Abstract
Spontaneous calcium waves in enzymatically isolated rat cardiac myocytes were investigated by confocal laser scanning microscopy (CLSM) using the fluorescent Ca2+-indicator fluo-3 AM. As recently shown, a spreading wave of enhanced cytosolic calcium appears, most probably during Ca2+ overload, and is initiated by an elementary event called a "calcium spark." When measured by conventional fluorescence microscopy the propagation velocity of spontaneous calcium waves determined at several points along the cardiac myocyte was previously found to be constant. More precise measurements with a CLSM showed a nonlinear propagation. The wave velocity was low, close to the focus, and increased with increasing time and propagation length, approaching a maximum of 113 microns/s. This result was surprising, inasmuch as for geometrical reasons a decrease of the propagation velocity might be expected if the confocal plane is not identical with that plane where the focus of the wave was localized. It is suggested that the propagation velocity is essentially dependent on the curvature of the spreading wave. From the linear relationship of velocity versus curvature, a critical radius of 2.7 +/- 1.4 microns (mean +/- SD) was worked out, below which an outward propagation of the wave will not take place. Once released from a sufficiently extended cluster of sarcoplasmic reticulum release channels, calcium diffuses and will activate its neighbors. While traveling away, the volume into which calcium diffuses becomes effectively smaller than at low radii. This effect is the consequence of the summation of elementary events (Ca2+ sparks) and leads to a