Abstract
We model the space between the junctional sarcoplasmic reticulum (JSR) membrane and the inner leaflet of the transverse tubular ("T") sarcolemmal (SL) membrane, the diadic cleft, with respect to calcium (Ca) concentration and movement. The model predicts the following: 1) Ca influx via the "L" channel increases [Ca] to 1 microM within a distance of 50 nm from the channel mouth in < 500 microseconds. This is sufficient to trigger Ca release from a domain of 9 "feet." 2) By contrast, "reverse" Na/Ca exchange will increase [Ca] to approximately 0.5 microM throughout the cleft space in 10 ms, sufficient to trigger Ca release, but clearly to a lesser extent and more slowly than the channel. 3) After a 20-ms JSR release into the cleft via the "feet" [Ca] peaks at 600 microM (cleft center) to 100 microM (cleft periphery) and then declines to diastolic level (100 nM) within 150 ms throughout the cleft. 4) The ratio of flux out of the cleft via Na/Ca exchange to flux out of the cleft to the cytosol varies inversely as JSR Ca release. 5) Removal of SL anionic Ca-binding sites from the model will cause [Ca] to fall to 100 nM throughout the cleft in < 1 ms after JSR release ceases. This markedly reduces Na/Ca exchange. 6) Removal from or decreased concentration of Na/Ca exchangers in the cleft will cause [Ca] to fall too slowly after JSR release to permit triggered release upon subsequent excitation.
