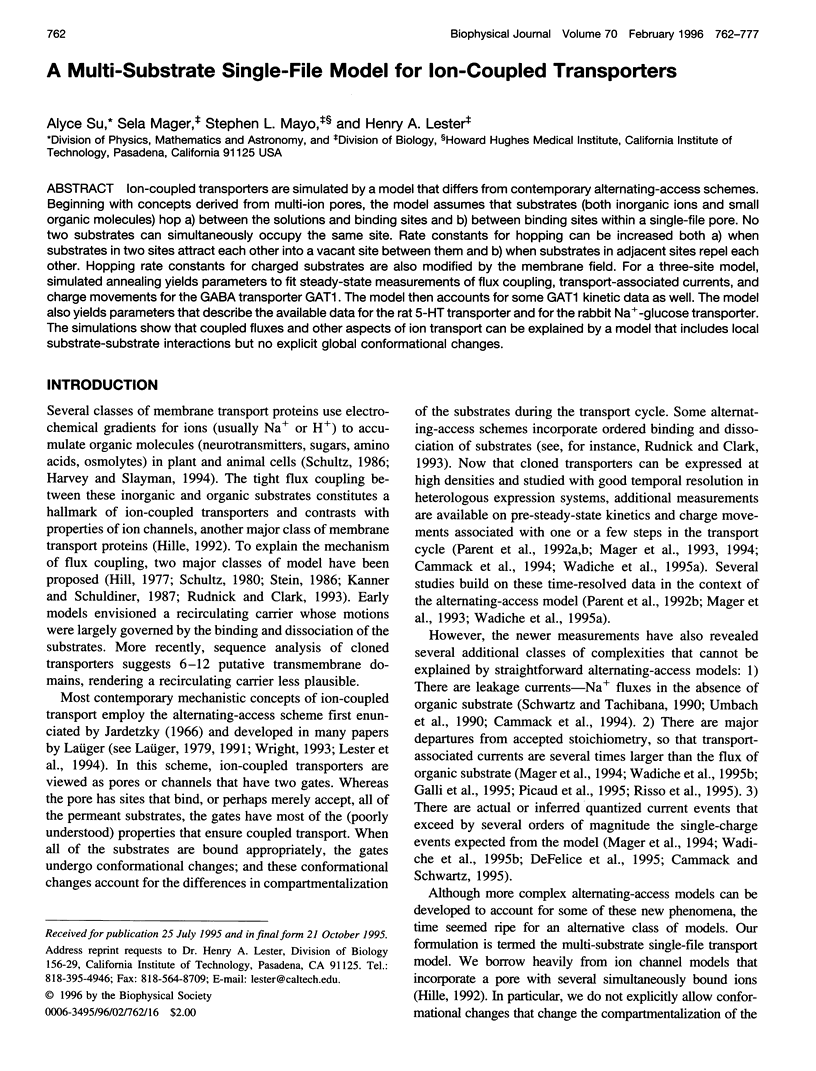
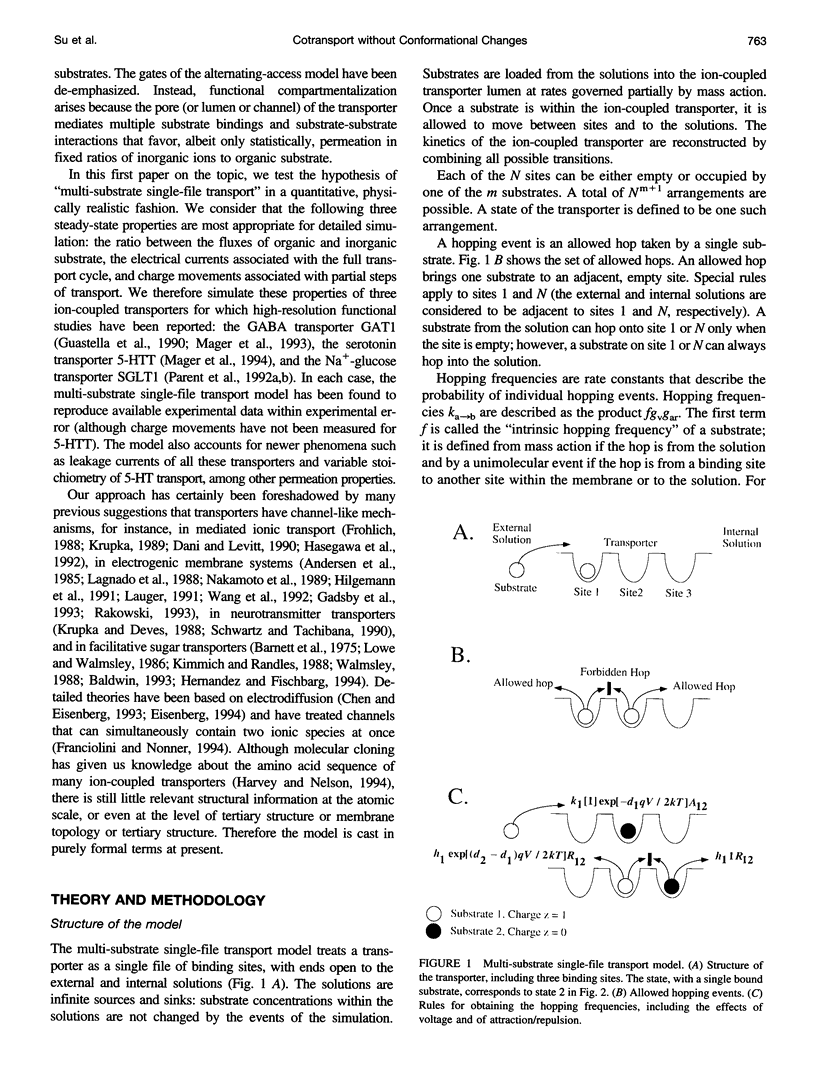
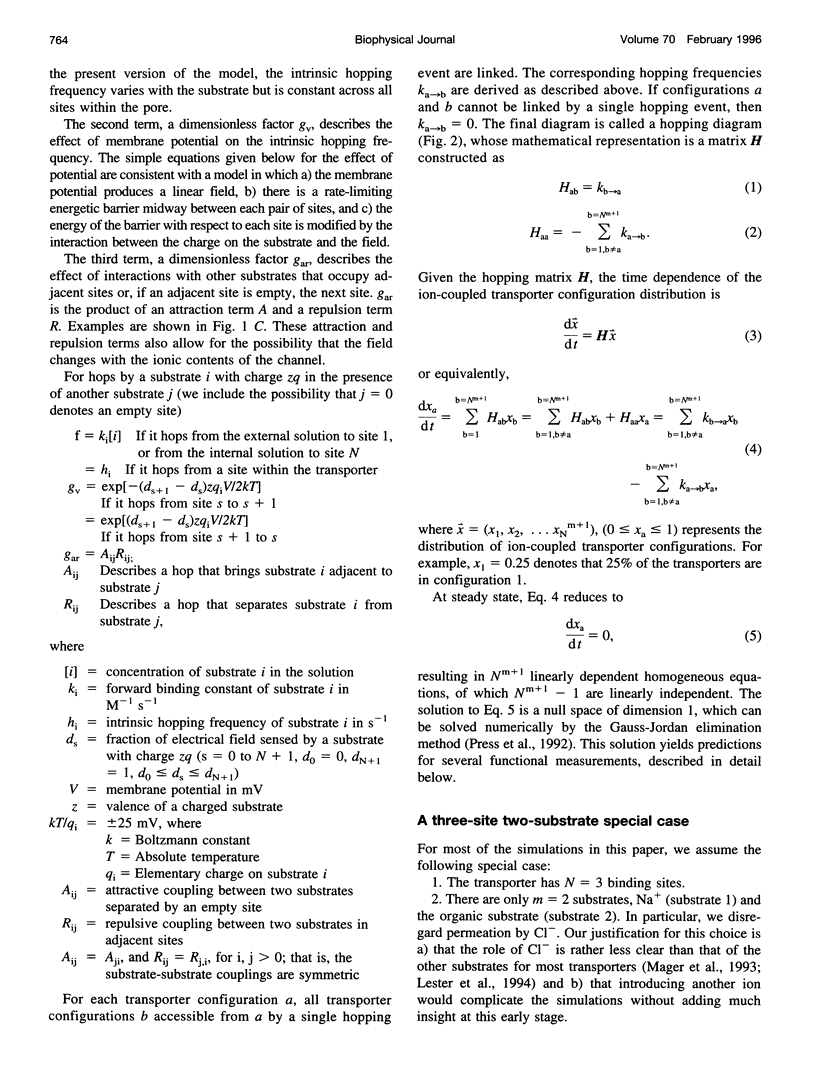
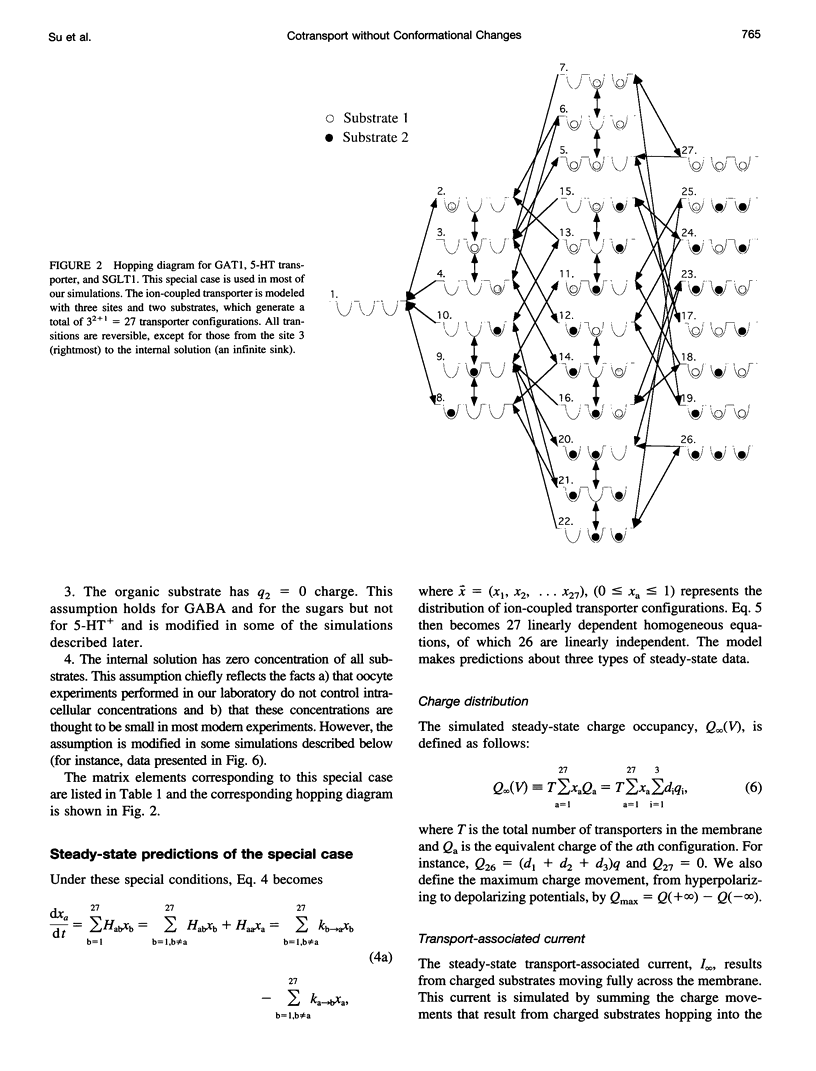
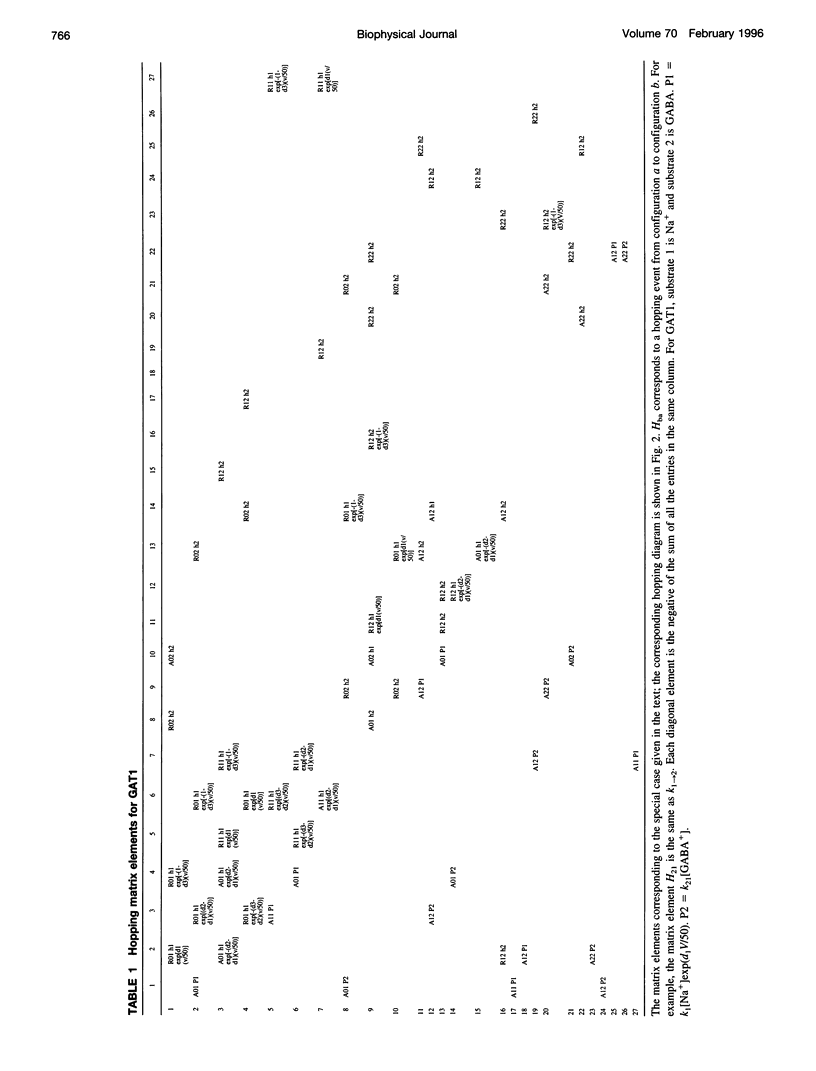
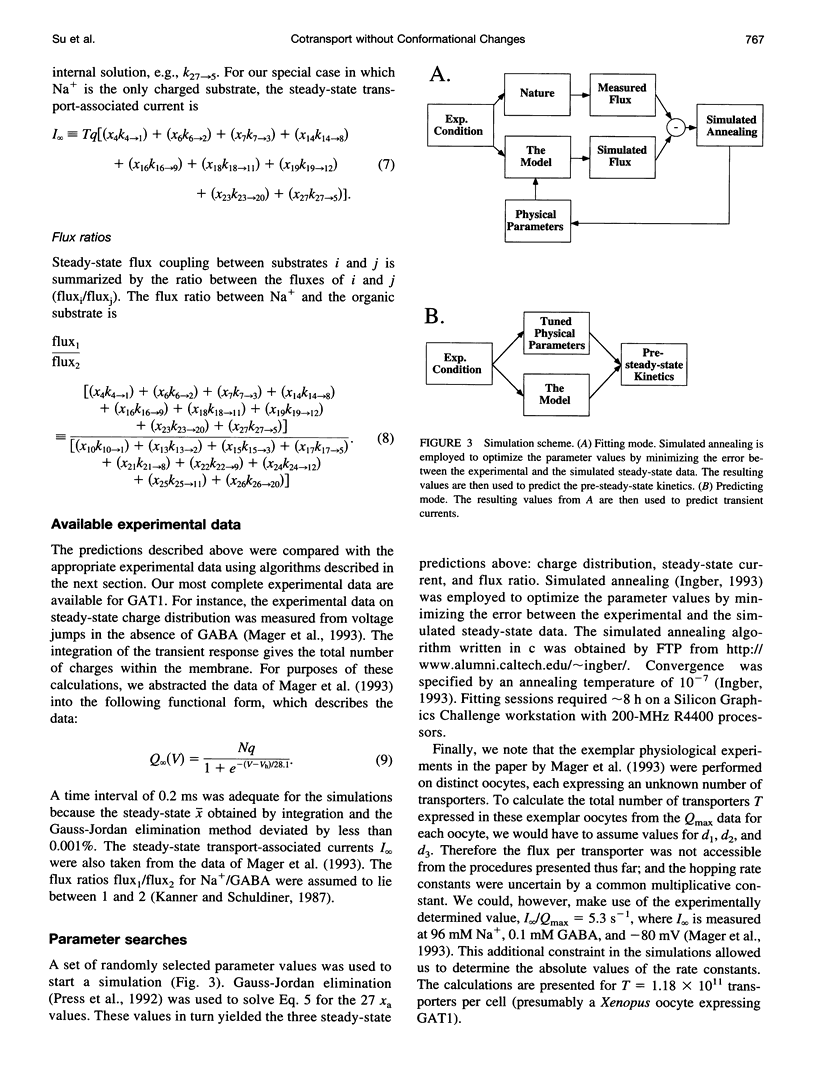
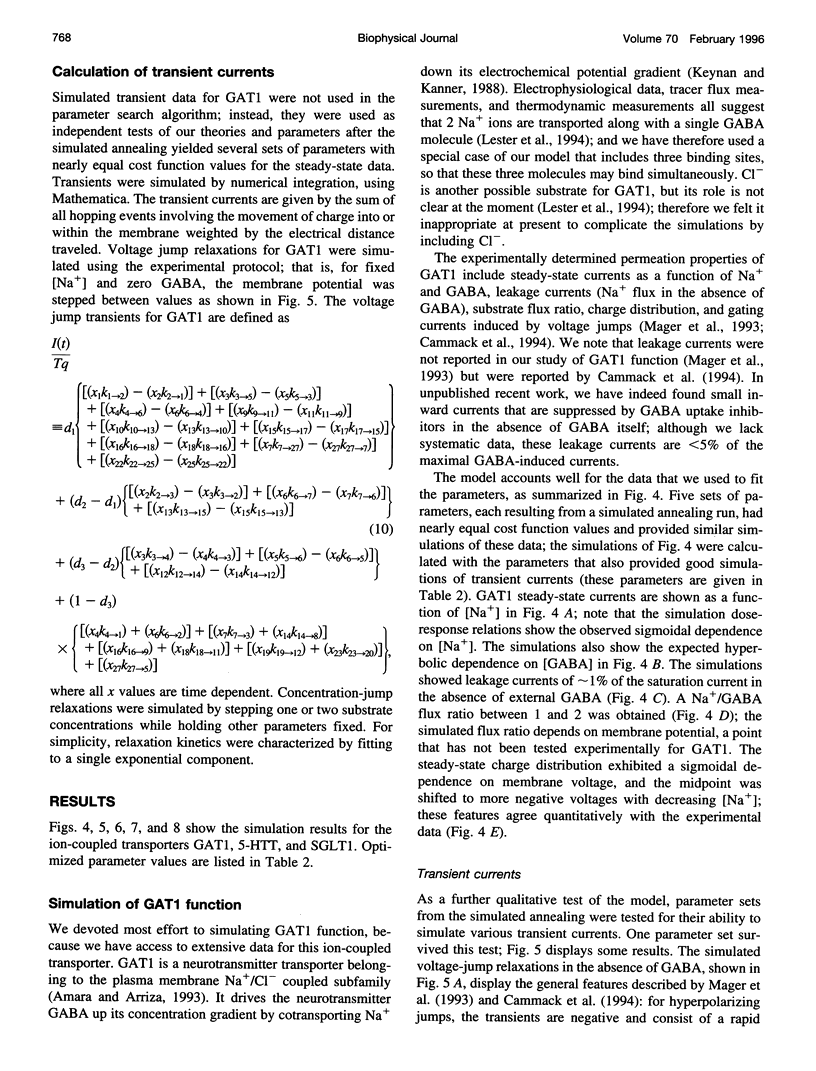
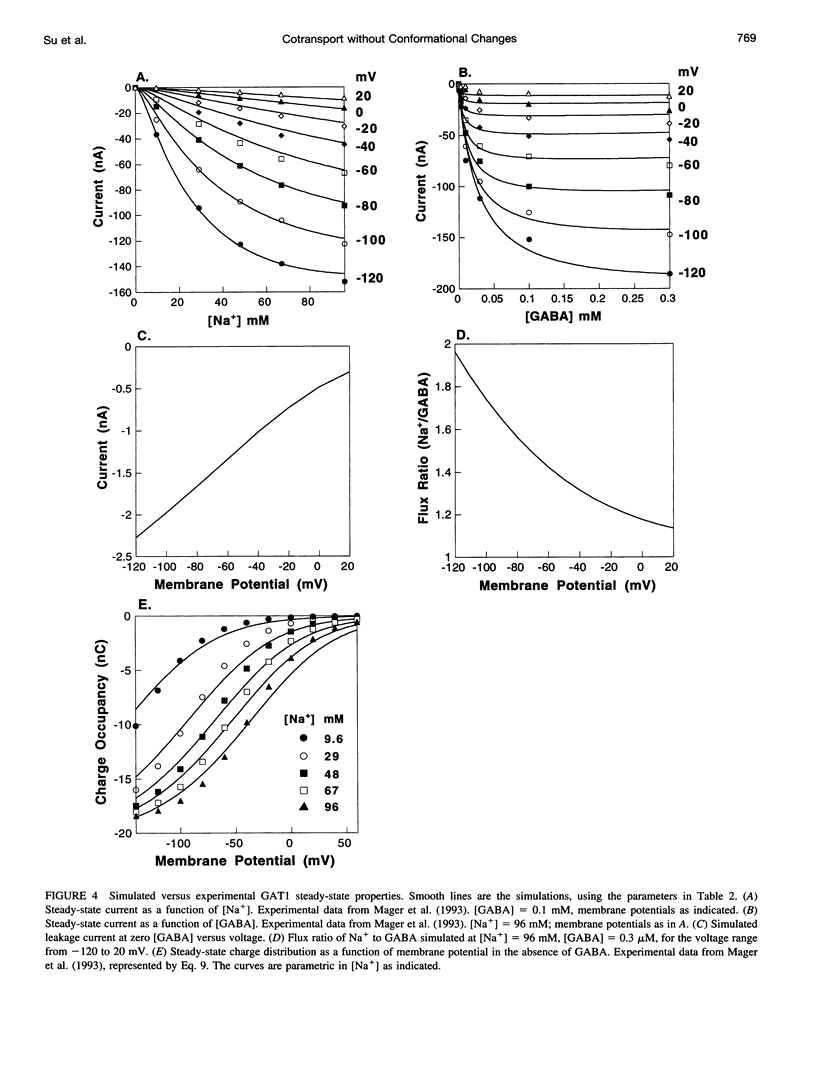
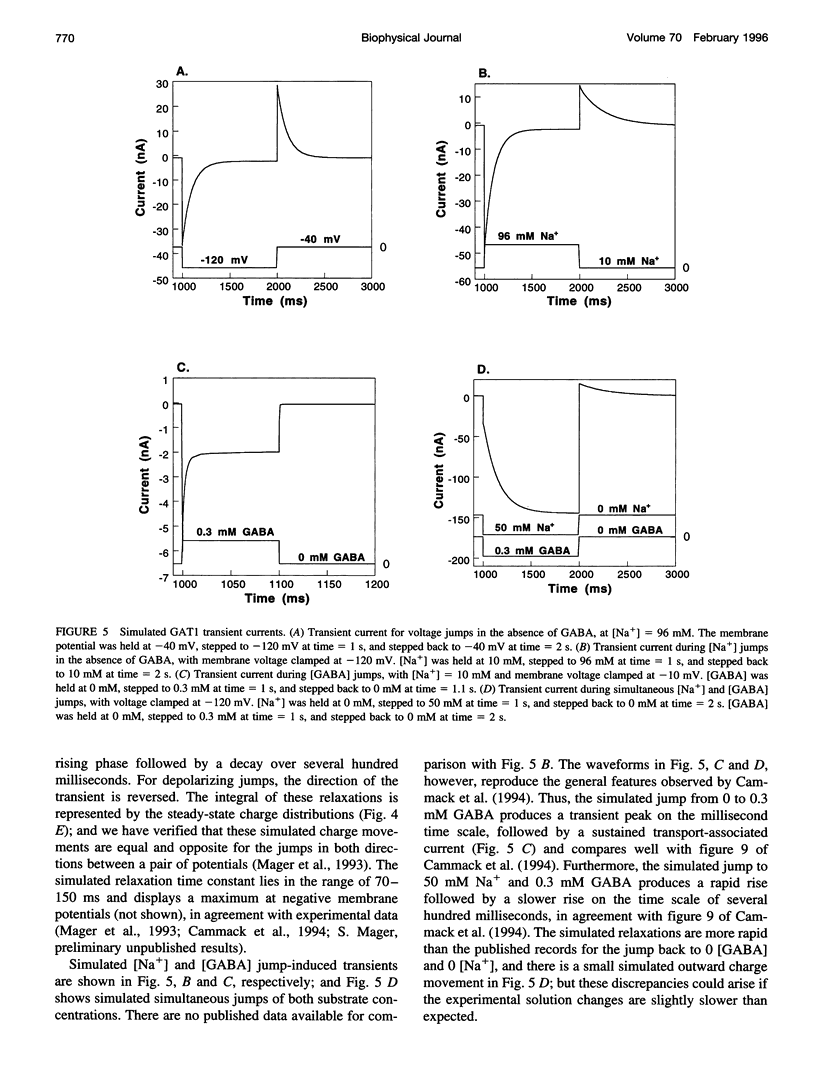
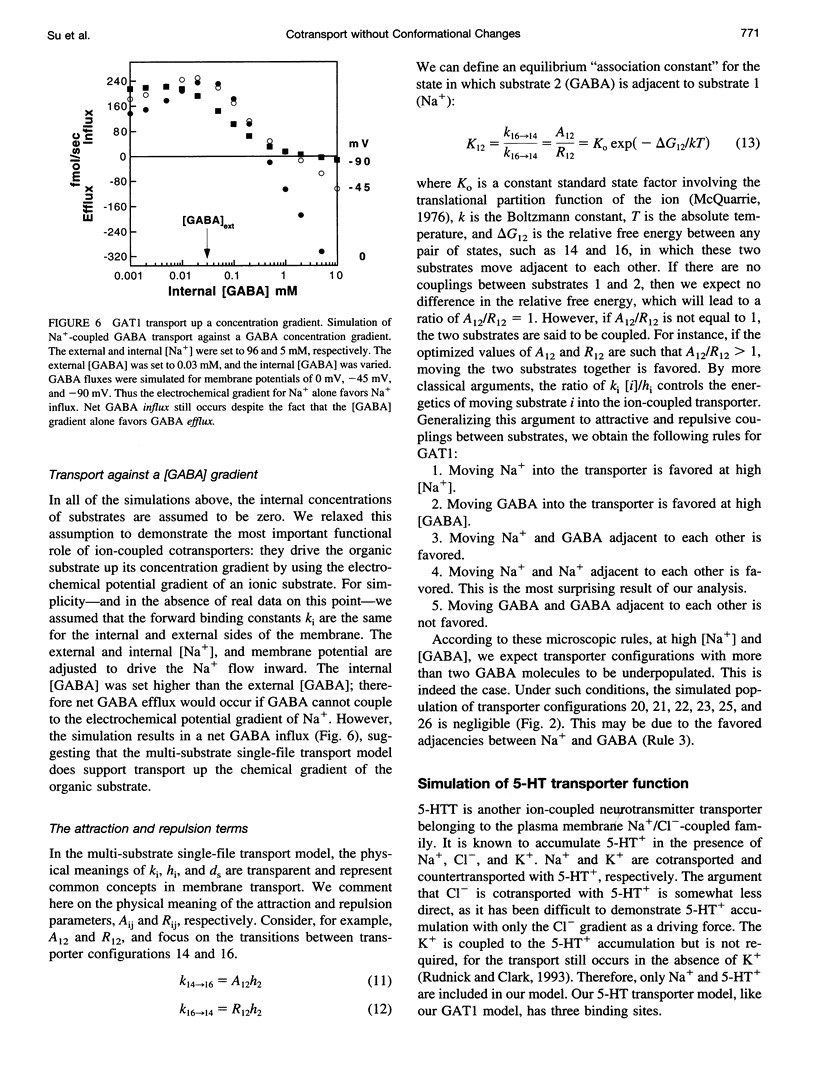
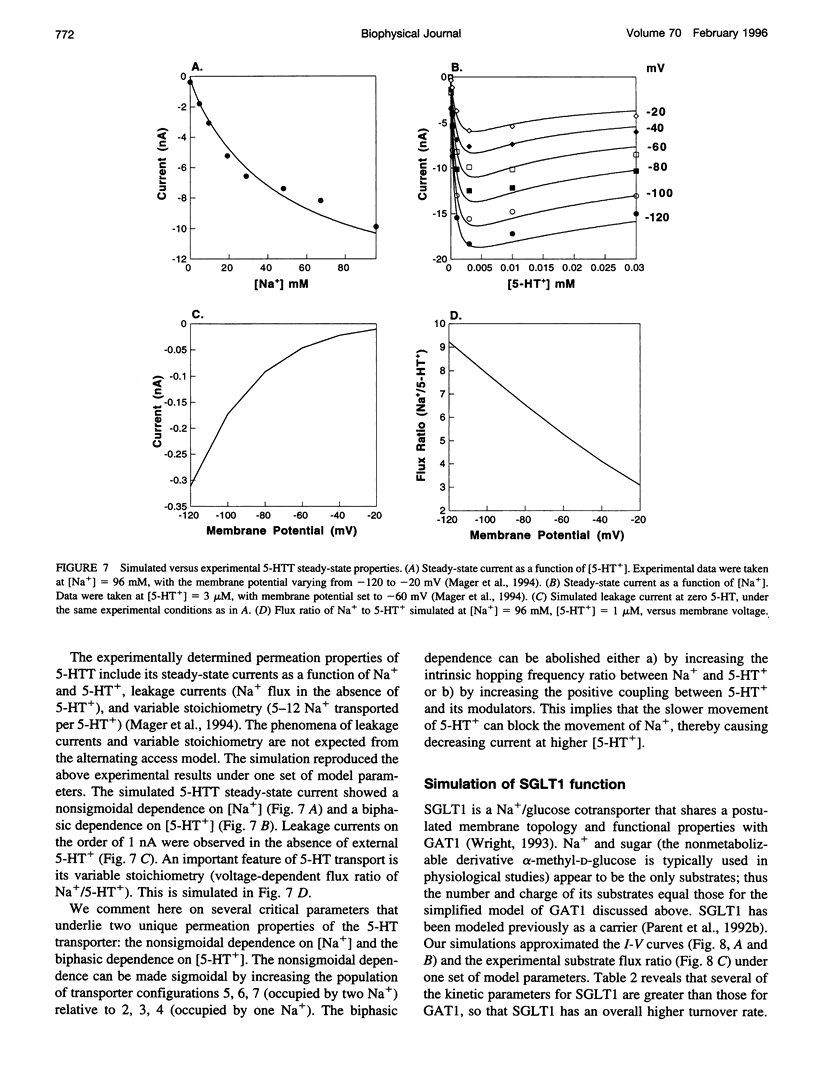
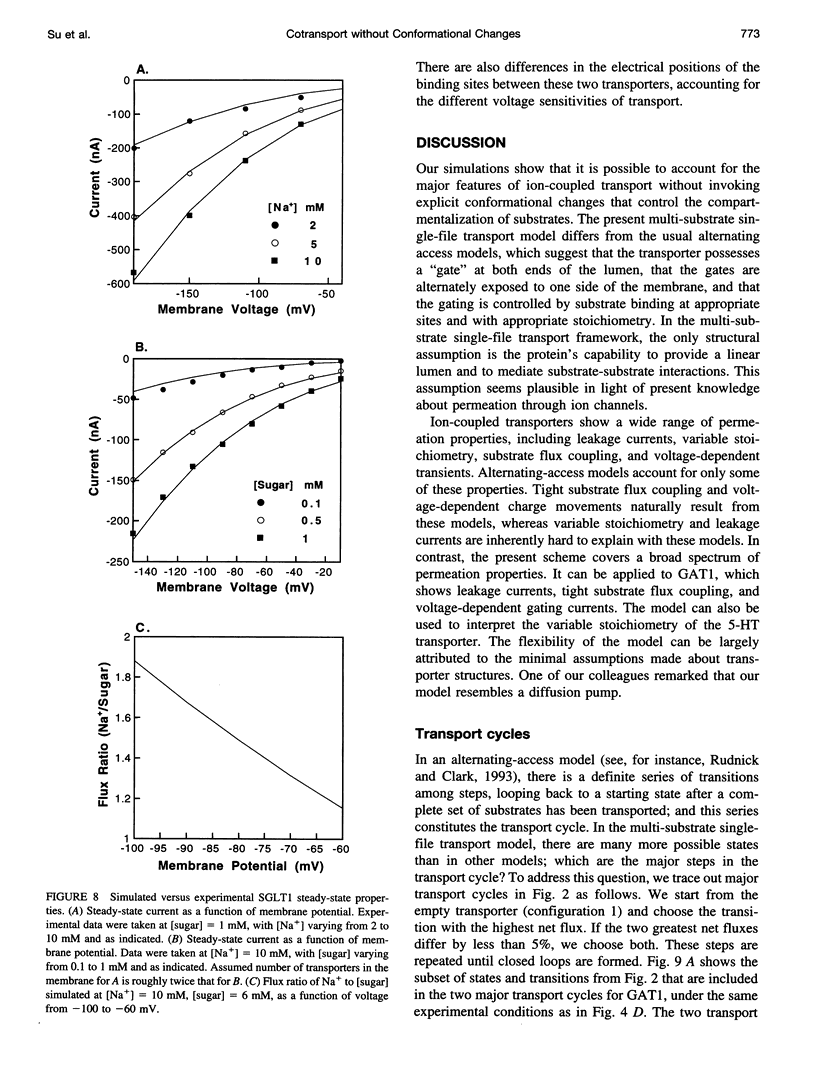
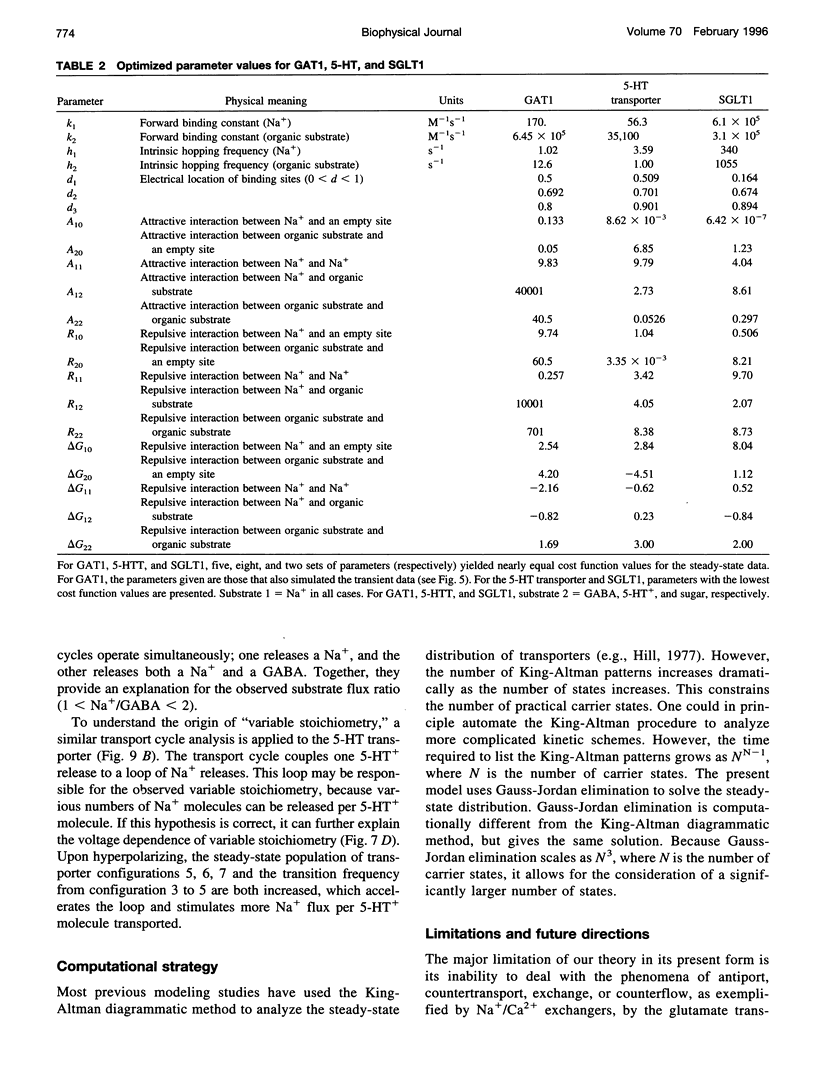
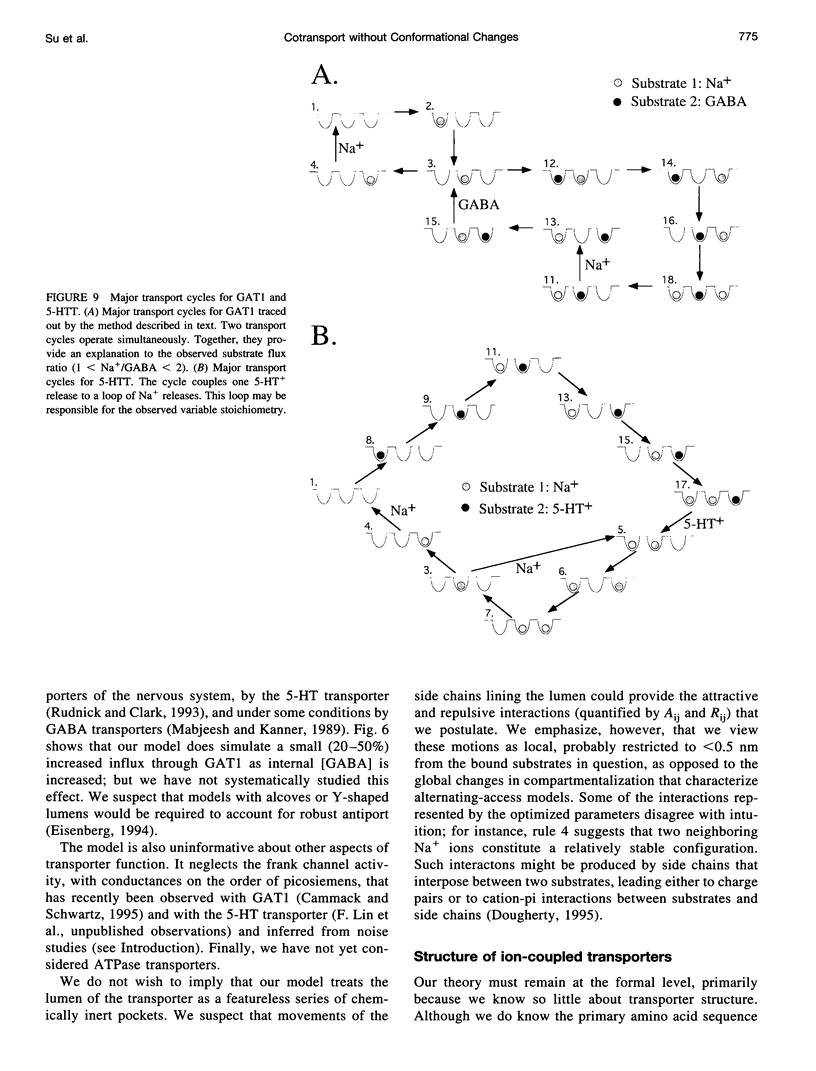
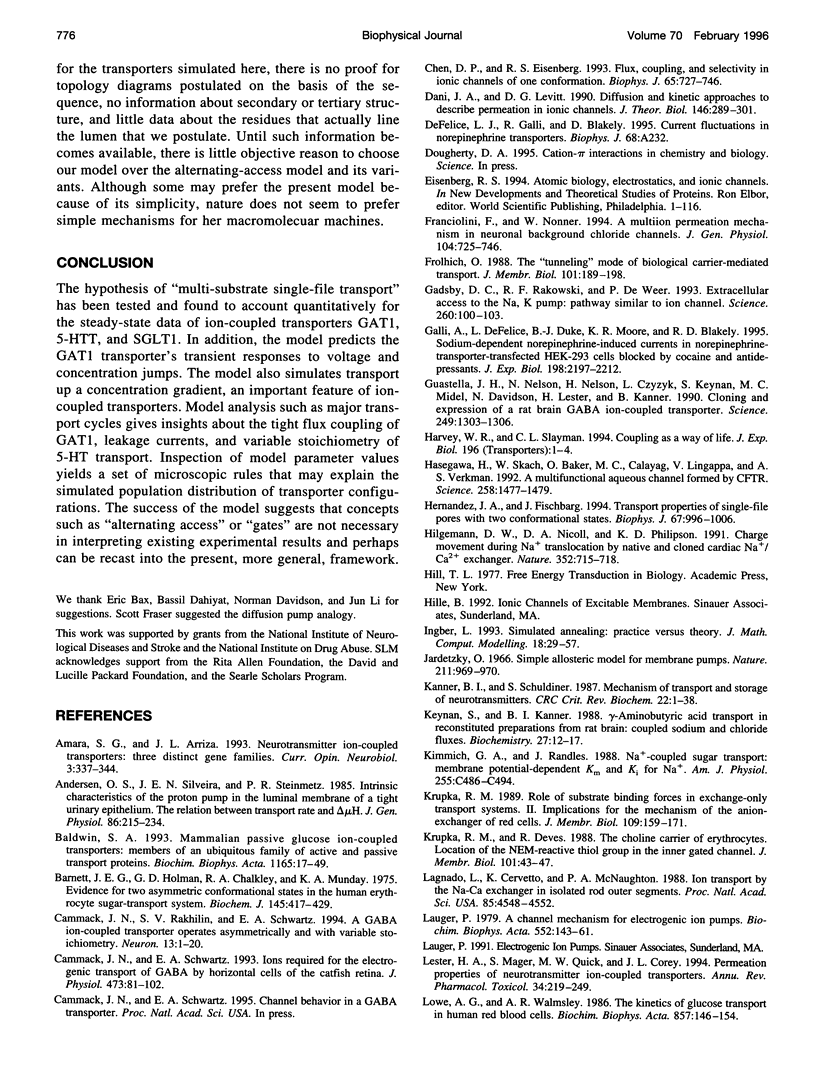
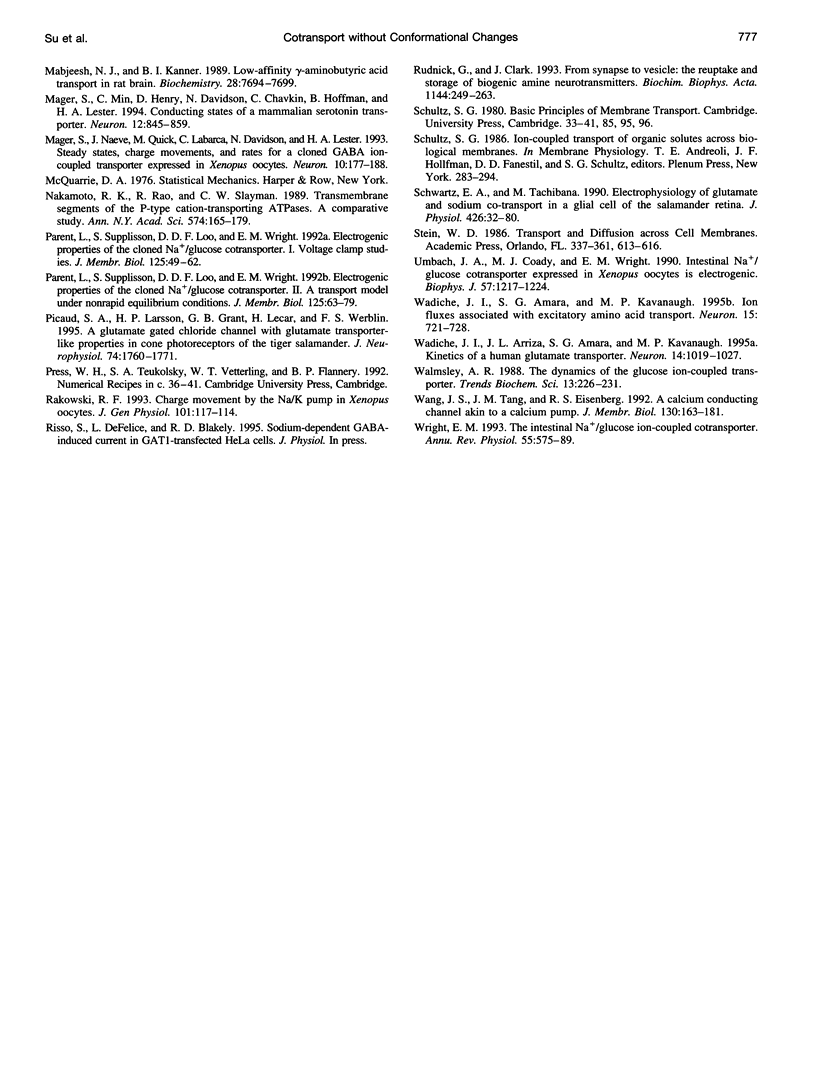
Images in this article
Selected References
These references are in PubMed. This may not be the complete list of references from this article.
- Amara S. G., Arriza J. L. Neurotransmitter transporters: three distinct gene families. Curr Opin Neurobiol. 1993 Jun;3(3):337–344. doi: 10.1016/0959-4388(93)90126-j. [DOI] [PubMed] [Google Scholar]
- Andersen O. S., Silveira J. E., Steinmetz P. R. Intrinsic characteristics of the proton pump in the luminal membrane of a tight urinary epithelium. The relation between transport rate and delta mu H. J Gen Physiol. 1985 Aug;86(2):215–234. doi: 10.1085/jgp.86.2.215. [DOI] [PMC free article] [PubMed] [Google Scholar]
