Abstract
There are many proteins that interact simultaneously with two or more DNA sites that are separated along the DNA contour. These sites must be brought close together to form productive complexes with the proteins. We used Monte Carlo simulation of supercoiled DNA conformations to study the effect of supercoiling and DNA length on the juxtaposition of DNA sites, the angle between them, and the branching of the interwound superhelix. Branching decreases the probability of juxtaposition of two DNA sites but increases the probability of juxtaposition of three sites at branch points. We found that the number of superhelix branches increases linearly with the length of DNA from 3 to 20 kb. The simulations showed that for all contour distances between two sites, the juxtaposition probability in supercoiled DNA is two orders of magnitude higher than in relaxed DNA. Supercoiling also results in a strong asymmetry of the angular distribution of juxtaposed sites. The effect of supercoiling on site-specific recombination and the introduction of supercoils by DNA gyrase is discussed in the context of the simulation results.
Full text
PDF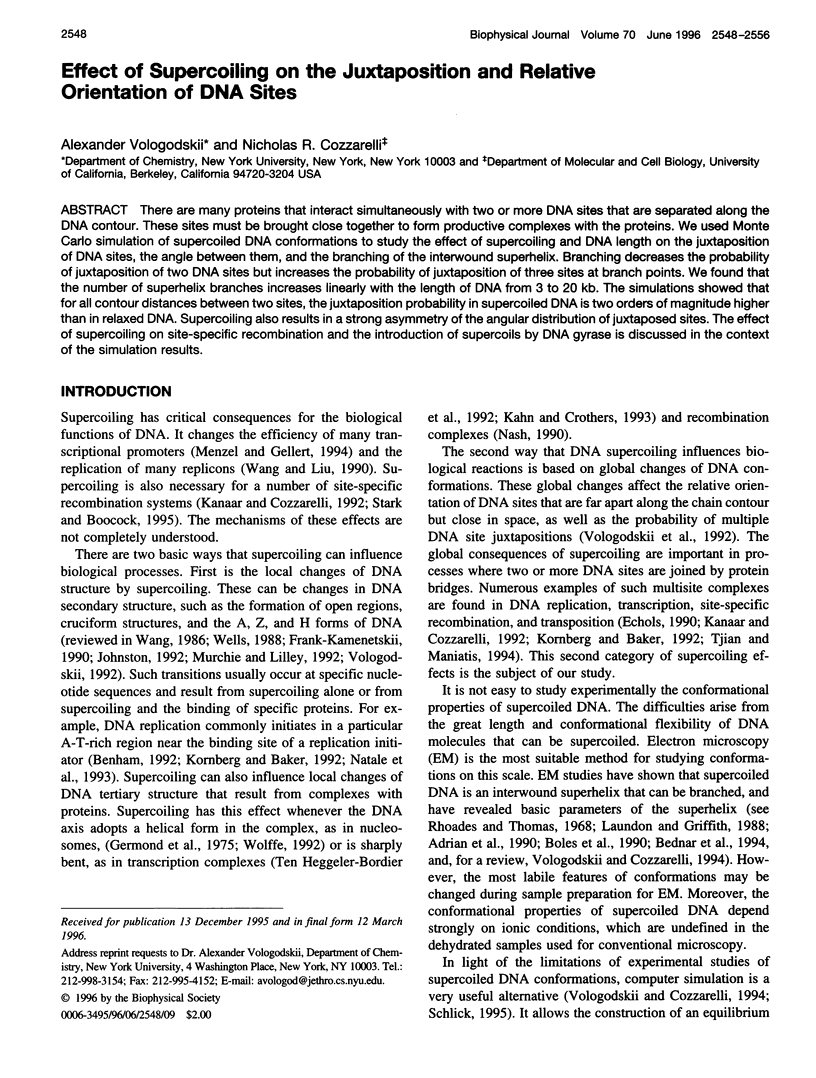
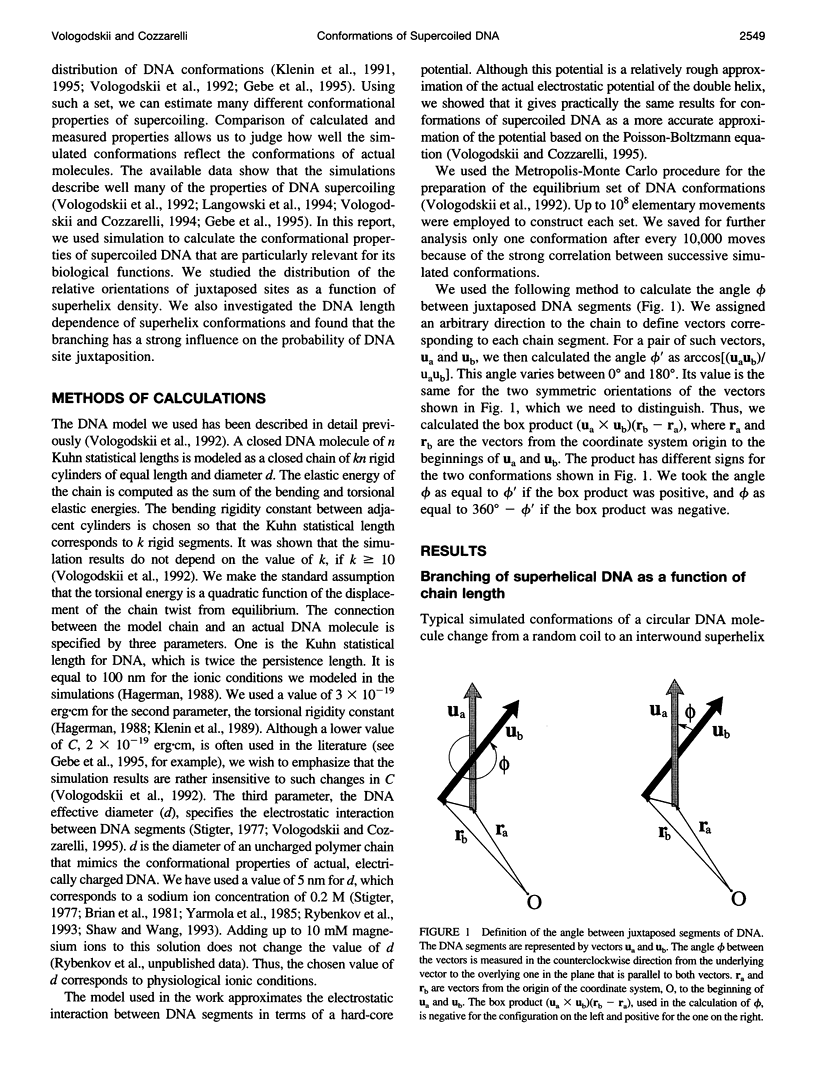
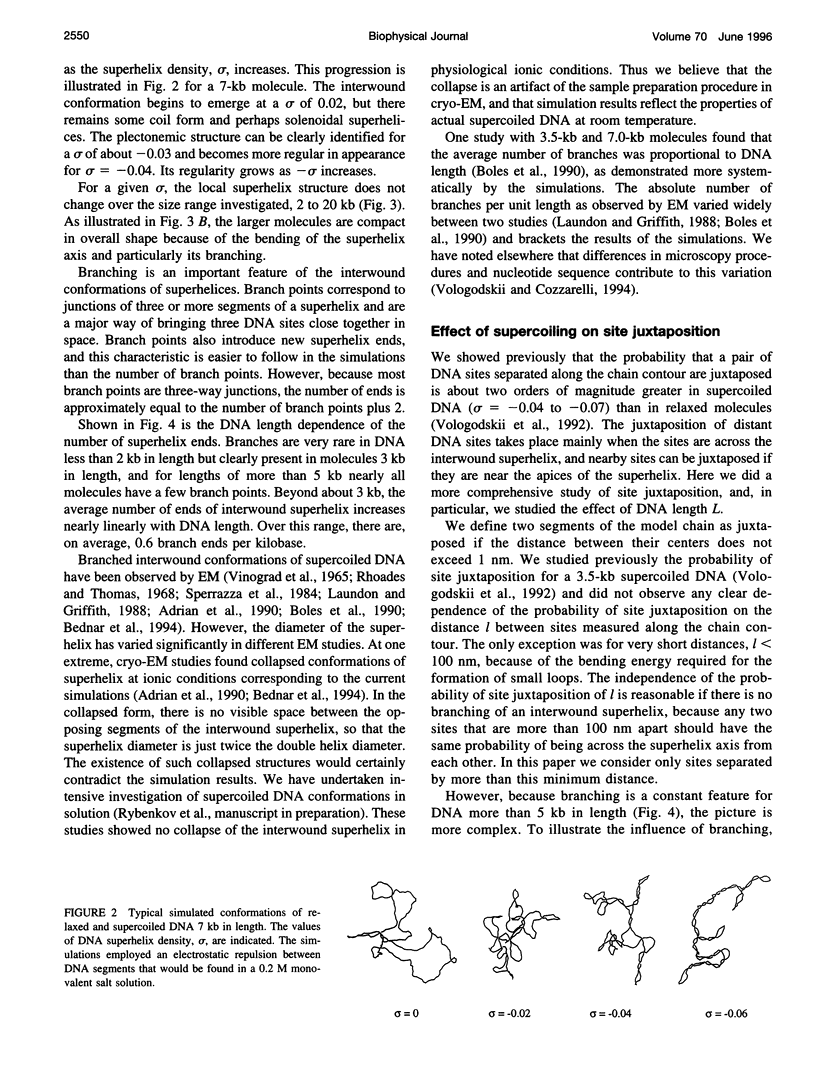
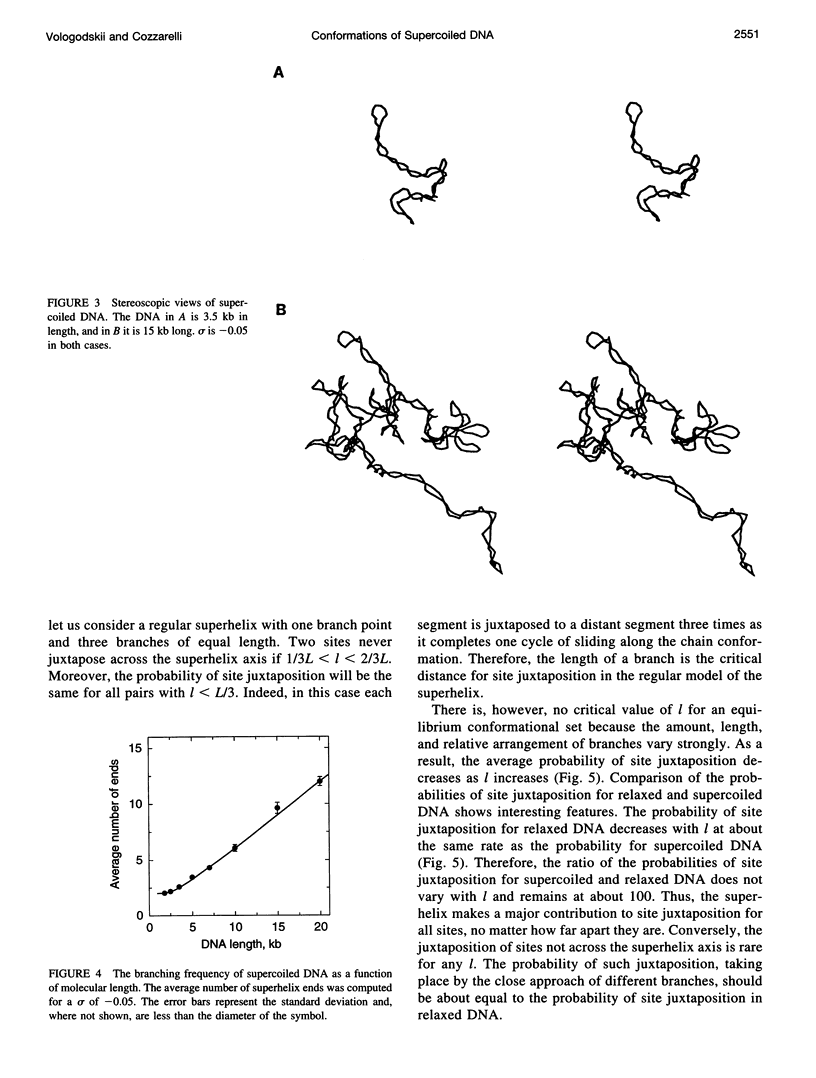