Full text
PDF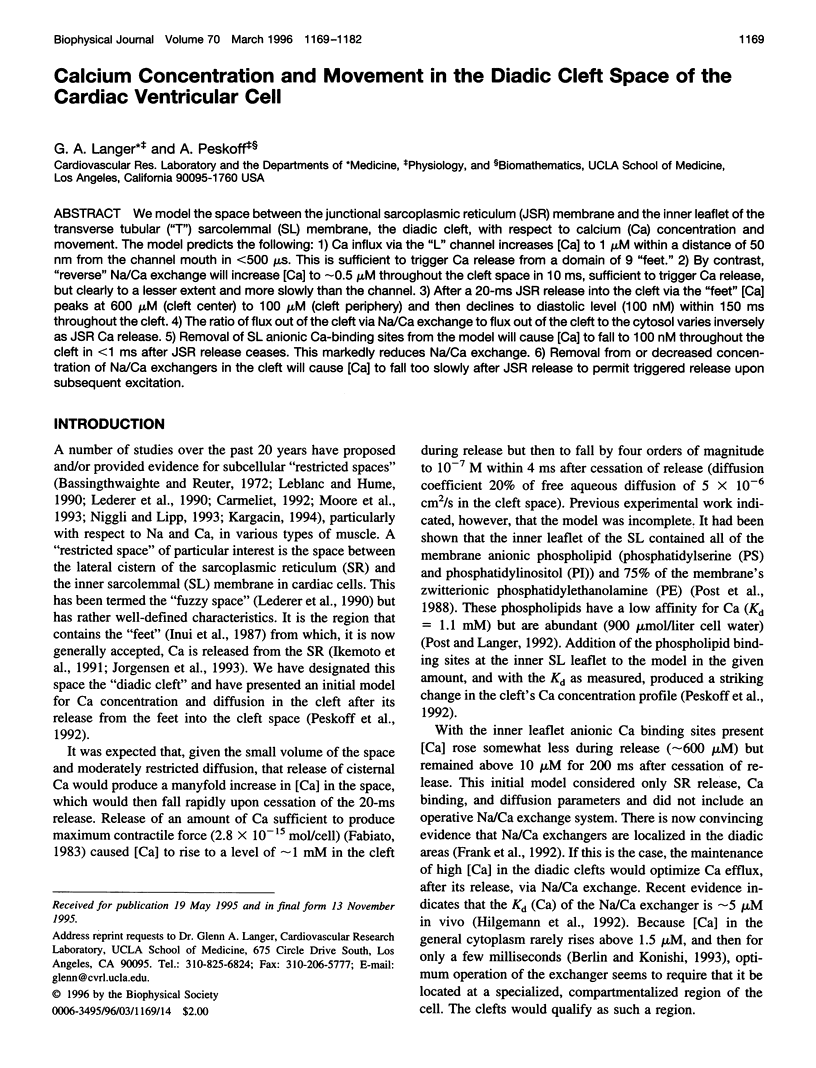
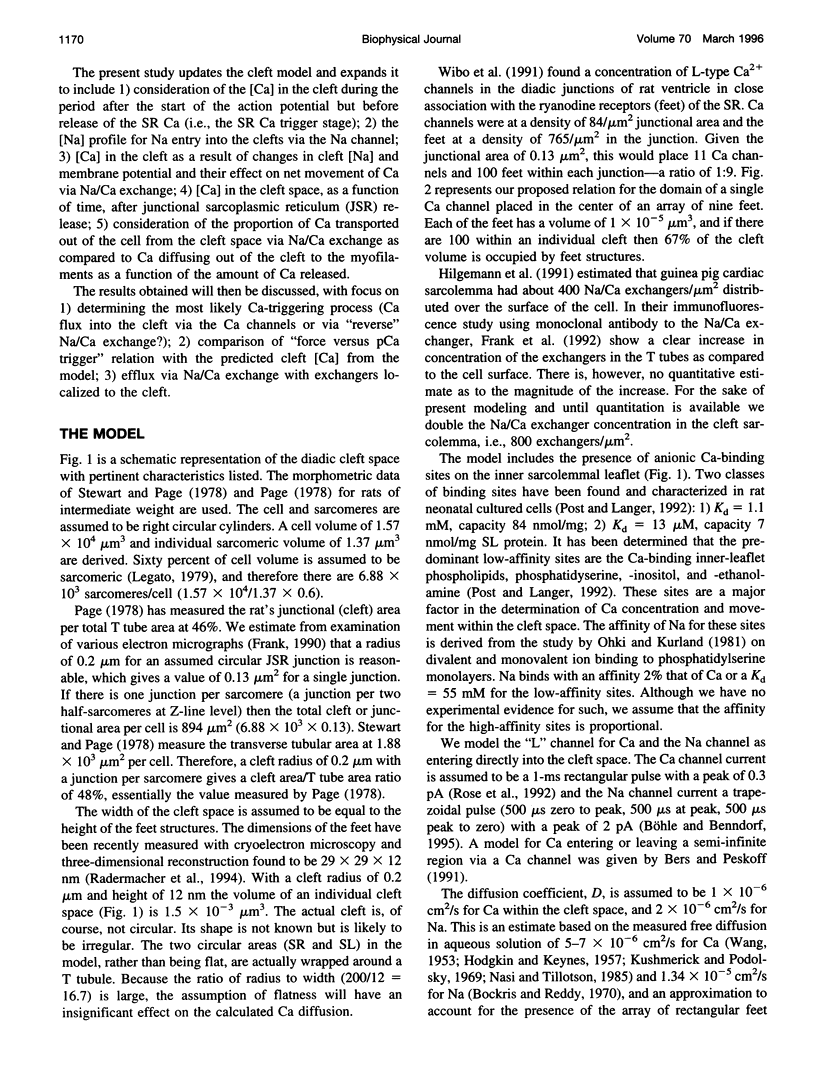
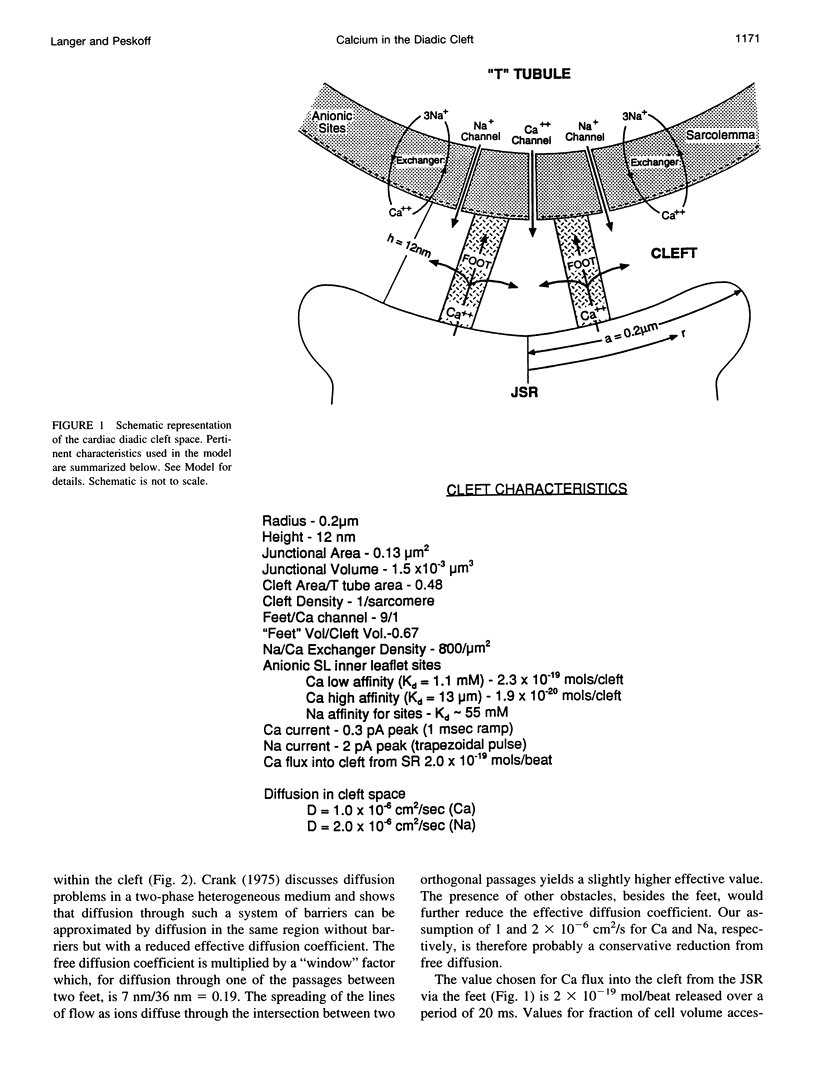
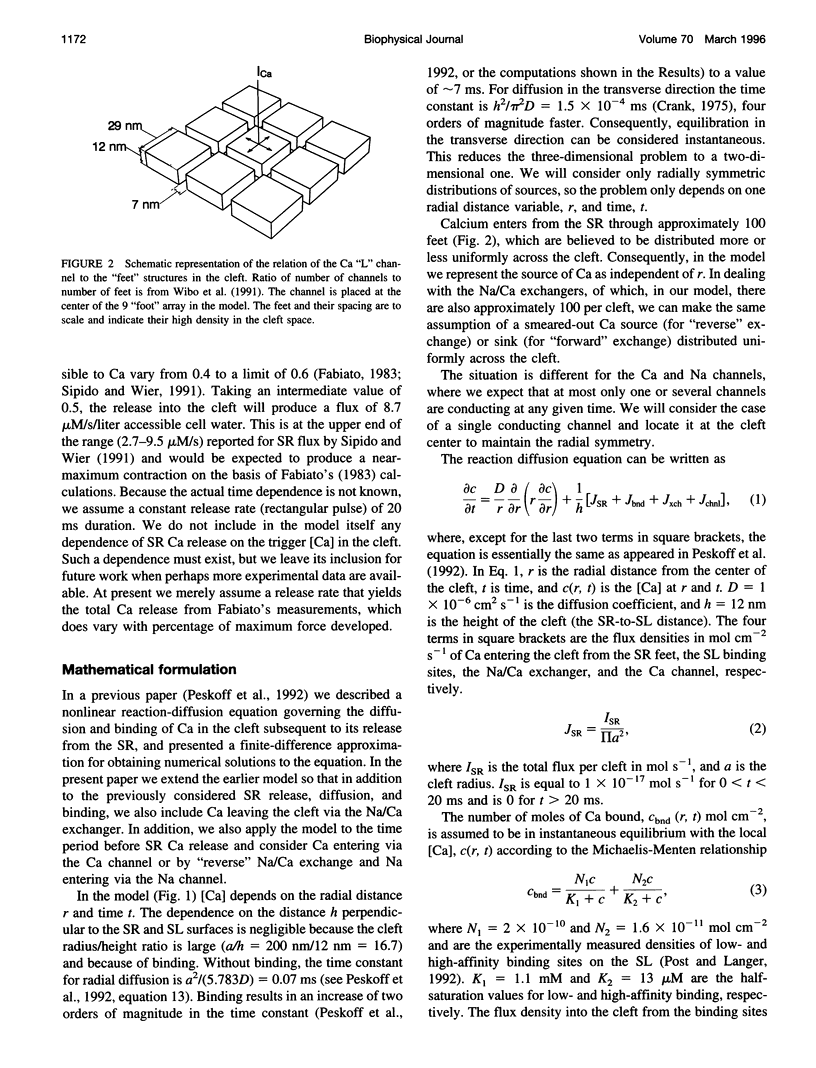
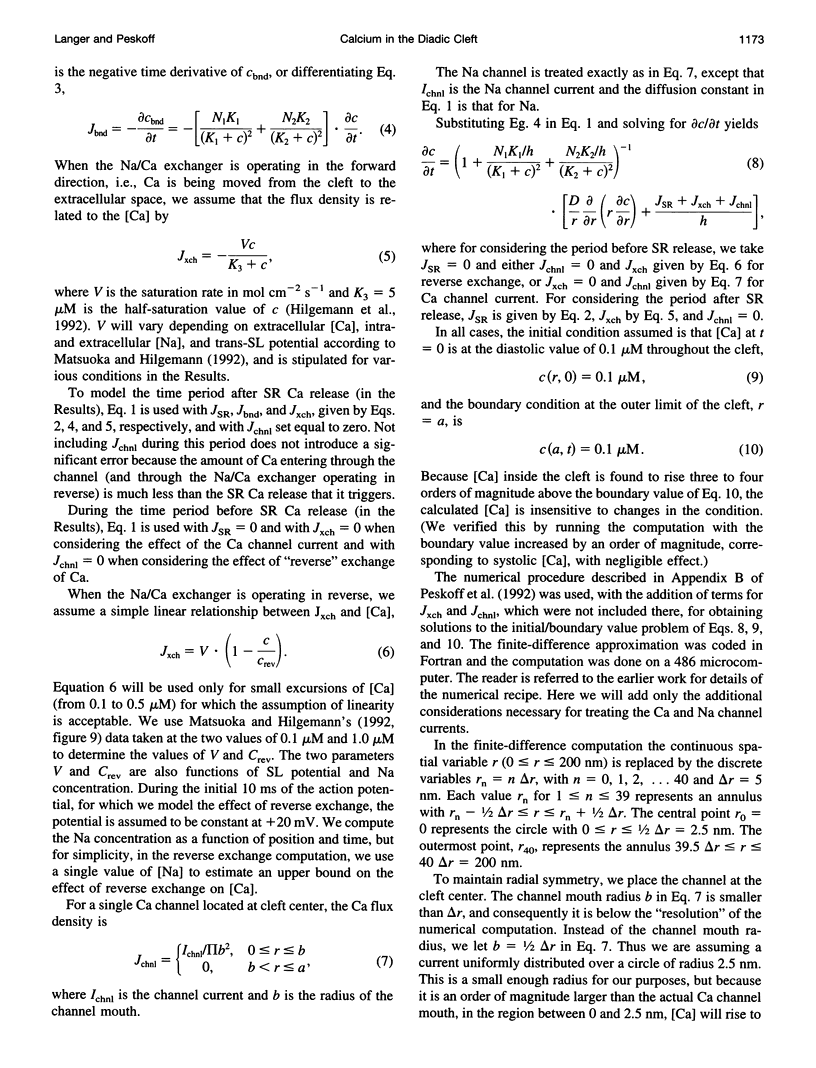
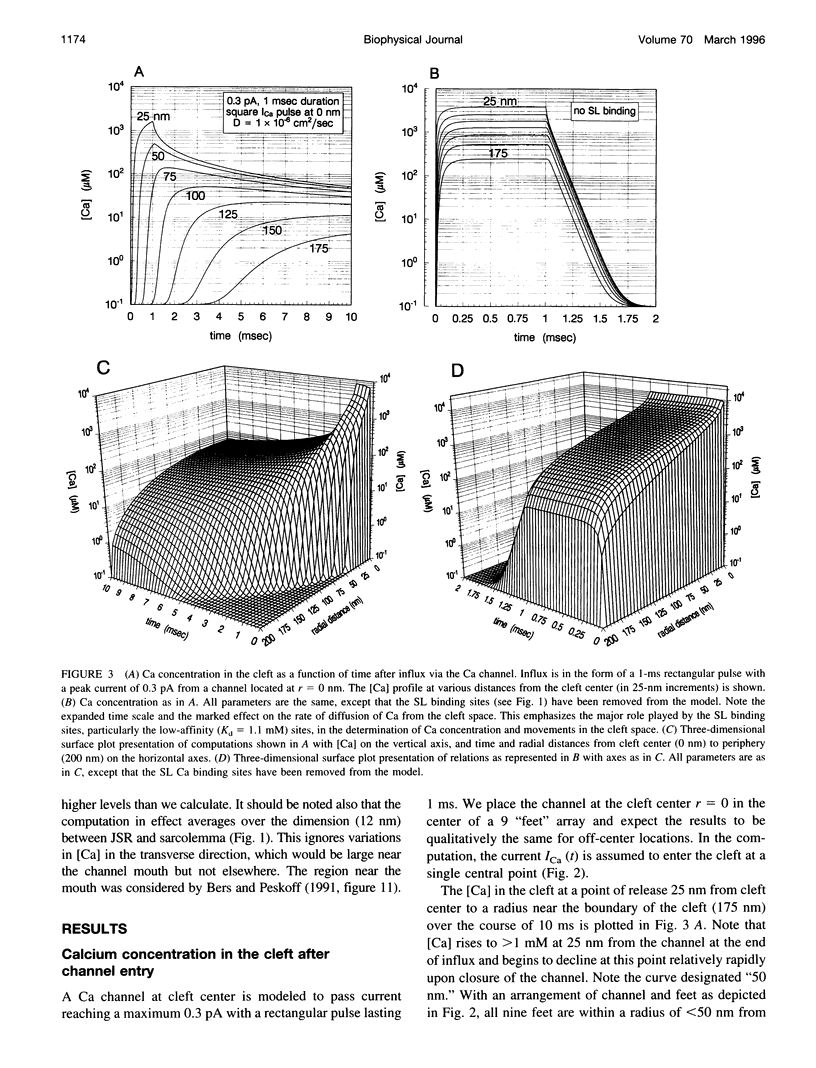
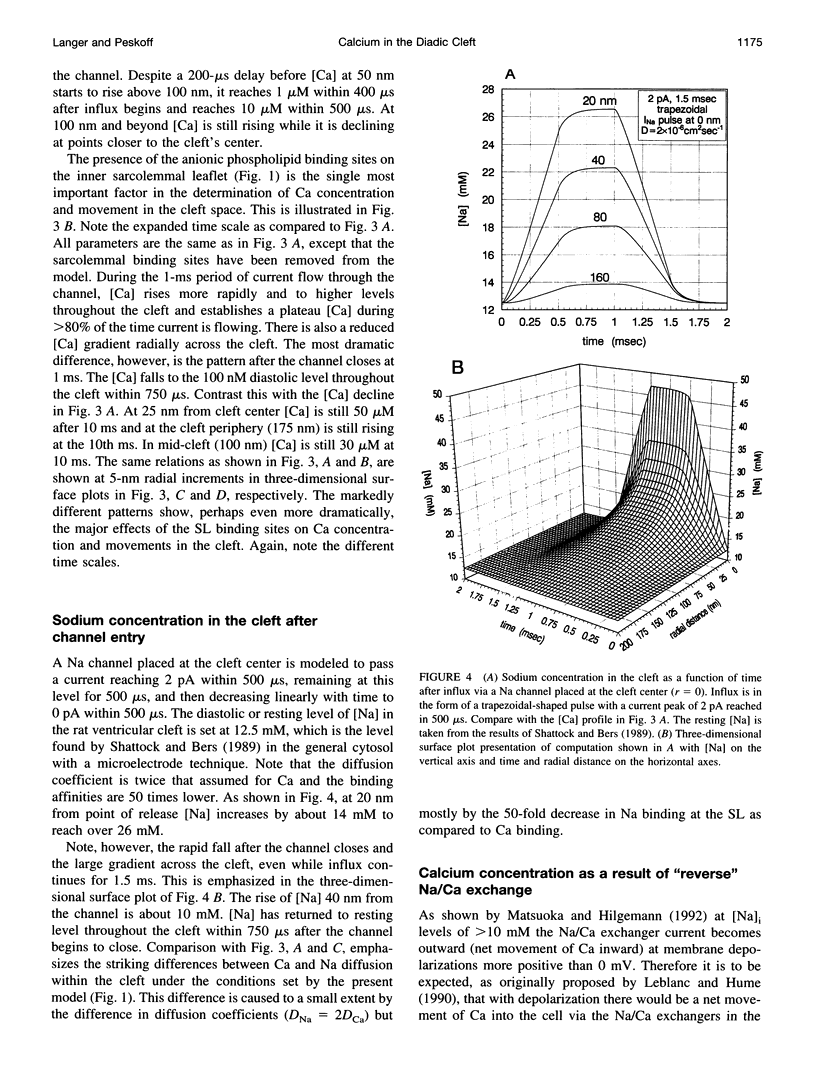
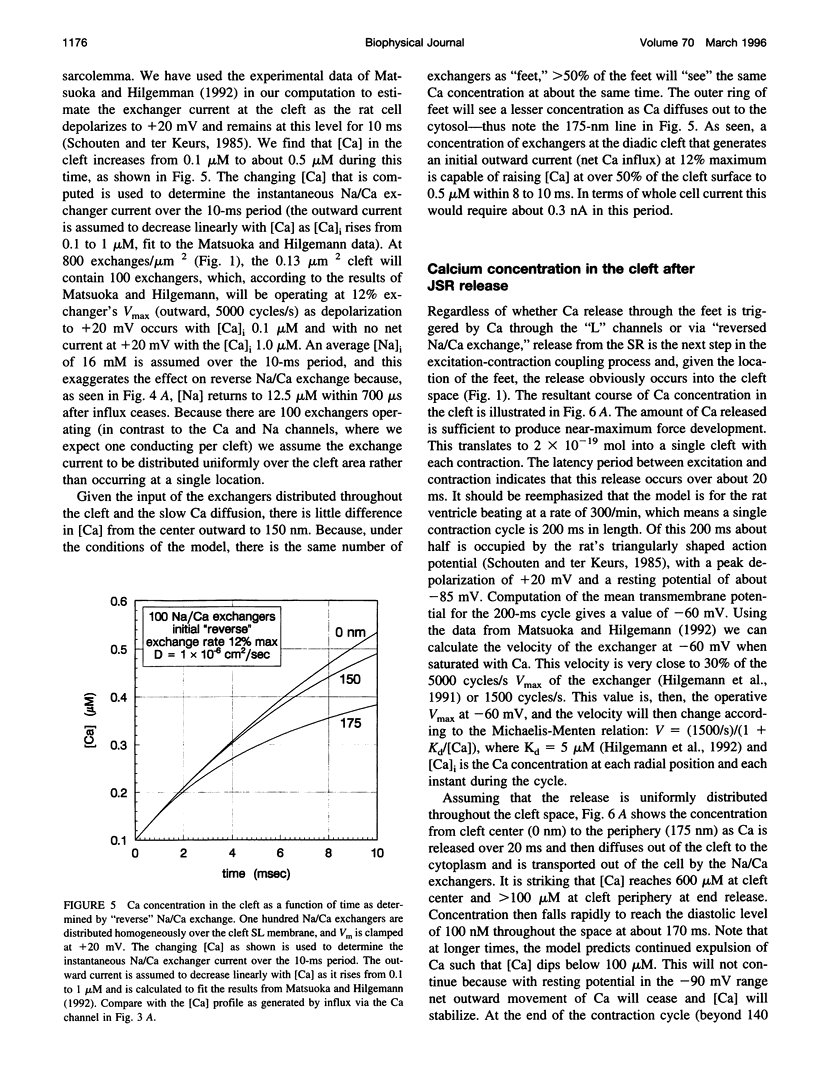