- Baldwin S. A. Mammalian passive glucose transporters: members of an ubiquitous family of active and passive transport proteins. Biochim Biophys Acta. 1993 Jun 8;1154(1):17–49. doi: 10.1016/0304-4157(93)90015-g. [DOI] [PubMed] [Google Scholar]
- Barnett J. E., Holman G. D., Chalkley R. A., Munday K. A. Evidence for two asymmetric conformational states in the human erythrocyte sugar-transport system. Biochem J. 1975 Mar;145(3):417–429. doi: 10.1042/bj1450417a. [DOI] [PMC free article] [PubMed] [Google Scholar]
- Cammack J. N., Schwartz E. A. Ions required for the electrogenic transport of GABA by horizontal cells of the catfish retina. J Physiol. 1993 Dec;472:81–102. doi: 10.1113/jphysiol.1993.sp019938. [DOI] [PMC free article] [PubMed] [Google Scholar]
- Chen D. P., Eisenberg R. S. Flux, coupling, and selectivity in ionic channels of one conformation. Biophys J. 1993 Aug;65(2):727–746. doi: 10.1016/S0006-3495(93)81099-3. [DOI] [PMC free article] [PubMed] [Google Scholar]
- Dani J. A., Levitt D. G. Diffusion and kinetic approaches to describe permeation in ionic channels. J Theor Biol. 1990 Oct 7;146(3):289–301. doi: 10.1016/s0022-5193(05)80740-4. [DOI] [PubMed] [Google Scholar]
