Abstract
An isotonic control system for studying dynamic properties of single myofibrils was developed to evaluate the change of sarcomere lengths in glycerinated skeletal myofibrils under conditions of spontaneous oscillatory contraction (SPOC) in the presence of inorganic phosphate and a high ADP-to-ATP ratio. Sarcomere length oscillated spontaneously with a peak-to-peak amplitude of about 0.5 microns under isotonic conditions in which the external loads were maintained constant at values between 1.5 x 10(4) and 3.5 x 10(4) N/m2. The shortening and yielding of sarcomeres occurred in concert, in contrast to the previously reported conditions (isomeric or auxotonic) under which the myofibrillar tension is allowed to oscillate. This synchronous SPOC appears to be at a higher level of synchrony than in the organized state of SPOC previously observed under auxotonic conditions. The period of sarcomere length oscillation did not largely depend on external load. The active tension under SPOC conditions increased as the sarcomere length increased from 2.1 to 3.2 microns, although it was still smaller than the tension under normal Ca2+ contraction (which is on the order of 10(5) N/m2). The synchronous SPOC implies that there is a mechanism for transmitting information between sarcomeres such that the state of activation of sarcomeres is affected by the state of adjacent sarcomeres. We conclude that the change of myofibrillar tension is not responsible for the SPOC of each sarcomere but that it affects the level of synchrony of sarcomere oscillations.
Full text
PDF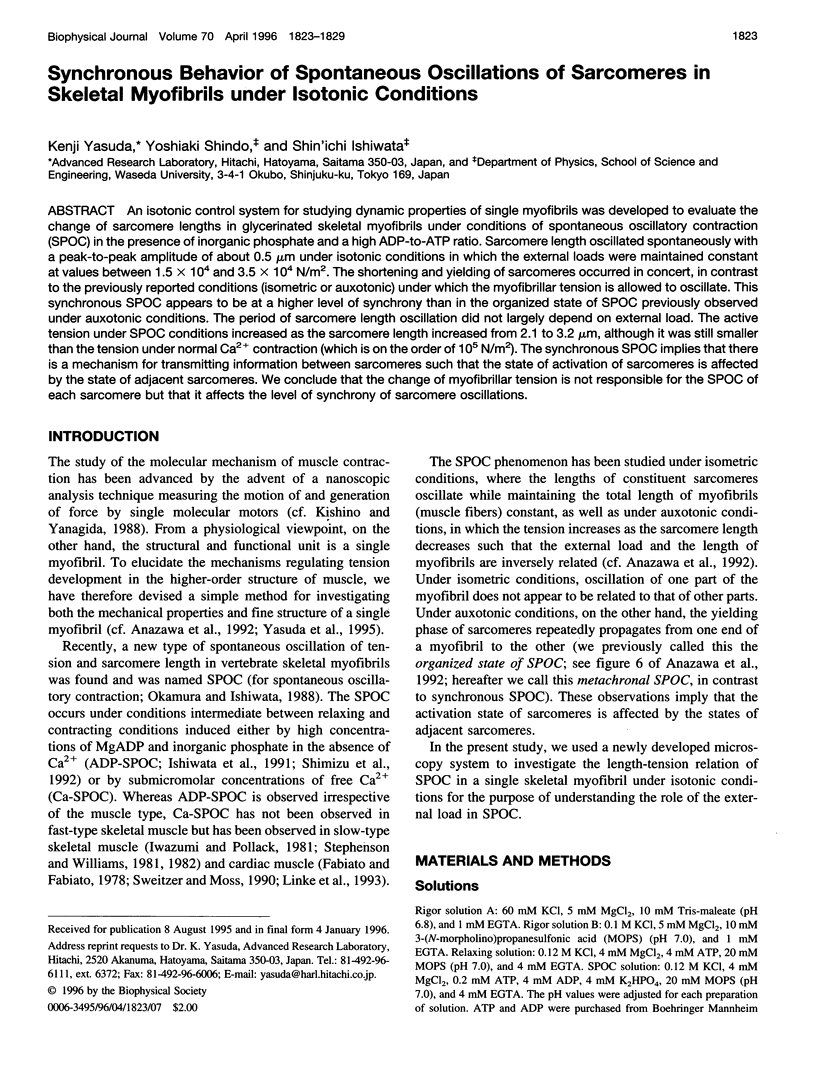
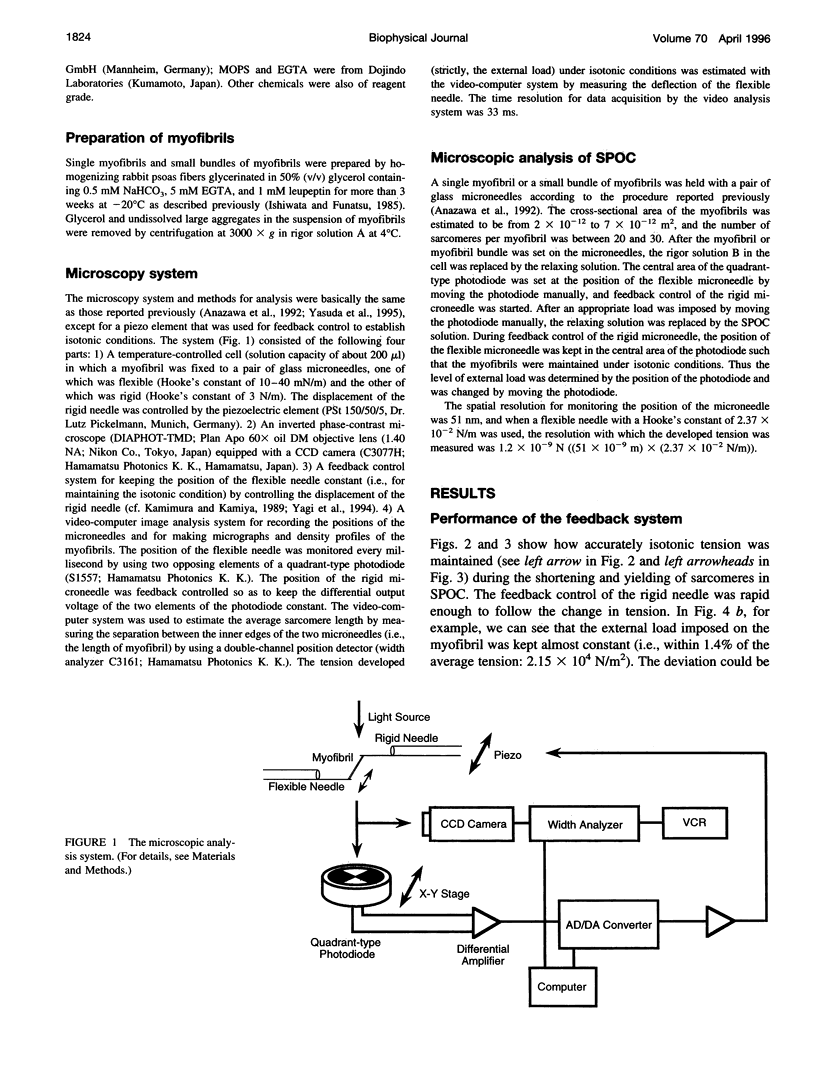
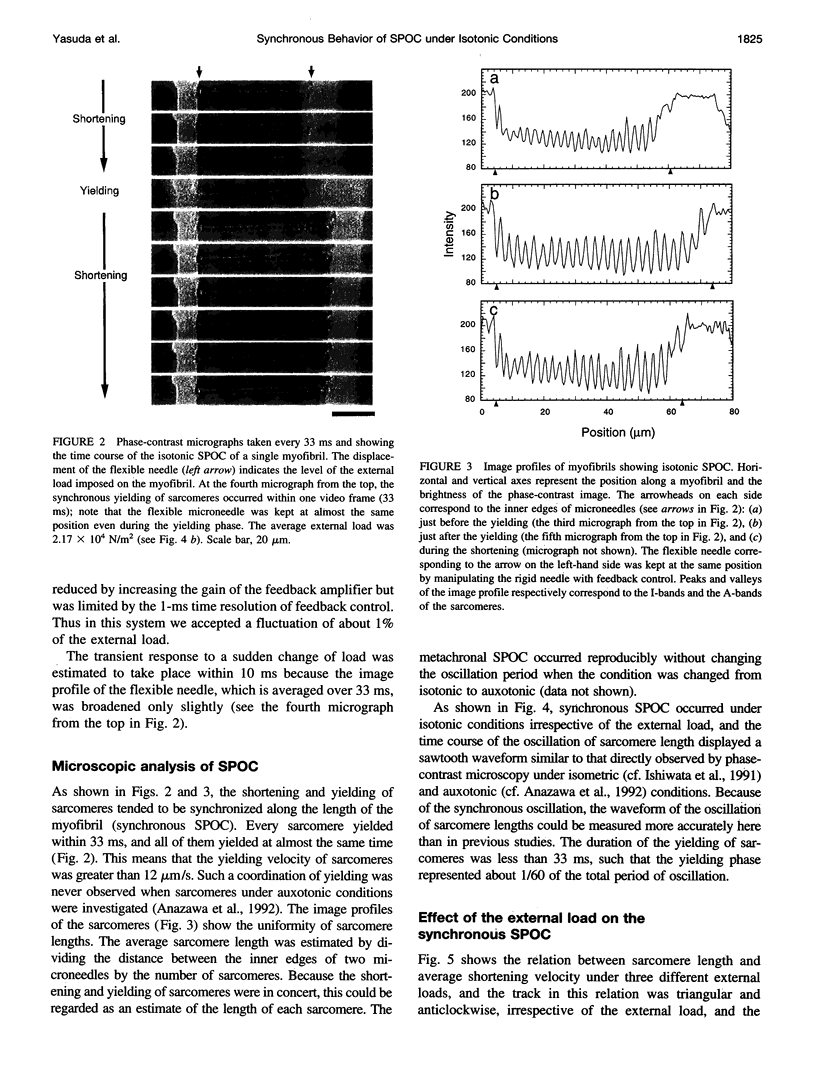
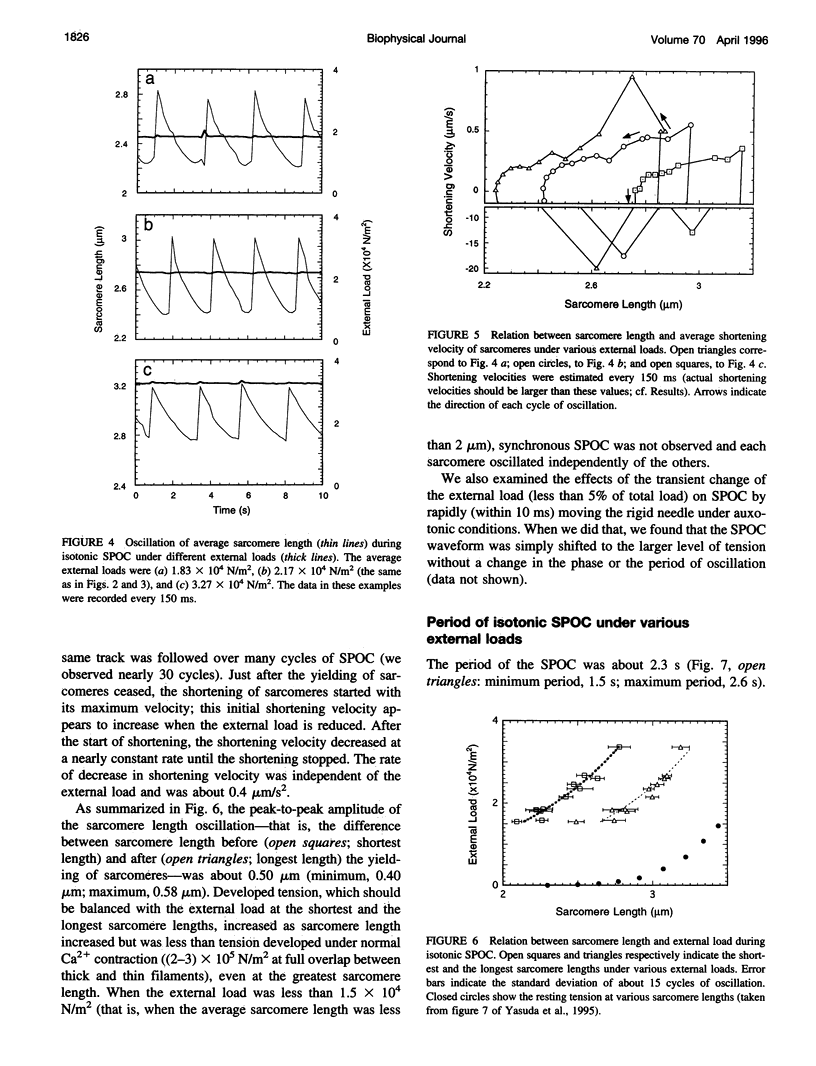
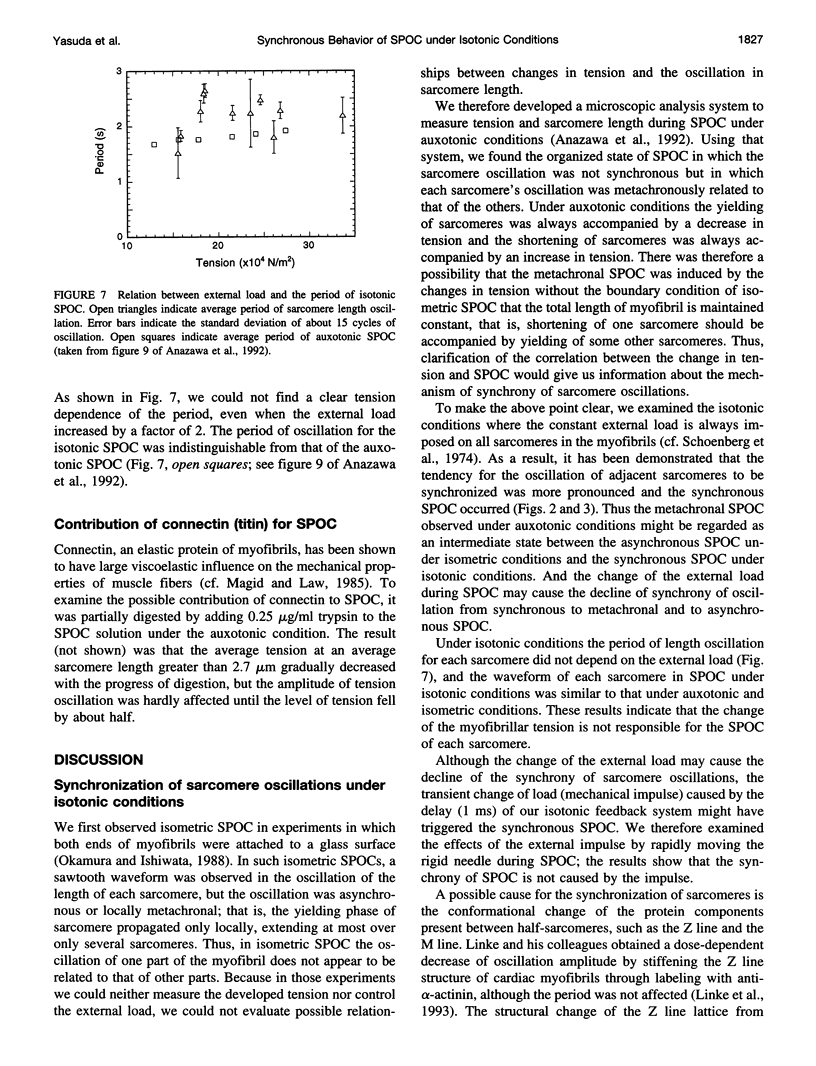
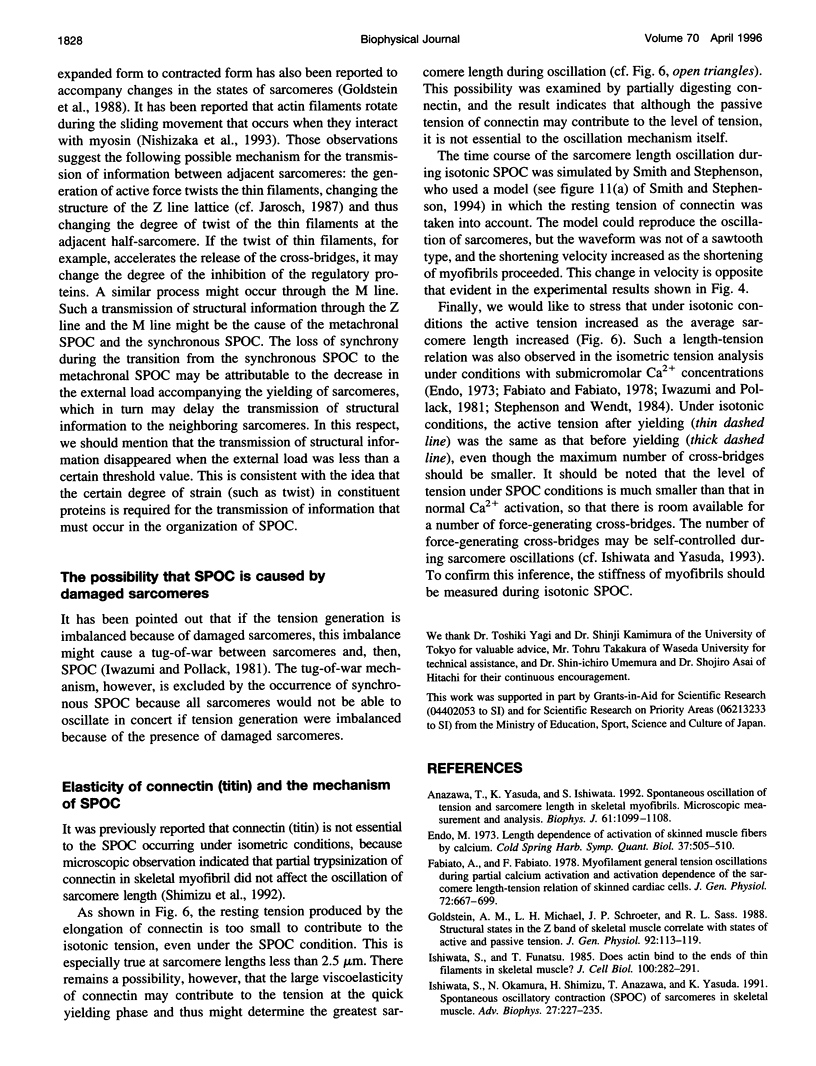
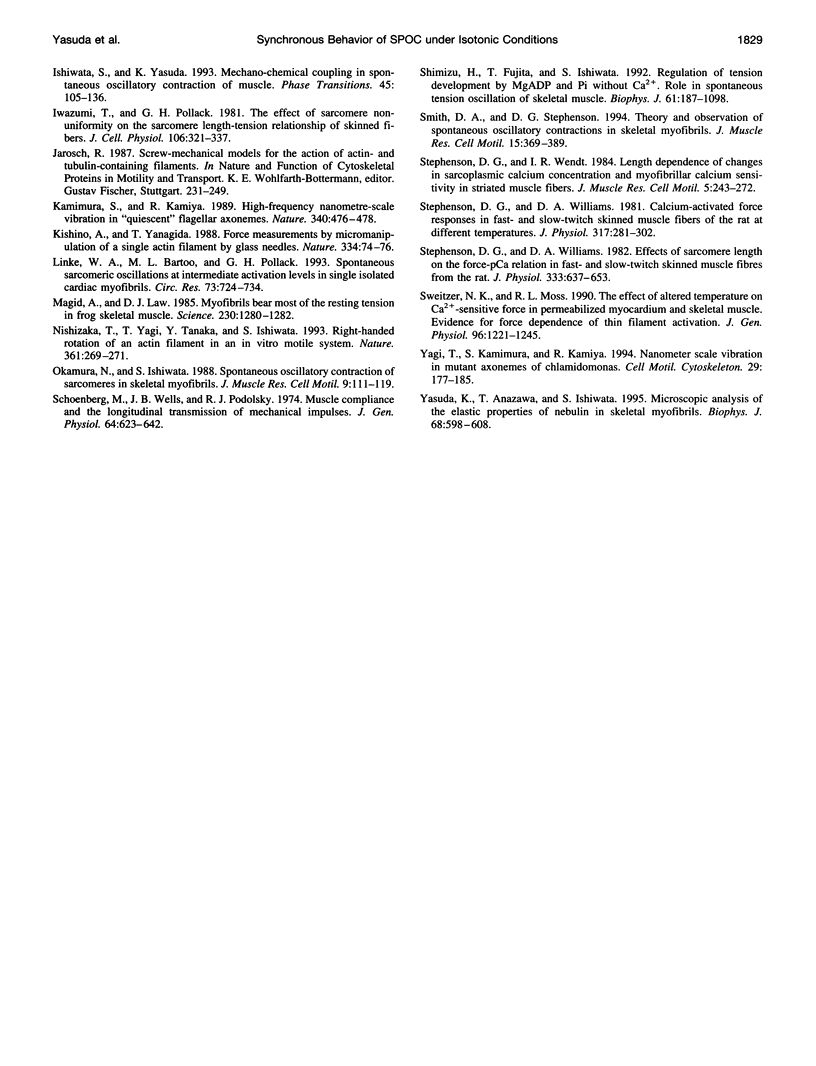
Images in this article
Selected References
These references are in PubMed. This may not be the complete list of references from this article.