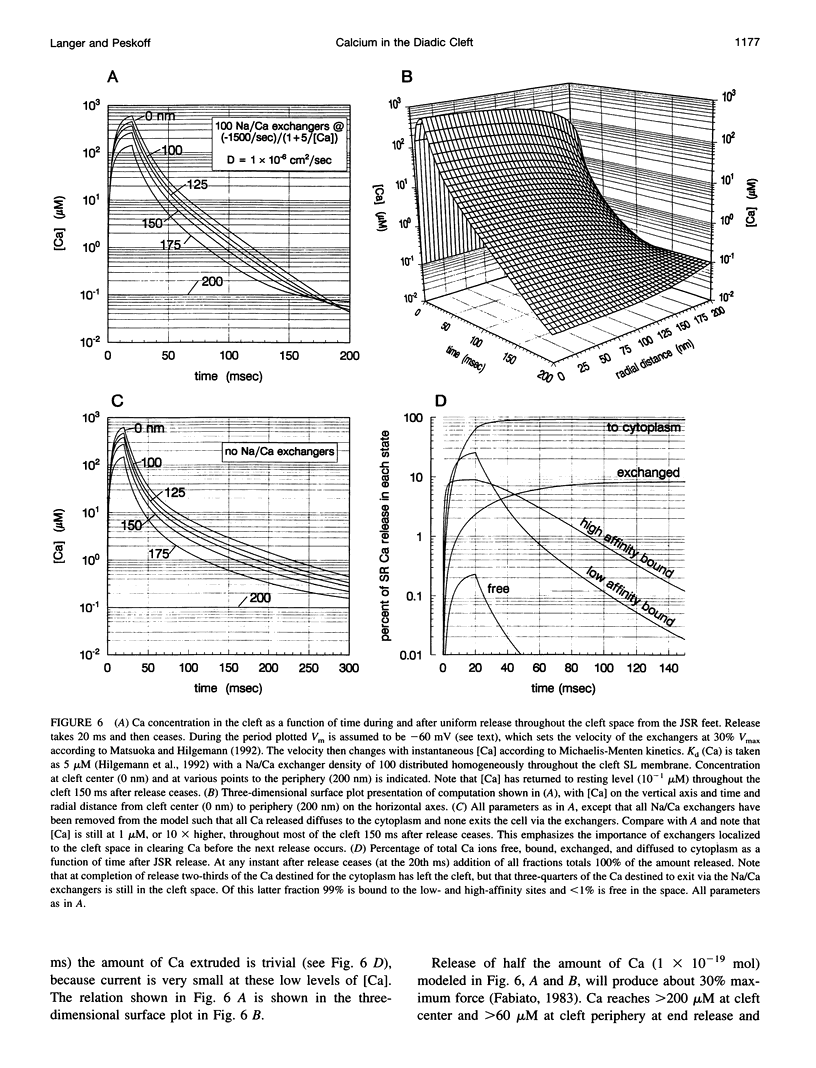
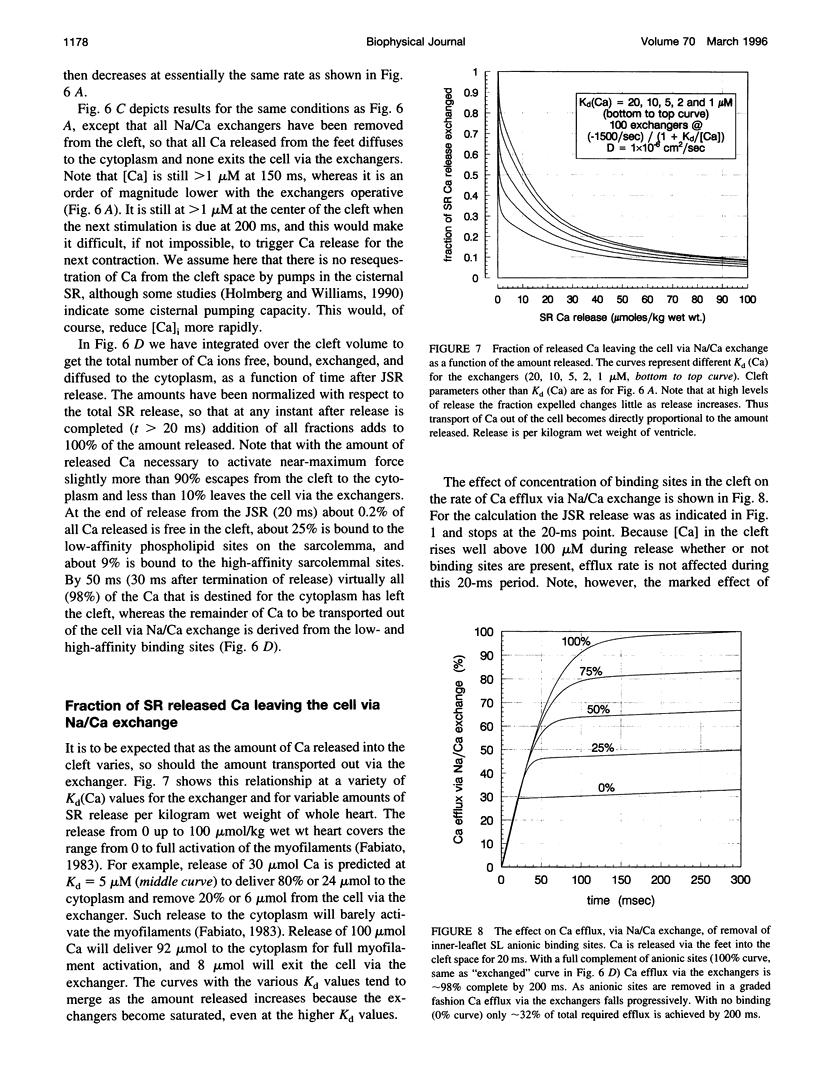
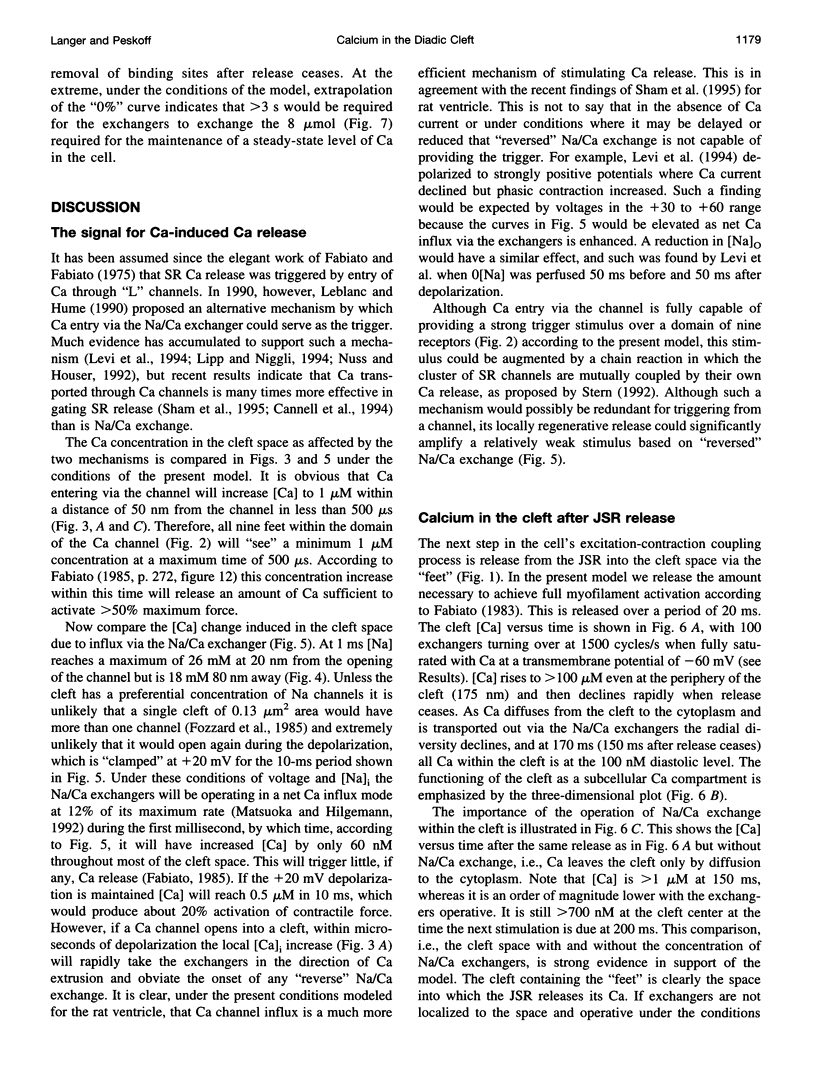
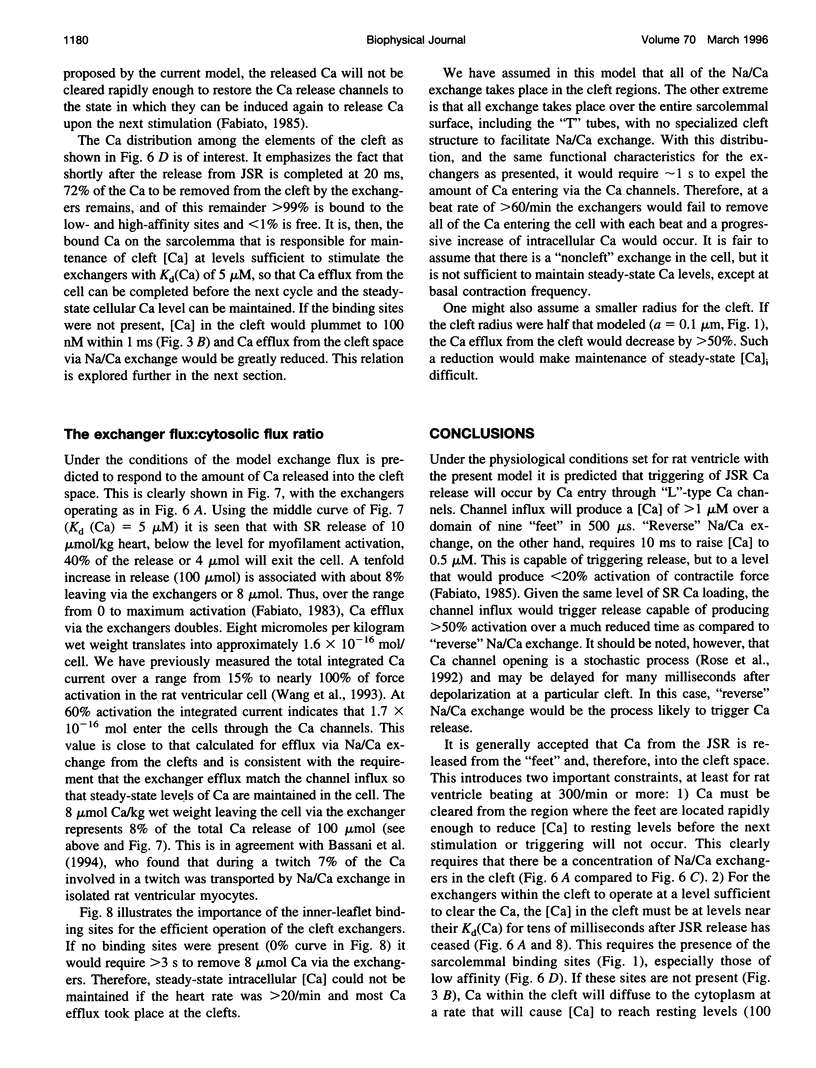
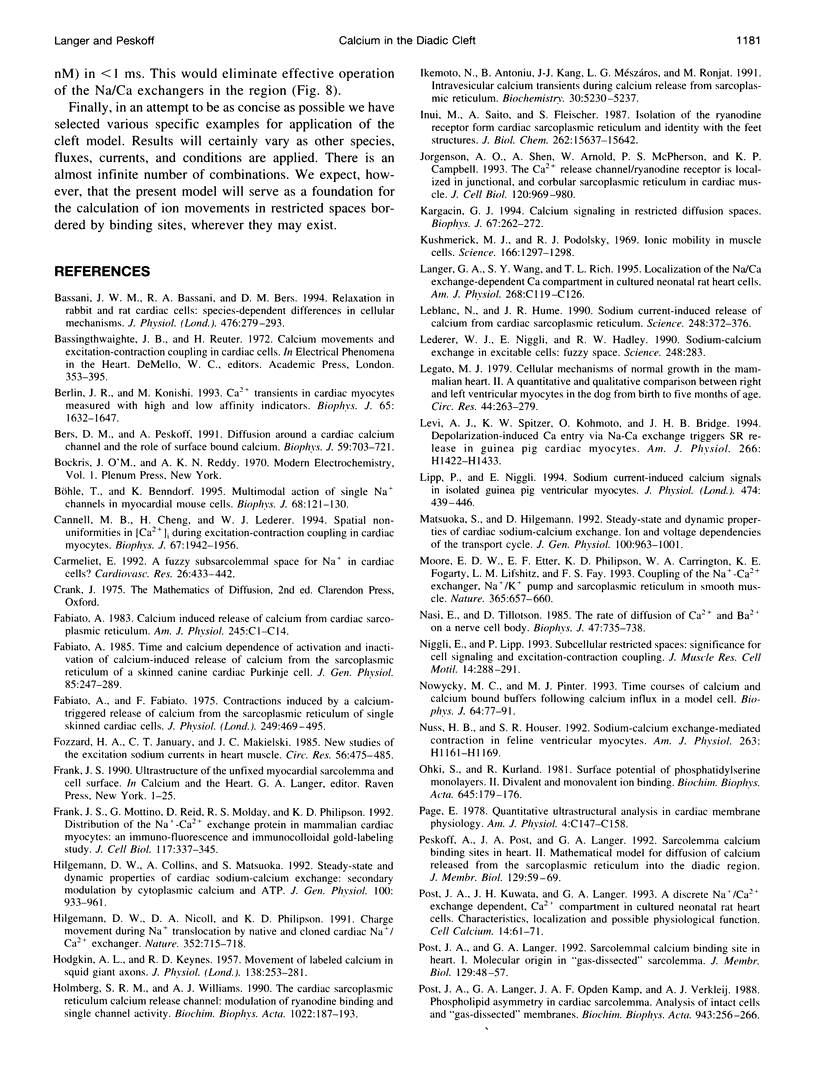
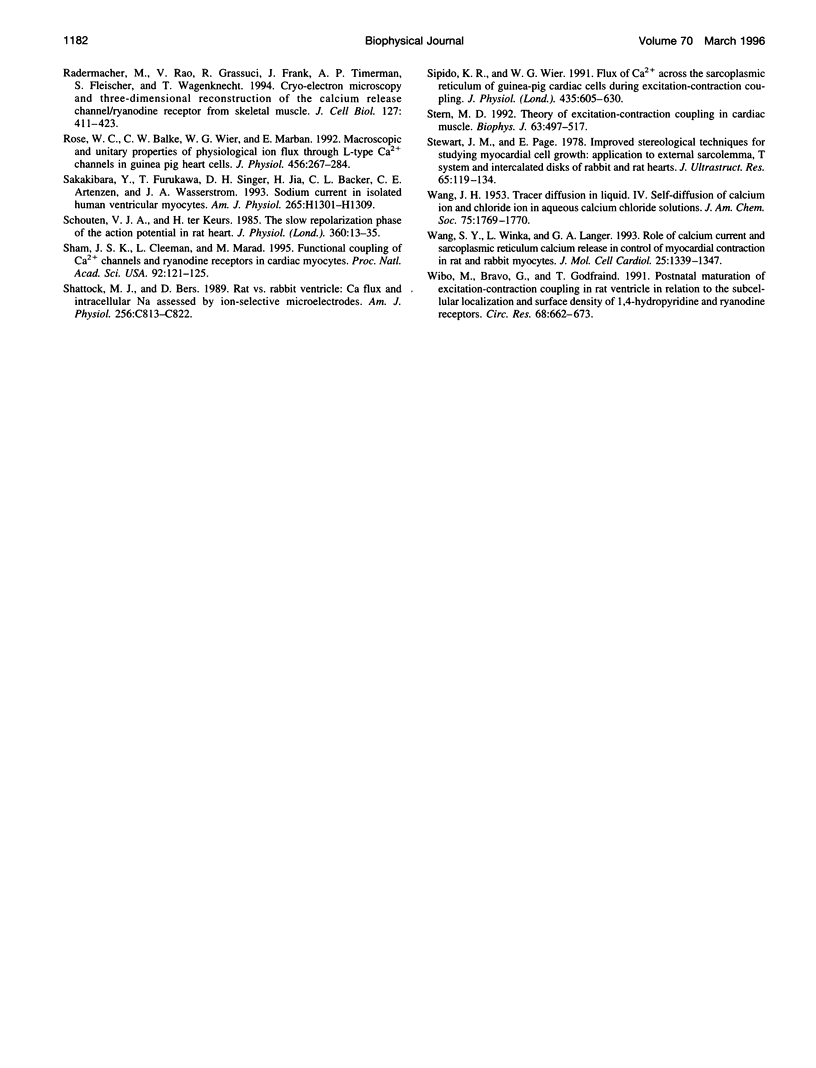
Selected References
These references are in PubMed. This may not be the complete list of references from this article.