- Franciolini F., Nonner W. A multi-ion permeation mechanism in neuronal background chloride channels. J Gen Physiol. 1994 Oct;104(4):725–746. doi: 10.1085/jgp.104.4.725. [DOI] [PMC free article] [PubMed] [Google Scholar]
- Fröhlich O. The "tunneling" mode of biological carrier-mediated transport. J Membr Biol. 1988 Mar;101(3):189–198. doi: 10.1007/BF01872834. [DOI] [PubMed] [Google Scholar]
- Gadsby D. C., Rakowski R. F., De Weer P. Extracellular access to the Na,K pump: pathway similar to ion channel. Science. 1993 Apr 2;260(5104):100–103. doi: 10.1126/science.7682009. [DOI] [PubMed] [Google Scholar]
- Galli A., DeFelice L. J., Duke B. J., Moore K. R., Blakely R. D. Sodium-dependent norepinephrine-induced currents in norepinephrine-transporter-transfected HEK-293 cells blocked by cocaine and antidepressants. J Exp Biol. 1995 Oct;198(Pt 10):2197–2212. doi: 10.1242/jeb.198.10.2197. [DOI] [PubMed] [Google Scholar]
- Guastella J., Nelson N., Nelson H., Czyzyk L., Keynan S., Miedel M. C., Davidson N., Lester H. A., Kanner B. I. Cloning and expression of a rat brain GABA transporter. Science. 1990 Sep 14;249(4974):1303–1306. doi: 10.1126/science.1975955. [DOI] [PubMed] [Google Scholar]
- Harvey W. R., Slayman C. L. Coupling as a way of life. J Exp Biol. 1994 Nov;196:1–4. doi: 10.1242/jeb.196.1.1. [DOI] [PubMed] [Google Scholar]