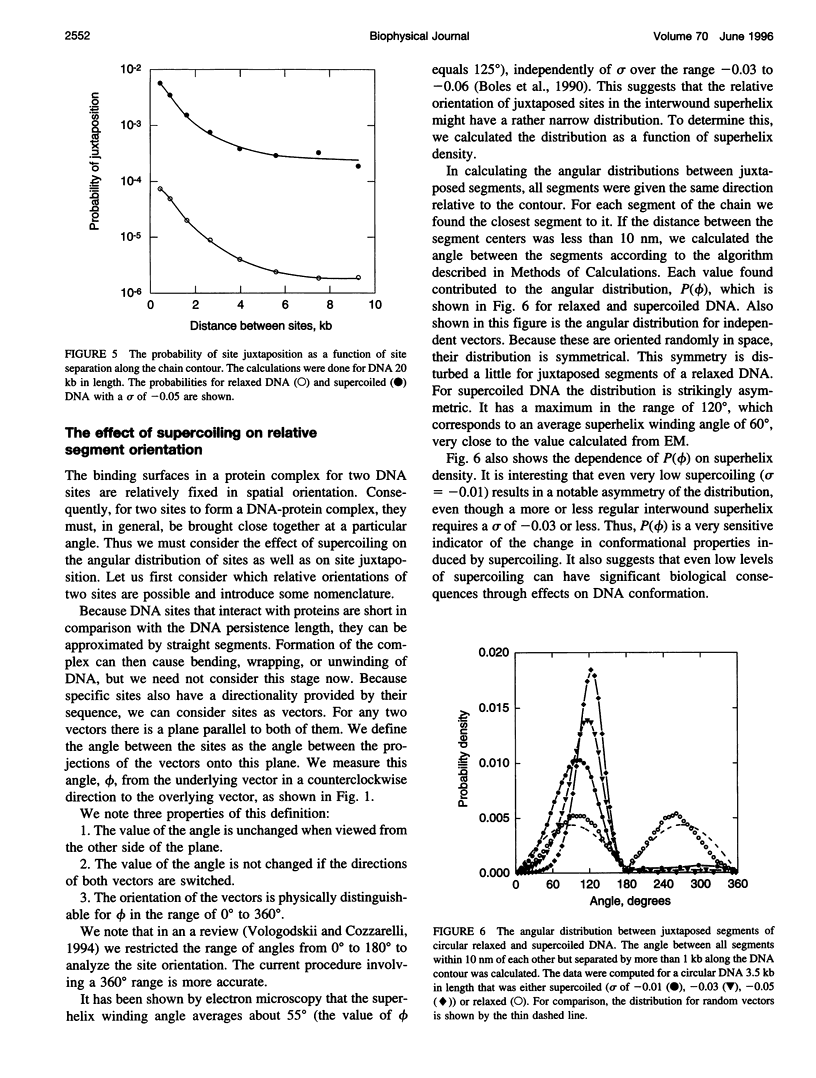
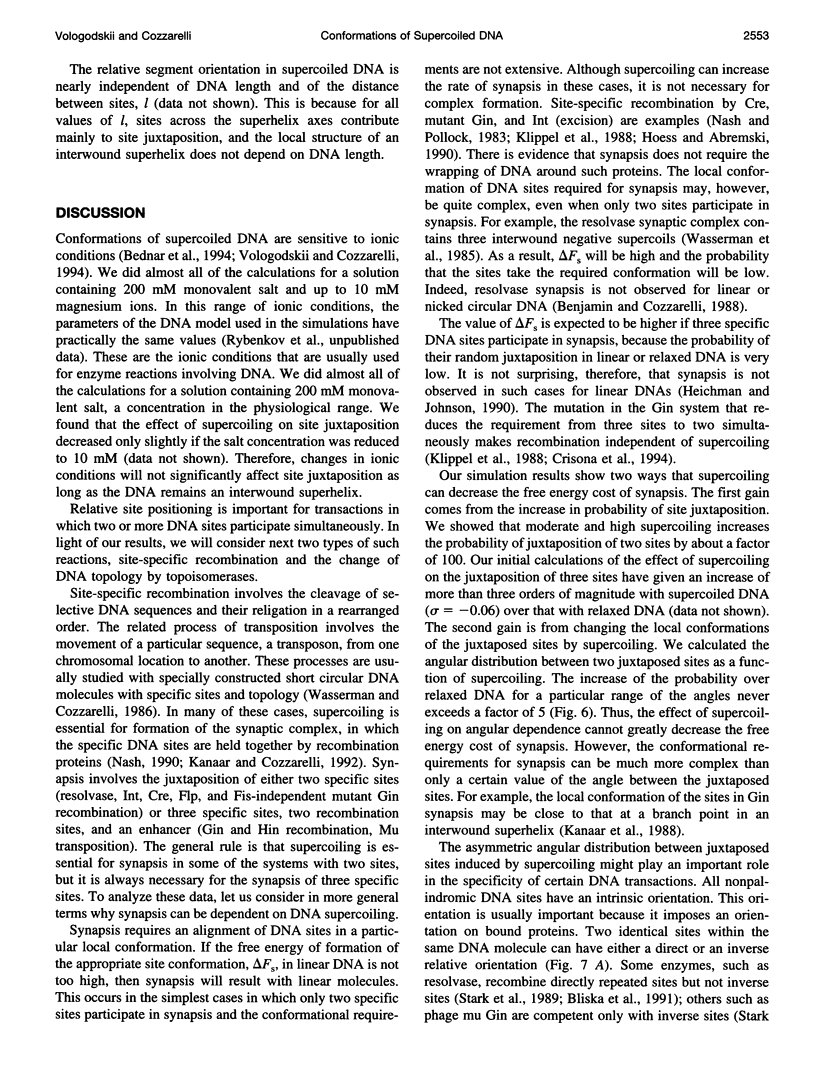
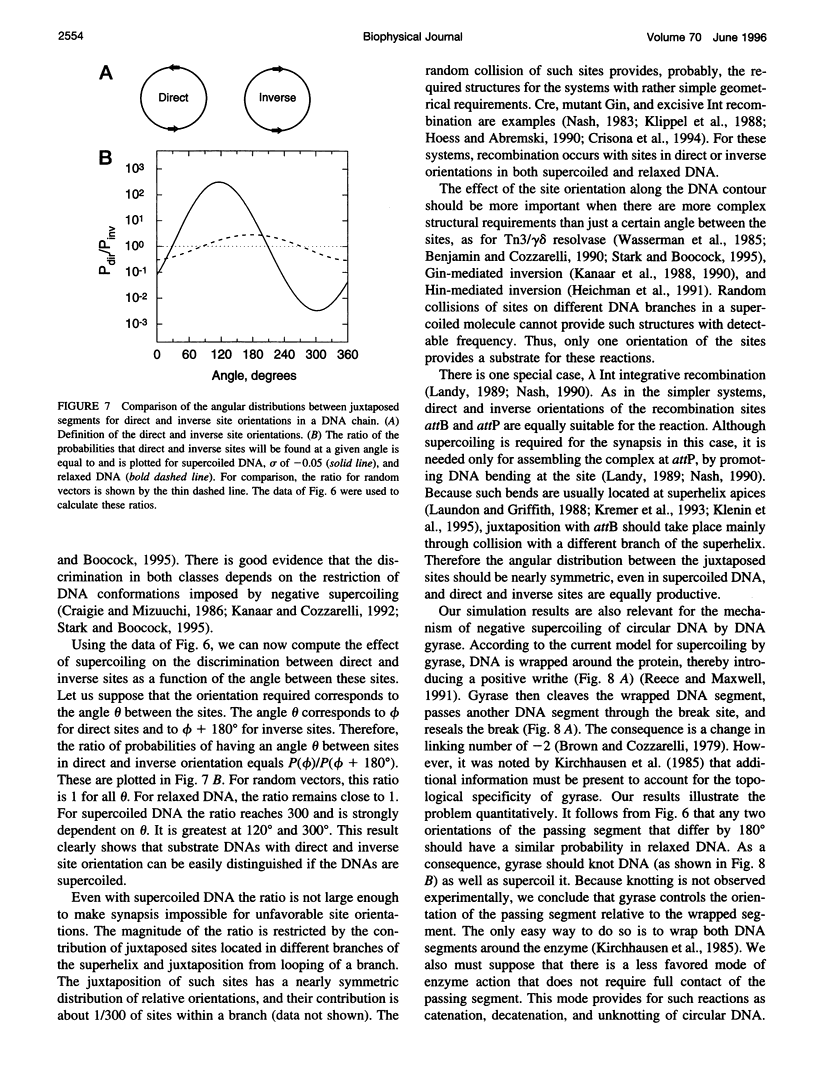
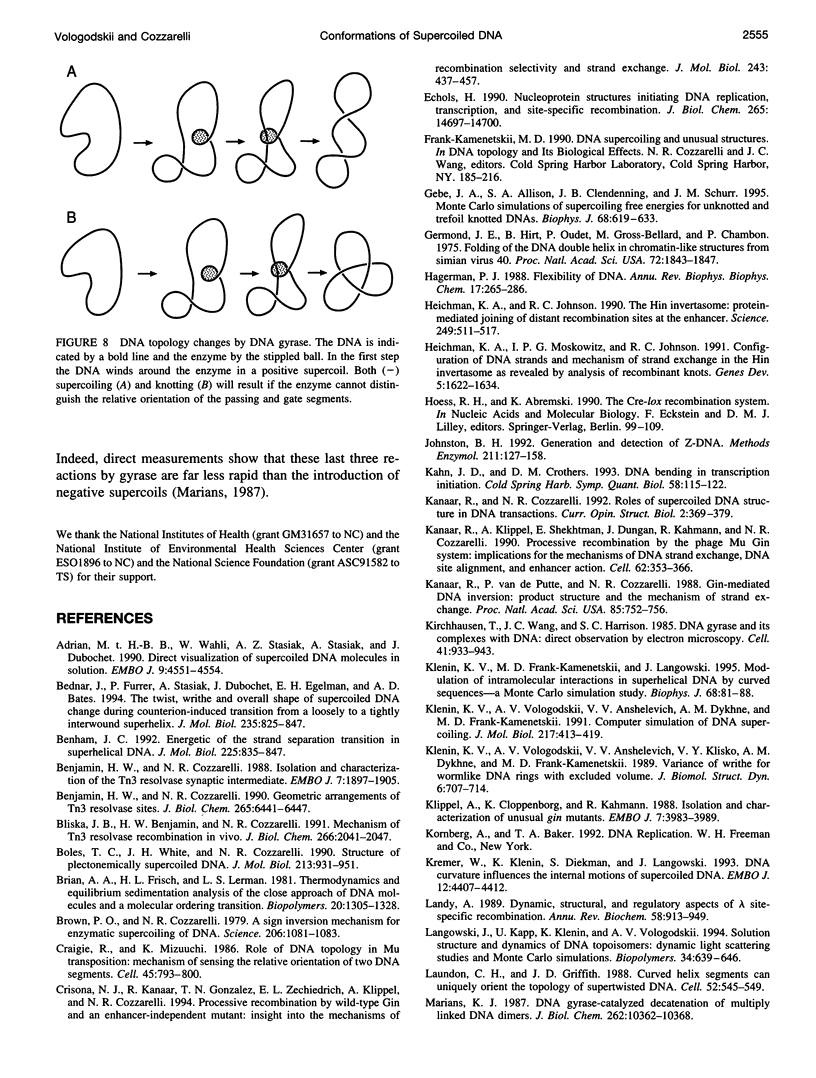
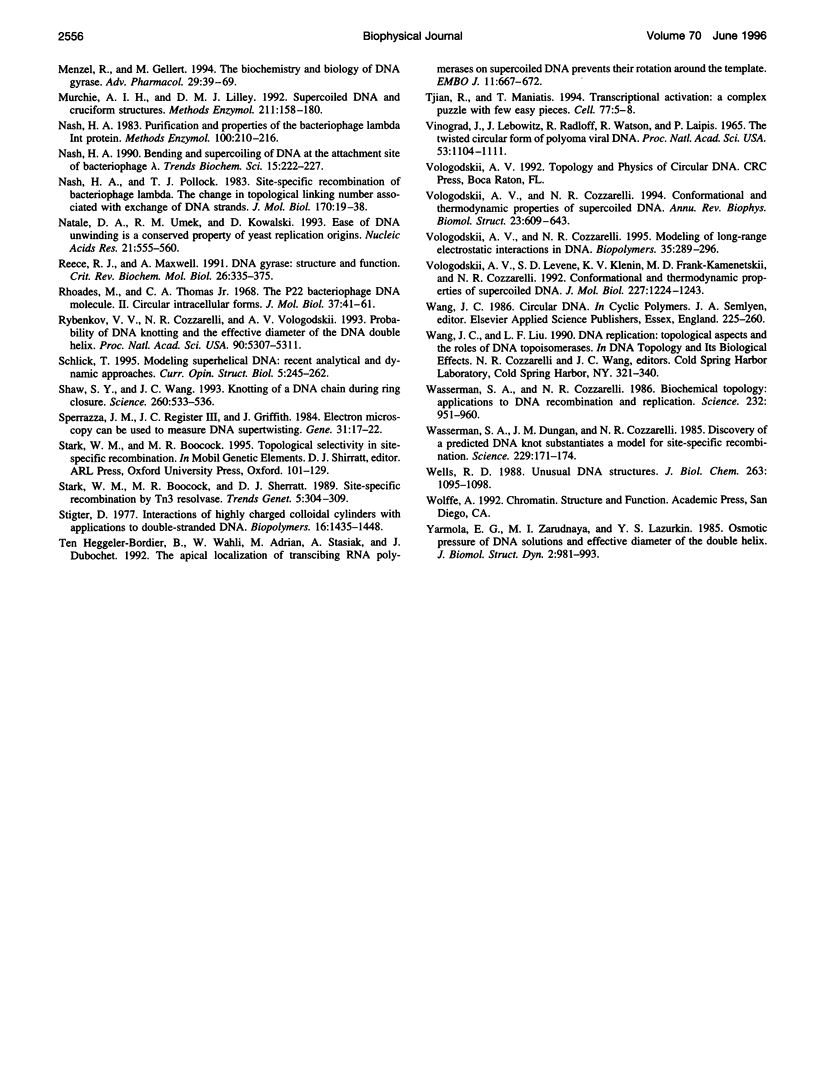
Selected References
These references are in PubMed. This may not be the complete list of references from this article.