- Anazawa T., Yasuda K., Ishiwata S. Spontaneous oscillation of tension and sarcomere length in skeletal myofibrils. Microscopic measurement and analysis. Biophys J. 1992 May;61(5):1099–1108. doi: 10.1016/S0006-3495(92)81919-7. [DOI] [PMC free article] [PubMed] [Google Scholar]
- Fabiato A., Fabiato F. Myofilament-generated tension oscillations during partial calcium activation and activation dependence of the sarcomere length-tension relation of skinned cardiac cells. J Gen Physiol. 1978 Nov;72(5):667–699. doi: 10.1085/jgp.72.5.667. [DOI] [PMC free article] [PubMed] [Google Scholar]
- Goldstein M. A., Michael L. H., Schroeter J. P., Sass R. L. Structural states in the Z band of skeletal muscle correlate with states of active and passive tension. J Gen Physiol. 1988 Jul;92(1):113–119. doi: 10.1085/jgp.92.1.113. [DOI] [PMC free article] [PubMed] [Google Scholar]
- Ishiwata S., Funatsu T. Does actin bind to the ends of thin filaments in skeletal muscle? J Cell Biol. 1985 Jan;100(1):282–291. doi: 10.1083/jcb.100.1.282. [DOI] [PMC free article] [PubMed] [Google Scholar]
