Abstract
Cylindrical cell model Poisson-Boltzmann (P-B) calculations are used to evaluate the electrostatic contributions to the relative stability of various DNA conformations (A, B, C, Z, and single-stranded (ss) with charge spacings of 3.38 and 4.2 A) as a function of interhelix distance in a concentrated solution of divalent cations. The divalent ion concentration was set at 100 mM, to compare with our earlier reports of spectroscopic and calorimetric experiments, which demonstrate substantial disruption of B-DNA geometry. Monovalent cations neutralize the DNA phosphates in two ways, corresponding to different experimental situations: 1) There is no significant contribution to the ionic strength from the neutralizing cations, corresponding to DNA condensation from dilute solution and to osmotic stress experiments in which DNA segments are brought into close proximity to each other in the presence of a large excess of buffer. 2) The solution is uniformly concentrated in DNA, so that the neutralizing cations add significantly to those in the buffer at close DNA packing. In case 1), conformations with lower charge density (Z and ssDNA) have markedly lower electrostatic free energies than B-DNA as the DNA molecules approach closely, due largely to ionic entropy. If the divalent cations bind preferentially to single-stranded DNA or a distorted form of B-DNA, as is the case with transition metals, the base pairing and stacking free energies that stabilize the double helix against electrostatic denaturation may be overcome. Strong binding to the bases is favored by the high concentration of divalent cations at the DNA surface arising from the large negative surface potential; the surface concentration increases sharply as the interhelical distance decreases. In case 2), the concentration of neutralizing monovalent cations becomes very large and the electrostatic free energy difference between secondary structures becomes small as the interhelical spacing decreases. Such high ionic concentrations will be expected to modify the stability of DNA by changing water activity as well as by screening electrostatic interactions. This may be the root of the decreased thermal stability of DNA in the presence of high concentrations of magnesium ions.
