Abstract
Using the patch clamp technique, we have investigated the blockade of maxi-K+ channels present on vas deferens epithelial cells by extracellular Ba2+. With symmetrical 140 mM K+ solutions, Ba2+ produced discrete blocking events consisting of both long closings of seconds duration (slow block) and fast closings of milliseconds duration (flickering block). Kinetic analysis showed that flickering block occurred according to an "open channel blocking" scheme and was eliminated by reducing external K+ to 4.5 mM. Slow block showed a complex voltage-dependence. At potentials between -20 mV and 20 mV, blockade was voltage-dependent; at potentials greater than 20 mV, blockade was voltage-independent, but markedly sensitive to the extracellular K+ concentration. These data reveal that the vas deferens maxi-K+ channel has two Ba2+ binding sites accessible from the extracellular side. Site one is located at the cytoplasmic side of the gating region and binding to this site causes flickering block. Site two is located close to the extracellular mouth of the channel and binding to this site causes slow block.
Full text
PDF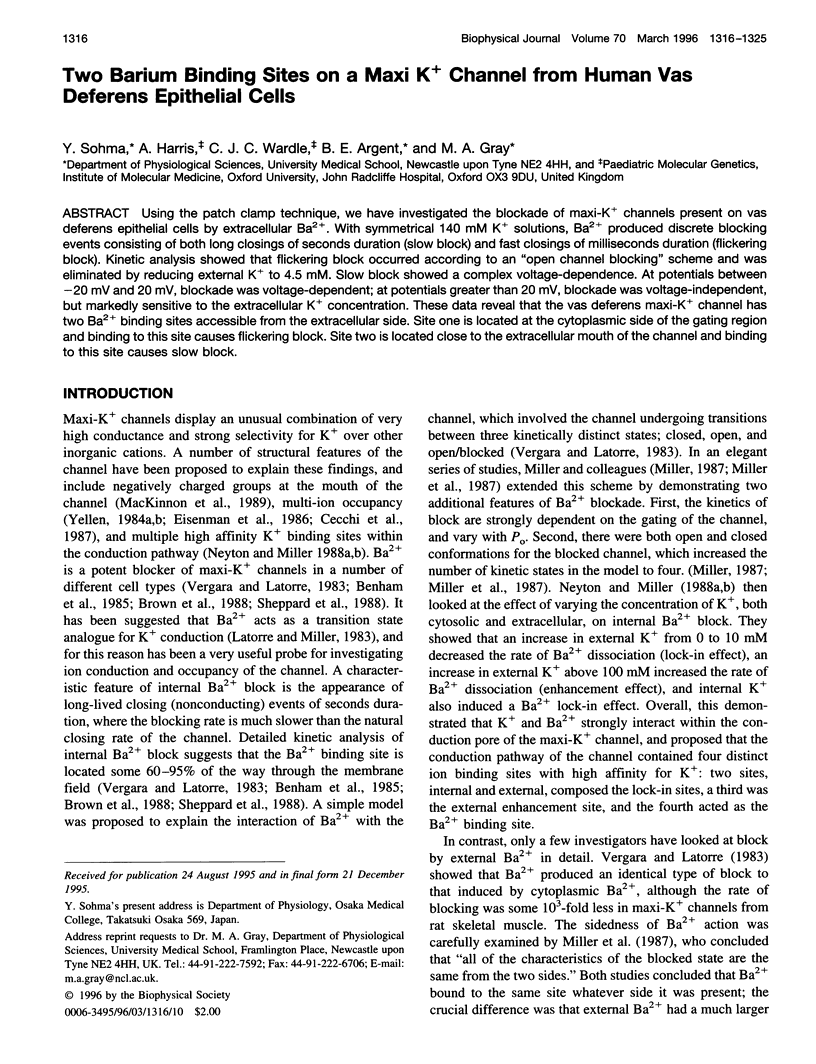
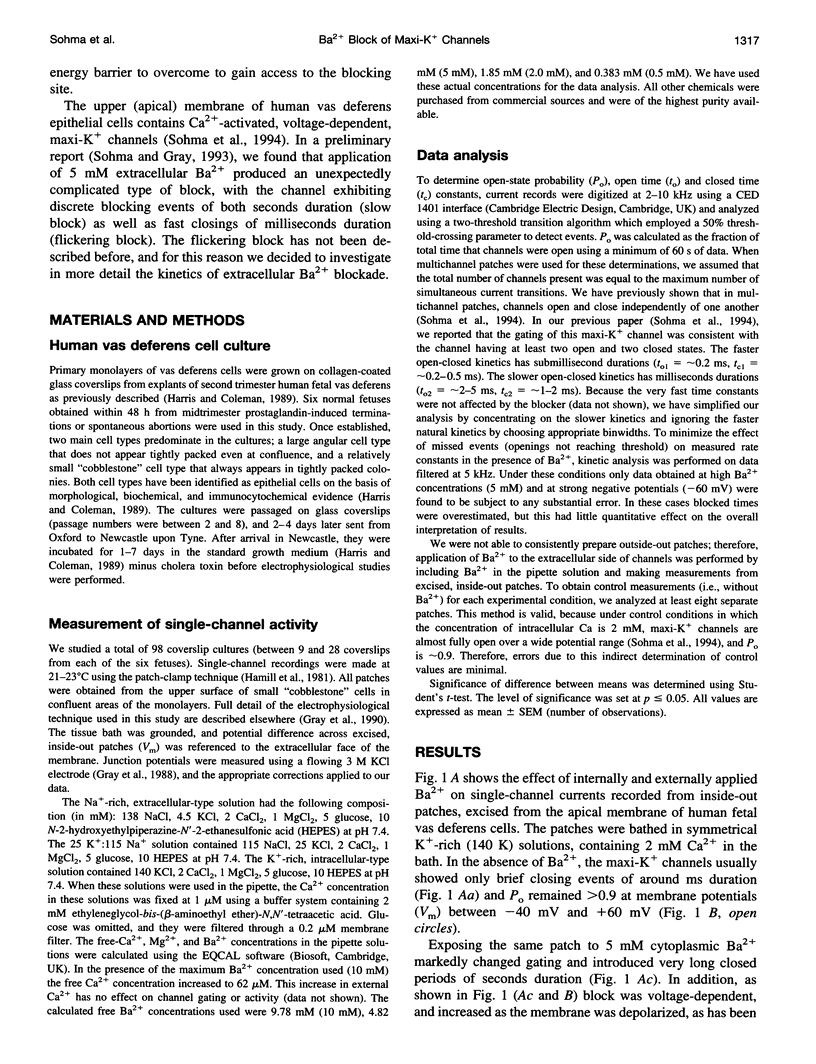
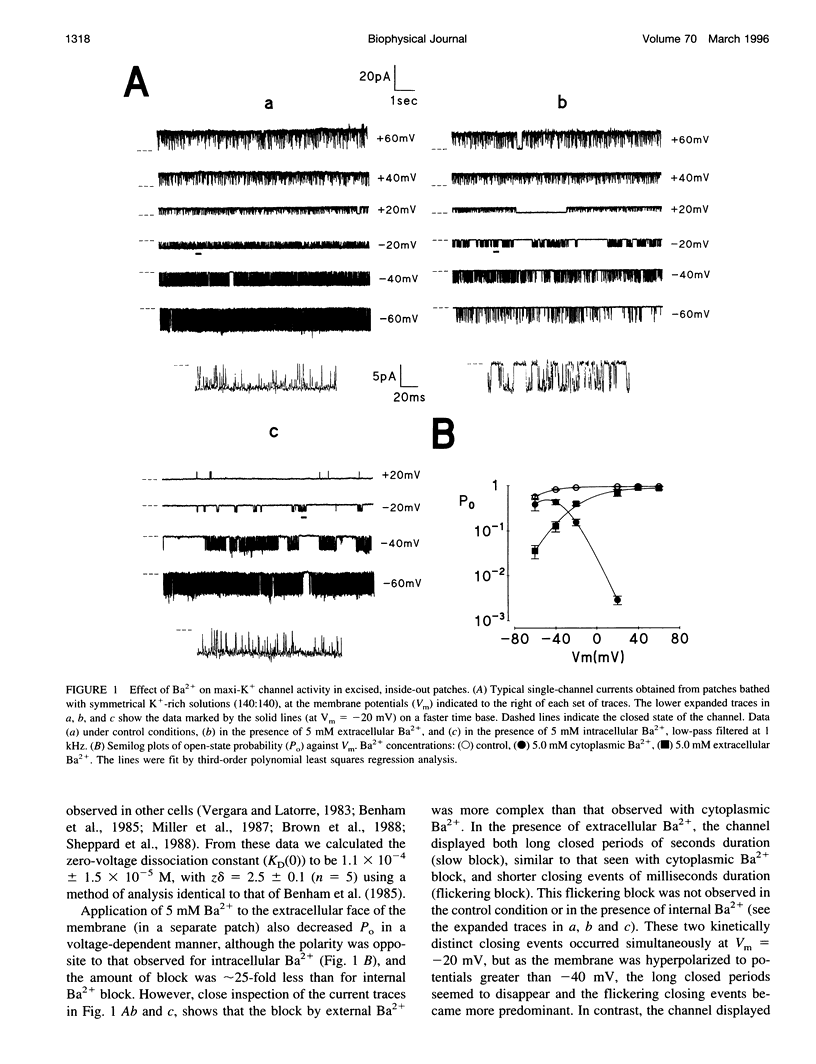
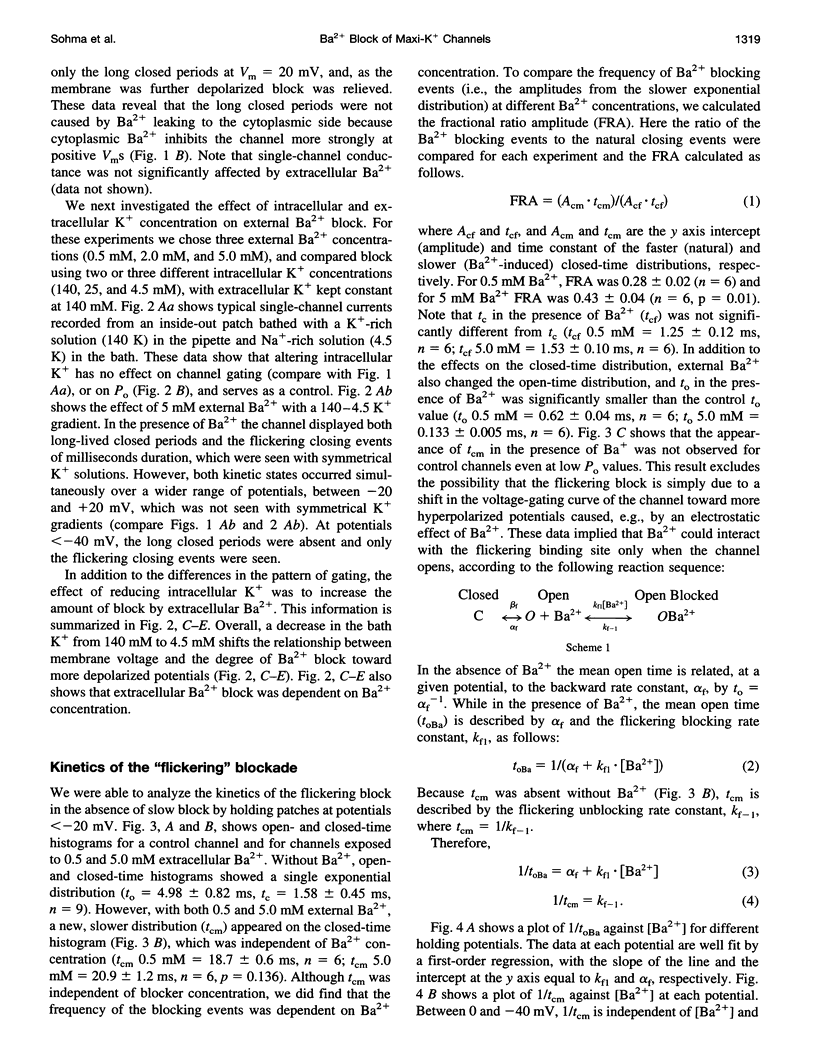
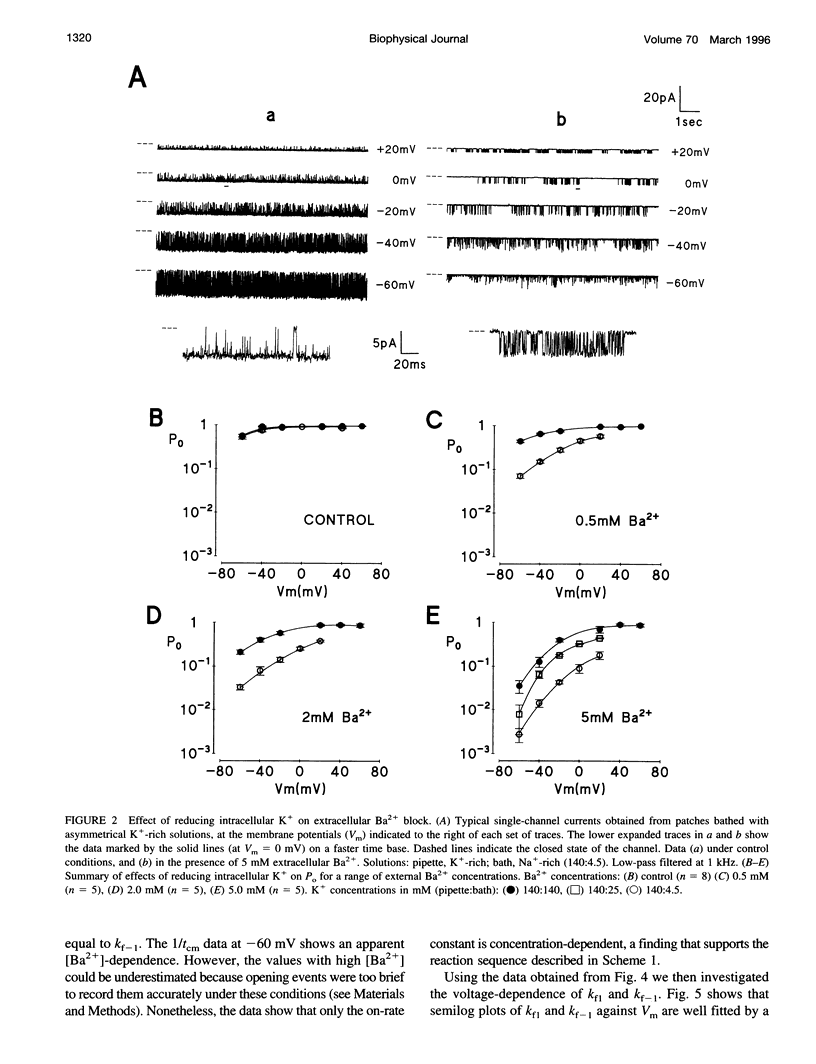
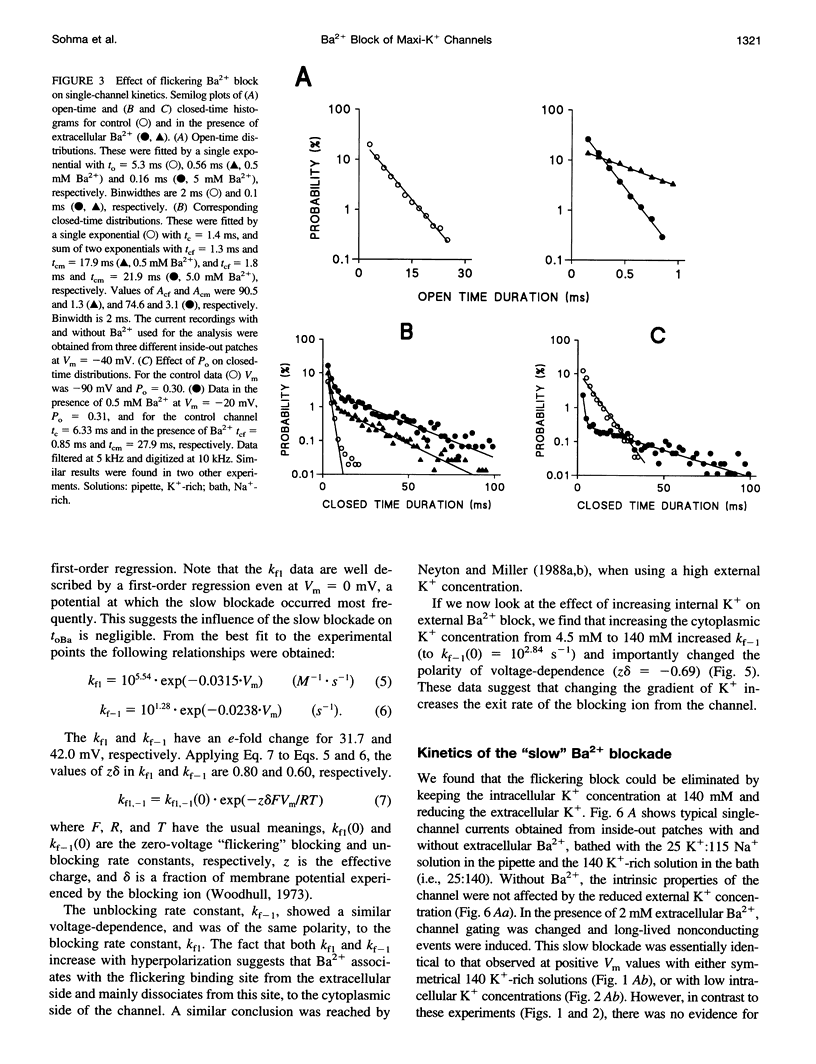
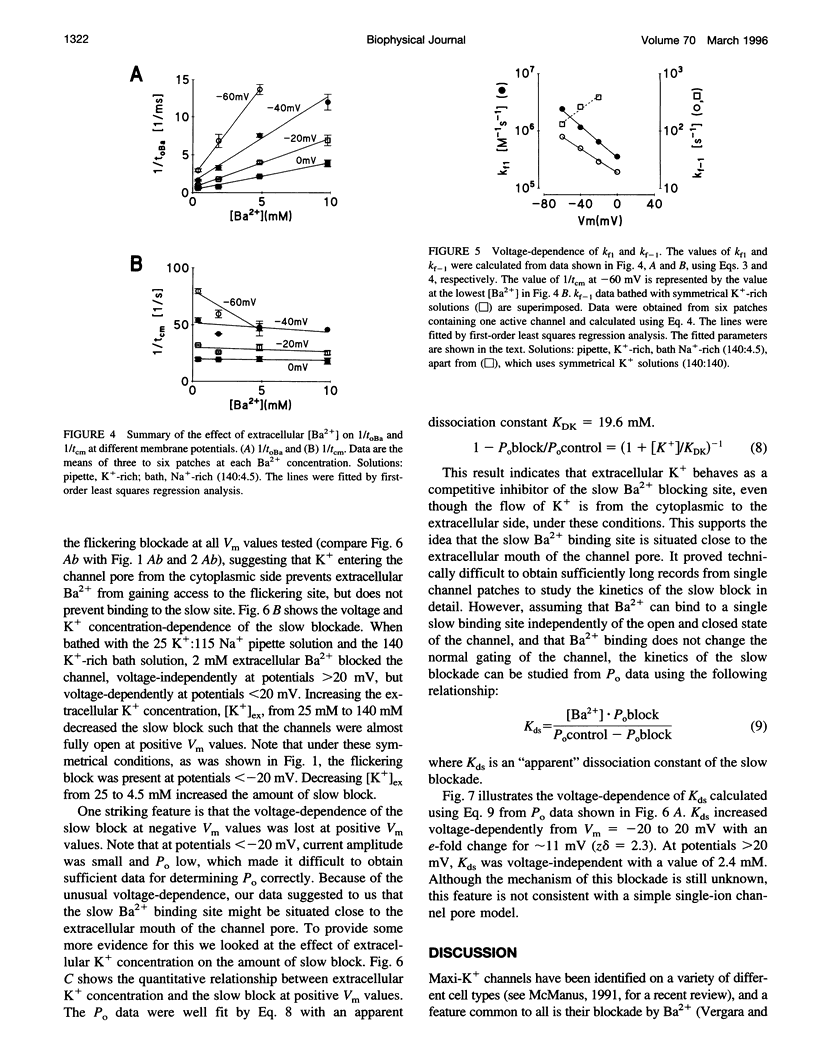
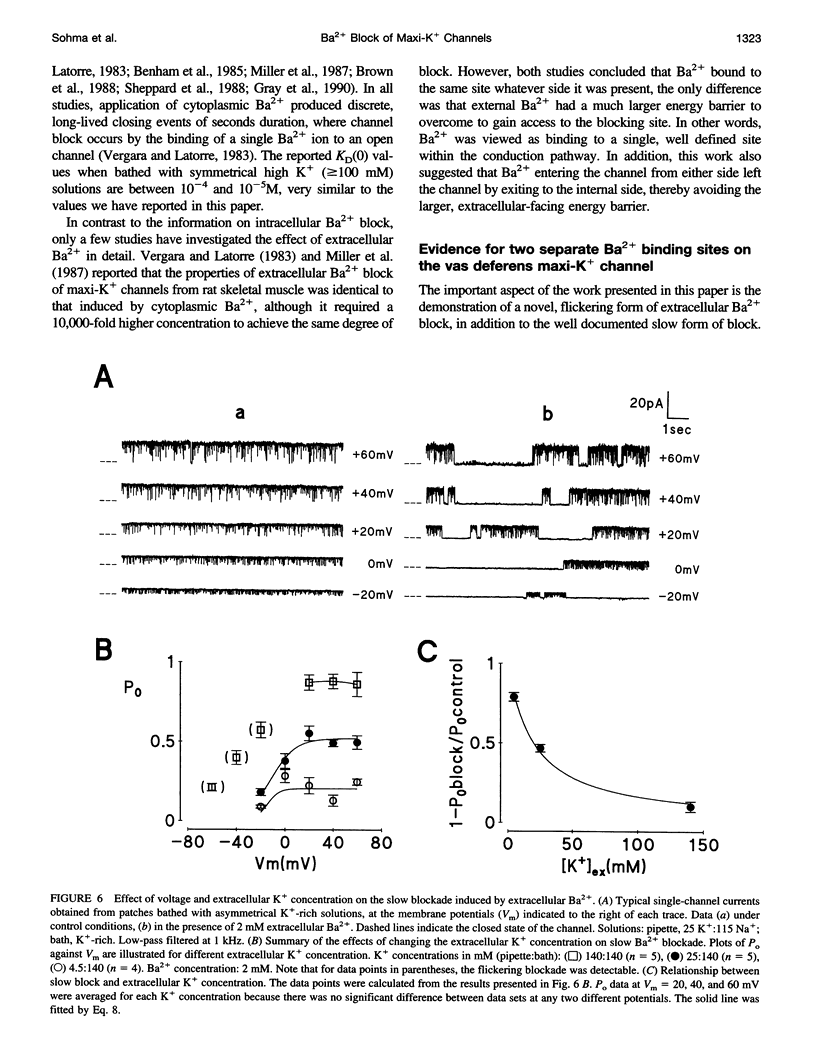
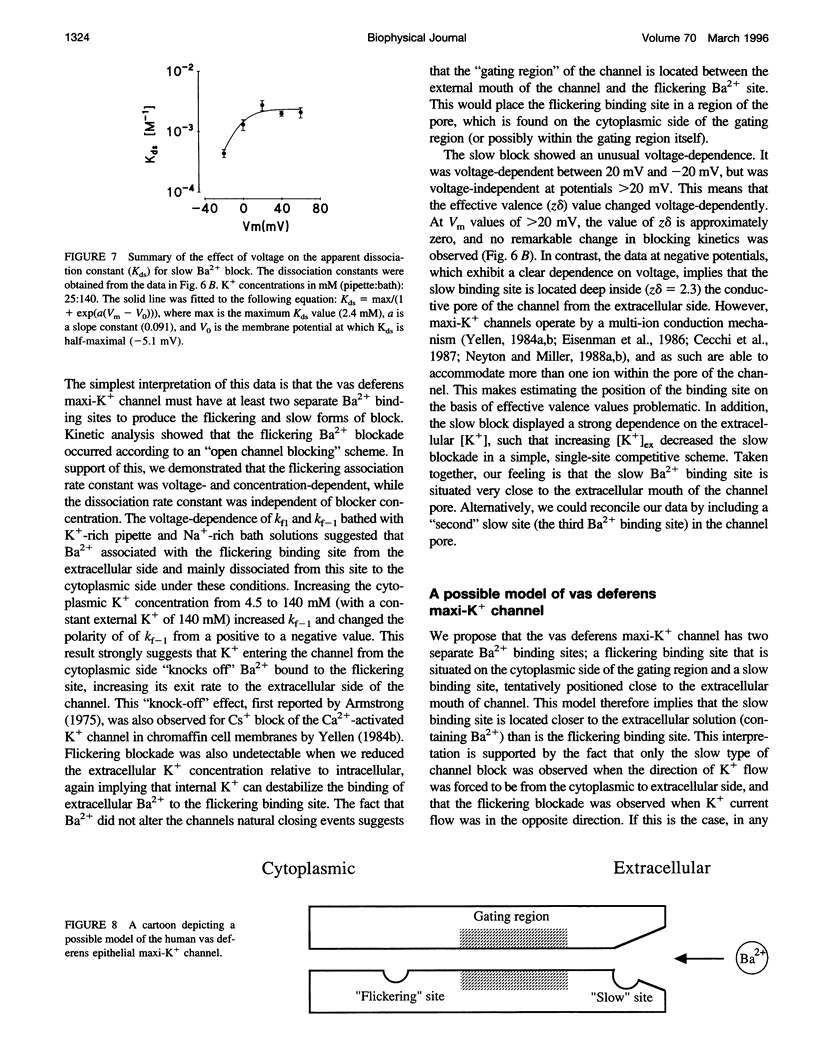
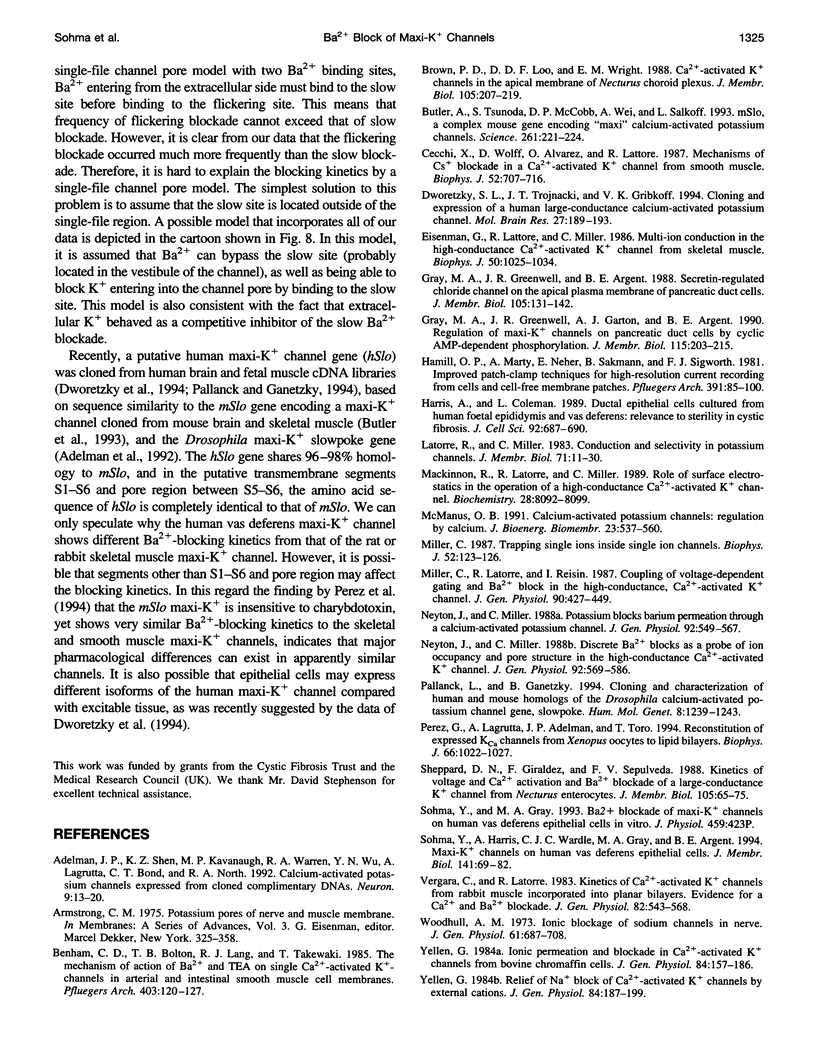
Selected References
These references are in PubMed. This may not be the complete list of references from this article.
- Armstrong C. M. Potassium pores of nerve and muscle membranes. Membranes. 1975;3:325–358. [PubMed] [Google Scholar]