- Ishiwata S., Okamura N., Shimizu H., Anazawa T., Yasuda K. Spontaneous oscillatory contraction (SPOC) of sarcomeres in skeletal muscle. Adv Biophys. 1991;27:227–235. doi: 10.1016/0065-227x(91)90022-6. [DOI] [PubMed] [Google Scholar]
- Iwazumi T., Pollack G. H. The effect of sarcomere non-uniformity on the sarcomere length-tension relationship of skinned fibers. J Cell Physiol. 1981 Mar;106(3):321–337. doi: 10.1002/jcp.1041060302. [DOI] [PubMed] [Google Scholar]
- Kamimura S., Kamiya R. High-frequency nanometre-scale vibration in 'quiescent' flagellar axonemes. Nature. 1989 Aug 10;340(6233):476–478. doi: 10.1038/340476a0. [DOI] [PubMed] [Google Scholar]
- Kishino A., Yanagida T. Force measurements by micromanipulation of a single actin filament by glass needles. Nature. 1988 Jul 7;334(6177):74–76. doi: 10.1038/334074a0. [DOI] [PubMed] [Google Scholar]
- Linke W. A., Bartoo M. L., Pollack G. H. Spontaneous sarcomeric oscillations at intermediate activation levels in single isolated cardiac myofibrils. Circ Res. 1993 Oct;73(4):724–734. doi: 10.1161/01.res.73.4.724. [DOI] [PubMed] [Google Scholar]
- Magid A., Law D. J. Myofibrils bear most of the resting tension in frog skeletal muscle. Science. 1985 Dec 13;230(4731):1280–1282. doi: 10.1126/science.4071053. [DOI] [PubMed] [Google Scholar]
- Nishizaka T., Yagi T., Tanaka Y., Ishiwata S. Right-handed rotation of an actin filament in an in vitro motile system. Nature. 1993 Jan 21;361(6409):269–271. doi: 10.1038/361269a0. [DOI] [PubMed] [Google Scholar]
- Okamura N., Ishiwata S. Spontaneous oscillatory contraction of sarcomeres in skeletal myofibrils. J Muscle Res Cell Motil. 1988 Apr;9(2):111–119. doi: 10.1007/BF01773733. [DOI] [PubMed] [Google Scholar]
- Schoenberg M., Wells J. B., Podolsky R. J. Muscle compliance and the longitudinal transmission of mechanical impulses. J Gen Physiol. 1974 Dec;64(6):623–642. doi: 10.1085/jgp.64.6.623. [DOI] [PMC free article] [PubMed] [Google Scholar]