- Hasegawa H., Skach W., Baker O., Calayag M. C., Lingappa V., Verkman A. S. A multifunctional aqueous channel formed by CFTR. Science. 1992 Nov 27;258(5087):1477–1479. doi: 10.1126/science.1279809. [DOI] [PubMed] [Google Scholar]
- Hernández J. A., Fischbarg J. Transport properties of single-file pores with two conformational states. Biophys J. 1994 Sep;67(3):996–1006. doi: 10.1016/S0006-3495(94)80565-X. [DOI] [PMC free article] [PubMed] [Google Scholar]
- Hilgemann D. W., Nicoll D. A., Philipson K. D. Charge movement during Na+ translocation by native and cloned cardiac Na+/Ca2+ exchanger. Nature. 1991 Aug 22;352(6337):715–718. doi: 10.1038/352715a0. [DOI] [PubMed] [Google Scholar]
- Jardetzky O. Simple allosteric model for membrane pumps. Nature. 1966 Aug 27;211(5052):969–970. doi: 10.1038/211969a0. [DOI] [PubMed] [Google Scholar]
- Kanner B. I., Schuldiner S. Mechanism of transport and storage of neurotransmitters. CRC Crit Rev Biochem. 1987;22(1):1–38. doi: 10.3109/10409238709082546. [DOI] [PubMed] [Google Scholar]
- Keynan S., Kanner B. I. gamma-Aminobutyric acid transport in reconstituted preparations from rat brain: coupled sodium and chloride fluxes. Biochemistry. 1988 Jan 12;27(1):12–17. doi: 10.1021/bi00401a003. [DOI] [PubMed] [Google Scholar]
