Abstract
We have determined the rate and quantum yield of retinal photoisomerization, the spectra of the primary transients, and the energy stored in the K intermediate in the photocycle of some bacteriorhodopsin mutants (V49A, A53G, and W182F) in which residue replacements are found to change the Schiff base deprotonation kinetics (and thus the protein-retinal interaction). Because of their change in the local volume resulting from these individual replacements, these substitutions perturb the proton donor-acceptor relative orientation change and thus the Schiff base deprotonation kinetics. These replacements are thus expected to change the charge distribution around the retinal, which controls its photoisomerization dynamics. Subpicosecond transient spectroscopy as well as photoacoustic technique are used to determine the retinal photoisomerization rate, quantum yield, and the energy stored in the K-intermediate for these mutants. The results are compared with those obtained for wild-type bacteriorhodopsin and other mutants in which charged residues in the cavity are replaced by neutral ones. In some of the mutants the rate of photoisomerization is changed, but in none is the quantum yield or the energy stored in the K intermediate altered from that in the wild type. These results are discussed in terms of the shapes of the potential energy surfaces of the excited and ground states of retinal in the perpendicular configuration within the protein and the stabilization of the positive charge in the ground and the excited state of the electronic system of retinal.
Full text
PDF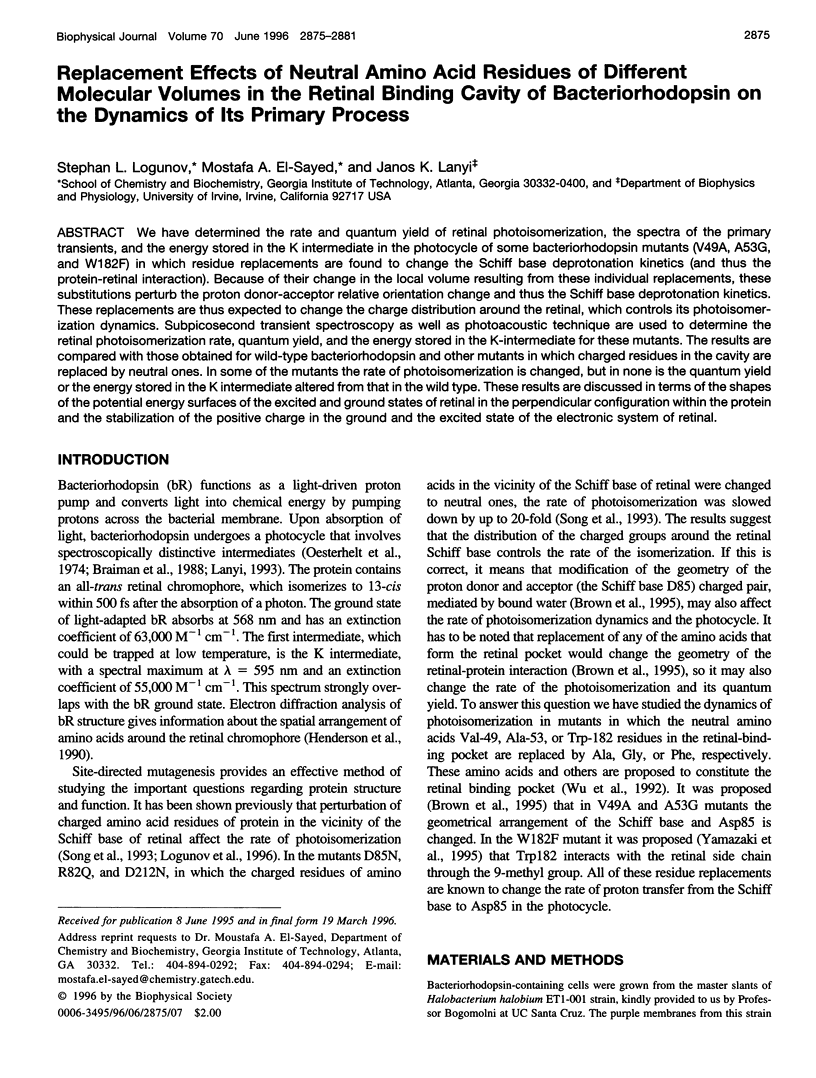
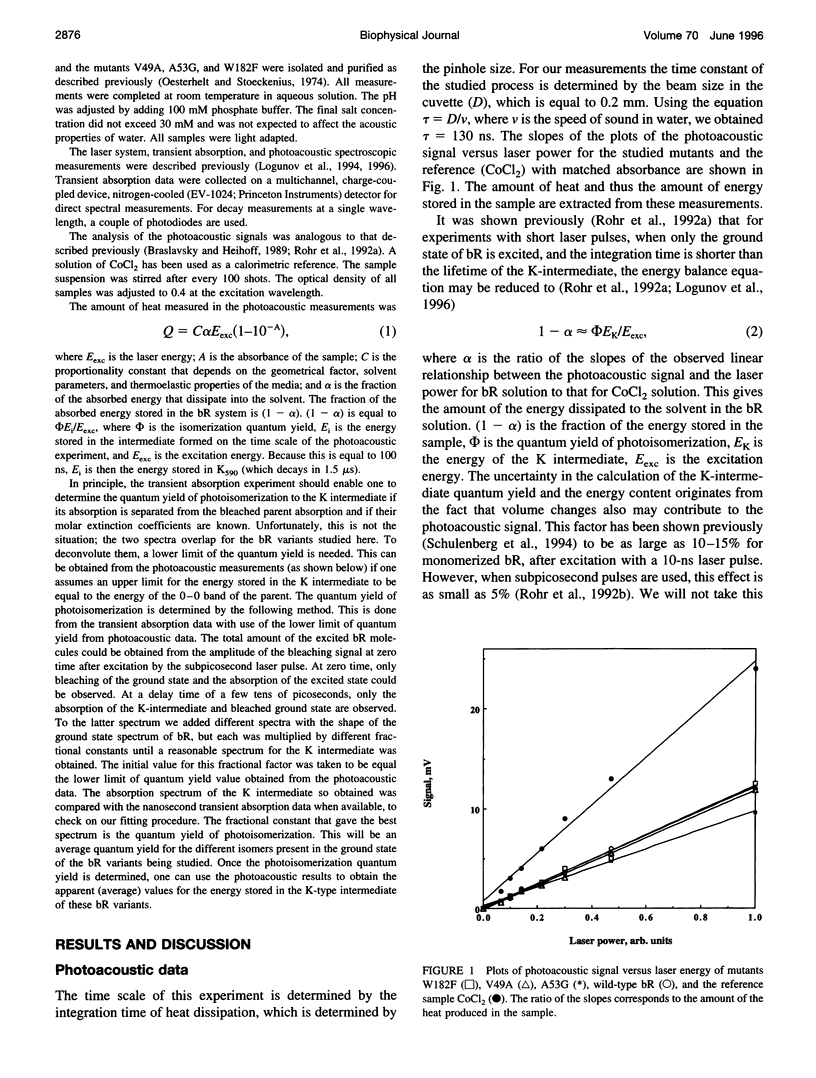
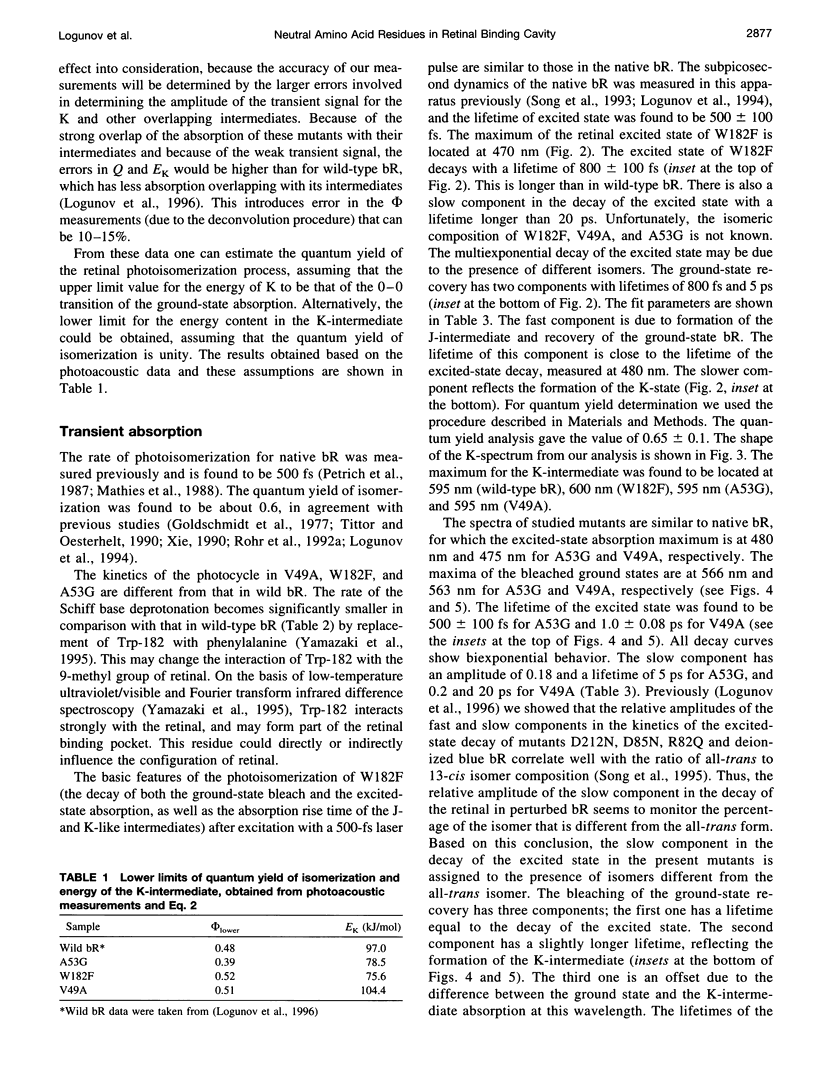
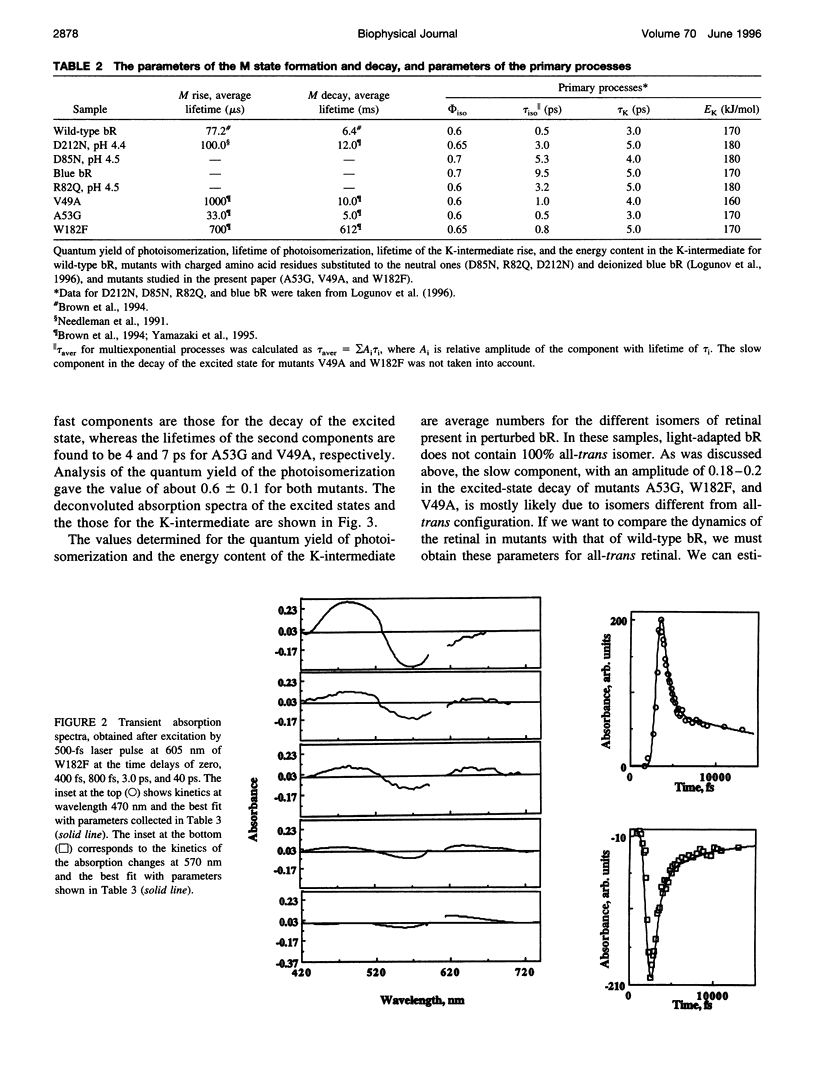
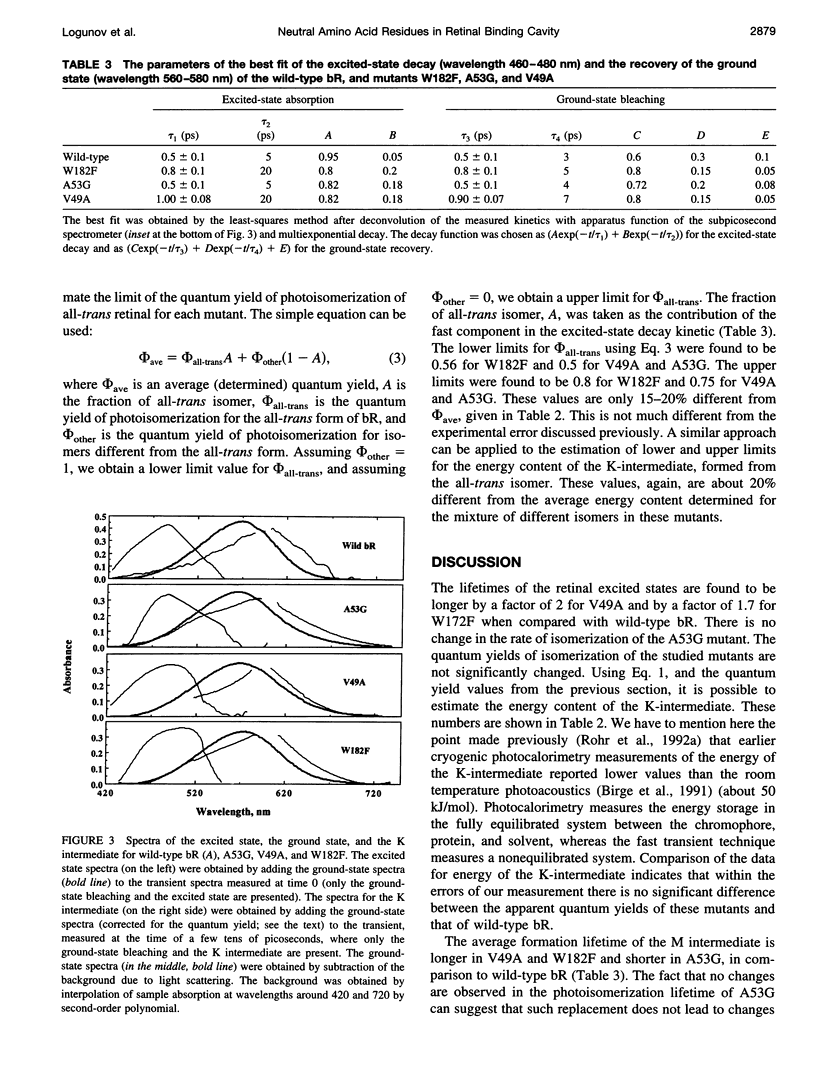
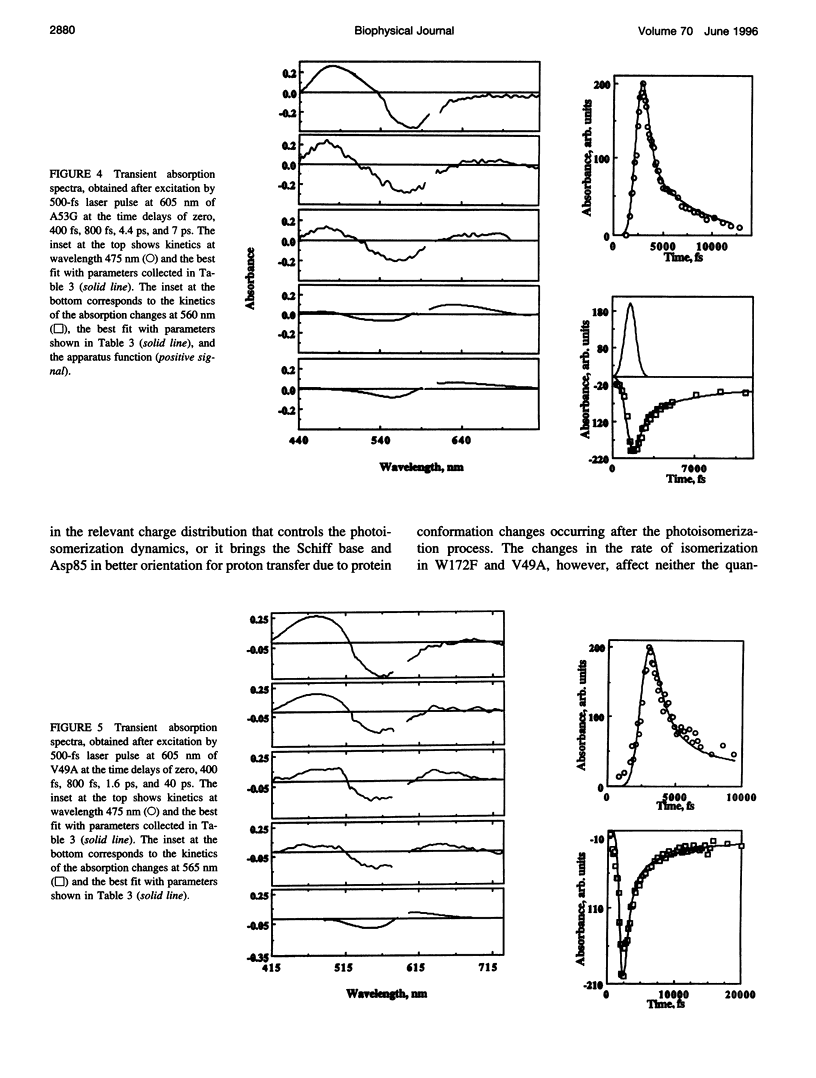
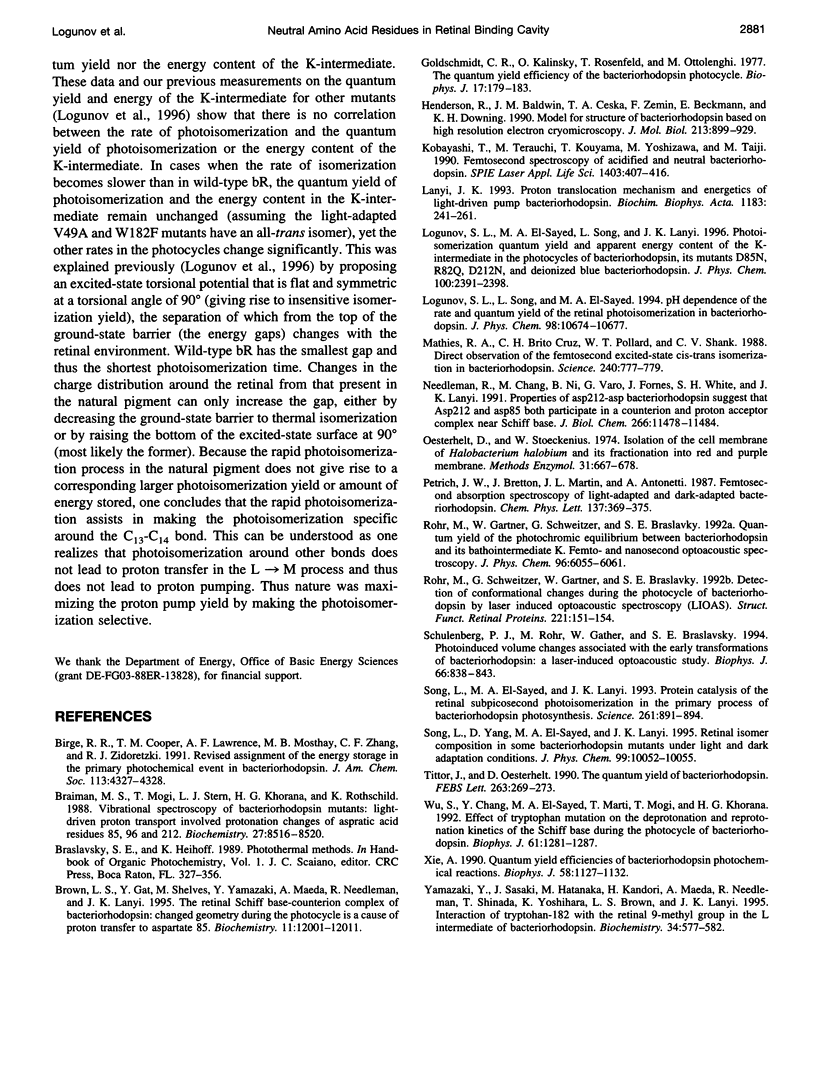
Selected References
These references are in PubMed. This may not be the complete list of references from this article.