Full text
PDF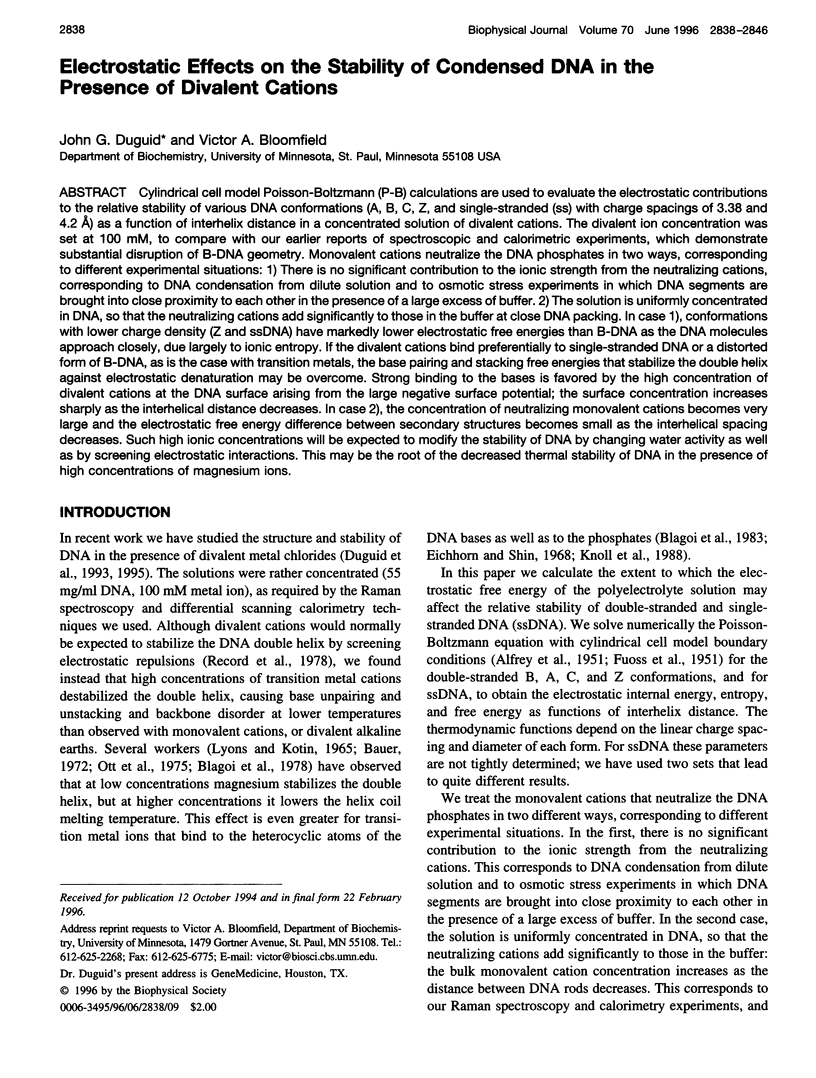
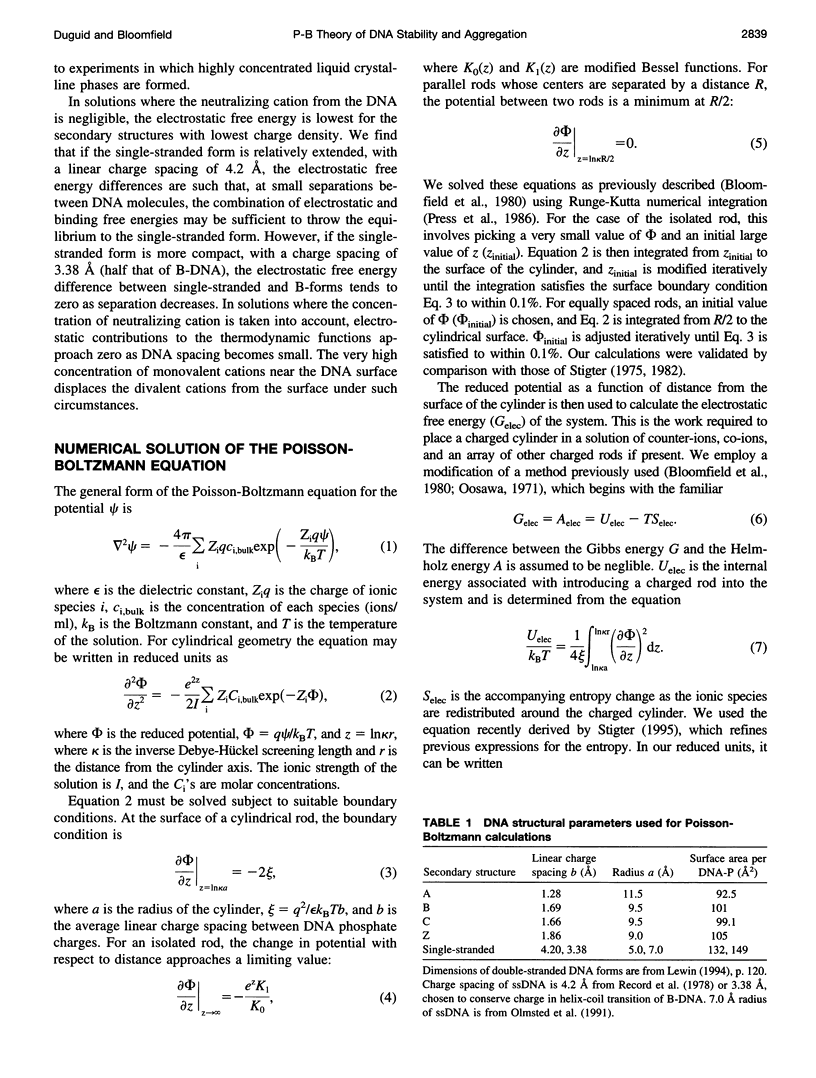
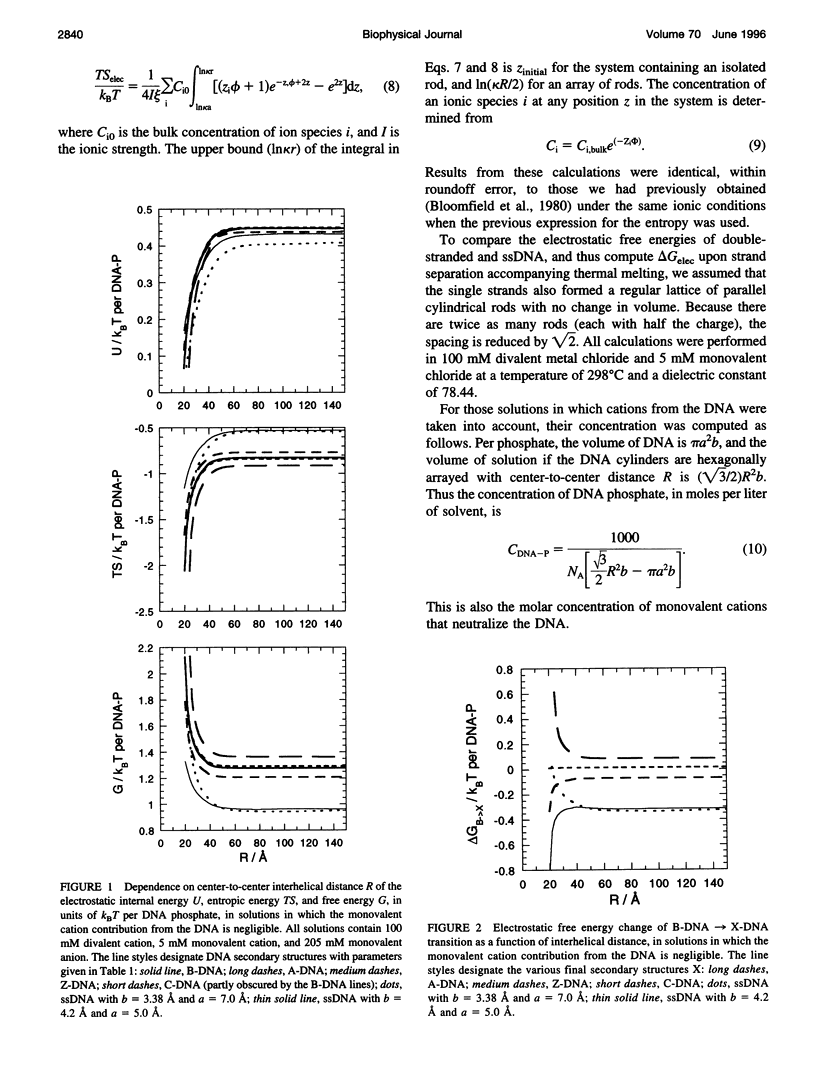
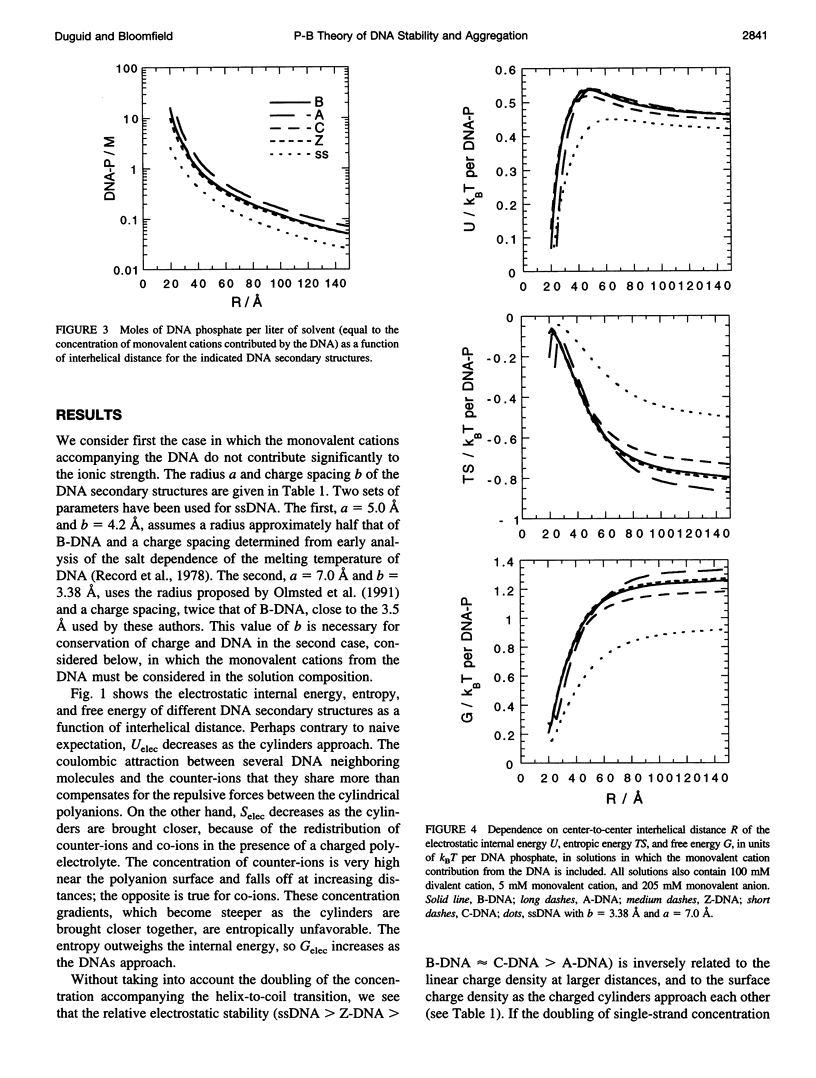
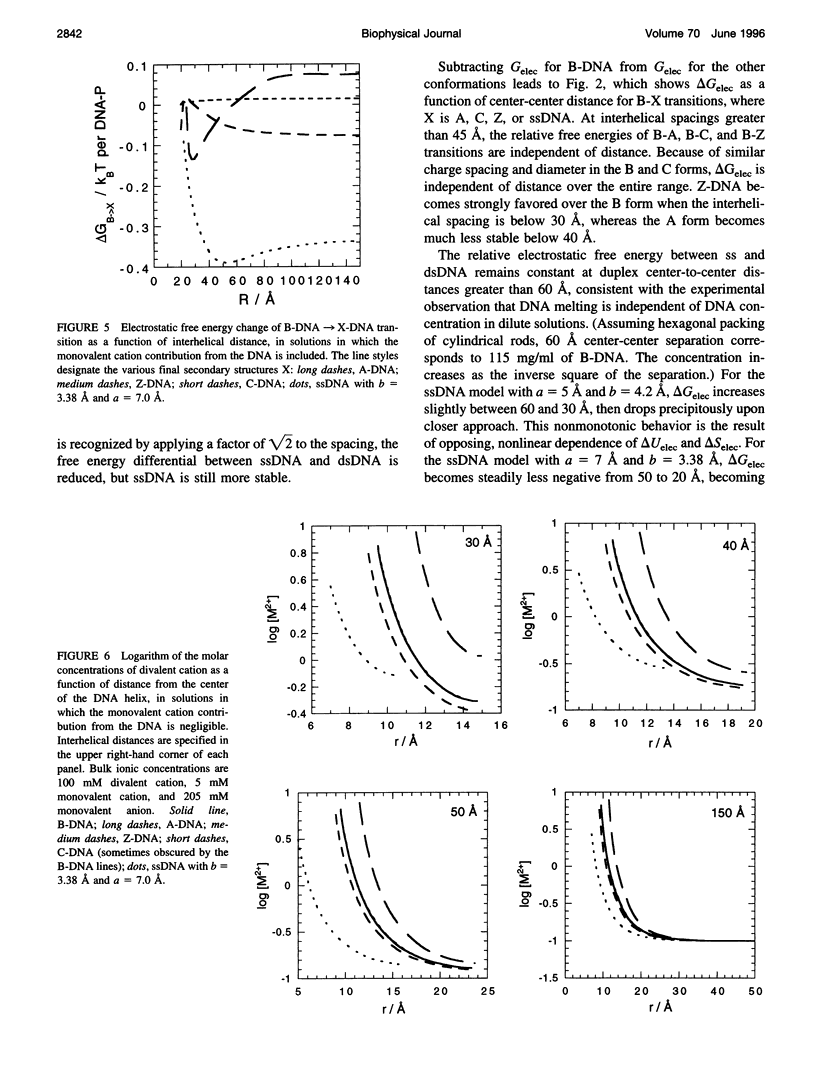
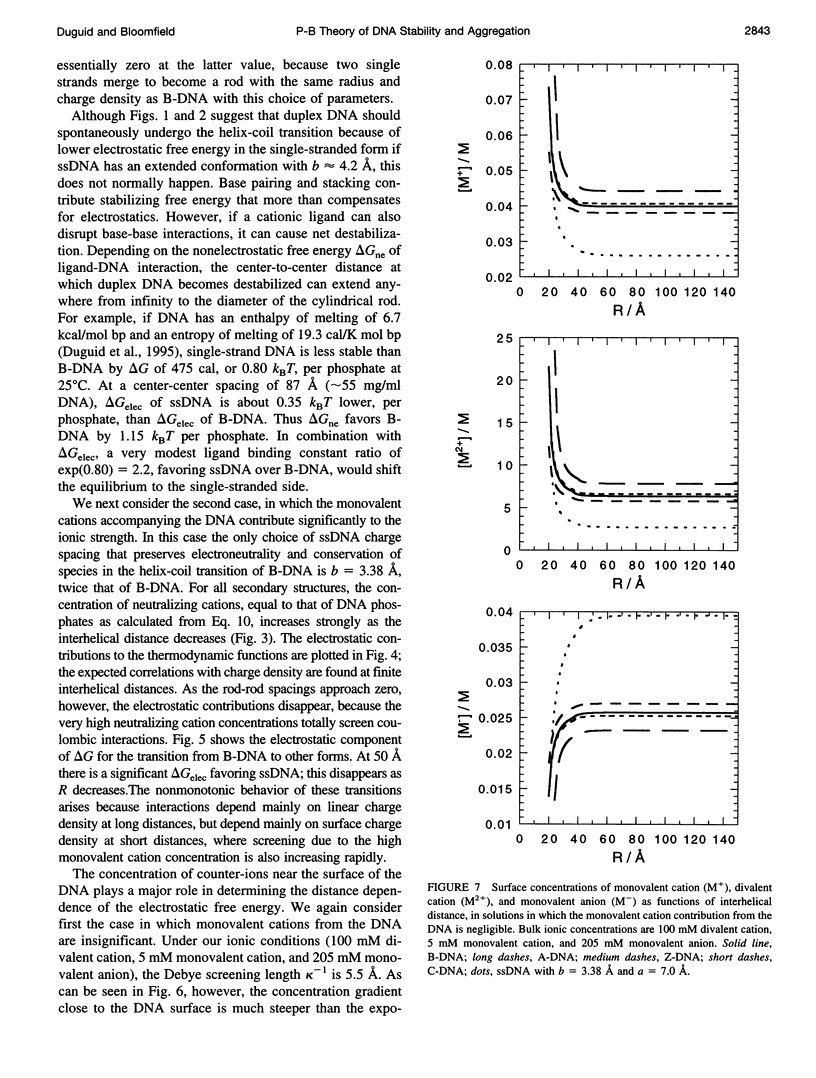
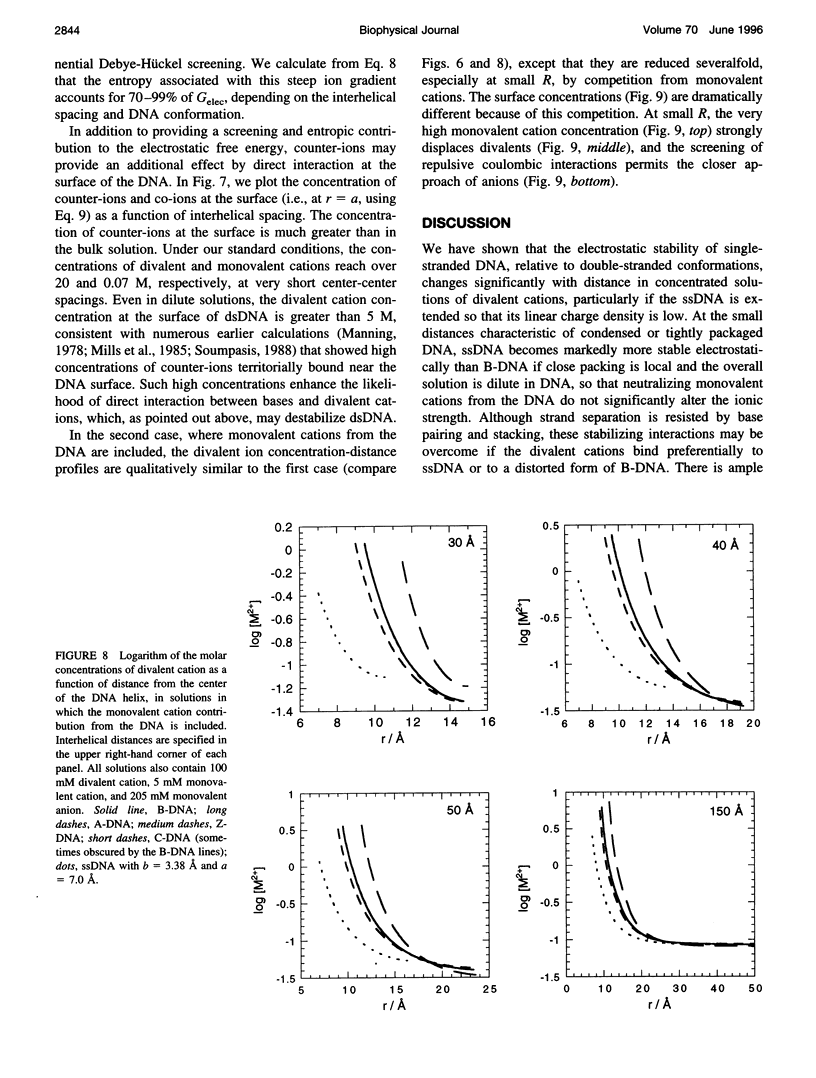
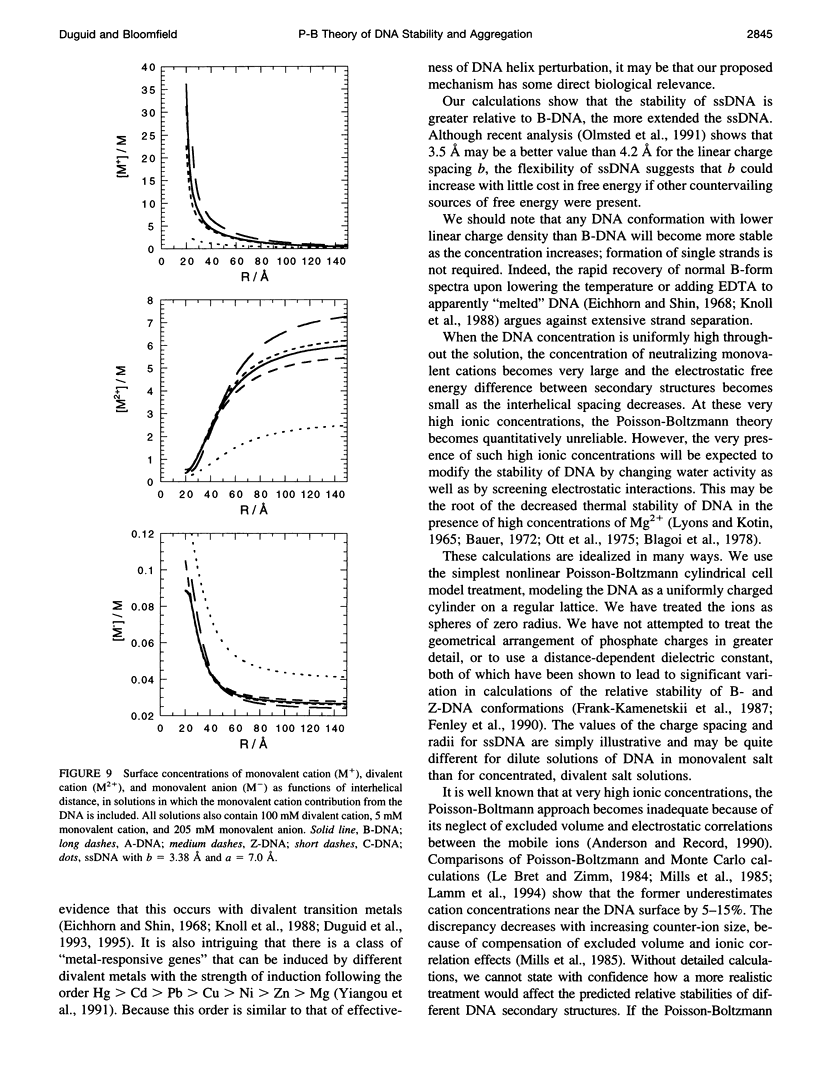
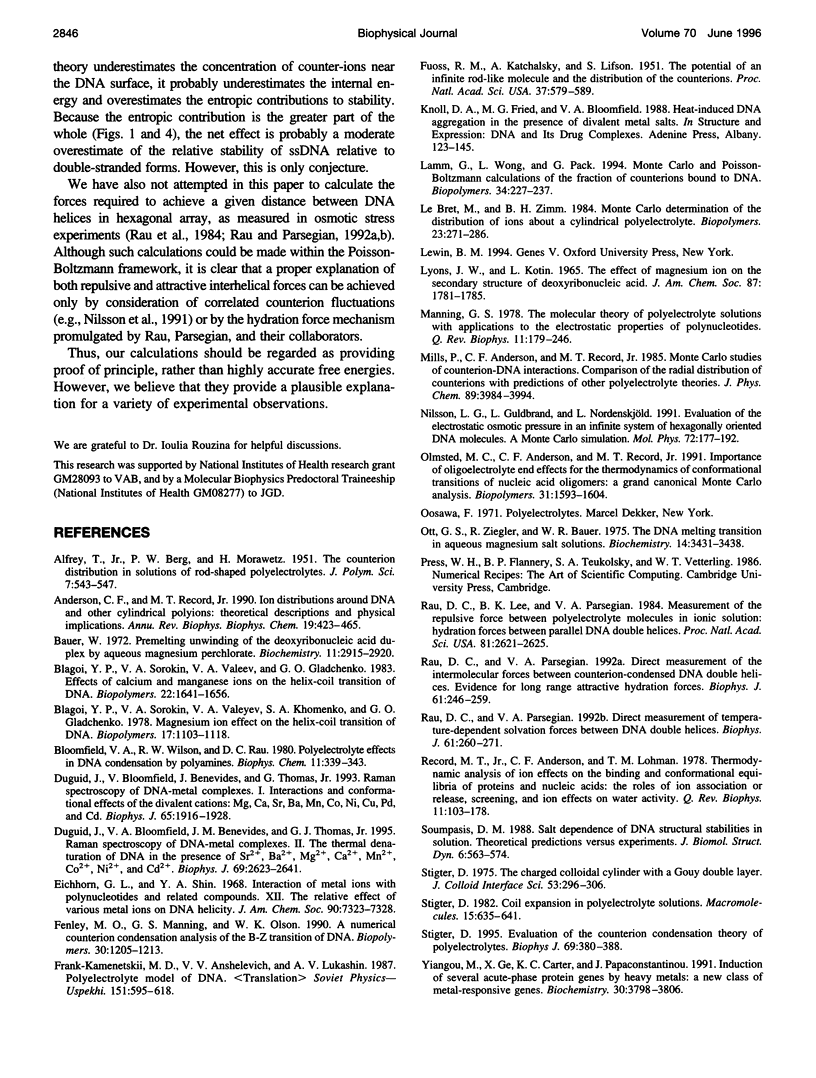
Selected References
These references are in PubMed. This may not be the complete list of references from this article.
- Anderson C. F., Record M. T., Jr Ion distributions around DNA and other cylindrical polyions: theoretical descriptions and physical implications. Annu Rev Biophys Biophys Chem. 1990;19:423–465. doi: 10.1146/annurev.bb.19.060190.002231. [DOI] [PubMed] [Google Scholar]
- Bauer W. R. Premelting unwinding of the deoxyribonucleic acid duplex by aqueous magnesium perchlorate. Biochemistry. 1972 Jul 18;11(15):2915–2920. doi: 10.1021/bi00765a027. [DOI] [PubMed] [Google Scholar]