- Adrian M., ten Heggeler-Bordier B., Wahli W., Stasiak A. Z., Stasiak A., Dubochet J. Direct visualization of supercoiled DNA molecules in solution. EMBO J. 1990 Dec;9(13):4551–4554. doi: 10.1002/j.1460-2075.1990.tb07907.x. [DOI] [PMC free article] [PubMed] [Google Scholar]
- Bednar J., Furrer P., Stasiak A., Dubochet J., Egelman E. H., Bates A. D. The twist, writhe and overall shape of supercoiled DNA change during counterion-induced transition from a loosely to a tightly interwound superhelix. Possible implications for DNA structure in vivo. J Mol Biol. 1994 Jan 21;235(3):825–847. doi: 10.1006/jmbi.1994.1042. [DOI] [PubMed] [Google Scholar]
- Benham C. J. Energetics of the strand separation transition in superhelical DNA. J Mol Biol. 1992 Jun 5;225(3):835–847. doi: 10.1016/0022-2836(92)90404-8. [DOI] [PubMed] [Google Scholar]
- Benjamin H. W., Cozzarelli N. R. Geometric arrangements of Tn3 resolvase sites. J Biol Chem. 1990 Apr 15;265(11):6441–6447. [PubMed] [Google Scholar]
- Benjamin H. W., Cozzarelli N. R. Isolation and characterization of the Tn3 resolvase synaptic intermediate. EMBO J. 1988 Jun;7(6):1897–1905. doi: 10.1002/j.1460-2075.1988.tb03023.x. [DOI] [PMC free article] [PubMed] [Google Scholar]
- Bliska J. B., Benjamin H. W., Cozzarelli N. R. Mechanism of Tn3 resolvase recombination in vivo. J Biol Chem. 1991 Feb 5;266(4):2041–2047. [PubMed] [Google Scholar]
- Boles T. C., White J. H., Cozzarelli N. R. Structure of plectonemically supercoiled DNA. J Mol Biol. 1990 Jun 20;213(4):931–951. doi: 10.1016/S0022-2836(05)80272-4. [DOI] [PubMed] [Google Scholar]
- Brian A. A., Frisch H. L., Lerman L. S. Thermodynamics and equilibrium sedimentation analysis of the close approach of DNA molecules and a molecular ordering transition. Biopolymers. 1981 Jun;20(6):1305–1328. doi: 10.1002/bip.1981.360200615. [DOI] [PubMed] [Google Scholar]
- Brown P. O., Cozzarelli N. R. A sign inversion mechanism for enzymatic supercoiling of DNA. Science. 1979 Nov 30;206(4422):1081–1083. doi: 10.1126/science.227059. [DOI] [PubMed] [Google Scholar]
- Craigie R., Mizuuchi K. Role of DNA topology in Mu transposition: mechanism of sensing the relative orientation of two DNA segments. Cell. 1986 Jun 20;45(6):793–800. doi: 10.1016/0092-8674(86)90554-4. [DOI] [PubMed] [Google Scholar]
- Crisona N. J., Kanaar R., Gonzalez T. N., Zechiedrich E. L., Klippel A., Cozzarelli N. R. Processive recombination by wild-type gin and an enhancer-independent mutant. Insight into the mechanisms of recombination selectivity and strand exchange. J Mol Biol. 1994 Oct 28;243(3):437–457. doi: 10.1006/jmbi.1994.1671. [DOI] [PubMed] [Google Scholar]
- Echols H. Nucleoprotein structures initiating DNA replication, transcription, and site-specific recombination. J Biol Chem. 1990 Sep 5;265(25):14697–14700. [PubMed] [Google Scholar]
- Gebe J. A., Allison S. A., Clendenning J. B., Schurr J. M. Monte Carlo simulations of supercoiling free energies for unknotted and trefoil knotted DNAs. Biophys J. 1995 Feb;68(2):619–633. doi: 10.1016/S0006-3495(95)80223-7. [DOI] [PMC free article] [PubMed] [Google Scholar]
- Germond J. E., Hirt B., Oudet P., Gross-Bellark M., Chambon P. Folding of the DNA double helix in chromatin-like structures from simian virus 40. Proc Natl Acad Sci U S A. 1975 May;72(5):1843–1847. doi: 10.1073/pnas.72.5.1843. [DOI] [PMC free article] [PubMed] [Google Scholar]
- Hagerman P. J. Flexibility of DNA. Annu Rev Biophys Biophys Chem. 1988;17:265–286. doi: 10.1146/annurev.bb.17.060188.001405. [DOI] [PubMed] [Google Scholar]
- Heichman K. A., Johnson R. C. The Hin invertasome: protein-mediated joining of distant recombination sites at the enhancer. Science. 1990 Aug 3;249(4968):511–517. doi: 10.1126/science.2166334. [DOI] [PubMed] [Google Scholar]
- Heichman K. A., Moskowitz I. P., Johnson R. C. Configuration of DNA strands and mechanism of strand exchange in the Hin invertasome as revealed by analysis of recombinant knots. Genes Dev. 1991 Sep;5(9):1622–1634. doi: 10.1101/gad.5.9.1622. [DOI] [PubMed] [Google Scholar]