- Benham C. D., Bolton T. B., Lang R. J., Takewaki T. The mechanism of action of Ba2+ and TEA on single Ca2+-activated K+ -channels in arterial and intestinal smooth muscle cell membranes. Pflugers Arch. 1985 Feb;403(2):120–127. doi: 10.1007/BF00584088. [DOI] [PubMed] [Google Scholar]
- Brown P. D., Loo D. D., Wright E. M. Ca2+-activated K+ channels in the apical membrane of Necturus choroid plexus. J Membr Biol. 1988 Nov;105(3):207–219. doi: 10.1007/BF01870998. [DOI] [PubMed] [Google Scholar]
- Butler A., Tsunoda S., McCobb D. P., Wei A., Salkoff L. mSlo, a complex mouse gene encoding "maxi" calcium-activated potassium channels. Science. 1993 Jul 9;261(5118):221–224. doi: 10.1126/science.7687074. [DOI] [PubMed] [Google Scholar]
- Cecchi X., Wolff D., Alvarez O., Latorre R. Mechanisms of Cs+ blockade in a Ca2+-activated K+ channel from smooth muscle. Biophys J. 1987 Nov;52(5):707–716. doi: 10.1016/S0006-3495(87)83265-4. [DOI] [PMC free article] [PubMed] [Google Scholar]