- Kimmich G. A., Randles J. Na+-coupled sugar transport: membrane potential-dependent Km and Ki for Na+. Am J Physiol. 1988 Oct;255(4 Pt 1):C486–C494. doi: 10.1152/ajpcell.1988.255.4.C486. [DOI] [PubMed] [Google Scholar]
- Krupka R. M., Devés R. The choline carrier of erythrocytes: location of the NEM-reactive thiol group in the inner gated channel. J Membr Biol. 1988;101(1):43–47. doi: 10.1007/BF01872818. [DOI] [PubMed] [Google Scholar]
- Krupka R. M. Role of substrate binding forces in exchange-only transport systems: II. Implications for the mechanism of the anion exchanger of red cells. J Membr Biol. 1989 Jul;109(2):159–171. doi: 10.1007/BF01870855. [DOI] [PubMed] [Google Scholar]
- Lagnado L., Cervetto L., McNaughton P. A. Ion transport by the Na-Ca exchange in isolated rod outer segments. Proc Natl Acad Sci U S A. 1988 Jun;85(12):4548–4552. doi: 10.1073/pnas.85.12.4548. [DOI] [PMC free article] [PubMed] [Google Scholar]
- Lester H. A., Mager S., Quick M. W., Corey J. L. Permeation properties of neurotransmitter transporters. Annu Rev Pharmacol Toxicol. 1994;34:219–249. doi: 10.1146/annurev.pa.34.040194.001251. [DOI] [PubMed] [Google Scholar]
- Lowe A. G., Walmsley A. R. The kinetics of glucose transport in human red blood cells. Biochim Biophys Acta. 1986 May 28;857(2):146–154. doi: 10.1016/0005-2736(86)90342-1. [DOI] [PubMed] [Google Scholar]