- Bassani J. W., Bassani R. A., Bers D. M. Relaxation in rabbit and rat cardiac cells: species-dependent differences in cellular mechanisms. J Physiol. 1994 Apr 15;476(2):279–293. doi: 10.1113/jphysiol.1994.sp020130. [DOI] [PMC free article] [PubMed] [Google Scholar]
- Berlin J. R., Konishi M. Ca2+ transients in cardiac myocytes measured with high and low affinity Ca2+ indicators. Biophys J. 1993 Oct;65(4):1632–1647. doi: 10.1016/S0006-3495(93)81211-6. [DOI] [PMC free article] [PubMed] [Google Scholar]
- Bers D. M., Peskoff A. Diffusion around a cardiac calcium channel and the role of surface bound calcium. Biophys J. 1991 Mar;59(3):703–721. doi: 10.1016/S0006-3495(91)82284-6. [DOI] [PMC free article] [PubMed] [Google Scholar]
- Böhle T., Benndorf K. Multimodal action of single Na+ channels in myocardial mouse cells. Biophys J. 1995 Jan;68(1):121–130. doi: 10.1016/S0006-3495(95)80166-9. [DOI] [PMC free article] [PubMed] [Google Scholar]
- Cannell M. B., Cheng H., Lederer W. J. Spatial non-uniformities in [Ca2+]i during excitation-contraction coupling in cardiac myocytes. Biophys J. 1994 Nov;67(5):1942–1956. doi: 10.1016/S0006-3495(94)80677-0. [DOI] [PMC free article] [PubMed] [Google Scholar]
- Carmeliet E. A fuzzy subsarcolemmal space for intracellular Na+ in cardiac cells? Cardiovasc Res. 1992 May;26(5):433–442. doi: 10.1093/cvr/26.5.433. [DOI] [PubMed] [Google Scholar]
- Fabiato A. Calcium-induced release of calcium from the cardiac sarcoplasmic reticulum. Am J Physiol. 1983 Jul;245(1):C1–14. doi: 10.1152/ajpcell.1983.245.1.C1. [DOI] [PubMed] [Google Scholar]
- Fabiato A., Fabiato F. Contractions induced by a calcium-triggered release of calcium from the sarcoplasmic reticulum of single skinned cardiac cells. J Physiol. 1975 Aug;249(3):469–495. doi: 10.1113/jphysiol.1975.sp011026. [DOI] [PMC free article] [PubMed] [Google Scholar]
- Fabiato A. Time and calcium dependence of activation and inactivation of calcium-induced release of calcium from the sarcoplasmic reticulum of a skinned canine cardiac Purkinje cell. J Gen Physiol. 1985 Feb;85(2):247–289. doi: 10.1085/jgp.85.2.247. [DOI] [PMC free article] [PubMed] [Google Scholar]
- Fozzard H. A., January C. T., Makielski J. C. New studies of the excitatory sodium currents in heart muscle. Circ Res. 1985 Apr;56(4):475–485. doi: 10.1161/01.res.56.4.475. [DOI] [PubMed] [Google Scholar]
- Frank J. S., Mottino G., Reid D., Molday R. S., Philipson K. D. Distribution of the Na(+)-Ca2+ exchange protein in mammalian cardiac myocytes: an immunofluorescence and immunocolloidal gold-labeling study. J Cell Biol. 1992 Apr;117(2):337–345. doi: 10.1083/jcb.117.2.337. [DOI] [PMC free article] [PubMed] [Google Scholar]
- HODGKIN A. L., KEYNES R. D. Movements of labelled calcium in squid giant axons. J Physiol. 1957 Sep 30;138(2):253–281. doi: 10.1113/jphysiol.1957.sp005850. [DOI] [PMC free article] [PubMed] [Google Scholar]
- Hilgemann D. W., Collins A., Matsuoka S. Steady-state and dynamic properties of cardiac sodium-calcium exchange. Secondary modulation by cytoplasmic calcium and ATP. J Gen Physiol. 1992 Dec;100(6):933–961. doi: 10.1085/jgp.100.6.933. [DOI] [PMC free article] [PubMed] [Google Scholar]
- Hilgemann D. W., Nicoll D. A., Philipson K. D. Charge movement during Na+ translocation by native and cloned cardiac Na+/Ca2+ exchanger. Nature. 1991 Aug 22;352(6337):715–718. doi: 10.1038/352715a0. [DOI] [PubMed] [Google Scholar]
- Holmberg S. R., Williams A. J. The cardiac sarcoplasmic reticulum calcium-release channel: modulation of ryanodine binding and single-channel activity. Biochim Biophys Acta. 1990 Feb 28;1022(2):187–193. doi: 10.1016/0005-2736(90)90113-3. [DOI] [PubMed] [Google Scholar]
- Ikemoto N., Antoniu B., Kang J. J., Mészáros L. G., Ronjat M. Intravesicular calcium transient during calcium release from sarcoplasmic reticulum. Biochemistry. 1991 May 28;30(21):5230–5237. doi: 10.1021/bi00235a017. [DOI] [PubMed] [Google Scholar]
- Inui M., Saito A., Fleischer S. Isolation of the ryanodine receptor from cardiac sarcoplasmic reticulum and identity with the feet structures. J Biol Chem. 1987 Nov 15;262(32):15637–15642. [PubMed] [Google Scholar]
- Jorgensen A. O., Shen A. C., Arnold W., McPherson P. S., Campbell K. P. The Ca2+-release channel/ryanodine receptor is localized in junctional and corbular sarcoplasmic reticulum in cardiac muscle. J Cell Biol. 1993 Feb;120(4):969–980. doi: 10.1083/jcb.120.4.969. [DOI] [PMC free article] [PubMed] [Google Scholar]
- Kargacin G. J. Calcium signaling in restricted diffusion spaces. Biophys J. 1994 Jul;67(1):262–272. doi: 10.1016/S0006-3495(94)80477-1. [DOI] [PMC free article] [PubMed] [Google Scholar]
- Kushmerick M. J., Podolsky R. J. Ionic mobility in muscle cells. Science. 1969 Dec 5;166(3910):1297–1298. doi: 10.1126/science.166.3910.1297. [DOI] [PubMed] [Google Scholar]
- Leblanc N., Hume J. R. Sodium current-induced release of calcium from cardiac sarcoplasmic reticulum. Science. 1990 Apr 20;248(4953):372–376. doi: 10.1126/science.2158146. [DOI] [PubMed] [Google Scholar]
- Lederer W. J., Niggli E., Hadley R. W. Sodium-calcium exchange in excitable cells: fuzzy space. Science. 1990 Apr 20;248(4953):283–283. doi: 10.1126/science.2326638. [DOI] [PubMed] [Google Scholar]
- Legato M. J. Cellular mechanisms of normal growth in the mammalian heart. II. A quantitative and qualitative comparison between the right and left ventricular myocytes in the dog from birth to five months of age. Circ Res. 1979 Feb;44(2):263–279. doi: 10.1161/01.res.44.2.263. [DOI] [PubMed] [Google Scholar]