- Shimizu H., Fujita T., Ishiwata S. Regulation of tension development by MgADP and Pi without Ca2+. Role in spontaneous tension oscillation of skeletal muscle. Biophys J. 1992 May;61(5):1087–1098. doi: 10.1016/S0006-3495(92)81918-5. [DOI] [PMC free article] [PubMed] [Google Scholar]
- Smith D. A., Stephenson D. G. Theory and observation of spontaneous oscillatory contractions in skeletal myofibrils. J Muscle Res Cell Motil. 1994 Aug;15(4):369–389. doi: 10.1007/BF00122112. [DOI] [PubMed] [Google Scholar]
- Stephenson D. G., Wendt I. R. Length dependence of changes in sarcoplasmic calcium concentration and myofibrillar calcium sensitivity in striated muscle fibres. J Muscle Res Cell Motil. 1984 Jun;5(3):243–272. doi: 10.1007/BF00713107. [DOI] [PubMed] [Google Scholar]
- Stephenson D. G., Williams D. A. Calcium-activated force responses in fast- and slow-twitch skinned muscle fibres of the rat at different temperatures. J Physiol. 1981 Aug;317:281–302. doi: 10.1113/jphysiol.1981.sp013825. [DOI] [PMC free article] [PubMed] [Google Scholar]