- Läuger P. A channel mechanism for electrogenic ion pumps. Biochim Biophys Acta. 1979 Mar 23;552(1):143–161. doi: 10.1016/0005-2736(79)90253-0. [DOI] [PubMed] [Google Scholar]
- Mabjeesh N. J., Kanner B. I. Low-affinity gamma-aminobutyric acid transport in rat brain. Biochemistry. 1989 Sep 19;28(19):7694–7699. doi: 10.1021/bi00445a026. [DOI] [PubMed] [Google Scholar]
- Mager S., Min C., Henry D. J., Chavkin C., Hoffman B. J., Davidson N., Lester H. A. Conducting states of a mammalian serotonin transporter. Neuron. 1994 Apr;12(4):845–859. doi: 10.1016/0896-6273(94)90337-9. [DOI] [PubMed] [Google Scholar]
- Mager S., Naeve J., Quick M., Labarca C., Davidson N., Lester H. A. Steady states, charge movements, and rates for a cloned GABA transporter expressed in Xenopus oocytes. Neuron. 1993 Feb;10(2):177–188. doi: 10.1016/0896-6273(93)90309-f. [DOI] [PubMed] [Google Scholar]
- Nakamoto R. K., Rao R., Slayman C. W. Transmembrane segments of the P-type cation-transporting ATPases. A comparative study. Ann N Y Acad Sci. 1989;574:165–179. doi: 10.1111/j.1749-6632.1989.tb25155.x. [DOI] [PubMed] [Google Scholar]
- Parent L., Supplisson S., Loo D. D., Wright E. M. Electrogenic properties of the cloned Na+/glucose cotransporter: I. Voltage-clamp studies. J Membr Biol. 1992 Jan;125(1):49–62. doi: 10.1007/BF00235797. [DOI] [PubMed] [Google Scholar]