steeper increase of the cytosolic calcium concentration after a certain diffusion path length. Thus the time taken to reach a critical threshold of [Ca2+]i at the neighboring calcium release sites decreases with decreasing curvature and the wave will propagate faster.
Full text
PDF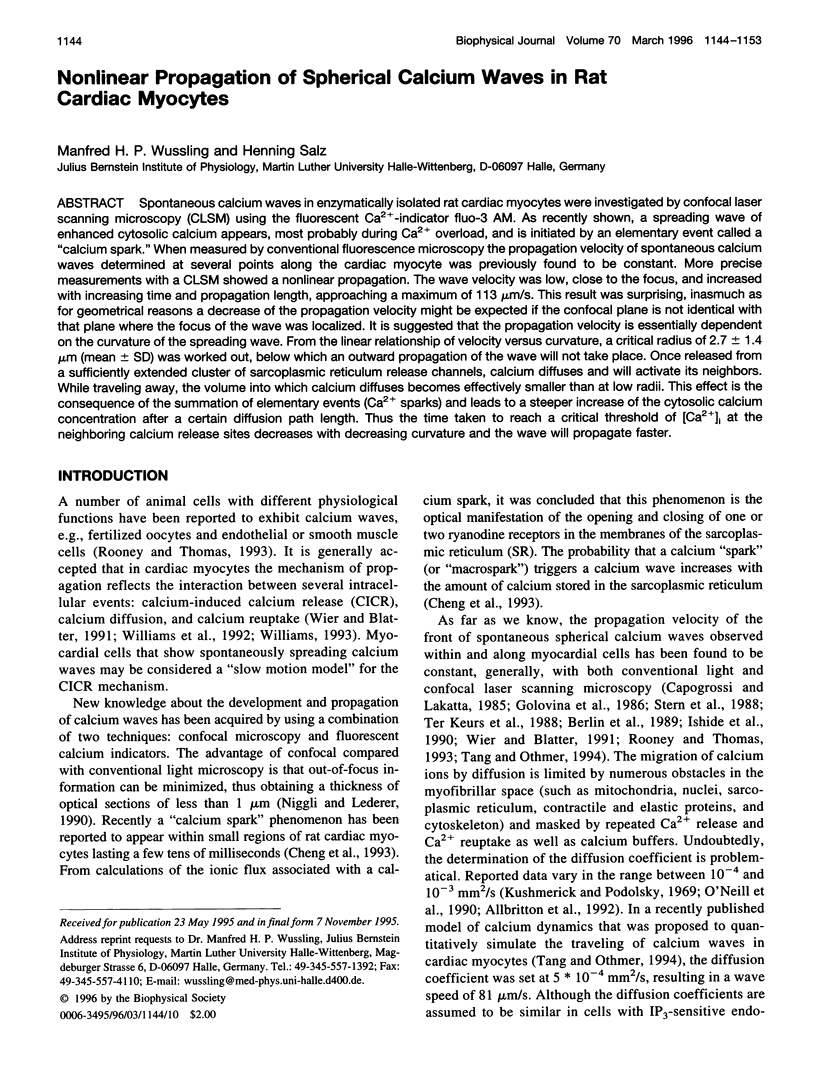
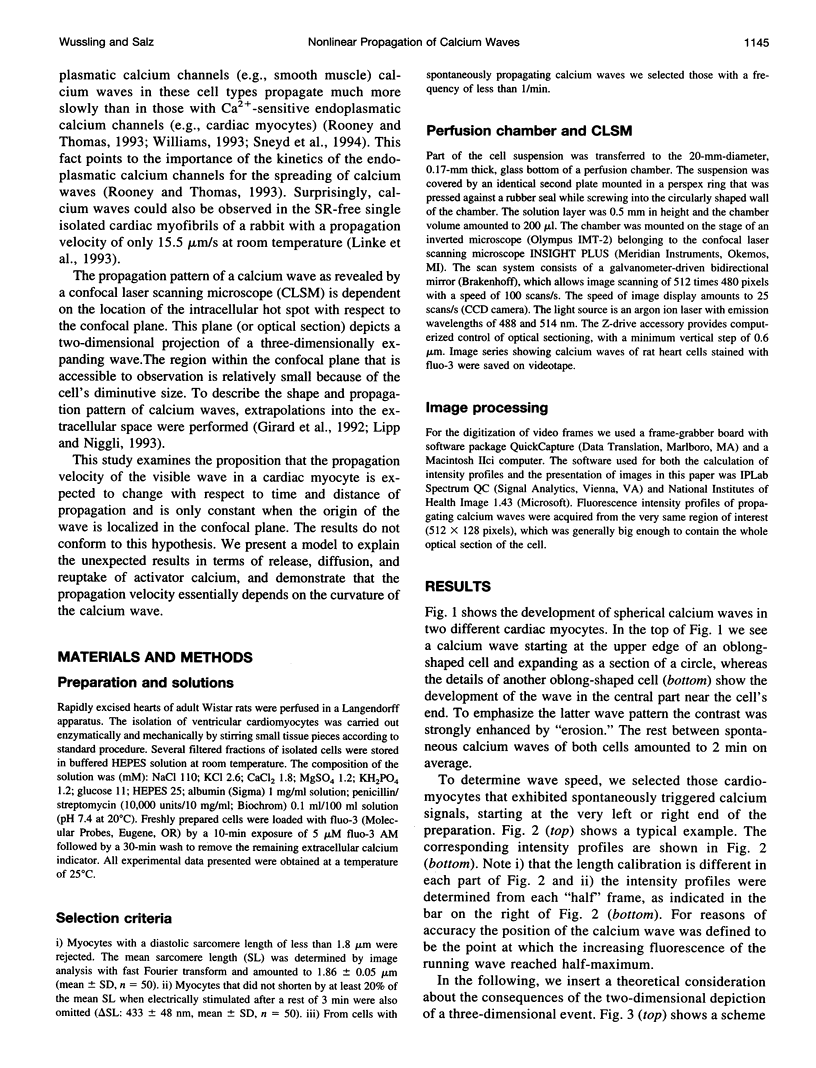
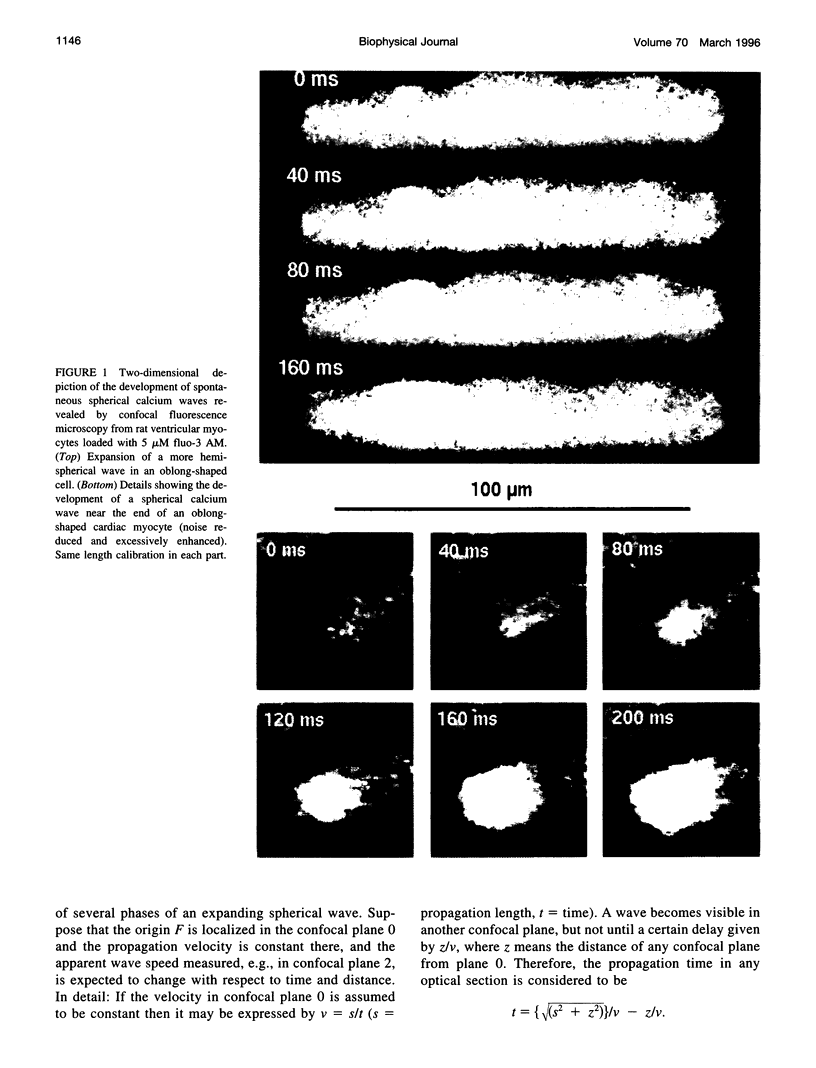