- Blagoi YuP, Sorokin V. A., Valeev V. A., Gladchenko G. O. Effects of calcium and manganese ions on the helix-coil transition of DNA. Biopolymers. 1983 Jul;22(7):1641–1656. doi: 10.1002/bip.360220704. [DOI] [PubMed] [Google Scholar]
- Blagoi Y. P., Sorokin V. A., Valeyev V. A., Khomenko S. A., Gladchenko G. O. Magnesium ion effect on the helix-coil transition of DNA. Biopolymers. 1978 May;17(5):1103–1118. doi: 10.1002/bip.1978.360170502. [DOI] [PubMed] [Google Scholar]
- Bloomfield V. A., Wilson R. W., Rau D. C. Polyelectrolyte effects in DNA condensation by polyamines. Biophys Chem. 1980 Jun;11(3-4):339–343. doi: 10.1016/0301-4622(80)87006-2. [DOI] [PubMed] [Google Scholar]
- Duguid J. G., Bloomfield V. A., Benevides J. M., Thomas G. J., Jr Raman spectroscopy of DNA-metal complexes. II. The thermal denaturation of DNA in the presence of Sr2+, Ba2+, Mg2+, Ca2+, Mn2+, Co2+, Ni2+, and Cd2+. Biophys J. 1995 Dec;69(6):2623–2641. doi: 10.1016/S0006-3495(95)80133-5. [DOI] [PMC free article] [PubMed] [Google Scholar]