- Parent L., Supplisson S., Loo D. D., Wright E. M. Electrogenic properties of the cloned Na+/glucose cotransporter: II. A transport model under nonrapid equilibrium conditions. J Membr Biol. 1992 Jan;125(1):63–79. doi: 10.1007/BF00235798. [DOI] [PubMed] [Google Scholar]
- Picaud S. A., Larsson H. P., Grant G. B., Lecar H., Werblin F. S. Glutamate-gated chloride channel with glutamate-transporter-like properties in cone photoreceptors of the tiger salamander. J Neurophysiol. 1995 Oct;74(4):1760–1771. doi: 10.1152/jn.1995.74.4.1760. [DOI] [PubMed] [Google Scholar]
- Rakowski R. F. Charge movement by the Na/K pump in Xenopus oocytes. J Gen Physiol. 1993 Jan;101(1):117–144. doi: 10.1085/jgp.101.1.117. [DOI] [PMC free article] [PubMed] [Google Scholar]
- Rudnick G., Clark J. From synapse to vesicle: the reuptake and storage of biogenic amine neurotransmitters. Biochim Biophys Acta. 1993 Oct 4;1144(3):249–263. doi: 10.1016/0005-2728(93)90109-s. [DOI] [PubMed] [Google Scholar]
- Schwartz E. A., Tachibana M. Electrophysiology of glutamate and sodium co-transport in a glial cell of the salamander retina. J Physiol. 1990 Jul;426:43–80. doi: 10.1113/jphysiol.1990.sp018126. [DOI] [PMC free article] [PubMed] [Google Scholar]