- Johnston B. H. Generation and detection of Z-DNA. Methods Enzymol. 1992;211:127–158. doi: 10.1016/0076-6879(92)11009-8. [DOI] [PubMed] [Google Scholar]
- Kahn J. D., Crothers D. M. DNA bending in transcription initiation. Cold Spring Harb Symp Quant Biol. 1993;58:115–122. doi: 10.1101/sqb.1993.058.01.015. [DOI] [PubMed] [Google Scholar]
- Kanaar R., Klippel A., Shekhtman E., Dungan J. M., Kahmann R., Cozzarelli N. R. Processive recombination by the phage Mu Gin system: implications for the mechanisms of DNA strand exchange, DNA site alignment, and enhancer action. Cell. 1990 Jul 27;62(2):353–366. doi: 10.1016/0092-8674(90)90372-l. [DOI] [PubMed] [Google Scholar]
- Kanaar R., van de Putte P., Cozzarelli N. R. Gin-mediated DNA inversion: product structure and the mechanism of strand exchange. Proc Natl Acad Sci U S A. 1988 Feb;85(3):752–756. doi: 10.1073/pnas.85.3.752. [DOI] [PMC free article] [PubMed] [Google Scholar]
- Kirchhausen T., Wang J. C., Harrison S. C. DNA gyrase and its complexes with DNA: direct observation by electron microscopy. Cell. 1985 Jul;41(3):933–943. doi: 10.1016/s0092-8674(85)80074-x. [DOI] [PubMed] [Google Scholar]
- Klenin K. V., Frank-Kamenetskii M. D., Langowski J. Modulation of intramolecular interactions in superhelical DNA by curved sequences: a Monte Carlo simulation study. Biophys J. 1995 Jan;68(1):81–88. doi: 10.1016/S0006-3495(95)80161-X. [DOI] [PMC free article] [PubMed] [Google Scholar]
- Klenin K. V., Vologodskii A. V., Anshelevich V. V., Dykhne A. M., Frank-Kamenetskii M. D. Computer simulation of DNA supercoiling. J Mol Biol. 1991 Feb 5;217(3):413–419. doi: 10.1016/0022-2836(91)90745-r. [DOI] [PubMed] [Google Scholar]
- Klenin K. V., Vologodskii A. V., Anshelevich V. V., Klishko VYu, Dykhne A. M., Frank-Kamenetskii M. D. Variance of writhe for wormlike DNA rings with excluded volume. J Biomol Struct Dyn. 1989 Feb;6(4):707–714. doi: 10.1080/07391102.1989.10507731. [DOI] [PubMed] [Google Scholar]
- Klippel A., Cloppenborg K., Kahmann R. Isolation and characterization of unusual gin mutants. EMBO J. 1988 Dec 1;7(12):3983–3989. doi: 10.1002/j.1460-2075.1988.tb03286.x. [DOI] [PMC free article] [PubMed] [Google Scholar]
- Kremer W., Klenin K., Diekmann S., Langowski J. DNA curvature influences the internal motions of supercoiled DNA. EMBO J. 1993 Nov;12(11):4407–4412. doi: 10.1002/j.1460-2075.1993.tb06125.x. [DOI] [PMC free article] [PubMed] [Google Scholar]
- Landy A. Dynamic, structural, and regulatory aspects of lambda site-specific recombination. Annu Rev Biochem. 1989;58:913–949. doi: 10.1146/annurev.bi.58.070189.004405. [DOI] [PubMed] [Google Scholar]
- Laundon C. H., Griffith J. D. Curved helix segments can uniquely orient the topology of supertwisted DNA. Cell. 1988 Feb 26;52(4):545–549. doi: 10.1016/0092-8674(88)90467-9. [DOI] [PubMed] [Google Scholar]
- Marians K. J. DNA gyrase-catalyzed decatenation of multiply linked DNA dimers. J Biol Chem. 1987 Jul 25;262(21):10362–10368. [PubMed] [Google Scholar]
- Menzel R., Gellert M. The biochemistry and biology of DNA gyrase. Adv Pharmacol. 1994;29A:39–69. doi: 10.1016/s1054-3589(08)60539-6. [DOI] [PubMed] [Google Scholar]
- Murchie A. I., Lilley D. M. Supercoiled DNA and cruciform structures. Methods Enzymol. 1992;211:158–180. doi: 10.1016/0076-6879(92)11010-g. [DOI] [PubMed] [Google Scholar]
- Nash H. A. Bending and supercoiling of DNA at the attachment site of bacteriophage lambda. Trends Biochem Sci. 1990 Jun;15(6):222–227. doi: 10.1016/0968-0004(90)90034-9. [DOI] [PubMed] [Google Scholar]
- Nash H. A., Pollock T. J. Site-specific recombination of bacteriophage lambda. The change in topological linking number associated with exchange of DNA strands. J Mol Biol. 1983 Oct 15;170(1):19–38. doi: 10.1016/s0022-2836(83)80225-3. [DOI] [PubMed] [Google Scholar]
- Nash H. A. Purification and properties of the bacteriophage lambda Int protein. Methods Enzymol. 1983;100:210–216. doi: 10.1016/0076-6879(83)00057-9. [DOI] [PubMed] [Google Scholar]