- Duguid J., Bloomfield V. A., Benevides J., Thomas G. J., Jr Raman spectroscopy of DNA-metal complexes. I. Interactions and conformational effects of the divalent cations: Mg, Ca, Sr, Ba, Mn, Co, Ni, Cu, Pd, and Cd. Biophys J. 1993 Nov;65(5):1916–1928. doi: 10.1016/S0006-3495(93)81263-3. [DOI] [PMC free article] [PubMed] [Google Scholar]
- Eichhorn G. L., Shin Y. A. Interaction of metal ions with polynucleotides and related compounds. XII. The relative effect of various metal ions on DNA helicity. J Am Chem Soc. 1968 Dec 18;90(26):7323–7328. doi: 10.1021/ja01028a024. [DOI] [PubMed] [Google Scholar]
- Fenley M. O., Manning G. S., Olson W. K. A numerical counterion condensation analysis of the B-Z transition of DNA. Biopolymers. 1990;30(13-14):1205–1213. doi: 10.1002/bip.360301306. [DOI] [PubMed] [Google Scholar]
- Fuoss R. M., Katchalsky A., Lifson S. The Potential of an Infinite Rod-Like Molecule and the Distribution of the Counter Ions. Proc Natl Acad Sci U S A. 1951 Sep;37(9):579–589. doi: 10.1073/pnas.37.9.579. [DOI] [PMC free article] [PubMed] [Google Scholar]