- Dworetzky S. I., Trojnacki J. T., Gribkoff V. K. Cloning and expression of a human large-conductance calcium-activated potassium channel. Brain Res Mol Brain Res. 1994 Nov;27(1):189–193. doi: 10.1016/0169-328x(94)90203-8. [DOI] [PubMed] [Google Scholar]
- Eisenman G., Latorre R., Miller C. Multi-ion conduction and selectivity in the high-conductance Ca++-activated K+ channel from skeletal muscle. Biophys J. 1986 Dec;50(6):1025–1034. doi: 10.1016/S0006-3495(86)83546-9. [DOI] [PMC free article] [PubMed] [Google Scholar]
- Gray M. A., Greenwell J. R., Argent B. E. Secretin-regulated chloride channel on the apical plasma membrane of pancreatic duct cells. J Membr Biol. 1988 Oct;105(2):131–142. doi: 10.1007/BF02009166. [DOI] [PubMed] [Google Scholar]
- Gray M. A., Greenwell J. R., Garton A. J., Argent B. E. Regulation of maxi-K+ channels on pancreatic duct cells by cyclic AMP-dependent phosphorylation. J Membr Biol. 1990 May;115(3):203–215. doi: 10.1007/BF01868636. [DOI] [PubMed] [Google Scholar]