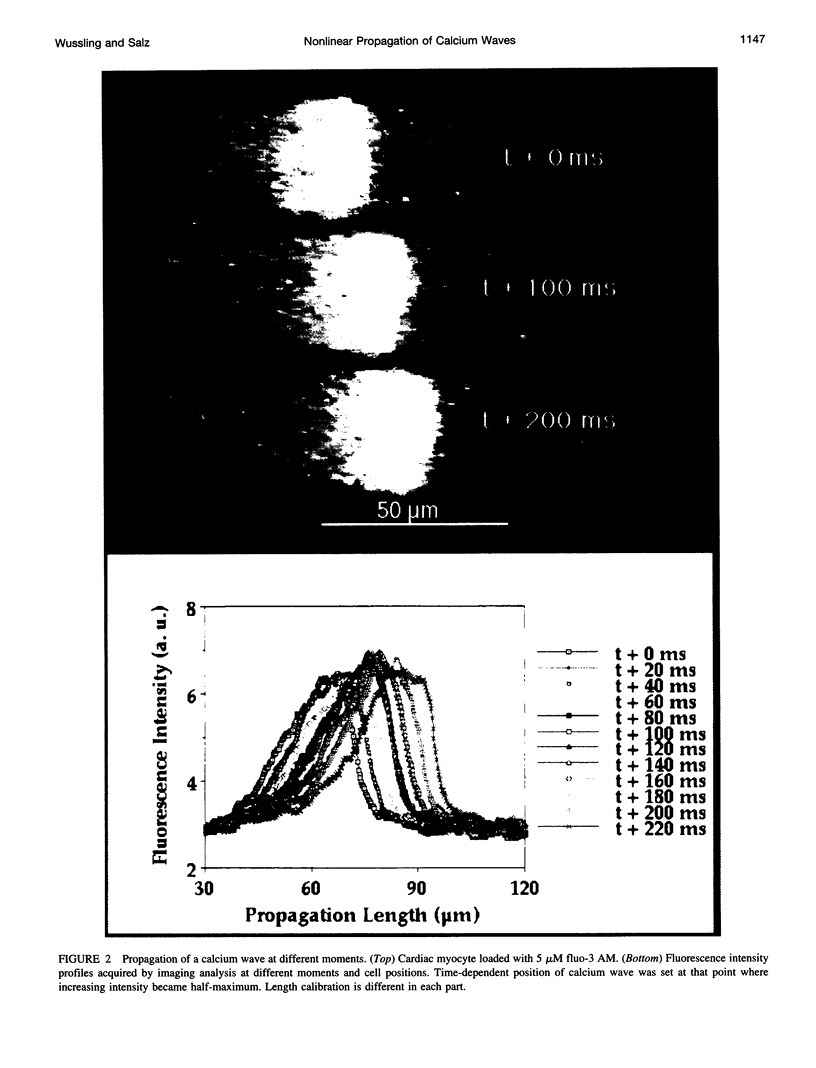
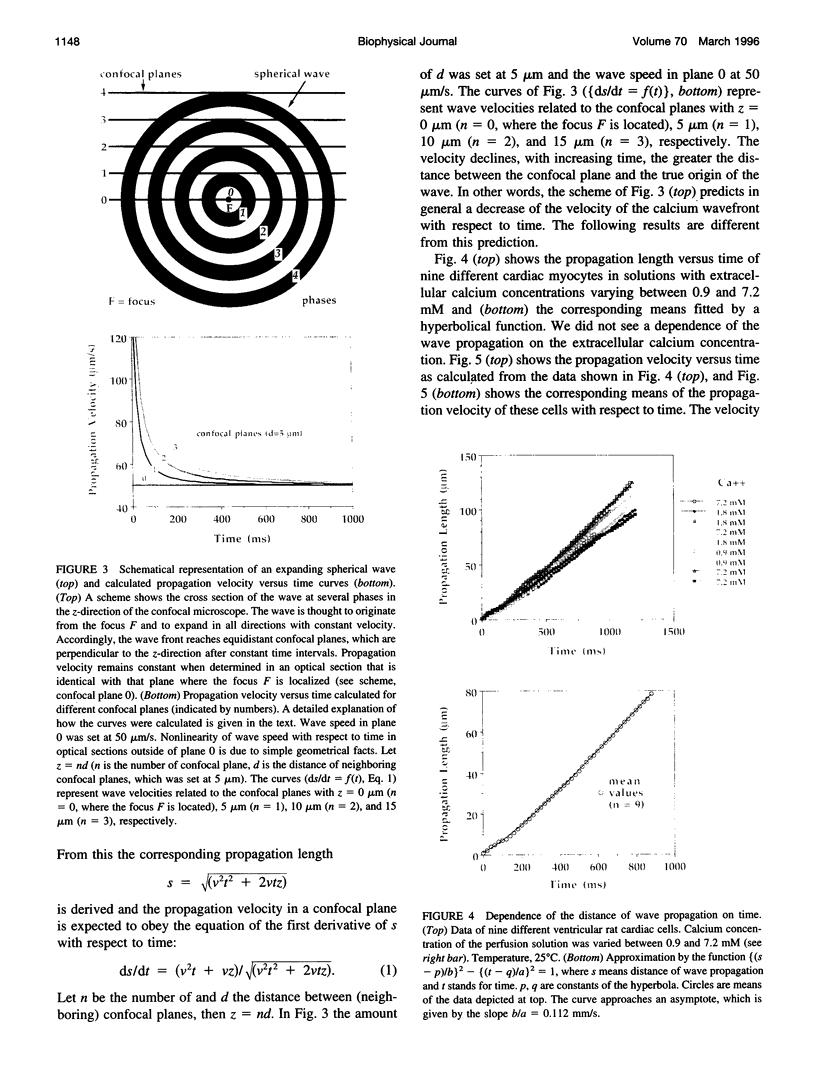
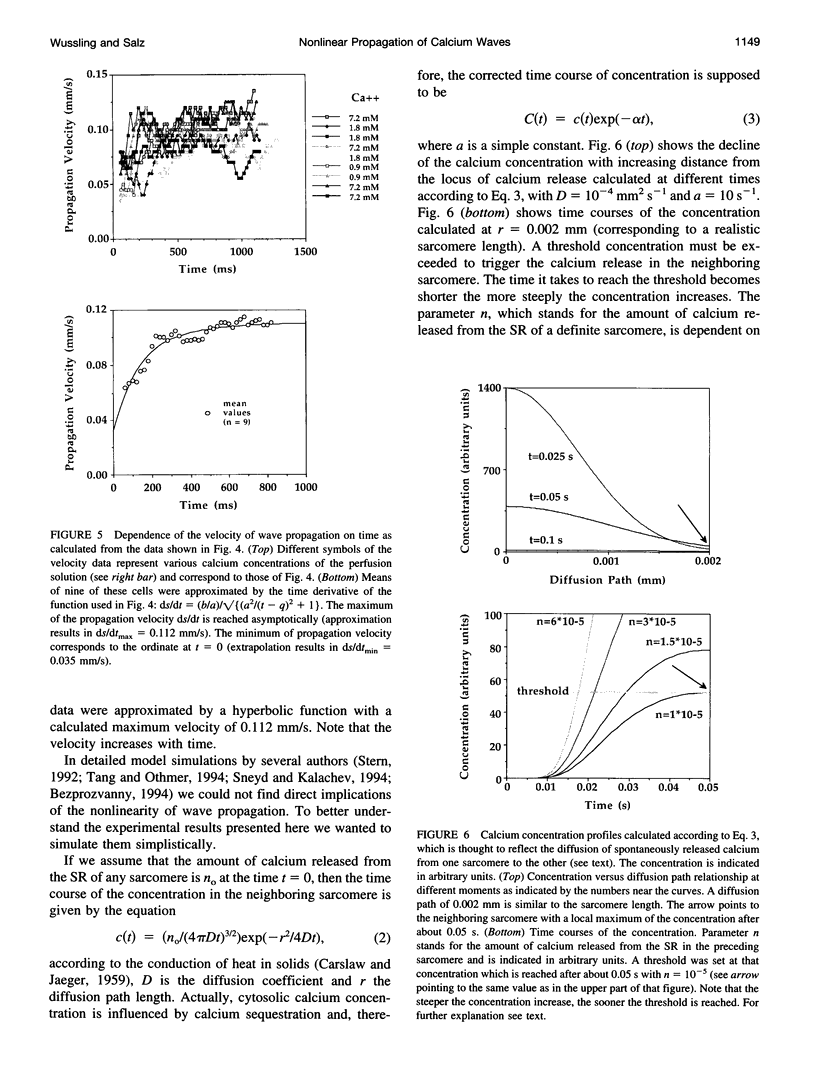
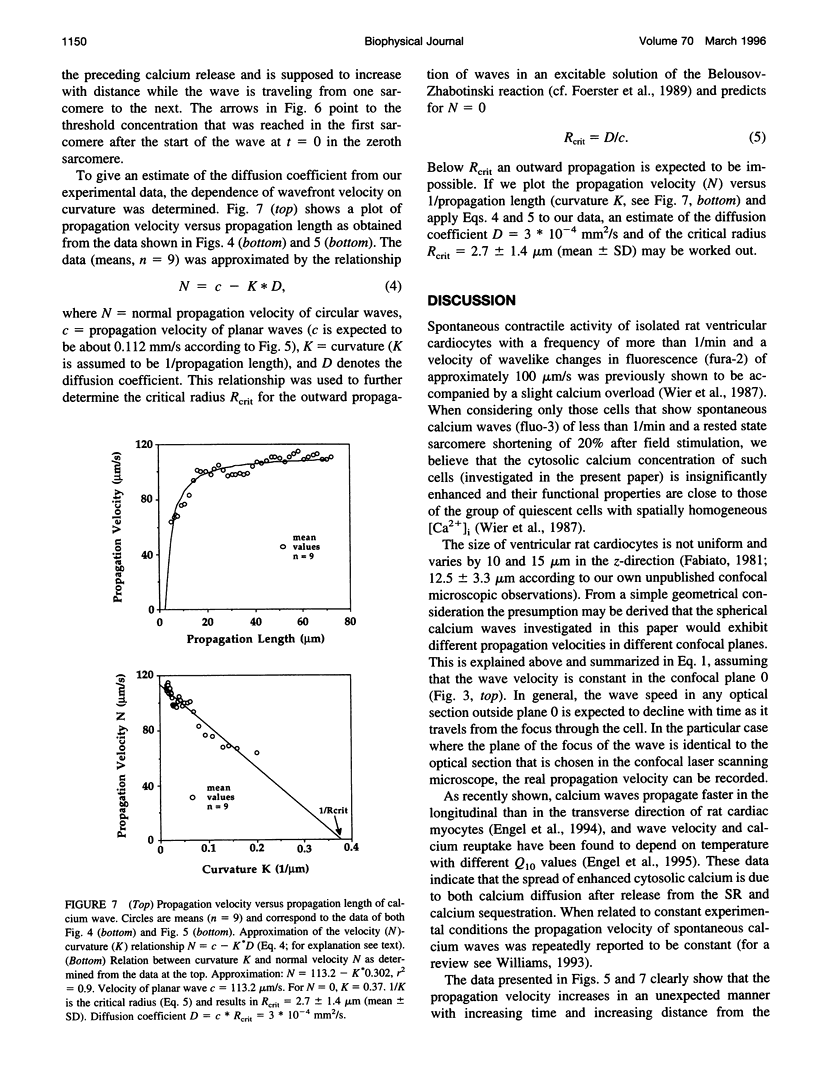
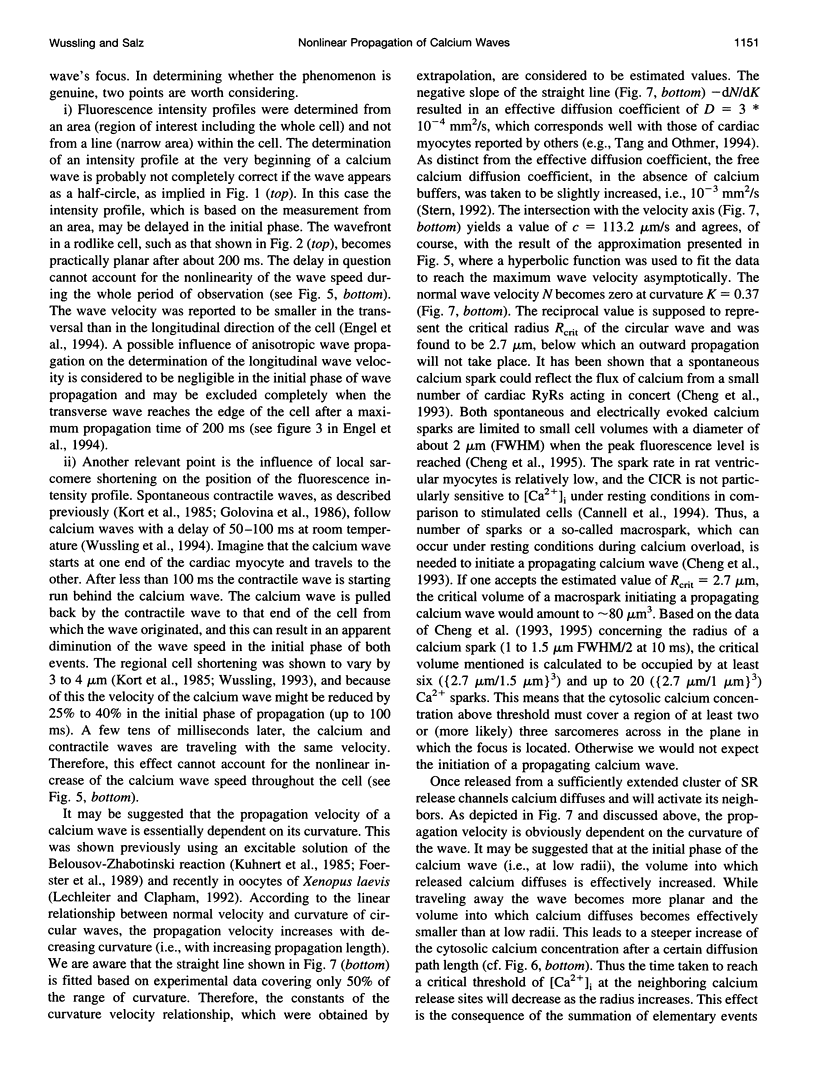
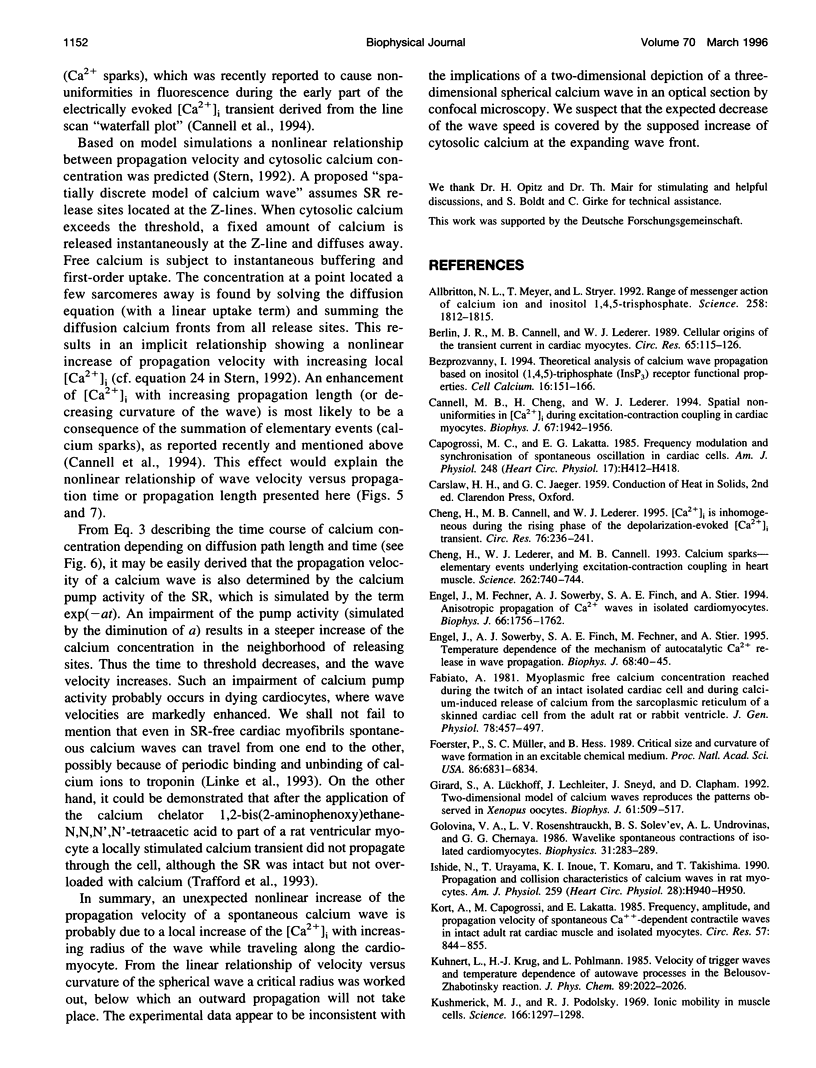
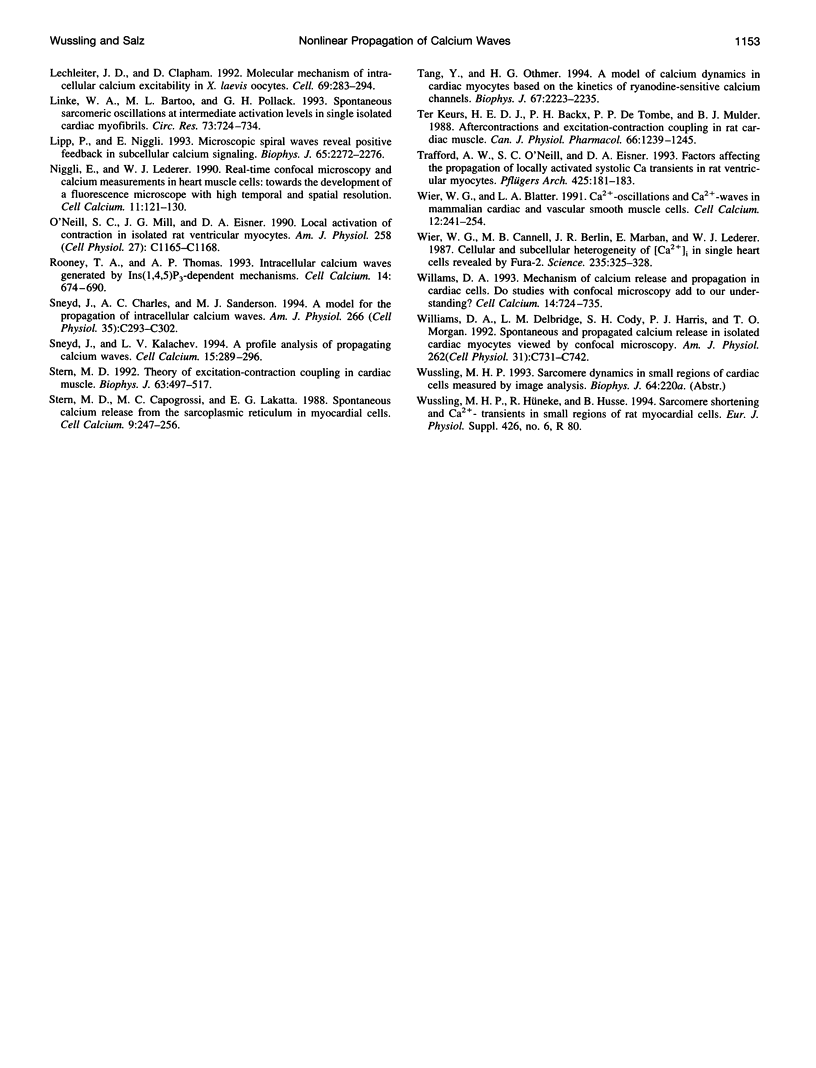
Images in this article
Selected References
These references are in PubMed. This may not be the complete list of references from this article.
