Abstract
Regulation of skeletal muscle contraction is achieved through the interaction of six different proteins: actin, myosin, tropomyosin, and troponins C, I, and T. Many experiments have been performed on the interactions of these proteins, but comparatively less effort has been spent on attempts to integrate the results into a coherent description of the system as a whole. In this paper, we present a new way of approaching the integration problem by using a cellular automaton. We assign rate constants for state changes within each constituent molecule of the muscle thin filament as functions of the states of its neighboring molecules. The automaton shows how the interactions among constituent molecules give rise to the overall regulatory behavior of thin filaments as observed in vitro and is extendable to in vivo measurements. The model is used to predict myosin binding and ATPase activity, and the result is compared with various experimental data. Two important aspects of regulation are revealed by the requirement that the model fit the experimental data: (1) strong interactions must exist between two successively bound myosin heads, and (2) the cooperative binding of calcium to the thin filament can be attributed in a simple way to the interaction between neighboring troponin-tropomyosin units.
Full text
PDF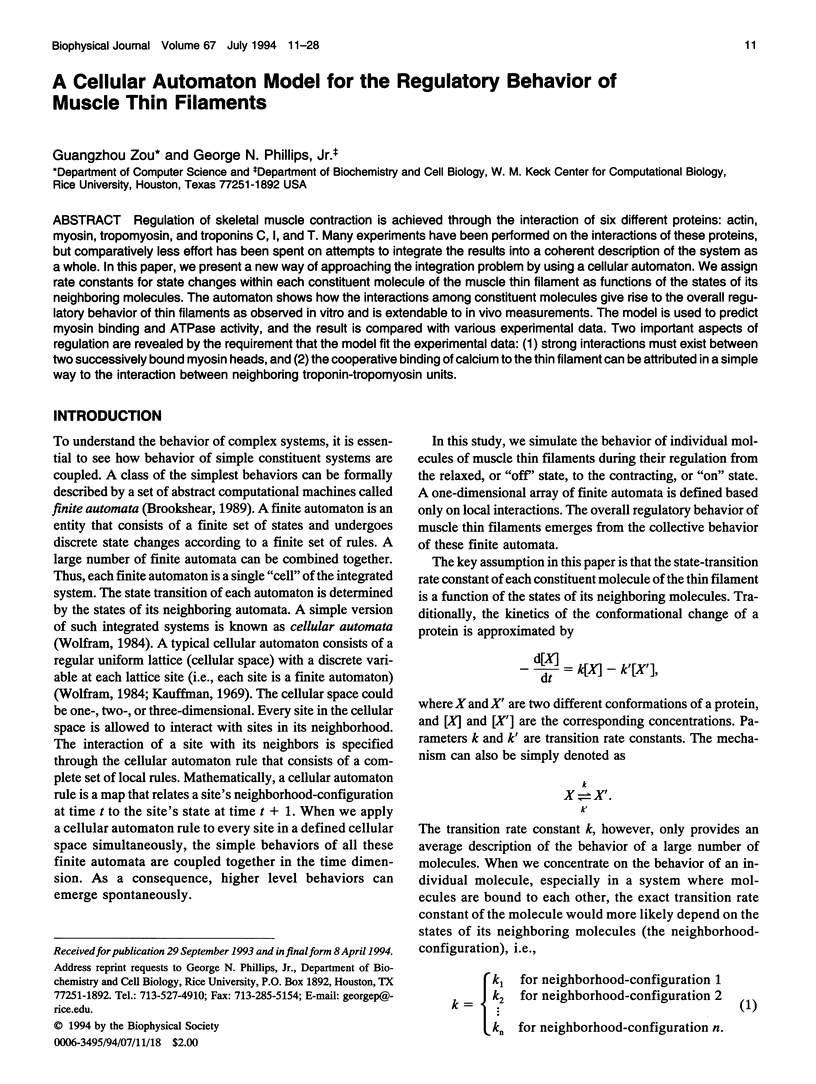
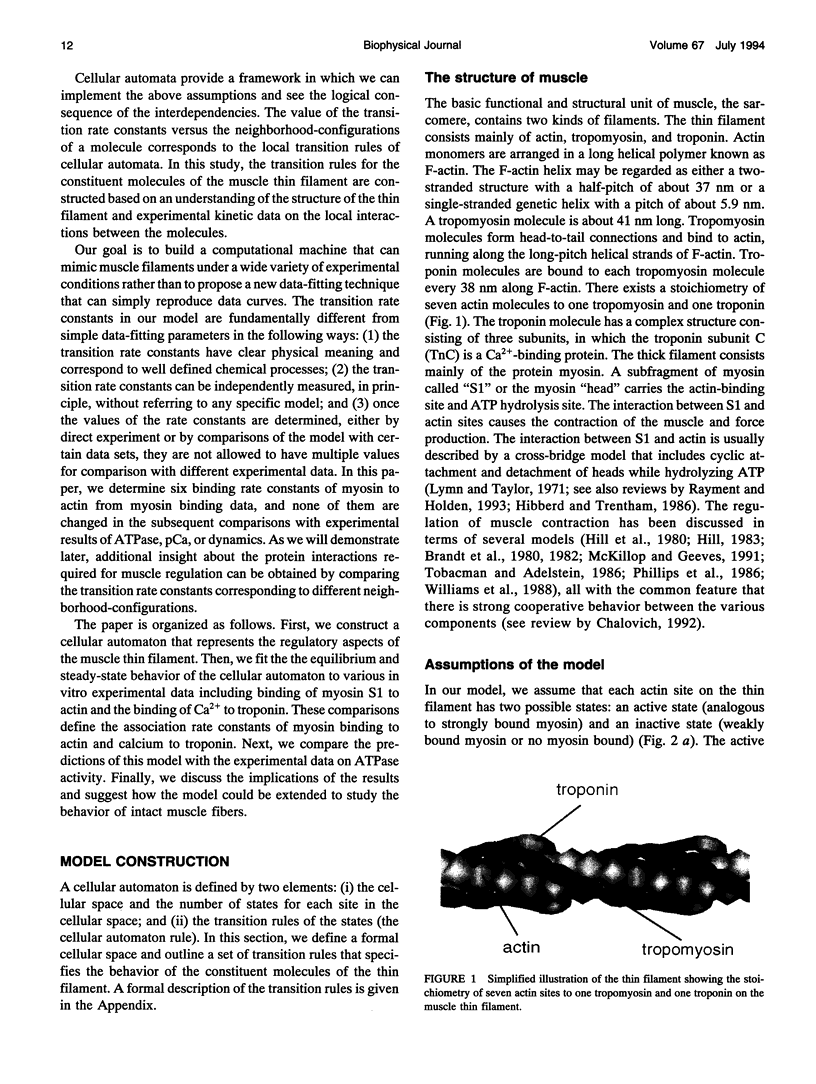
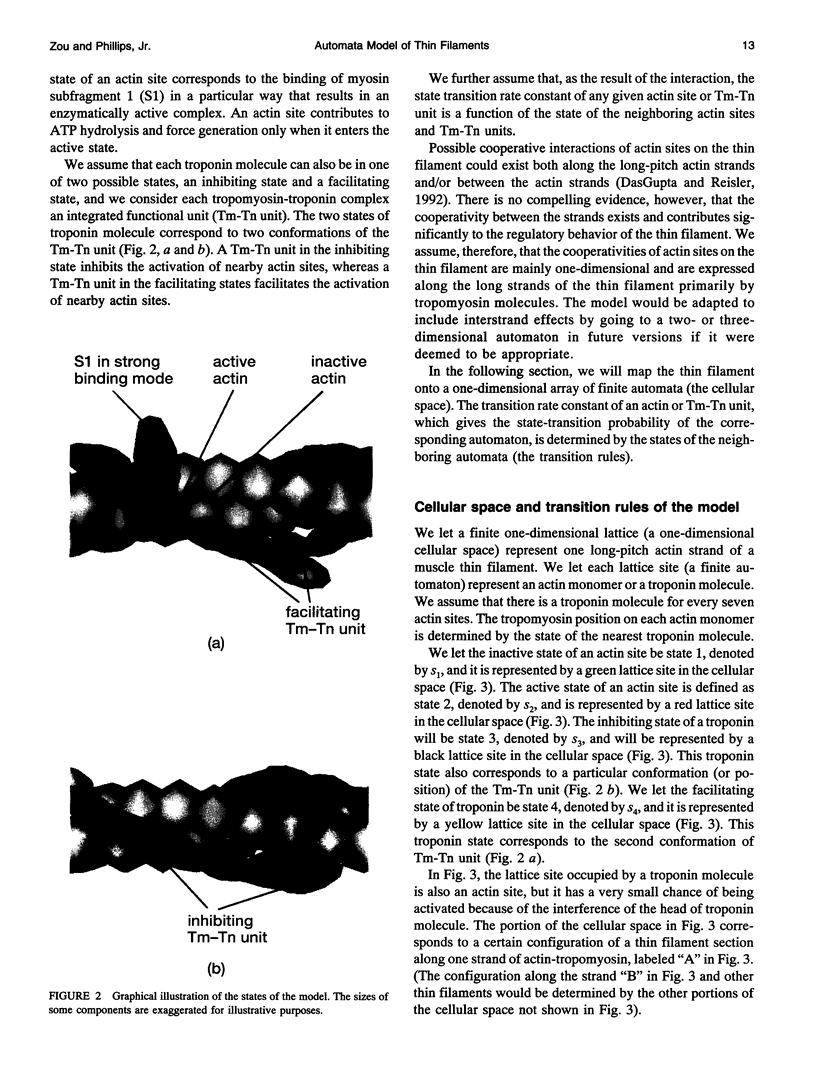
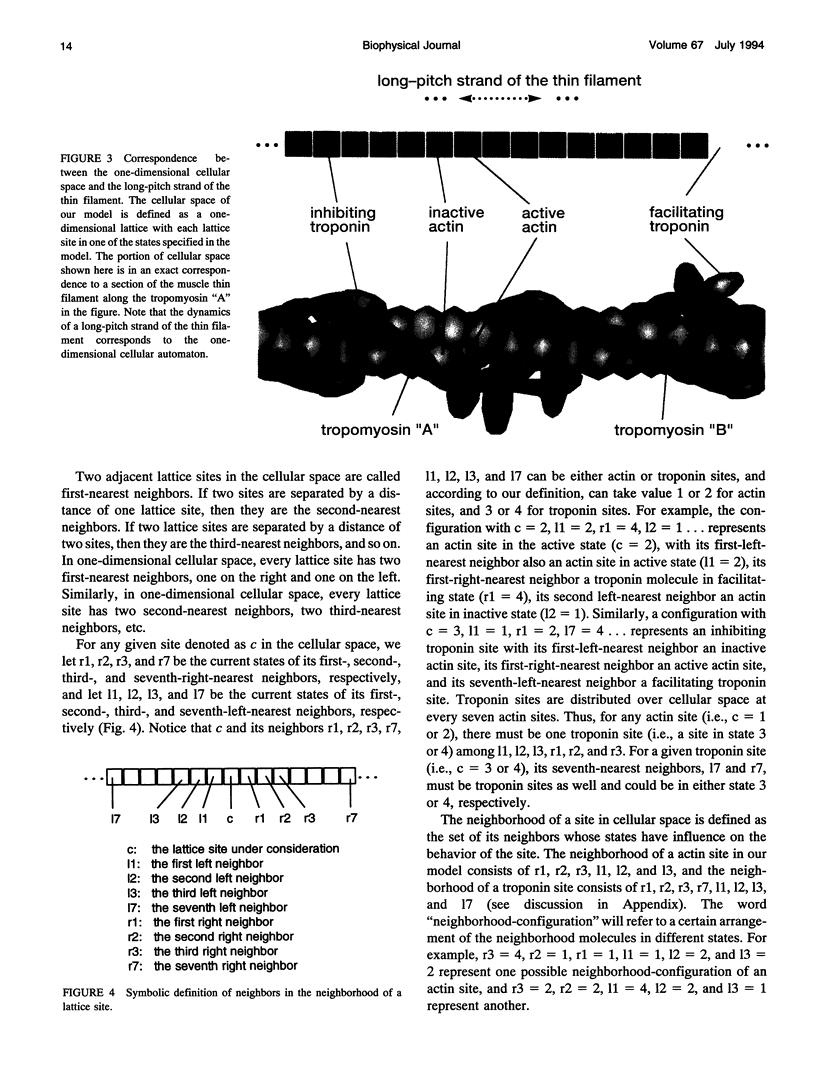
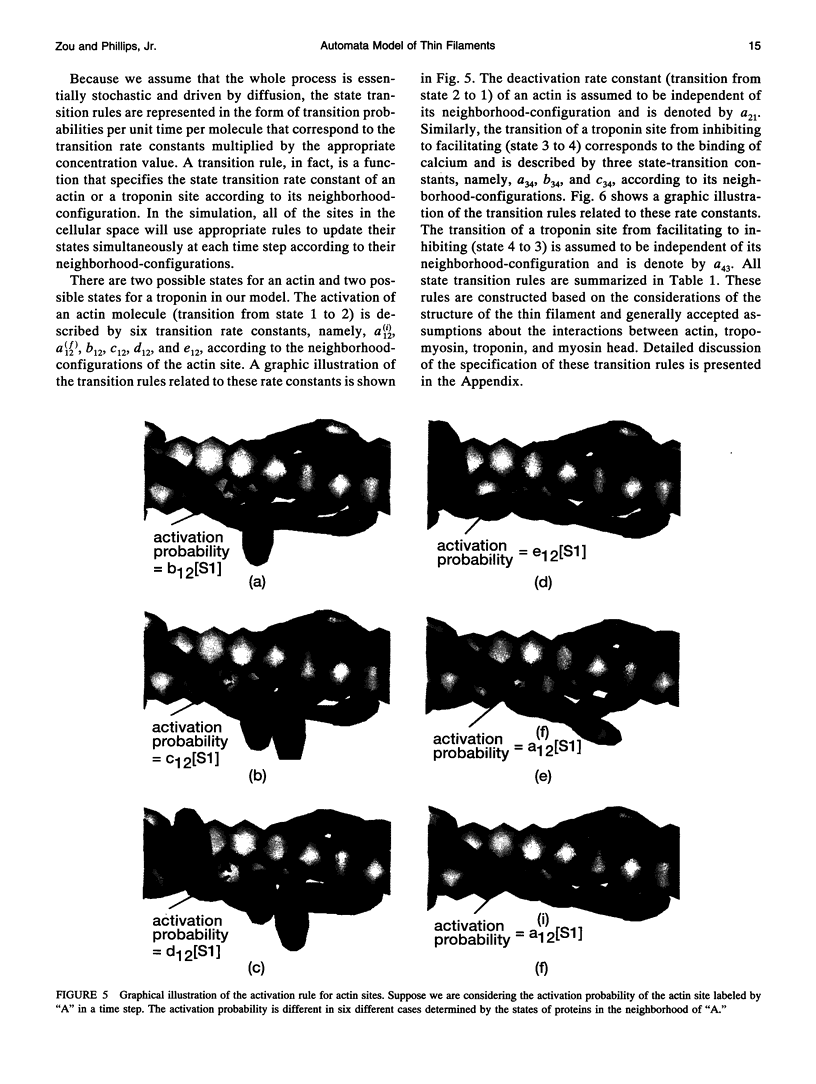
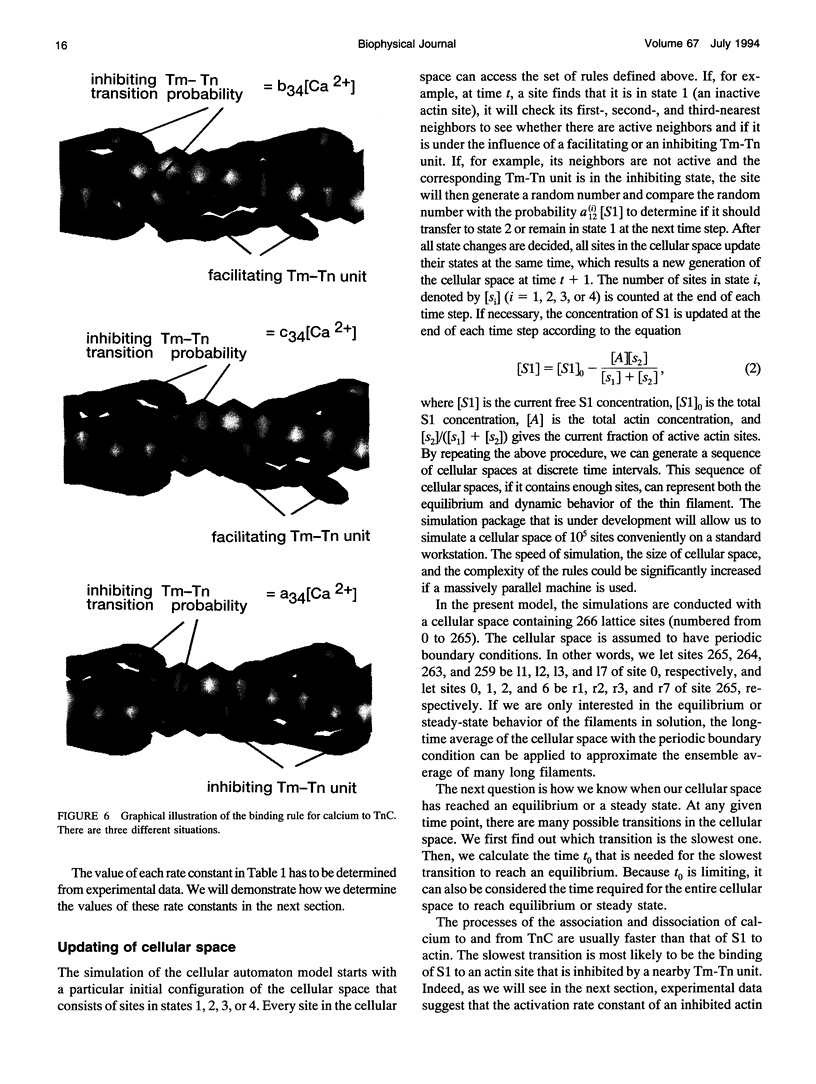