- Lipp P., Niggli E. Sodium current-induced calcium signals in isolated guinea-pig ventricular myocytes. J Physiol. 1994 Feb 1;474(3):439–446. doi: 10.1113/jphysiol.1994.sp020035. [DOI] [PMC free article] [PubMed] [Google Scholar]
- Matsuoka S., Hilgemann D. W. Steady-state and dynamic properties of cardiac sodium-calcium exchange. Ion and voltage dependencies of the transport cycle. J Gen Physiol. 1992 Dec;100(6):963–1001. doi: 10.1085/jgp.100.6.963. [DOI] [PMC free article] [PubMed] [Google Scholar]
- Moore E. D., Etter E. F., Philipson K. D., Carrington W. A., Fogarty K. E., Lifshitz L. M., Fay F. S. Coupling of the Na+/Ca2+ exchanger, Na+/K+ pump and sarcoplasmic reticulum in smooth muscle. Nature. 1993 Oct 14;365(6447):657–660. doi: 10.1038/365657a0. [DOI] [PubMed] [Google Scholar]
- Nasi E., Tillotson D. The rate of diffusion of Ca2+ and Ba2+ in a nerve cell body. Biophys J. 1985 May;47(5):735–738. doi: 10.1016/S0006-3495(85)83972-2. [DOI] [PMC free article] [PubMed] [Google Scholar]
- Niggli E., Lipp P. Subcellular restricted spaces: significance for cell signalling and excitation-contraction coupling. J Muscle Res Cell Motil. 1993 Jun;14(3):288–291. doi: 10.1007/BF00123093. [DOI] [PubMed] [Google Scholar]
- Nowycky M. C., Pinter M. J. Time courses of calcium and calcium-bound buffers following calcium influx in a model cell. Biophys J. 1993 Jan;64(1):77–91. doi: 10.1016/S0006-3495(93)81342-0. [DOI] [PMC free article] [PubMed] [Google Scholar]
- Nuss H. B., Houser S. R. Sodium-calcium exchange-mediated contractions in feline ventricular myocytes. Am J Physiol. 1992 Oct;263(4 Pt 2):H1161–H1169. doi: 10.1152/ajpheart.1992.263.4.H1161. [DOI] [PubMed] [Google Scholar]
- Ohki S., Kurland R. Surface potential of phosphatidylserine monolayers. II. Divalent and monovalent ion binding. Biochim Biophys Acta. 1981 Jul 20;645(2):170–176. doi: 10.1016/0005-2736(81)90187-5. [DOI] [PubMed] [Google Scholar]
- Peskoff A., Post J. A., Langer G. A. Sarcolemmal calcium binding sites in heart: II. Mathematical model for diffusion of calcium released from the sarcoplasmic reticulum into the diadic region. J Membr Biol. 1992 Jul;129(1):59–69. doi: 10.1007/BF00232055. [DOI] [PubMed] [Google Scholar]
- Post J. A., Kuwata J. H., Langer G. A. A discrete Na+/Ca2+ exchange dependent, Ca2+ compartment in cultured neonatal rat heart cells. Characteristics, localization and possible physiological function. Cell Calcium. 1993 Jan;14(1):61–71. doi: 10.1016/0143-4160(93)90019-3. [DOI] [PubMed] [Google Scholar]
- Post J. A., Langer G. A., Op den Kamp J. A., Verkleij A. J. Phospholipid asymmetry in cardiac sarcolemma. Analysis of intact cells and 'gas-dissected' membranes. Biochim Biophys Acta. 1988 Aug 18;943(2):256–266. doi: 10.1016/0005-2736(88)90557-3. [DOI] [PubMed] [Google Scholar]
- Post J. A., Langer G. A. Sarcolemmal calcium binding sites in heart: I. Molecular origin in "gas-dissected" sarcolemma. J Membr Biol. 1992 Jul;129(1):49–57. doi: 10.1007/BF00232054. [DOI] [PubMed] [Google Scholar]
- Radermacher M., Rao V., Grassucci R., Frank J., Timerman A. P., Fleischer S., Wagenknecht T. Cryo-electron microscopy and three-dimensional reconstruction of the calcium release channel/ryanodine receptor from skeletal muscle. J Cell Biol. 1994 Oct;127(2):411–423. doi: 10.1083/jcb.127.2.411. [DOI] [PMC free article] [PubMed] [Google Scholar]
- Rose W. C., Balke C. W., Wier W. G., Marban E. Macroscopic and unitary properties of physiological ion flux through L-type Ca2+ channels in guinea-pig heart cells. J Physiol. 1992 Oct;456:267–284. doi: 10.1113/jphysiol.1992.sp019336. [DOI] [PMC free article] [PubMed] [Google Scholar]
- Sakakibara Y., Furukawa T., Singer D. H., Jia H., Backer C. L., Arentzen C. E., Wasserstrom J. A. Sodium current in isolated human ventricular myocytes. Am J Physiol. 1993 Oct;265(4 Pt 2):H1301–H1309. doi: 10.1152/ajpheart.1993.265.4.H1301. [DOI] [PubMed] [Google Scholar]
- Sham J. S., Cleemann L., Morad M. Functional coupling of Ca2+ channels and ryanodine receptors in cardiac myocytes. Proc Natl Acad Sci U S A. 1995 Jan 3;92(1):121–125. doi: 10.1073/pnas.92.1.121. [DOI] [PMC free article] [PubMed] [Google Scholar]
- Shattock M. J., Bers D. M. Rat vs. rabbit ventricle: Ca flux and intracellular Na assessed by ion-selective microelectrodes. Am J Physiol. 1989 Apr;256(4 Pt 1):C813–C822. doi: 10.1152/ajpcell.1989.256.4.C813. [DOI] [PubMed] [Google Scholar]
- Sipido K. R., Wier W. G. Flux of Ca2+ across the sarcoplasmic reticulum of guinea-pig cardiac cells during excitation-contraction coupling. J Physiol. 1991 Apr;435:605–630. doi: 10.1113/jphysiol.1991.sp018528. [DOI] [PMC free article] [PubMed] [Google Scholar]
- Stern M. D. Theory of excitation-contraction coupling in cardiac muscle. Biophys J. 1992 Aug;63(2):497–517. doi: 10.1016/S0006-3495(92)81615-6. [DOI] [PMC free article] [PubMed] [Google Scholar]
- Stewart J. M., Page E. Improved stereological techniques for studying myocardial cell growth: application to external sarcolemma, T system, and intercalated disks of rabbit and rat hearts. J Ultrastruct Res. 1978 Nov;65(2):119–134. doi: 10.1016/s0022-5320(78)90050-3. [DOI] [PubMed] [Google Scholar]
- Wang S. Y., Winka L., Langer G. A. Role of calcium current and sarcoplasmic reticulum calcium release in control of myocardial contraction in rat and rabbit myocytes. J Mol Cell Cardiol. 1993 Nov;25(11):1339–1347. doi: 10.1006/jmcc.1993.1146. [DOI] [PubMed] [Google Scholar]
- Wibo M., Bravo G., Godfraind T. Postnatal maturation of excitation-contraction coupling in rat ventricle in relation to the subcellular localization and surface density of 1,4-dihydropyridine and ryanodine receptors. Circ Res. 1991 Mar;68(3):662–673. doi: 10.1161/01.res.68.3.662. [DOI] [PubMed] [Google Scholar]