- Allbritton N. L., Meyer T., Stryer L. Range of messenger action of calcium ion and inositol 1,4,5-trisphosphate. Science. 1992 Dec 11;258(5089):1812–1815. doi: 10.1126/science.1465619. [DOI] [PubMed] [Google Scholar]
- Berlin J. R., Cannell M. B., Lederer W. J. Cellular origins of the transient inward current in cardiac myocytes. Role of fluctuations and waves of elevated intracellular calcium. Circ Res. 1989 Jul;65(1):115–126. doi: 10.1161/01.res.65.1.115. [DOI] [PubMed] [Google Scholar]
- Bezprozvanny I. Theoretical analysis of calcium wave propagation based on inositol (1,4,5)-trisphosphate (InsP3) receptor functional properties. Cell Calcium. 1994 Sep;16(3):151–166. doi: 10.1016/0143-4160(94)90019-1. [DOI] [PubMed] [Google Scholar]
- Cannell M. B., Cheng H., Lederer W. J. Spatial non-uniformities in [Ca2+]i during excitation-contraction coupling in cardiac myocytes. Biophys J. 1994 Nov;67(5):1942–1956. doi: 10.1016/S0006-3495(94)80677-0. [DOI] [PMC free article] [PubMed] [Google Scholar]
- Capogrossi M. C., Lakatta E. G. Frequency modulation and synchronization of spontaneous oscillations in cardiac cells. Am J Physiol. 1985 Mar;248(3 Pt 2):H412–H418. doi: 10.1152/ajpheart.1985.248.3.H412. [DOI] [PubMed] [Google Scholar]
- Cheng H., Lederer W. J., Cannell M. B. Calcium sparks: elementary events underlying excitation-contraction coupling in heart muscle. Science. 1993 Oct 29;262(5134):740–744. doi: 10.1126/science.8235594. [DOI] [PubMed] [Google Scholar]
- Engel J., Fechner M., Sowerby A. J., Finch S. A., Stier A. Anisotropic propagation of Ca2+ waves in isolated cardiomyocytes. Biophys J. 1994 Jun;66(6):1756–1762. doi: 10.1016/S0006-3495(94)80997-X. [DOI] [PMC free article] [PubMed] [Google Scholar]
- Engel J., Sowerby A. J., Finch S. A., Fechner M., Stier A. Temperature dependence of Ca2+ wave properties in cardiomyocytes: implications for the mechanism of autocatalytic Ca2+ release in wave propagation. Biophys J. 1995 Jan;68(1):40–45. doi: 10.1016/S0006-3495(95)80196-7. [DOI] [PMC free article] [PubMed] [Google Scholar]
- Fabiato A. Myoplasmic free calcium concentration reached during the twitch of an intact isolated cardiac cell and during calcium-induced release of calcium from the sarcoplasmic reticulum of a skinned cardiac cell from the adult rat or rabbit ventricle. J Gen Physiol. 1981 Nov;78(5):457–497. doi: 10.1085/jgp.78.5.457. [DOI] [PMC free article] [PubMed] [Google Scholar]
- Foerster P., Müller S. C., Hess B. Critical size and curvature of wave formation in an excitable chemical medium. Proc Natl Acad Sci U S A. 1989 Sep;86(18):6831–6834. doi: 10.1073/pnas.86.18.6831. [DOI] [PMC free article] [PubMed] [Google Scholar]
- Girard S., Lückhoff A., Lechleiter J., Sneyd J., Clapham D. Two-dimensional model of calcium waves reproduces the patterns observed in Xenopus oocytes. Biophys J. 1992 Feb;61(2):509–517. doi: 10.1016/S0006-3495(92)81855-6. [DOI] [PMC free article] [PubMed] [Google Scholar]
- Golovina V. A., Rozenshtraukh L. V., Solov'ev B. S., Undrovinas A. I., Chernaia G. G. Volnoobraznye spontannye sokrashcheniia izolirovannykh kardiomiotsitov. Biofizika. 1986 Mar-Apr;31(2):283–289. [PubMed] [Google Scholar]
- Ishide N., Urayama T., Inoue K., Komaru T., Takishima T. Propagation and collision characteristics of calcium waves in rat myocytes. Am J Physiol. 1990 Sep;259(3 Pt 2):H940–H950. doi: 10.1152/ajpheart.1990.259.3.H940. [DOI] [PubMed] [Google Scholar]
- Kort A. A., Capogrossi M. C., Lakatta E. G. Frequency, amplitude, and propagation velocity of spontaneous Ca++-dependent contractile waves in intact adult rat cardiac muscle and isolated myocytes. Circ Res. 1985 Dec;57(6):844–855. doi: 10.1161/01.res.57.6.844. [DOI] [PubMed] [Google Scholar]
- Kushmerick M. J., Podolsky R. J. Ionic mobility in muscle cells. Science. 1969 Dec 5;166(3910):1297–1298. doi: 10.1126/science.166.3910.1297. [DOI] [PubMed] [Google Scholar]