- Natale D. A., Umek R. M., Kowalski D. Ease of DNA unwinding is a conserved property of yeast replication origins. Nucleic Acids Res. 1993 Feb 11;21(3):555–560. doi: 10.1093/nar/21.3.555. [DOI] [PMC free article] [PubMed] [Google Scholar]
- Reece R. J., Maxwell A. DNA gyrase: structure and function. Crit Rev Biochem Mol Biol. 1991;26(3-4):335–375. doi: 10.3109/10409239109114072. [DOI] [PubMed] [Google Scholar]
- Rhoades M., Thomas C. A., Jr The P22 bacteriophage DNA molecule. II. Circular intracellular forms. J Mol Biol. 1968 Oct 14;37(1):41–61. doi: 10.1016/0022-2836(68)90072-7. [DOI] [PubMed] [Google Scholar]
- Rybenkov V. V., Cozzarelli N. R., Vologodskii A. V. Probability of DNA knotting and the effective diameter of the DNA double helix. Proc Natl Acad Sci U S A. 1993 Jun 1;90(11):5307–5311. doi: 10.1073/pnas.90.11.5307. [DOI] [PMC free article] [PubMed] [Google Scholar]
- Schlick T. Modeling superhelical DNA: recent analytical and dynamic approaches. Curr Opin Struct Biol. 1995 Apr;5(2):245–262. doi: 10.1016/0959-440x(95)80083-2. [DOI] [PubMed] [Google Scholar]
- Shaw S. Y., Wang J. C. Knotting of a DNA chain during ring closure. Science. 1993 Apr 23;260(5107):533–536. doi: 10.1126/science.8475384. [DOI] [PubMed] [Google Scholar]
- Sperrazza J. M., Register J. C., 3rd, Griffith J. Electron microscopy can be used to measure DNA supertwisting. Gene. 1984 Nov;31(1-3):17–22. doi: 10.1016/0378-1119(84)90190-2. [DOI] [PubMed] [Google Scholar]
- Stark W. M., Boocock M. R., Sherratt D. J. Site-specific recombination by Tn3 resolvase. Trends Genet. 1989 Sep;5(9):304–309. doi: 10.1016/0168-9525(89)90113-3. [DOI] [PubMed] [Google Scholar]
- Stigter D. Interactions of highly charged colloidal cylinders with applications to double-stranded. Biopolymers. 1977 Jul;16(7):1435–1448. doi: 10.1002/bip.1977.360160705. [DOI] [PubMed] [Google Scholar]
- Tjian R., Maniatis T. Transcriptional activation: a complex puzzle with few easy pieces. Cell. 1994 Apr 8;77(1):5–8. doi: 10.1016/0092-8674(94)90227-5. [DOI] [PubMed] [Google Scholar]
- Vinograd J., Lebowitz J., Radloff R., Watson R., Laipis P. The twisted circular form of polyoma viral DNA. Proc Natl Acad Sci U S A. 1965 May;53(5):1104–1111. doi: 10.1073/pnas.53.5.1104. [DOI] [PMC free article] [PubMed] [Google Scholar]
- Vologodskii A. V., Cozzarelli N. R. Conformational and thermodynamic properties of supercoiled DNA. Annu Rev Biophys Biomol Struct. 1994;23:609–643. doi: 10.1146/annurev.bb.23.060194.003141. [DOI] [PubMed] [Google Scholar]
- Vologodskii A. V., Levene S. D., Klenin K. V., Frank-Kamenetskii M., Cozzarelli N. R. Conformational and thermodynamic properties of supercoiled DNA. J Mol Biol. 1992 Oct 20;227(4):1224–1243. doi: 10.1016/0022-2836(92)90533-p. [DOI] [PubMed] [Google Scholar]
- Vologodskii A., Cozzarelli N. Modeling of long-range electrostatic interactions in DNA. Biopolymers. 1995 Mar;35(3):289–296. doi: 10.1002/bip.360350304. [DOI] [PubMed] [Google Scholar]
- Wasserman S. A., Cozzarelli N. R. Biochemical topology: applications to DNA recombination and replication. Science. 1986 May 23;232(4753):951–960. doi: 10.1126/science.3010458. [DOI] [PubMed] [Google Scholar]
- Wasserman S. A., Dungan J. M., Cozzarelli N. R. Discovery of a predicted DNA knot substantiates a model for site-specific recombination. Science. 1985 Jul 12;229(4709):171–174. doi: 10.1126/science.2990045. [DOI] [PubMed] [Google Scholar]
- Wells R. D. Unusual DNA structures. J Biol Chem. 1988 Jan 25;263(3):1095–1098. [PubMed] [Google Scholar]
- Yarmola E. G., Zarudnaya M. I., Lazurkin YuS Osmotic pressure of DNA solutions and effective diameter of the double helix. J Biomol Struct Dyn. 1985 Feb;2(5):981–993. doi: 10.1080/07391102.1985.10507614. [DOI] [PubMed] [Google Scholar]
- ten Heggeler-Bordier B., Wahli W., Adrian M., Stasiak A., Dubochet J. The apical localization of transcribing RNA polymerases on supercoiled DNA prevents their rotation around the template. EMBO J. 1992 Feb;11(2):667–672. doi: 10.1002/j.1460-2075.1992.tb05098.x. [DOI] [PMC free article] [PubMed] [Google Scholar]