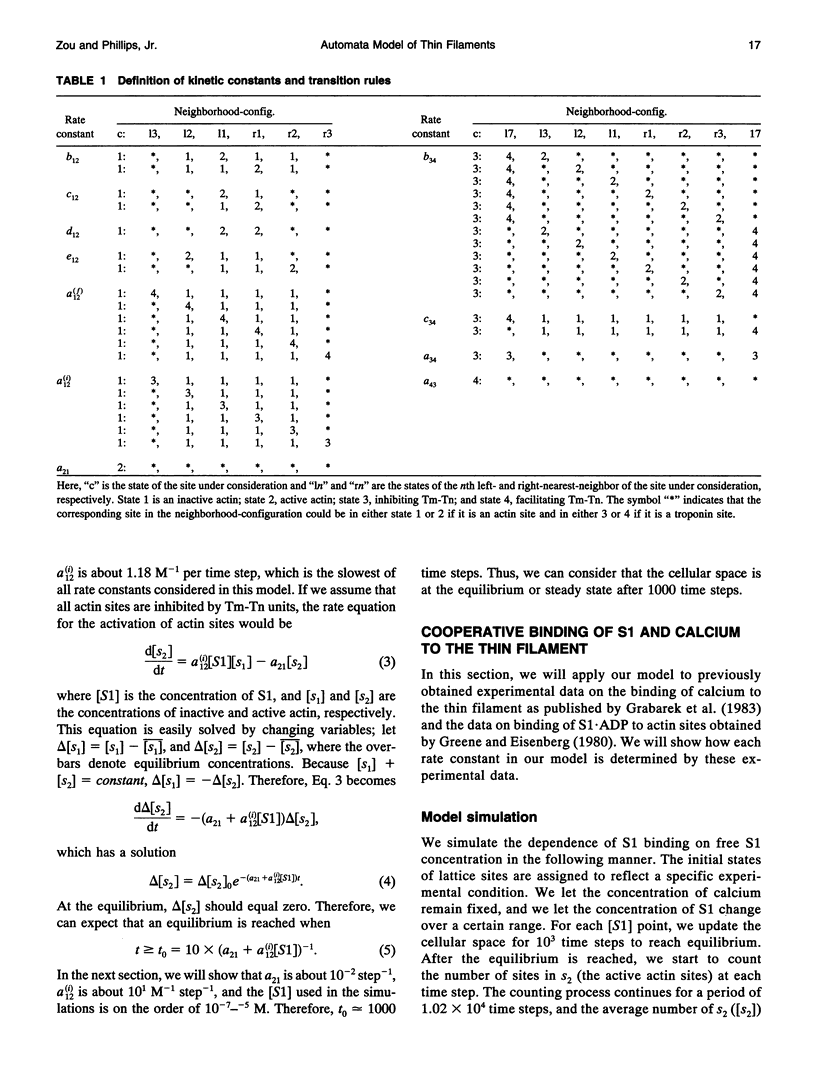
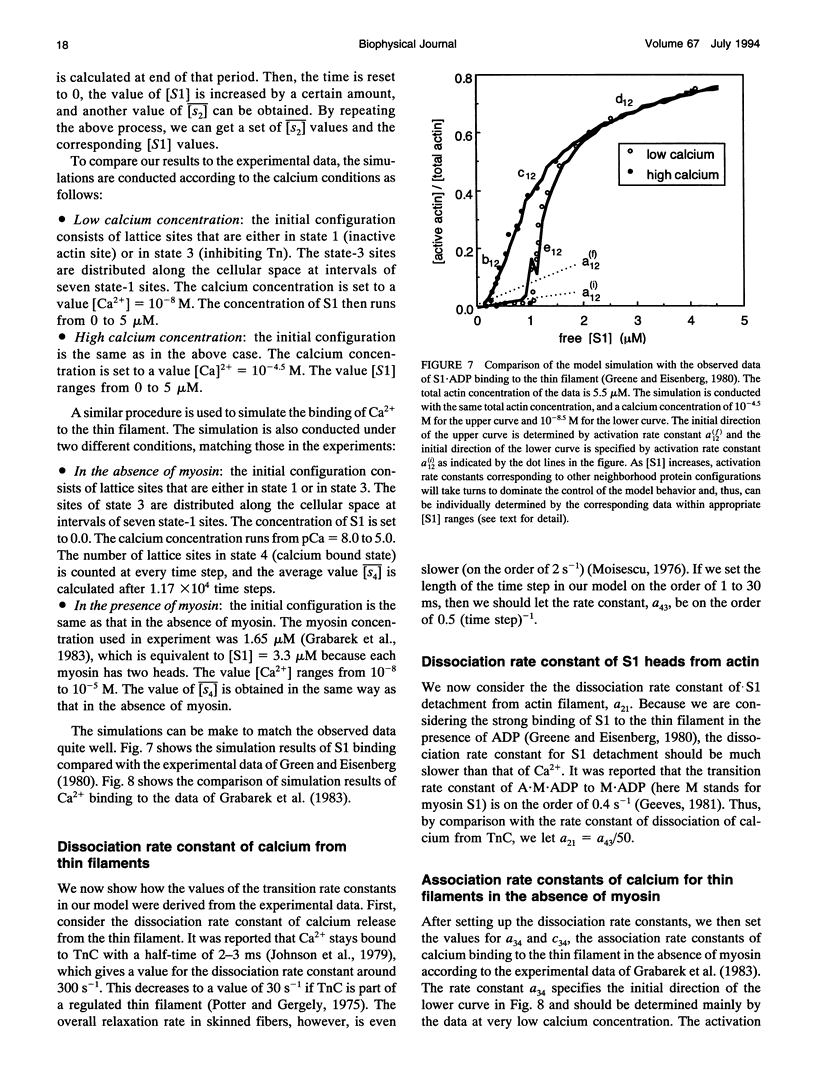
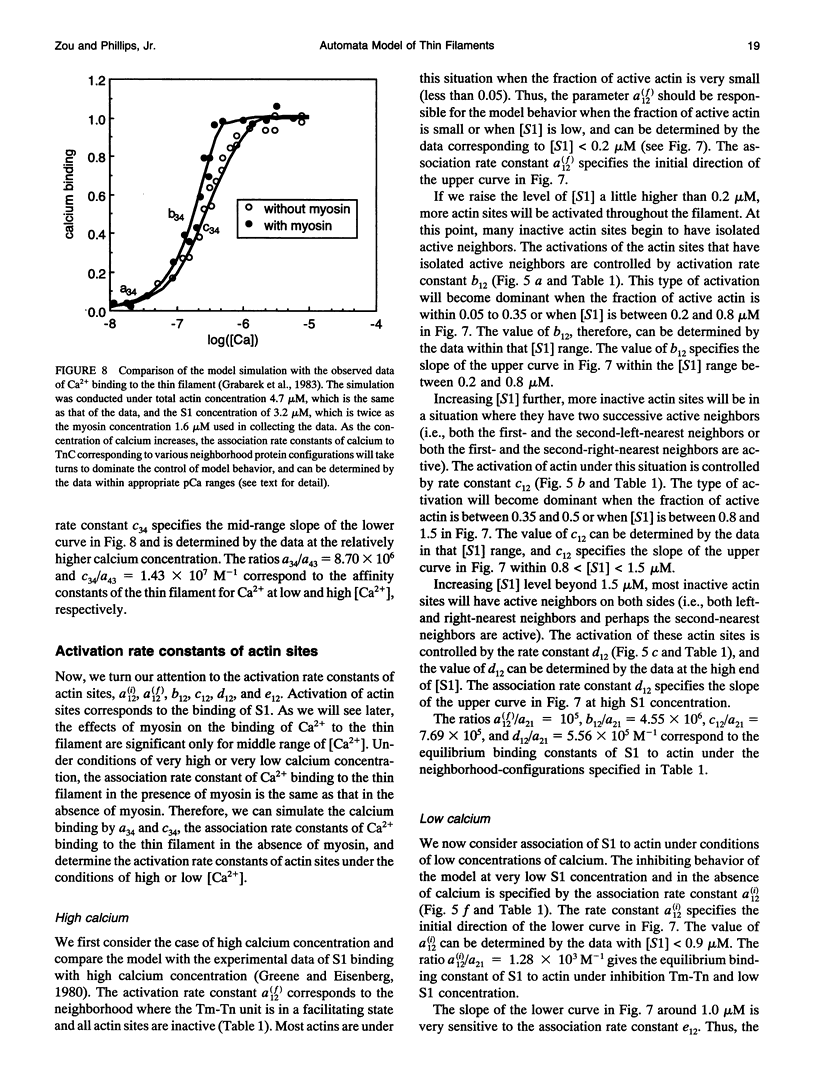
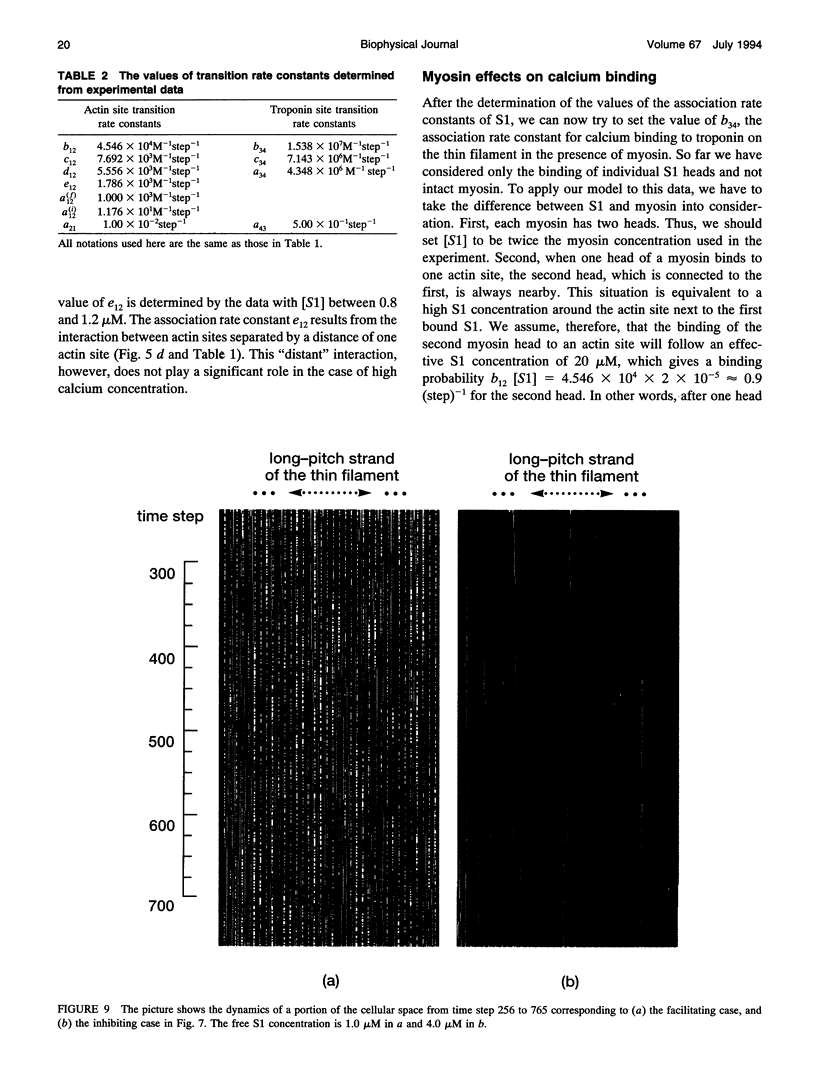
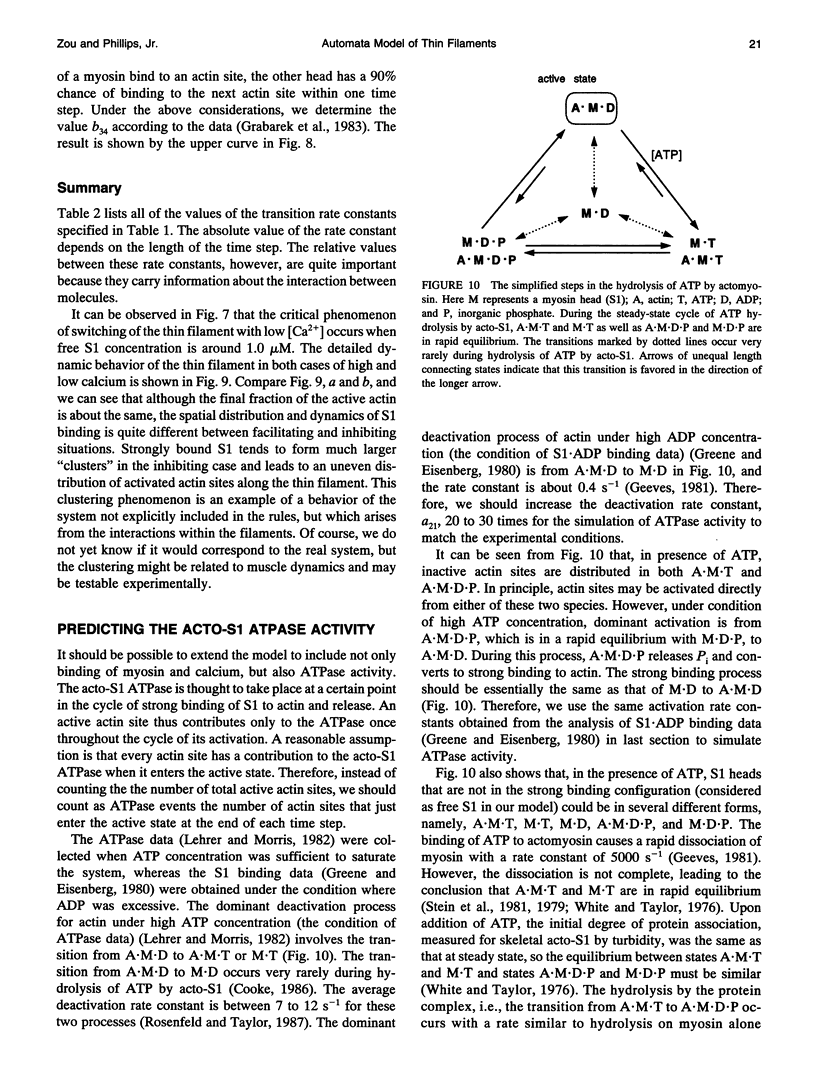
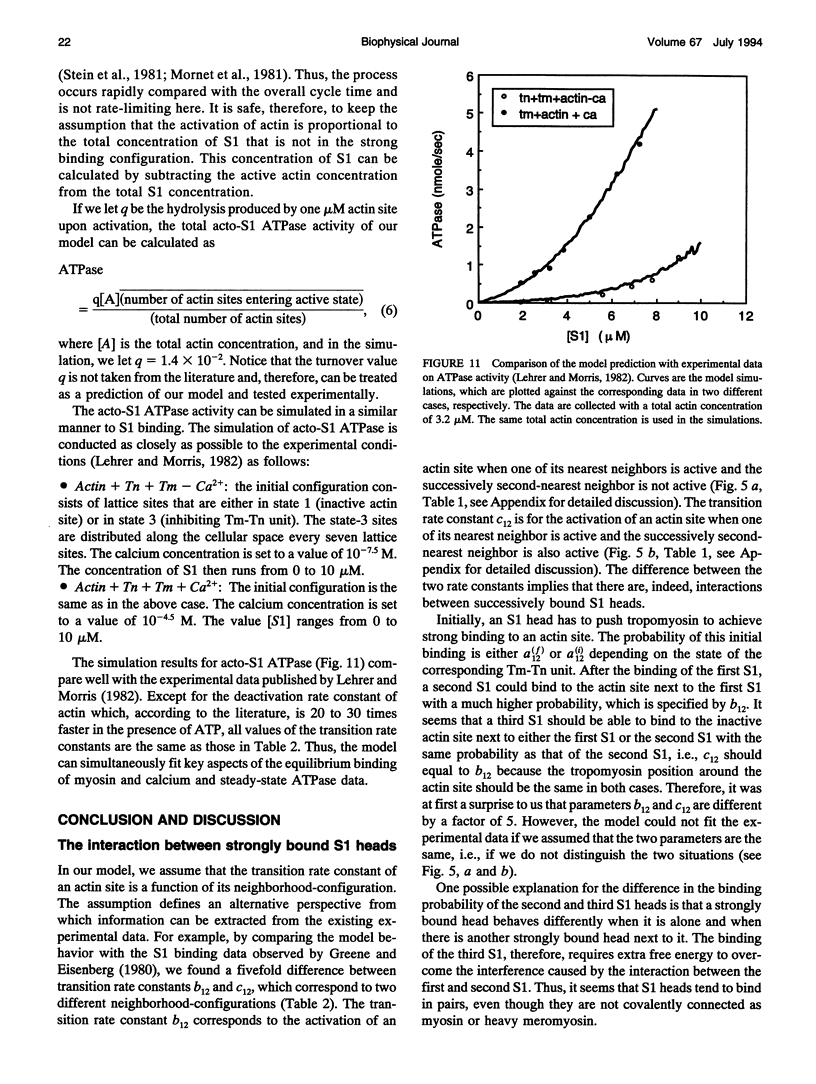
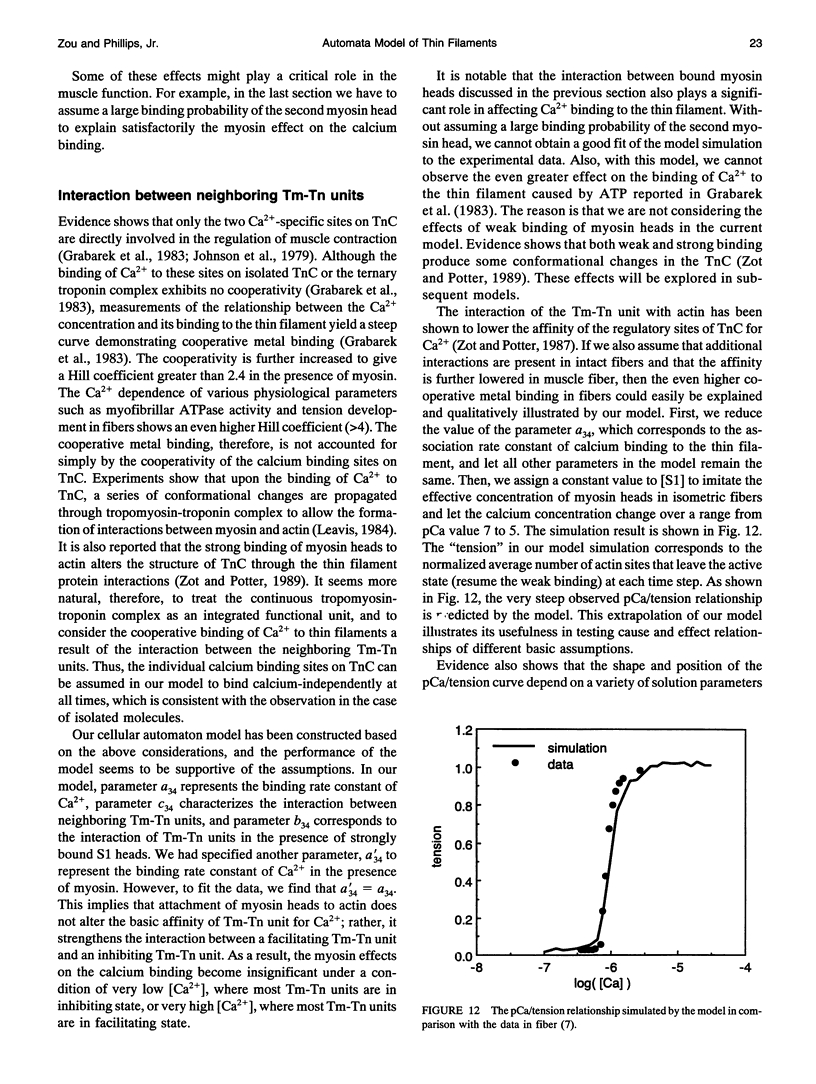
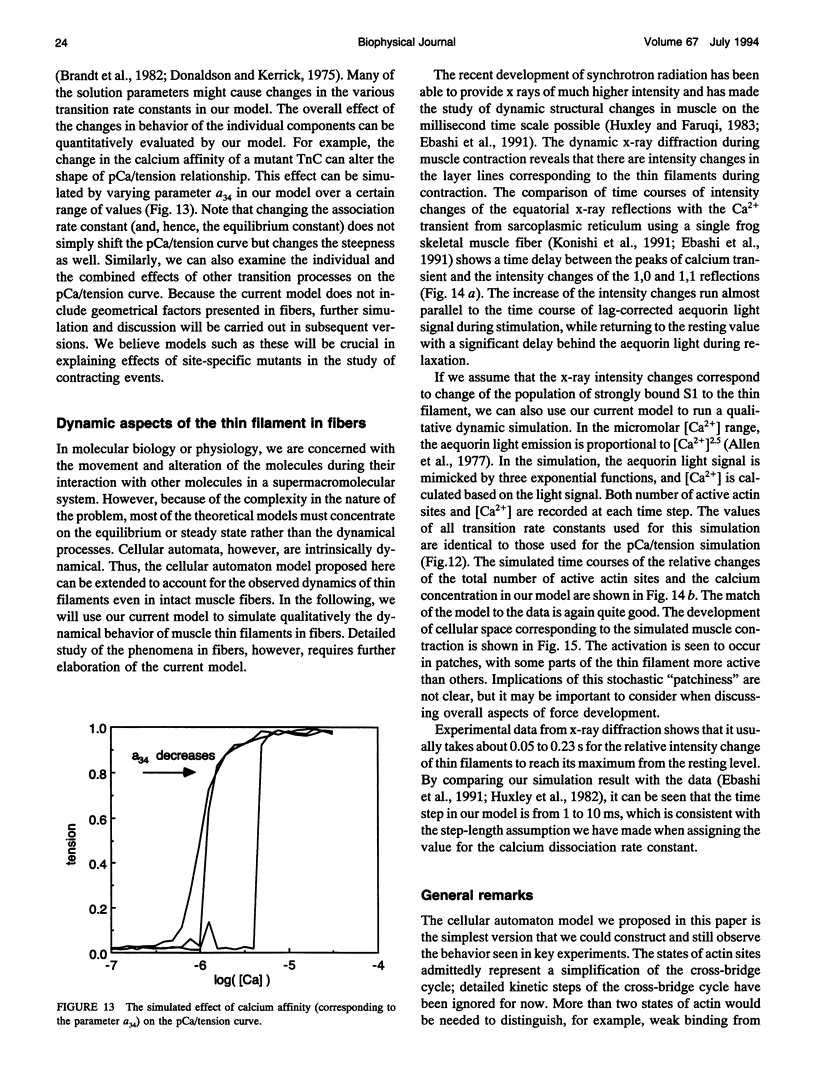