- Hamill O. P., Marty A., Neher E., Sakmann B., Sigworth F. J. Improved patch-clamp techniques for high-resolution current recording from cells and cell-free membrane patches. Pflugers Arch. 1981 Aug;391(2):85–100. doi: 10.1007/BF00656997. [DOI] [PubMed] [Google Scholar]
- Harris A., Coleman L. Ductal epithelial cells cultured from human foetal epididymis and vas deferens: relevance to sterility in cystic fibrosis. J Cell Sci. 1989 Apr;92(Pt 4):687–690. doi: 10.1242/jcs.92.4.687. [DOI] [PubMed] [Google Scholar]
- Latorre R., Miller C. Conduction and selectivity in potassium channels. J Membr Biol. 1983;71(1-2):11–30. doi: 10.1007/BF01870671. [DOI] [PubMed] [Google Scholar]
- MacKinnon R., Latorre R., Miller C. Role of surface electrostatics in the operation of a high-conductance Ca2+-activated K+ channel. Biochemistry. 1989 Oct 3;28(20):8092–8099. doi: 10.1021/bi00446a020. [DOI] [PubMed] [Google Scholar]
- McManus O. B. Calcium-activated potassium channels: regulation by calcium. J Bioenerg Biomembr. 1991 Aug;23(4):537–560. doi: 10.1007/BF00785810. [DOI] [PubMed] [Google Scholar]
- Miller C., Latorre R., Reisin I. Coupling of voltage-dependent gating and Ba++ block in the high-conductance, Ca++-activated K+ channel. J Gen Physiol. 1987 Sep;90(3):427–449. doi: 10.1085/jgp.90.3.427. [DOI] [PMC free article] [PubMed] [Google Scholar]
- Miller C. Trapping single ions inside single ion channels. Biophys J. 1987 Jul;52(1):123–126. doi: 10.1016/S0006-3495(87)83196-X. [DOI] [PMC free article] [PubMed] [Google Scholar]
- Neyton J., Miller C. Discrete Ba2+ block as a probe of ion occupancy and pore structure in the high-conductance Ca2+ -activated K+ channel. J Gen Physiol. 1988 Nov;92(5):569–586. doi: 10.1085/jgp.92.5.569. [DOI] [PMC free article] [PubMed] [Google Scholar]
- Neyton J., Miller C. Potassium blocks barium permeation through a calcium-activated potassium channel. J Gen Physiol. 1988 Nov;92(5):549–567. doi: 10.1085/jgp.92.5.549. [DOI] [PMC free article] [PubMed] [Google Scholar]