- LYONS J. W., KOTIN L. THE EFFECT OF MAGNESIUM ION ON THE SECONDARY STRUCTURE OF DEOXYRIBONUCLEIC ACID. J Am Chem Soc. 1965 Apr 20;87:1781–1785. doi: 10.1021/ja01086a030. [DOI] [PubMed] [Google Scholar]
- Lamm G., Wong L., Pack G. R. Monte Carlo and Poisson-Boltzmann calculations of the fraction of counterions bound to DNA. Biopolymers. 1994 Feb;34(2):227–237. doi: 10.1002/bip.360340209. [DOI] [PubMed] [Google Scholar]
- Le Bret M., Zimm B. H. Monte Carlo determination of the distribution of ions about a cylindrical polyelectrolyte. Biopolymers. 1984 Feb;23(2):271–285. doi: 10.1002/bip.360230208. [DOI] [PubMed] [Google Scholar]
- Manning G. S. The molecular theory of polyelectrolyte solutions with applications to the electrostatic properties of polynucleotides. Q Rev Biophys. 1978 May;11(2):179–246. doi: 10.1017/s0033583500002031. [DOI] [PubMed] [Google Scholar]
- Olmsted M. C., Anderson C. F., Record M. T., Jr Importance of oligoelectrolyte end effects for the thermodynamics of conformational transitions of nucleic acid oligomers: a grand canonical Monte Carlo analysis. Biopolymers. 1991 Nov;31(13):1593–1604. doi: 10.1002/bip.360311314. [DOI] [PubMed] [Google Scholar]