- Stephenson D. G., Williams D. A. Effects of sarcomere length on the force-pCa relation in fast- and slow-twitch skinned muscle fibres from the rat. J Physiol. 1982 Dec;333:637–653. doi: 10.1113/jphysiol.1982.sp014473. [DOI] [PMC free article] [PubMed] [Google Scholar]
- Sweitzer N. K., Moss R. L. The effect of altered temperature on Ca2(+)-sensitive force in permeabilized myocardium and skeletal muscle. Evidence for force dependence of thin filament activation. J Gen Physiol. 1990 Dec;96(6):1221–1245. doi: 10.1085/jgp.96.6.1221. [DOI] [PMC free article] [PubMed] [Google Scholar]
- Yagi T., Kamimura S., Kamiya R. Nanometer scale vibration in mutant axonemes of Chlamydomonas. Cell Motil Cytoskeleton. 1994;29(2):177–185. doi: 10.1002/cm.970290209. [DOI] [PubMed] [Google Scholar]
- Yasuda K., Anazawa T., Ishiwata S. Microscopic analysis of the elastic properties of nebulin in skeletal myofibrils. Biophys J. 1995 Feb;68(2):598–608. doi: 10.1016/S0006-3495(95)80221-3. [DOI] [PMC free article] [PubMed] [Google Scholar]