- Lechleiter J. D., Clapham D. E. Molecular mechanisms of intracellular calcium excitability in X. laevis oocytes. Cell. 1992 Apr 17;69(2):283–294. doi: 10.1016/0092-8674(92)90409-6. [DOI] [PubMed] [Google Scholar]
- Linke W. A., Bartoo M. L., Pollack G. H. Spontaneous sarcomeric oscillations at intermediate activation levels in single isolated cardiac myofibrils. Circ Res. 1993 Oct;73(4):724–734. doi: 10.1161/01.res.73.4.724. [DOI] [PubMed] [Google Scholar]
- Lipp P., Niggli E. Microscopic spiral waves reveal positive feedback in subcellular calcium signaling. Biophys J. 1993 Dec;65(6):2272–2276. doi: 10.1016/S0006-3495(93)81316-X. [DOI] [PMC free article] [PubMed] [Google Scholar]
- Niggli E., Lederer W. J. Real-time confocal microscopy and calcium measurements in heart muscle cells: towards the development of a fluorescence microscope with high temporal and spatial resolution. Cell Calcium. 1990 Feb-Mar;11(2-3):121–130. doi: 10.1016/0143-4160(90)90065-3. [DOI] [PubMed] [Google Scholar]
- O'Neill S. C., Mill J. G., Eisner D. A. Local activation of contraction in isolated rat ventricular myocytes. Am J Physiol. 1990 Jun;258(6 Pt 1):C1165–C1168. doi: 10.1152/ajpcell.1990.258.6.C1165. [DOI] [PubMed] [Google Scholar]
- Rooney T. A., Thomas A. P. Intracellular calcium waves generated by Ins(1,4,5)P3-dependent mechanisms. Cell Calcium. 1993 Nov;14(10):674–690. doi: 10.1016/0143-4160(93)90094-m. [DOI] [PubMed] [Google Scholar]
- Sneyd J., Charles A. C., Sanderson M. J. A model for the propagation of intercellular calcium waves. Am J Physiol. 1994 Jan;266(1 Pt 1):C293–C302. doi: 10.1152/ajpcell.1994.266.1.C293. [DOI] [PubMed] [Google Scholar]
- Sneyd J., Kalachev L. V. A profile analysis of propagating calcium waves. Cell Calcium. 1994 Apr;15(4):289–296. doi: 10.1016/0143-4160(94)90068-x. [DOI] [PubMed] [Google Scholar]
- Stern M. D., Capogrossi M. C., Lakatta E. G. Spontaneous calcium release from the sarcoplasmic reticulum in myocardial cells: mechanisms and consequences. Cell Calcium. 1988 Dec;9(5-6):247–256. doi: 10.1016/0143-4160(88)90005-x. [DOI] [PubMed] [Google Scholar]
- Stern M. D. Theory of excitation-contraction coupling in cardiac muscle. Biophys J. 1992 Aug;63(2):497–517. doi: 10.1016/S0006-3495(92)81615-6. [DOI] [PMC free article] [PubMed] [Google Scholar]
- Tang Y., Othmer H. G. A model of calcium dynamics in cardiac myocytes based on the kinetics of ryanodine-sensitive calcium channels. Biophys J. 1994 Dec;67(6):2223–2235. doi: 10.1016/S0006-3495(94)80707-6. [DOI] [PMC free article] [PubMed] [Google Scholar]
- Trafford A. W., O'Neill S. C., Eisner D. A. Factors affecting the propagation of locally activated systolic Ca transients in rat ventricular myocytes. Pflugers Arch. 1993 Oct;425(1-2):181–183. doi: 10.1007/BF00374521. [DOI] [PubMed] [Google Scholar]
- Wier W. G., Blatter L. A. Ca(2+)-oscillations and Ca(2+)-waves in mammalian cardiac and vascular smooth muscle cells. Cell Calcium. 1991 Feb-Mar;12(2-3):241–254. doi: 10.1016/0143-4160(91)90024-9. [DOI] [PubMed] [Google Scholar]
- Wier W. G., Cannell M. B., Berlin J. R., Marban E., Lederer W. J. Cellular and subcellular heterogeneity of [Ca2+]i in single heart cells revealed by fura-2. Science. 1987 Jan 16;235(4786):325–328. doi: 10.1126/science.3798114. [DOI] [PubMed] [Google Scholar]
- Williams D. A., Delbridge L. M., Cody S. H., Harris P. J., Morgan T. O. Spontaneous and propagated calcium release in isolated cardiac myocytes viewed by confocal microscopy. Am J Physiol. 1992 Mar;262(3 Pt 1):C731–C742. doi: 10.1152/ajpcell.1992.262.3.C731. [DOI] [PubMed] [Google Scholar]
- Williams D. A. Mechanisms of calcium release and propagation in cardiac cells. Do studies with confocal microscopy add to our understanding? Cell Calcium. 1993 Nov;14(10):724–735. doi: 10.1016/0143-4160(93)90098-q. [DOI] [PubMed] [Google Scholar]
- ter Keurs H. E., Backx P. H., de Tombe P. P., Mulder B. J. Aftercontractions and excitation-contraction coupling in rat cardiac muscle. Can J Physiol Pharmacol. 1988 Sep;66(9):1239–1245. doi: 10.1139/y88-204. [DOI] [PubMed] [Google Scholar]