Abstract
The effects of different cations on the hydrodynamic radius (RH) of a 48-bp synthetic DNA are measured by time-resolved fluorescence polarization anisotropy of intercalated ethidium. Relative statistical errors in RH are only approximately 1%. With increasing cation concentration, Na+ causes a small decrease in RH, Cs+ causes a somewhat larger decrease by up to 0.5 A at 100 mM, and (CH3CH2)4N+ causes an increase in RH by approximately 0.5 A at 100 mM. The qualitatively different effects of these monovalent cations indicates that the changes in RH with cation concentration do not arise primarily from electrolyte friction. Divalent cations cause much larger increases in RH with increasing cation concentration. Mg2+ causes an increase in RH by up to 1.0 A at 24.4 mM, and Mn2+ causes an increase in RH by up to 1.6 A at 24.4 mM. These effects are independent of DNA concentration. There is some positive correlation between the order of effects of the different cations on RH and the order of their effects on interhelical hydration forces. It is suggested that these different ions affect RH either by altering the hydration layer or possibly by some effect on DNA structure, such as stabilizing bends.
Full text
PDF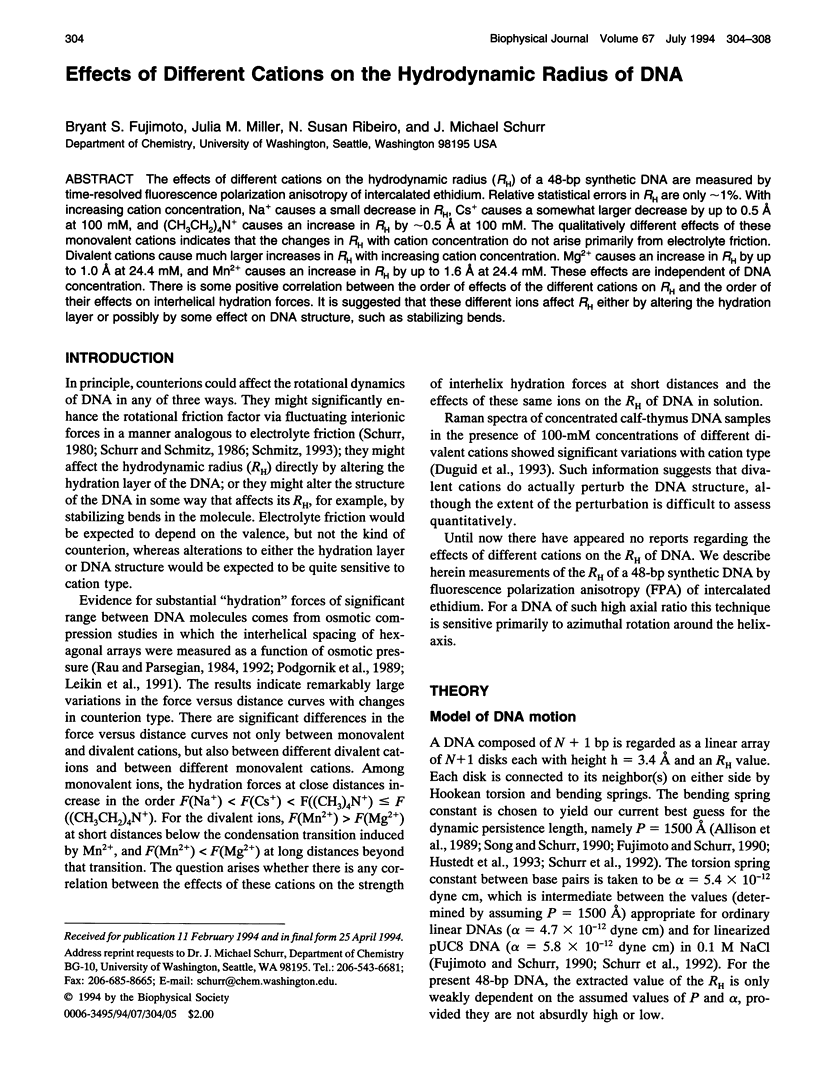
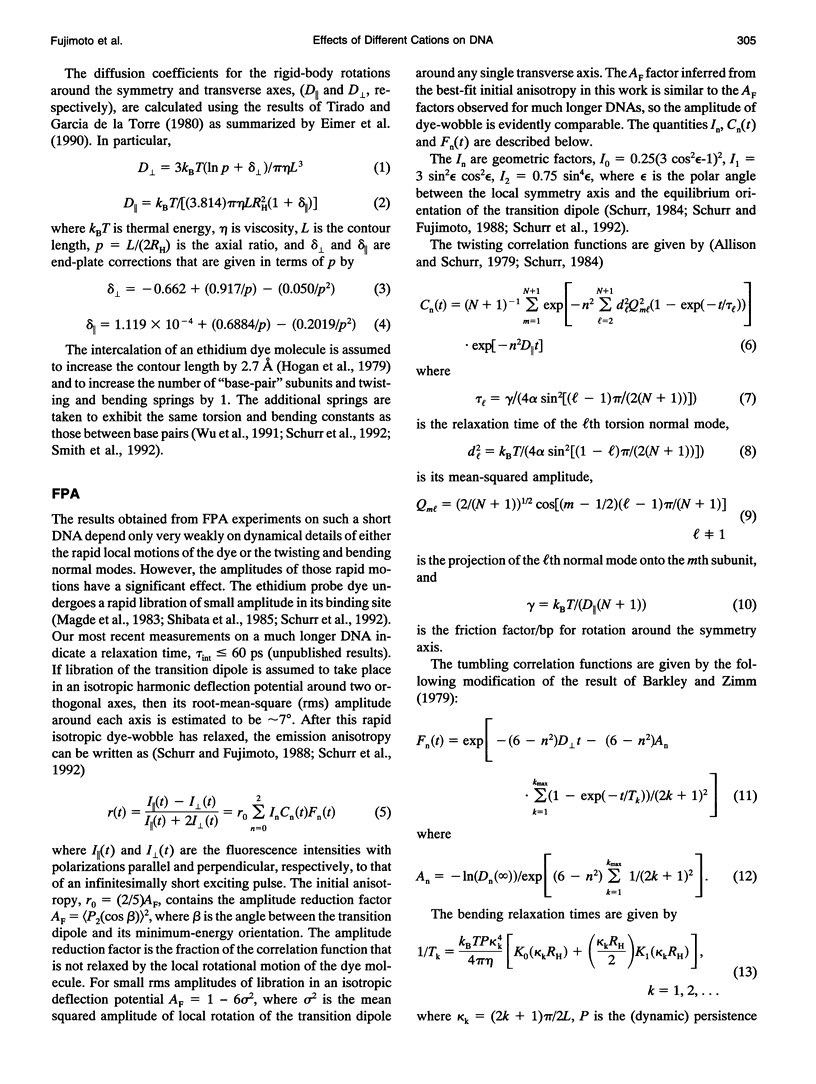
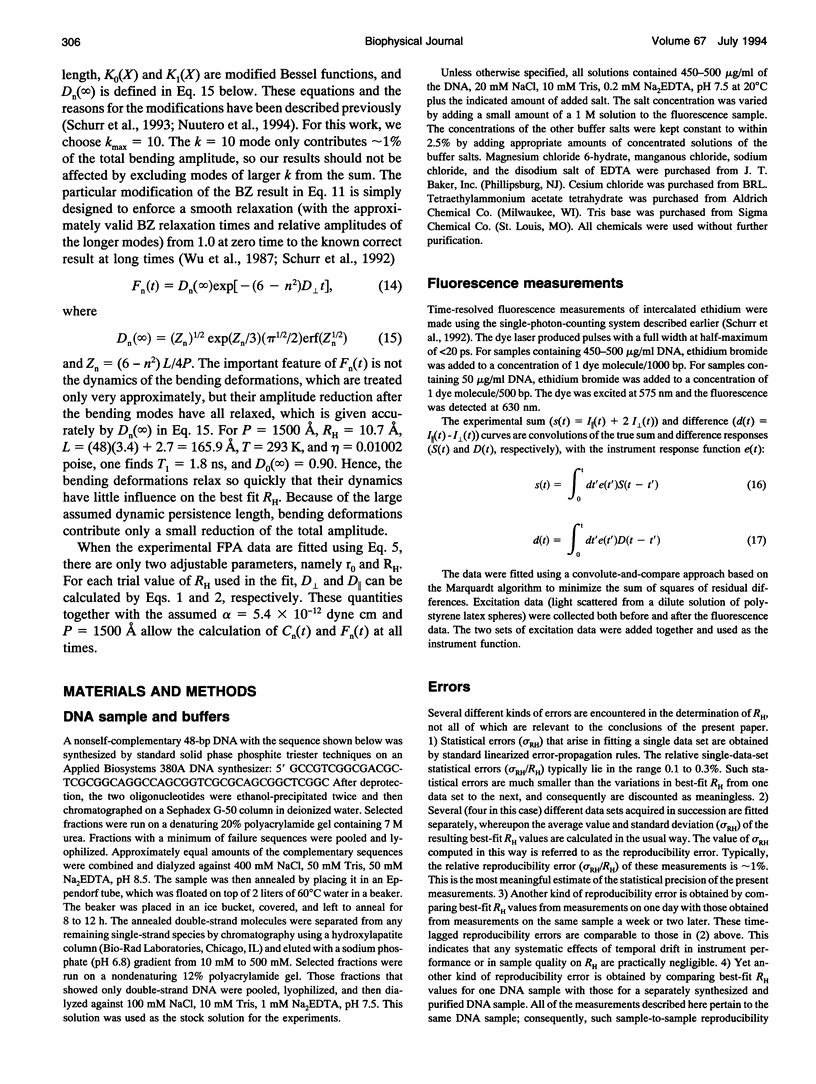
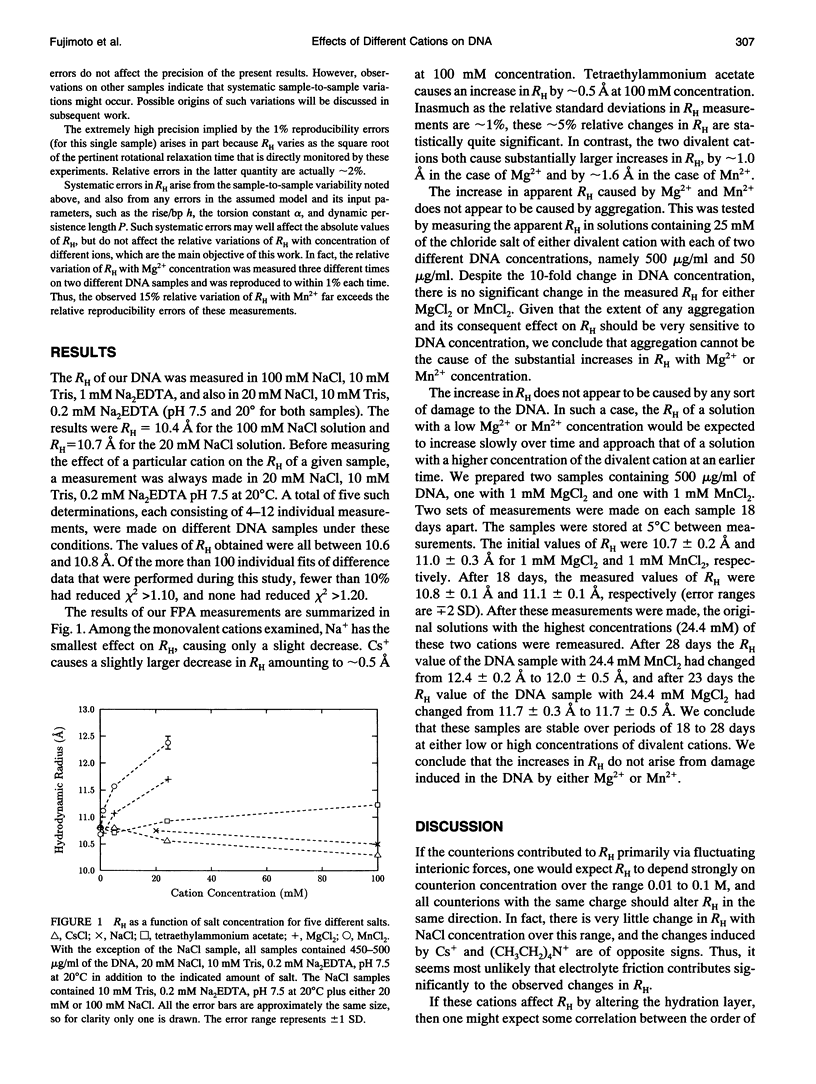
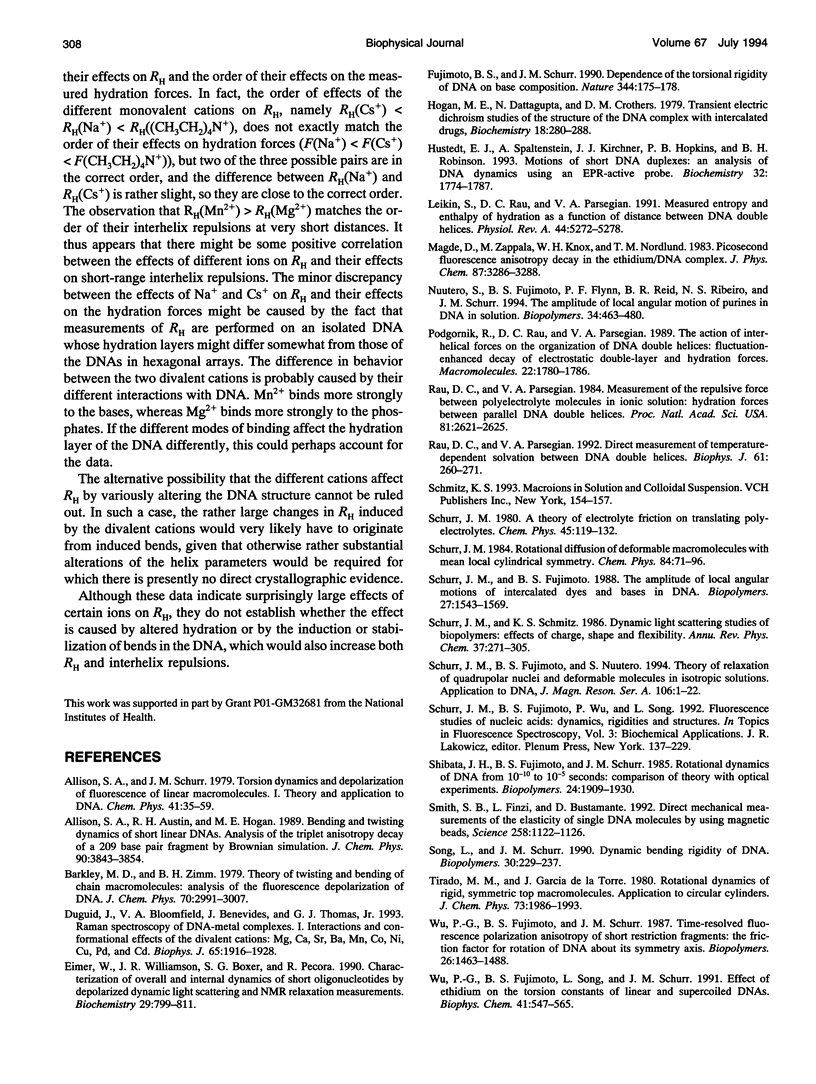
Selected References
These references are in PubMed. This may not be the complete list of references from this article.