- Braiman M. S., Mogi T., Marti T., Stern L. J., Khorana H. G., Rothschild K. J. Vibrational spectroscopy of bacteriorhodopsin mutants: light-driven proton transport involves protonation changes of aspartic acid residues 85, 96, and 212. Biochemistry. 1988 Nov 15;27(23):8516–8520. doi: 10.1021/bi00423a002. [DOI] [PubMed] [Google Scholar]
- Goldschmidt C. R., Kalisky O., Rosenfeld T., Ottolenghi M. The quantum efficiency of the bacteriorhodopsin photocycle. Biophys J. 1977 Feb;17(2):179–183. doi: 10.1016/S0006-3495(77)85635-X. [DOI] [PMC free article] [PubMed] [Google Scholar]
- Henderson R., Baldwin J. M., Ceska T. A., Zemlin F., Beckmann E., Downing K. H. Model for the structure of bacteriorhodopsin based on high-resolution electron cryo-microscopy. J Mol Biol. 1990 Jun 20;213(4):899–929. doi: 10.1016/S0022-2836(05)80271-2. [DOI] [PubMed] [Google Scholar]
- Lanyi J. K. Proton translocation mechanism and energetics in the light-driven pump bacteriorhodopsin. Biochim Biophys Acta. 1993 Dec 7;1183(2):241–261. doi: 10.1016/0005-2728(93)90226-6. [DOI] [PubMed] [Google Scholar]
- Mathies R. A., Brito Cruz C. H., Pollard W. T., Shank C. V. Direct observation of the femtosecond excited-state cis-trans isomerization in bacteriorhodopsin. Science. 1988 May 6;240(4853):777–779. doi: 10.1126/science.3363359. [DOI] [PubMed] [Google Scholar]
- Needleman R., Chang M., Ni B., Váró G., Fornés J., White S. H., Lanyi J. K. Properties of Asp212----Asn bacteriorhodopsin suggest that Asp212 and Asp85 both participate in a counterion and proton acceptor complex near the Schiff base. J Biol Chem. 1991 Jun 25;266(18):11478–11484. [PubMed] [Google Scholar]
- Oesterhelt D., Stoeckenius W. Isolation of the cell membrane of Halobacterium halobium and its fractionation into red and purple membrane. Methods Enzymol. 1974;31:667–678. doi: 10.1016/0076-6879(74)31072-5. [DOI] [PubMed] [Google Scholar]
- Schulenberg P. J., Rohr M., Gärtner W., Braslavsky S. E. Photoinduced volume changes associated with the early transformations of bacteriorhodopsin: a laser-induced optoacoustic spectroscopy study. Biophys J. 1994 Mar;66(3 Pt 1):838–843. doi: 10.1016/s0006-3495(94)80860-4. [DOI] [PMC free article] [PubMed] [Google Scholar]
- Song L., El-Sayed M. A., Lanyi J. K. Protein catalysis of the retinal subpicosecond photoisomerization in the primary process of bacteriorhodopsin photosynthesis. Science. 1993 Aug 13;261(5123):891–894. doi: 10.1126/science.261.5123.891. [DOI] [PubMed] [Google Scholar]
- Wu S., Chang Y., el-Sayed M. A., Marti T., Mogi T., Khorana H. G. Effects of tryptophan mutation on the deprotonation and reprotonation kinetics of the Schiff base during the photocycle of bacteriorhodopsin. Biophys J. 1992 May;61(5):1281–1288. doi: 10.1016/S0006-3495(92)81936-7. [DOI] [PMC free article] [PubMed] [Google Scholar]
- Xie A. H. Quantum efficiencies of bacteriorhodopsin photochemical reactions. Biophys J. 1990 Nov;58(5):1127–1132. doi: 10.1016/S0006-3495(90)82455-3. [DOI] [PMC free article] [PubMed] [Google Scholar]
- Yamazaki Y., Sasaki J., Hatanaka M., Kandori H., Maeda A., Needleman R., Shinada T., Yoshihara K., Brown L. S., Lanyi J. K. Interaction of tryptophan-182 with the retinal 9-methyl group in the L intermediate of bacteriorhodopsin. Biochemistry. 1995 Jan 17;34(2):577–582. doi: 10.1021/bi00002a024. [DOI] [PubMed] [Google Scholar]