- Umbach J. A., Coady M. J., Wright E. M. Intestinal Na+/glucose cotransporter expressed in Xenopus oocytes is electrogenic. Biophys J. 1990 Jun;57(6):1217–1224. doi: 10.1016/S0006-3495(90)82640-0. [DOI] [PMC free article] [PubMed] [Google Scholar]
- Van Essen D. C., Gallant J. L. Neural mechanisms of form and motion processing in the primate visual system. Neuron. 1994 Jul;13(1):1–10. doi: 10.1016/0896-6273(94)90455-3. [DOI] [PubMed] [Google Scholar]
- Wadiche J. I., Amara S. G., Kavanaugh M. P. Ion fluxes associated with excitatory amino acid transport. Neuron. 1995 Sep;15(3):721–728. doi: 10.1016/0896-6273(95)90159-0. [DOI] [PubMed] [Google Scholar]
- Wadiche J. I., Arriza J. L., Amara S. G., Kavanaugh M. P. Kinetics of a human glutamate transporter. Neuron. 1995 May;14(5):1019–1027. doi: 10.1016/0896-6273(95)90340-2. [DOI] [PubMed] [Google Scholar]
- Walmsley A. R. The dynamics of the glucose transporter. Trends Biochem Sci. 1988 Jun;13(6):226–231. doi: 10.1016/0968-0004(88)90089-8. [DOI] [PubMed] [Google Scholar]
- Wang J., Tang J. M., Eisenberg R. S. A calcium conducting channel akin to a calcium pump. J Membr Biol. 1992 Nov;130(2):163–181. doi: 10.1007/BF00231894. [DOI] [PubMed] [Google Scholar]
- Wright E. M. The intestinal Na+/glucose cotransporter. Annu Rev Physiol. 1993;55:575–589. doi: 10.1146/annurev.ph.55.030193.003043. [DOI] [PubMed] [Google Scholar]