- Duguid J., Bloomfield V. A., Benevides J., Thomas G. J., Jr Raman spectroscopy of DNA-metal complexes. I. Interactions and conformational effects of the divalent cations: Mg, Ca, Sr, Ba, Mn, Co, Ni, Cu, Pd, and Cd. Biophys J. 1993 Nov;65(5):1916–1928. doi: 10.1016/S0006-3495(93)81263-3. [DOI] [PMC free article] [PubMed] [Google Scholar]
- Eimer W., Williamson J. R., Boxer S. G., Pecora R. Characterization of the overall and internal dynamics of short oligonucleotides by depolarized dynamic light scattering and NMR relaxation measurements. Biochemistry. 1990 Jan 23;29(3):799–811. doi: 10.1021/bi00455a030. [DOI] [PubMed] [Google Scholar]
- Fujimoto B. S., Schurr J. M. Dependence of the torsional rigidity of DNA on base composition. Nature. 1990 Mar 8;344(6262):175–177. doi: 10.1038/344175a0. [DOI] [PubMed] [Google Scholar]
- Hogan M., Dattagupta N., Crothers D. M. Transient electric dichroism studies of the structure of the DNA complex with intercalated drugs. Biochemistry. 1979 Jan 23;18(2):280–288. doi: 10.1021/bi00569a007. [DOI] [PubMed] [Google Scholar]
- Hustedt E. J., Spaltenstein A., Kirchner J. J., Hopkins P. B., Robinson B. H. Motions of short DNA duplexes: an analysis of DNA dynamics using an EPR-active probe. Biochemistry. 1993 Feb 23;32(7):1774–1787. doi: 10.1021/bi00058a011. [DOI] [PubMed] [Google Scholar]
- Leikin S, Rau DC, Parsegian VA. Measured entropy and enthalpy of hydration as a function of distance between DNA double helices. Phys Rev A. 1991 Oct 15;44(8):5272–5278. doi: 10.1103/physreva.44.5272. [DOI] [PubMed] [Google Scholar]
- Nuutero S., Fujimoto B. S., Flynn P. F., Reid B. R., Ribeiro N. S., Schurr J. M. The amplitude of local angular motion of purines in DNA in solution. Biopolymers. 1994 Apr;34(4):463–480. doi: 10.1002/bip.360340404. [DOI] [PubMed] [Google Scholar]
- Rau D. C., Lee B., Parsegian V. A. Measurement of the repulsive force between polyelectrolyte molecules in ionic solution: hydration forces between parallel DNA double helices. Proc Natl Acad Sci U S A. 1984 May;81(9):2621–2625. doi: 10.1073/pnas.81.9.2621. [DOI] [PMC free article] [PubMed] [Google Scholar]
- Rau D. C., Parsegian V. A. Direct measurement of temperature-dependent solvation forces between DNA double helices. Biophys J. 1992 Jan;61(1):260–271. doi: 10.1016/S0006-3495(92)81832-5. [DOI] [PMC free article] [PubMed] [Google Scholar]
- Schurr J. M., Fujimoto B. S. The amplitude of local angular motions of intercalated dyes and bases in DNA. Biopolymers. 1988 Oct;27(10):1543–1569. doi: 10.1002/bip.360271003. [DOI] [PubMed] [Google Scholar]
- Shibata J. H., Fujimoto B. S., Schurr J. M. Rotational dynamics of DNA from 10(-10) to 10(-5) seconds: comparison of theory with optical experiments. Biopolymers. 1985 Oct;24(10):1909–1930. doi: 10.1002/bip.360241006. [DOI] [PubMed] [Google Scholar]
- Smith S. B., Finzi L., Bustamante C. Direct mechanical measurements of the elasticity of single DNA molecules by using magnetic beads. Science. 1992 Nov 13;258(5085):1122–1126. doi: 10.1126/science.1439819. [DOI] [PubMed] [Google Scholar]
- Song L., Schurr J. M. Dynamic bending rigidity of DNA. Biopolymers. 1990;30(3-4):229–237. doi: 10.1002/bip.360300302. [DOI] [PubMed] [Google Scholar]
- Wu P., Fujimoto B. S., Schurr J. M. Time-resolved fluorescence polarization anisotropy of short restriction fragments: the friction factor for rotation of DNA about its symmetry axis. Biopolymers. 1987 Sep;26(9):1463–1488. doi: 10.1002/bip.360260903. [DOI] [PubMed] [Google Scholar]