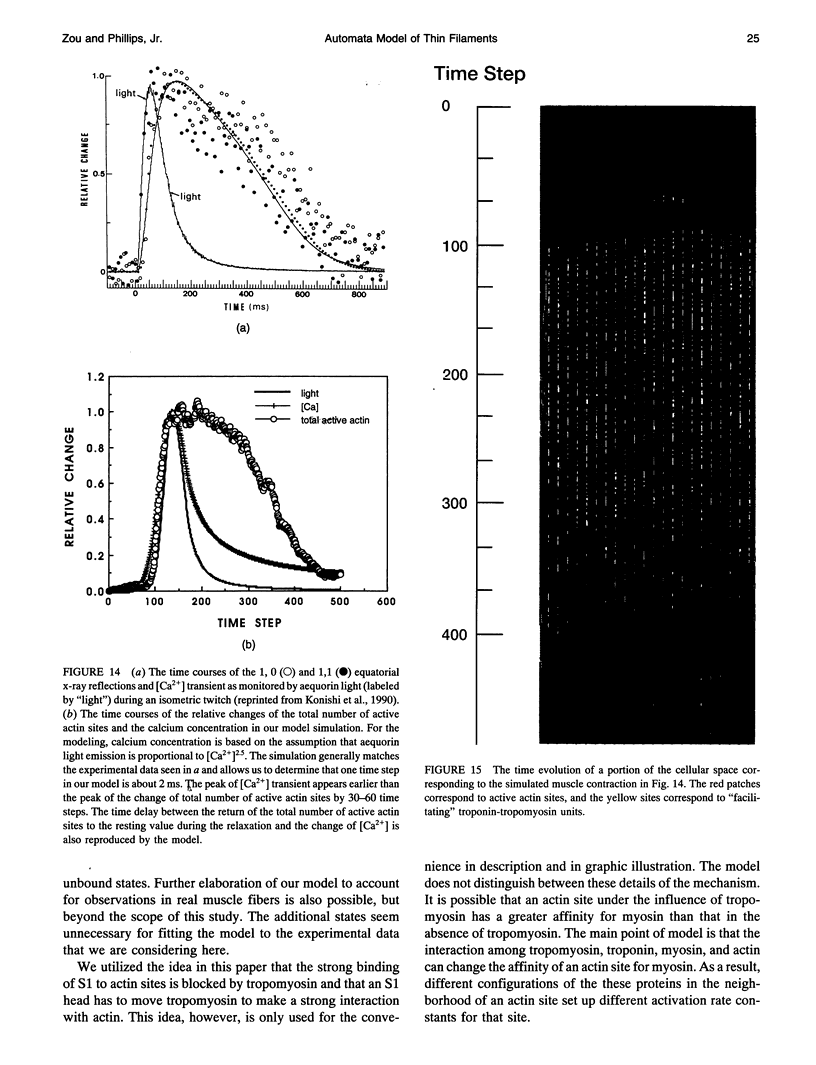
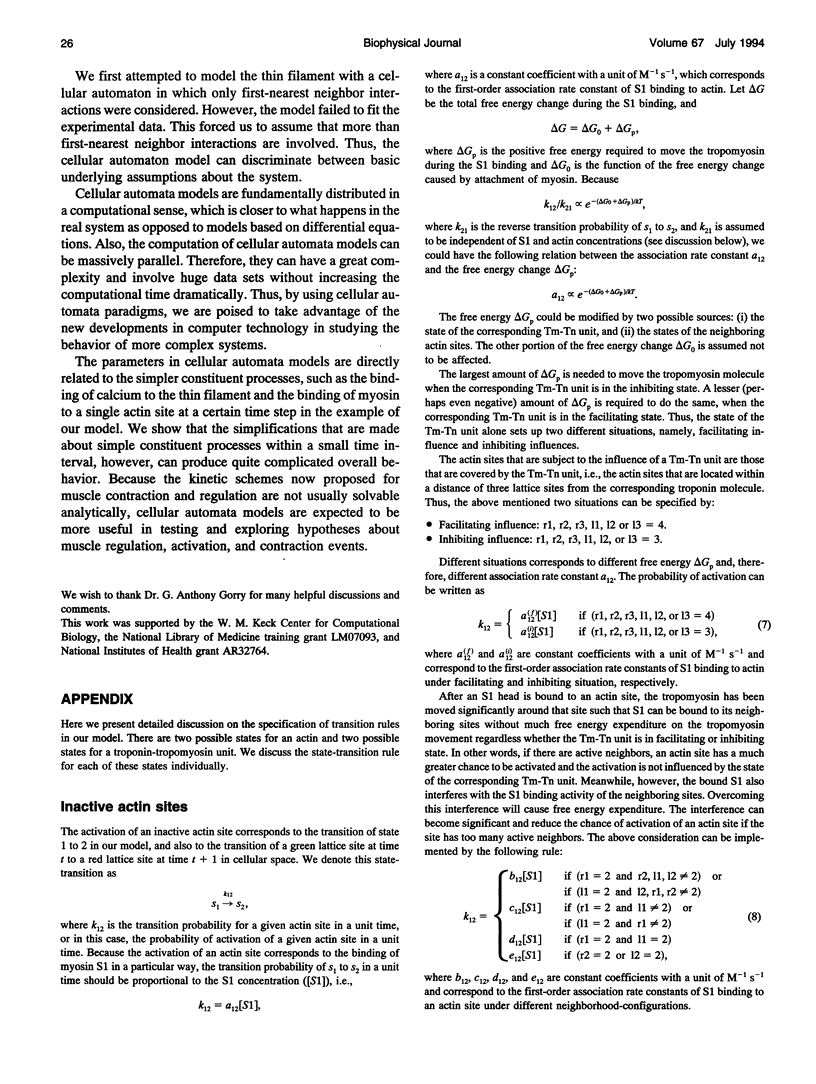
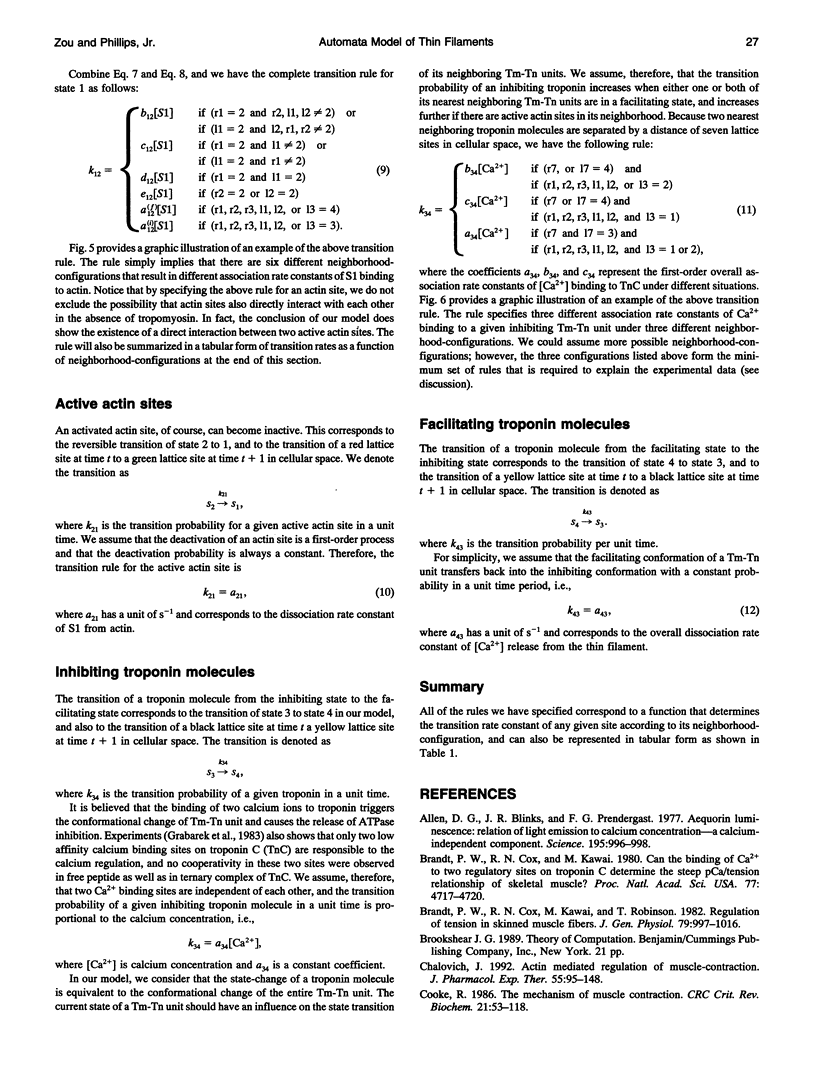
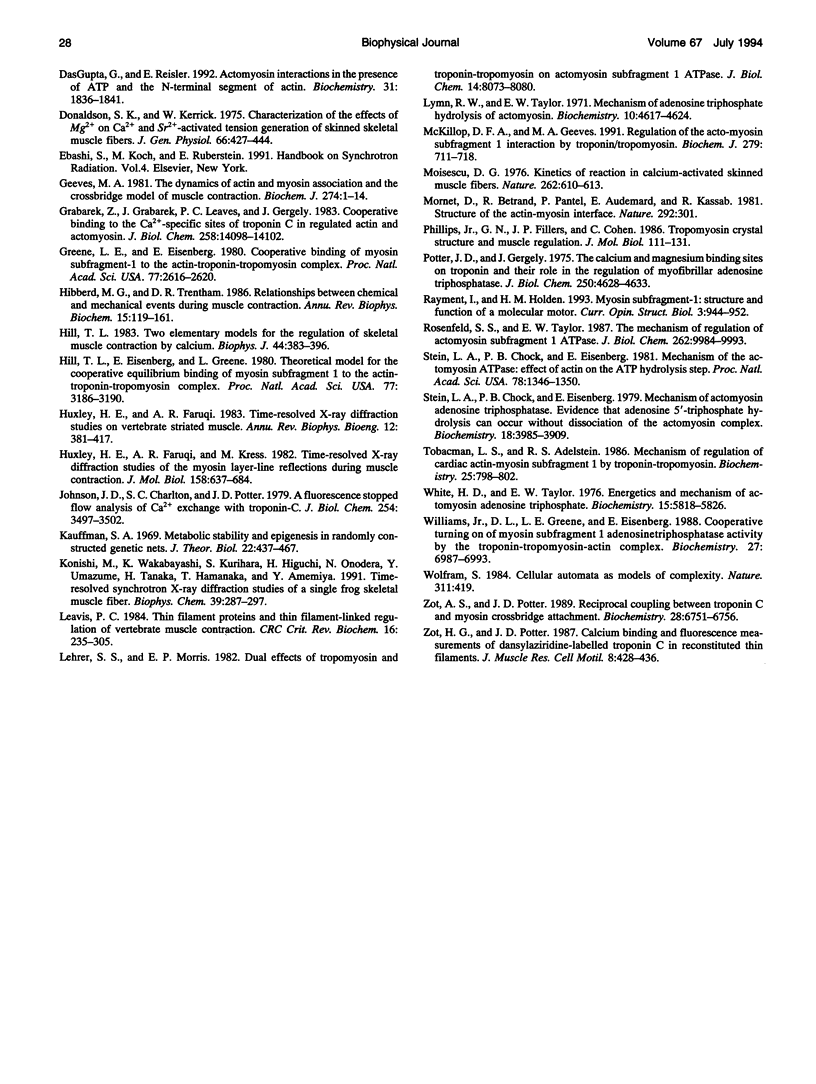
Images in this article
Selected References
These references are in PubMed. This may not be the complete list of references from this article.