- Pallanck L., Ganetzky B. Cloning and characterization of human and mouse homologs of the Drosophila calcium-activated potassium channel gene, slowpoke. Hum Mol Genet. 1994 Aug;3(8):1239–1243. doi: 10.1093/hmg/3.8.1239. [DOI] [PubMed] [Google Scholar]
- Pérez G., Lagrutta A., Adelman J. P., Toro L. Reconstitution of expressed KCa channels from Xenopus oocytes to lipid bilayers. Biophys J. 1994 Apr;66(4):1022–1027. doi: 10.1016/S0006-3495(94)80883-5. [DOI] [PMC free article] [PubMed] [Google Scholar]
- Sheppard D. N., Giraldez F., Sepúlveda F. V. Kinetics of voltage- and Ca2+ activation and Ba2+ blockade of a large-conductance K+ channel from Necturus enterocytes. J Membr Biol. 1988 Oct;105(1):65–75. doi: 10.1007/BF01871107. [DOI] [PubMed] [Google Scholar]
- Sohma Y., Harris A., Wardle C. J., Gray M. A., Argent B. E. Maxi K+ channels on human vas deferens epithelial cells. J Membr Biol. 1994 Jul;141(1):69–82. doi: 10.1007/BF00232875. [DOI] [PubMed] [Google Scholar]
- Vergara C., Latorre R. Kinetics of Ca2+-activated K+ channels from rabbit muscle incorporated into planar bilayers. Evidence for a Ca2+ and Ba2+ blockade. J Gen Physiol. 1983 Oct;82(4):543–568. doi: 10.1085/jgp.82.4.543. [DOI] [PMC free article] [PubMed] [Google Scholar]
- Woodhull A. M. Ionic blockage of sodium channels in nerve. J Gen Physiol. 1973 Jun;61(6):687–708. doi: 10.1085/jgp.61.6.687. [DOI] [PMC free article] [PubMed] [Google Scholar]
- Yellen G. Ionic permeation and blockade in Ca2+-activated K+ channels of bovine chromaffin cells. J Gen Physiol. 1984 Aug;84(2):157–186. doi: 10.1085/jgp.84.2.157. [DOI] [PMC free article] [PubMed] [Google Scholar]
- Yellen G. Relief of Na+ block of Ca2+-activated K+ channels by external cations. J Gen Physiol. 1984 Aug;84(2):187–199. doi: 10.1085/jgp.84.2.187. [DOI] [PMC free article] [PubMed] [Google Scholar]