- Ott G. S., Ziegler R., Bauer W. R. The DNA melting transition in aqueous magnesium salt solutions. Biochemistry. 1975 Jul 29;14(15):3431–3438. doi: 10.1021/bi00686a022. [DOI] [PubMed] [Google Scholar]
- Rau D. C., Lee B., Parsegian V. A. Measurement of the repulsive force between polyelectrolyte molecules in ionic solution: hydration forces between parallel DNA double helices. Proc Natl Acad Sci U S A. 1984 May;81(9):2621–2625. doi: 10.1073/pnas.81.9.2621. [DOI] [PMC free article] [PubMed] [Google Scholar]
- Rau D. C., Parsegian V. A. Direct measurement of temperature-dependent solvation forces between DNA double helices. Biophys J. 1992 Jan;61(1):260–271. doi: 10.1016/S0006-3495(92)81832-5. [DOI] [PMC free article] [PubMed] [Google Scholar]
- Rau D. C., Parsegian V. A. Direct measurement of the intermolecular forces between counterion-condensed DNA double helices. Evidence for long range attractive hydration forces. Biophys J. 1992 Jan;61(1):246–259. doi: 10.1016/S0006-3495(92)81831-3. [DOI] [PMC free article] [PubMed] [Google Scholar]
- Record M. T., Jr, Anderson C. F., Lohman T. M. Thermodynamic analysis of ion effects on the binding and conformational equilibria of proteins and nucleic acids: the roles of ion association or release, screening, and ion effects on water activity. Q Rev Biophys. 1978 May;11(2):103–178. doi: 10.1017/s003358350000202x. [DOI] [PubMed] [Google Scholar]
- Soumpasis D. M. Salt dependence of DNA structural stabilities in solution. Theoretical predictions versus experiments. J Biomol Struct Dyn. 1988 Dec;6(3):563–574. doi: 10.1080/07391102.1988.10506507. [DOI] [PubMed] [Google Scholar]
- Stigter D. Evaluation of the counterion condensation theory of polyelectrolytes. Biophys J. 1995 Aug;69(2):380–388. doi: 10.1016/S0006-3495(95)79910-6. [DOI] [PMC free article] [PubMed] [Google Scholar]
- Yiangou M., Ge X., Carter K. C., Papaconstantinou J. Induction of several acute-phase protein genes by heavy metals: a new class of metal-responsive genes. Biochemistry. 1991 Apr 16;30(15):3798–3806. doi: 10.1021/bi00229a029. [DOI] [PubMed] [Google Scholar]