- Allen D. G., Blinks J. R., Prendergast F. G. Aequorin luminescence: relation of light emission to calcium concentration--a calcium-independent component. Science. 1977 Mar 11;195(4282):996–998. doi: 10.1126/science.841325. [DOI] [PubMed] [Google Scholar]
- Brandt P. W., Cox R. N., Kawai M. Can the binding of Ca2+ to two regulatory sites on troponin C determine the steep pCa/tension relationship of skeletal muscle? Proc Natl Acad Sci U S A. 1980 Aug;77(8):4717–4720. doi: 10.1073/pnas.77.8.4717. [DOI] [PMC free article] [PubMed] [Google Scholar]
- Brandt P. W., Cox R. N., Kawai M., Robinson T. Effect of cross-bridge kinetics on apparent Ca2+ sensitivity. J Gen Physiol. 1982 Jun;79(6):997–1016. doi: 10.1085/jgp.79.6.997. [DOI] [PMC free article] [PubMed] [Google Scholar]
- Chalovich J. M. Actin mediated regulation of muscle contraction. Pharmacol Ther. 1992;55(2):95–148. doi: 10.1016/0163-7258(92)90013-p. [DOI] [PubMed] [Google Scholar]
- Cooke R. The mechanism of muscle contraction. CRC Crit Rev Biochem. 1986;21(1):53–118. doi: 10.3109/10409238609113609. [DOI] [PubMed] [Google Scholar]
- DasGupta G., Reisler E. Actomyosin interactions in the presence of ATP and the N-terminal segment of actin. Biochemistry. 1992 Feb 18;31(6):1836–1841. doi: 10.1021/bi00121a036. [DOI] [PubMed] [Google Scholar]
- Donaldson S. K., Kerrick W. G. Characterization of the effects of Mg2+ on Ca2+- and Sr2+-activated tension generation of skinned skeletal muscle fibers. J Gen Physiol. 1975 Oct;66(4):427–444. doi: 10.1085/jgp.66.4.427. [DOI] [PMC free article] [PubMed] [Google Scholar]
- Geeves M. A. The dynamics of actin and myosin association and the crossbridge model of muscle contraction. Biochem J. 1991 Feb 15;274(Pt 1):1–14. doi: 10.1042/bj2740001. [DOI] [PMC free article] [PubMed] [Google Scholar]
- Grabarek Z., Grabarek J., Leavis P. C., Gergely J. Cooperative binding to the Ca2+-specific sites of troponin C in regulated actin and actomyosin. J Biol Chem. 1983 Dec 10;258(23):14098–14102. [PubMed] [Google Scholar]
- Greene L. E., Eisenberg E. Cooperative binding of myosin subfragment-1 to the actin-troponin-tropomyosin complex. Proc Natl Acad Sci U S A. 1980 May;77(5):2616–2620. doi: 10.1073/pnas.77.5.2616. [DOI] [PMC free article] [PubMed] [Google Scholar]
- Hibberd M. G., Trentham D. R. Relationships between chemical and mechanical events during muscular contraction. Annu Rev Biophys Biophys Chem. 1986;15:119–161. doi: 10.1146/annurev.bb.15.060186.001003. [DOI] [PubMed] [Google Scholar]
- Hill T. L., Eisenberg E., Greene L. Theoretical model for the cooperative equilibrium binding of myosin subfragment 1 to the actin-troponin-tropomyosin complex. Proc Natl Acad Sci U S A. 1980 Jun;77(6):3186–3190. doi: 10.1073/pnas.77.6.3186. [DOI] [PMC free article] [PubMed] [Google Scholar]
- Hill T. L. Two elementary models for the regulation of skeletal muscle contraction by calcium. Biophys J. 1983 Dec;44(3):383–396. doi: 10.1016/S0006-3495(83)84312-4. [DOI] [PMC free article] [PubMed] [Google Scholar]
- Huxley H. E., Faruqi A. R., Kress M., Bordas J., Koch M. H. Time-resolved X-ray diffraction studies of the myosin layer-line reflections during muscle contraction. J Mol Biol. 1982 Jul 15;158(4):637–684. doi: 10.1016/0022-2836(82)90253-4. [DOI] [PubMed] [Google Scholar]
- Huxley H. E., Faruqi A. R. Time-resolved X-ray diffraction studies on vertebrate striated muscle. Annu Rev Biophys Bioeng. 1983;12:381–417. doi: 10.1146/annurev.bb.12.060183.002121. [DOI] [PubMed] [Google Scholar]
- Johnson J. D., Charlton S. C., Potter J. D. A fluorescence stopped flow analysis of Ca2+ exchange with troponin C. J Biol Chem. 1979 May 10;254(9):3497–3502. [PubMed] [Google Scholar]
- Kauffman S. A. Metabolic stability and epigenesis in randomly constructed genetic nets. J Theor Biol. 1969 Mar;22(3):437–467. doi: 10.1016/0022-5193(69)90015-0. [DOI] [PubMed] [Google Scholar]
- Konishi M., Wakabayashi K., Kurihara S., Higuchi H., Onodera N., Umazume Y., Tanaka H., Hamanaka T., Amemiya Y. Time-resolved synchrotron X-ray diffraction studies of a single frog skeletal muscle fiber. Time courses of intensity changes of the equatorial reflections and intracellular Ca2+ transients. Biophys Chem. 1991 Mar;39(3):287–297. doi: 10.1016/0301-4622(91)80007-e. [DOI] [PubMed] [Google Scholar]
- Leavis P. C., Gergely J. Thin filament proteins and thin filament-linked regulation of vertebrate muscle contraction. CRC Crit Rev Biochem. 1984;16(3):235–305. doi: 10.3109/10409238409108717. [DOI] [PubMed] [Google Scholar]
- Lehrer S. S., Morris E. P. Dual effects of tropomyosin and troponin-tropomyosin on actomyosin subfragment 1 ATPase. J Biol Chem. 1982 Jul 25;257(14):8073–8080. [PubMed] [Google Scholar]
- Lymn R. W., Taylor E. W. Mechanism of adenosine triphosphate hydrolysis by actomyosin. Biochemistry. 1971 Dec 7;10(25):4617–4624. doi: 10.1021/bi00801a004. [DOI] [PubMed] [Google Scholar]
- McKillop D. F., Geeves M. A. Regulation of the acto.myosin subfragment 1 interaction by troponin/tropomyosin. Evidence for control of a specific isomerization between two acto.myosin subfragment 1 states. Biochem J. 1991 Nov 1;279(Pt 3):711–718. doi: 10.1042/bj2790711. [DOI] [PMC free article] [PubMed] [Google Scholar]
- Moisescu D. G. Kinetics of reaction in calcium-activated skinned muscle fibres. Nature. 1976 Aug 12;262(5569):610–613. doi: 10.1038/262610a0. [DOI] [PubMed] [Google Scholar]
- Mornet D., Bertrand R., Pantel P., Audemard E., Kassab R. Structure of the actin-myosin interface. Nature. 1981 Jul 23;292(5821):301–306. doi: 10.1038/292301a0. [DOI] [PubMed] [Google Scholar]
- Phillips G. N., Jr, Fillers J. P., Cohen C. Tropomyosin crystal structure and muscle regulation. J Mol Biol. 1986 Nov 5;192(1):111–131. doi: 10.1016/0022-2836(86)90468-7. [DOI] [PubMed] [Google Scholar]
- Potter J. D., Gergely J. The calcium and magnesium binding sites on troponin and their role in the regulation of myofibrillar adenosine triphosphatase. J Biol Chem. 1975 Jun 25;250(12):4628–4633. [PubMed] [Google Scholar]
- Rosenfeld S. S., Taylor E. W. The mechanism of regulation of actomyosin subfragment 1 ATPase. J Biol Chem. 1987 Jul 25;262(21):9984–9993. [PubMed] [Google Scholar]
- Stein L. A., Chock P. B., Eisenberg E. Mechanism of the actomyosin ATPase: effect of actin on the ATP hydrolysis step. Proc Natl Acad Sci U S A. 1981 Mar;78(3):1346–1350. doi: 10.1073/pnas.78.3.1346. [DOI] [PMC free article] [PubMed] [Google Scholar]
- Stein L. A., Schwarz R. P., Jr, Chock P. B., Eisenberg E. Mechanism of actomyosin adenosine triphosphatase. Evidence that adenosine 5'-triphosphate hydrolysis can occur without dissociation of the actomyosin complex. Biochemistry. 1979 Sep 4;18(18):3895–3909. doi: 10.1021/bi00585a009. [DOI] [PubMed] [Google Scholar]
- Tobacman L. S., Adelstein R. S. Mechanism of regulation of cardiac actin-myosin subfragment 1 by troponin-tropomyosin. Biochemistry. 1986 Feb 25;25(4):798–802. doi: 10.1021/bi00352a010. [DOI] [PubMed] [Google Scholar]
- White H. D., Taylor E. W. Energetics and mechanism of actomyosin adenosine triphosphatase. Biochemistry. 1976 Dec 28;15(26):5818–5826. doi: 10.1021/bi00671a020. [DOI] [PubMed] [Google Scholar]
- Williams D. L., Jr, Greene L. E., Eisenberg E. Cooperative turning on of myosin subfragment 1 adenosinetriphosphatase activity by the troponin-tropomyosin-actin complex. Biochemistry. 1988 Sep 6;27(18):6987–6993. doi: 10.1021/bi00418a048. [DOI] [PubMed] [Google Scholar]
- Zot A. S., Potter J. D. Reciprocal coupling between troponin C and myosin crossbridge attachment. Biochemistry. 1989 Aug 8;28(16):6751–6756. doi: 10.1021/bi00442a031. [DOI] [PubMed] [Google Scholar]
- Zot H. G., Potter J. D. Calcium binding and fluorescence measurements of dansylaziridine-labelled troponin C in reconstituted thin filaments. J Muscle Res Cell Motil. 1987 Oct;8(5):428–436. doi: 10.1007/BF01578432. [DOI] [PubMed] [Google Scholar]