Abstract
To link quantitatively the cell surface binding of ligand to receptor with the production of cellular responses, it may be necessary to explore early events in signal transduction such as G-protein activation. Two different model frameworks relating receptor/ligand binding to G-protein activation are examined. In the first framework, a simple ordinary differential equation model is used to describe receptor/ligand binding and G-protein activation. In the second framework, the events leading to G-protein activation are simulated using a dynamic Monte Carlo model. In both models, reactions between ligand-bound receptors and G-proteins are assumed to be diffusion-limited. The Monte Carlo model predicts two regimes of G-protein activation, depending upon whether the lifetime of a receptor/ligand complex is long or short compared with the time needed for diffusional encounters of complexes and G-proteins. When the lifetime of a complex is relatively short compared with the diffusion time, the movement of ligand among free receptors by binding and unbinding ("switching") significantly enhances G-protein activation. Receptor antagonists dramatically reduce G-protein activation and, thus, signal transduction in this case, and significant clustering of active G-proteins near receptor/ligand complexes results. The simple ordinary differential equation model poorly predicts G-protein activation for this situation. In the alternative case, when diffusion is relatively fast, ligand movement among receptors is less important and the simple ordinary differential equation model and Monte Carlo model results are similar. In this case, there is little clustering of active G-proteins near receptor/ligand complexes. Results also indicate that as the GTPase activity of the alpha-subunit decreases, the steady-state level of alpha-GTP increases, although temporal sensitivity is compromised.
Full text
PDF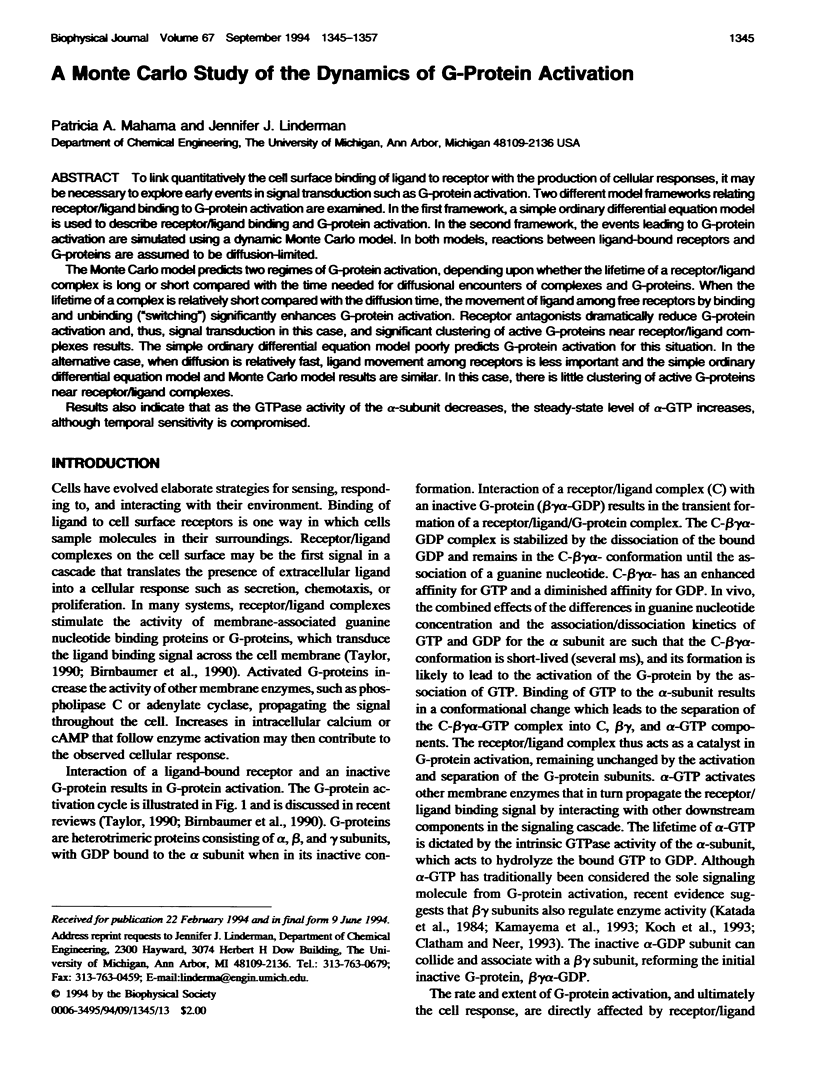
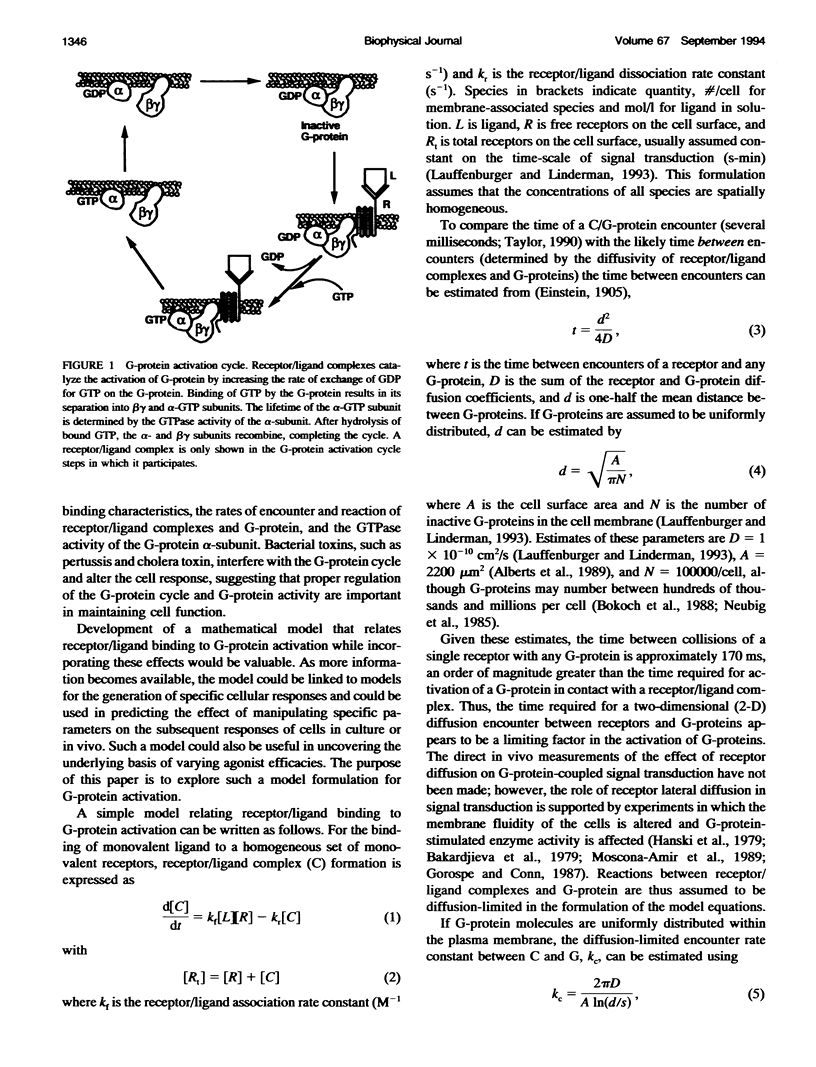
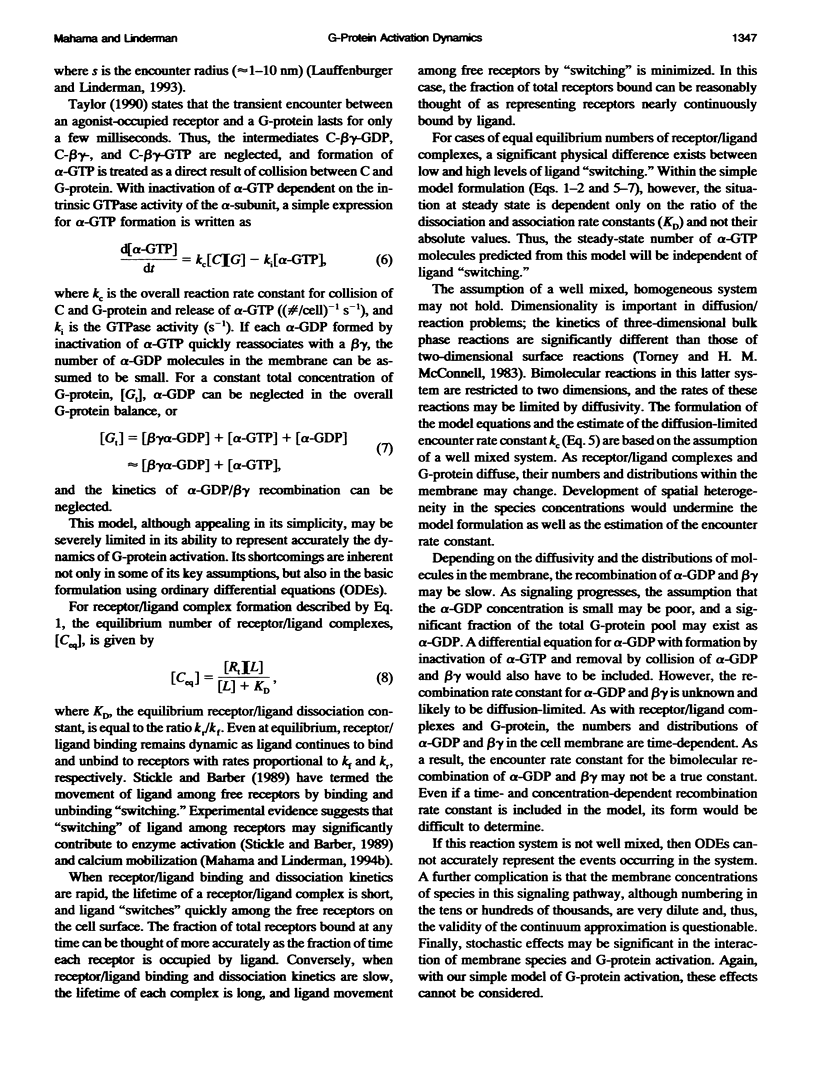
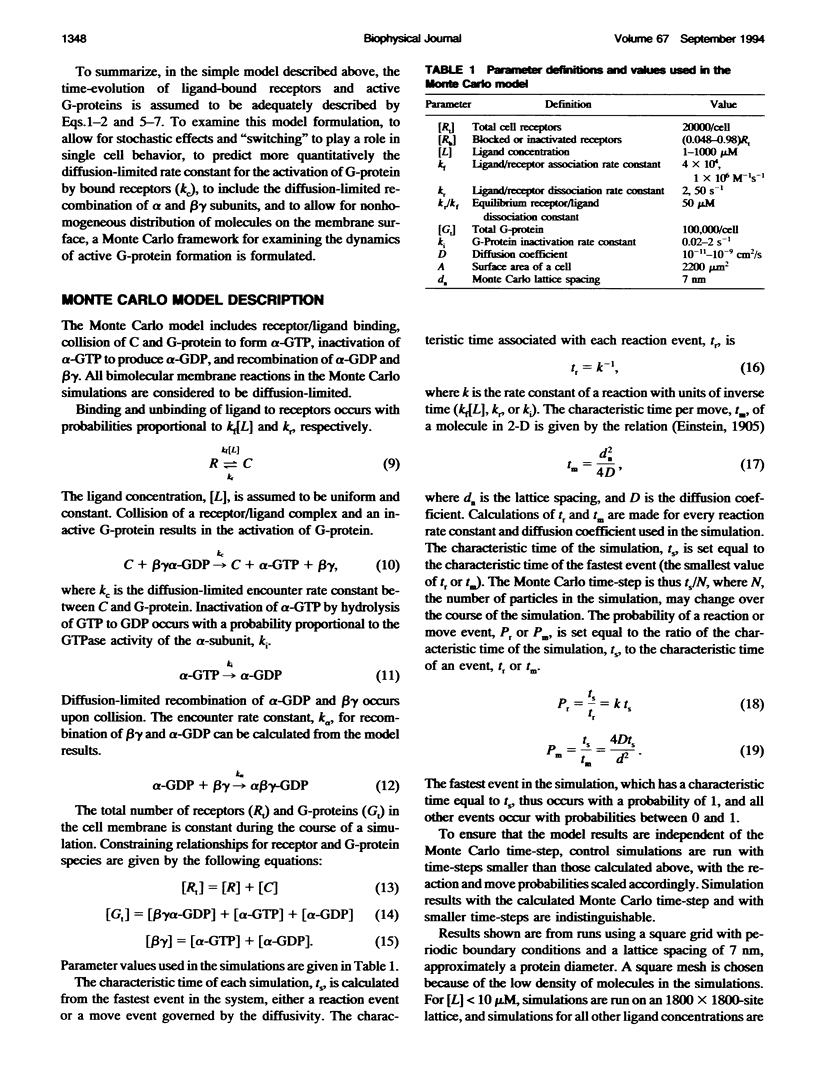

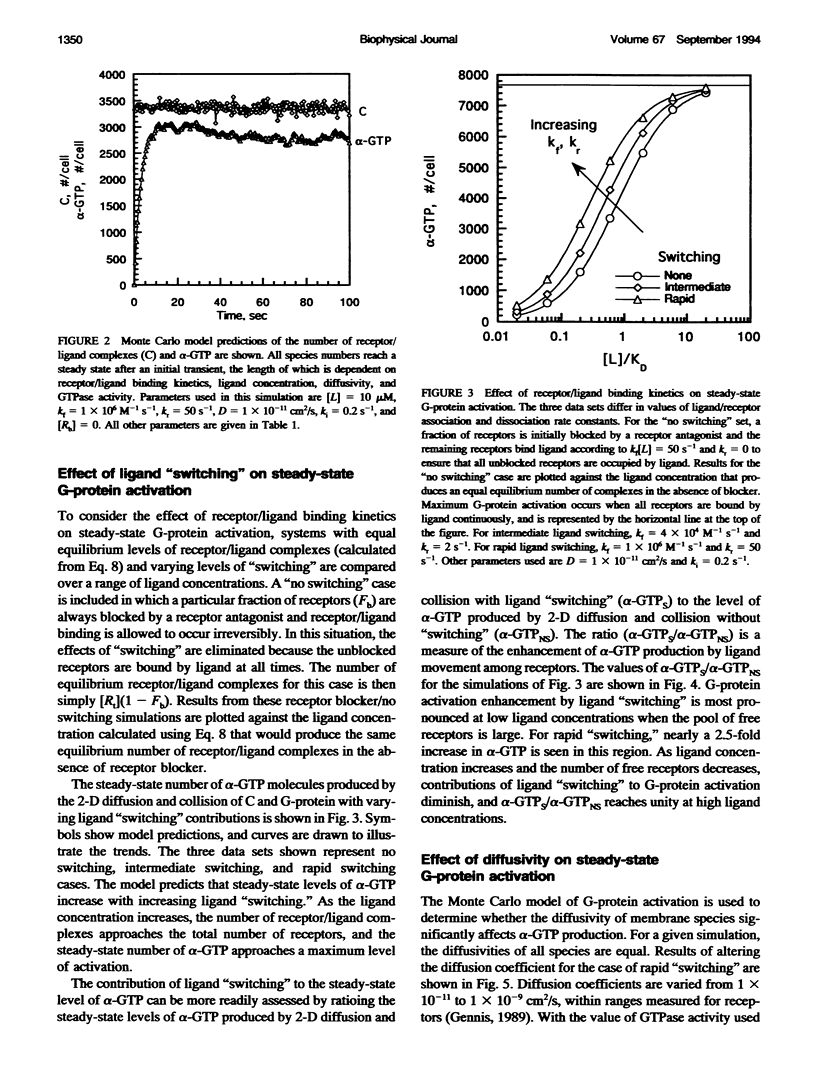
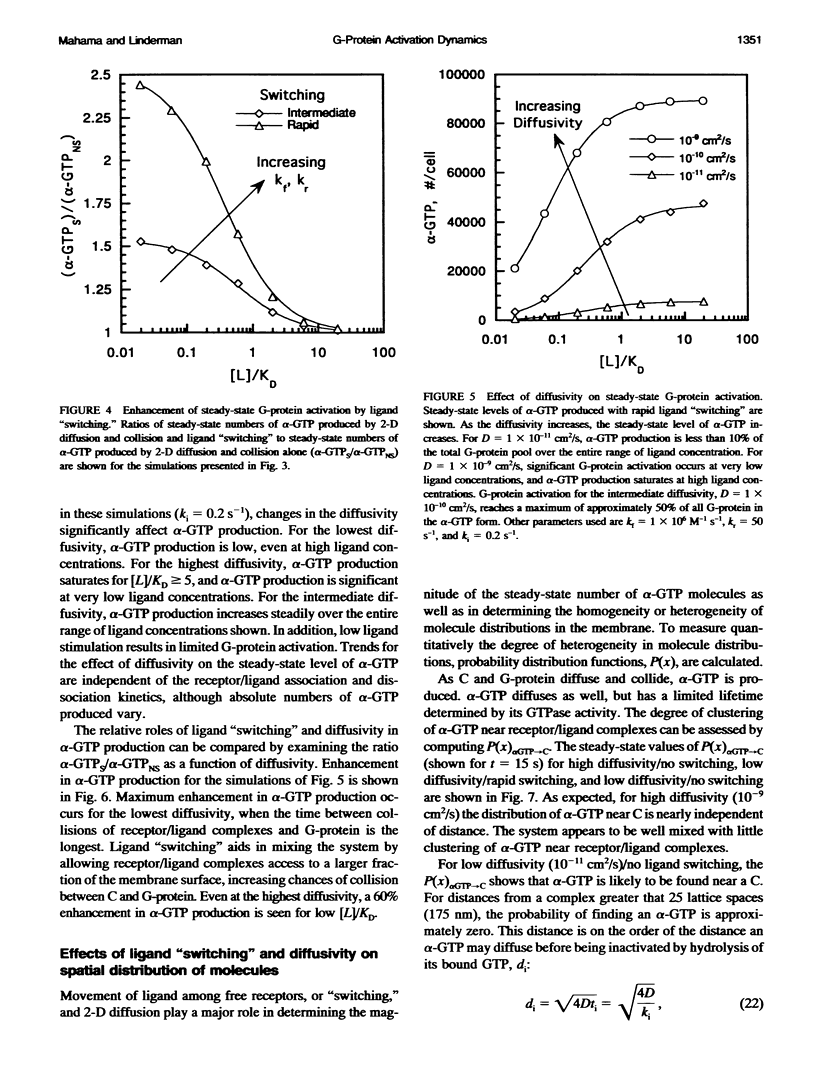
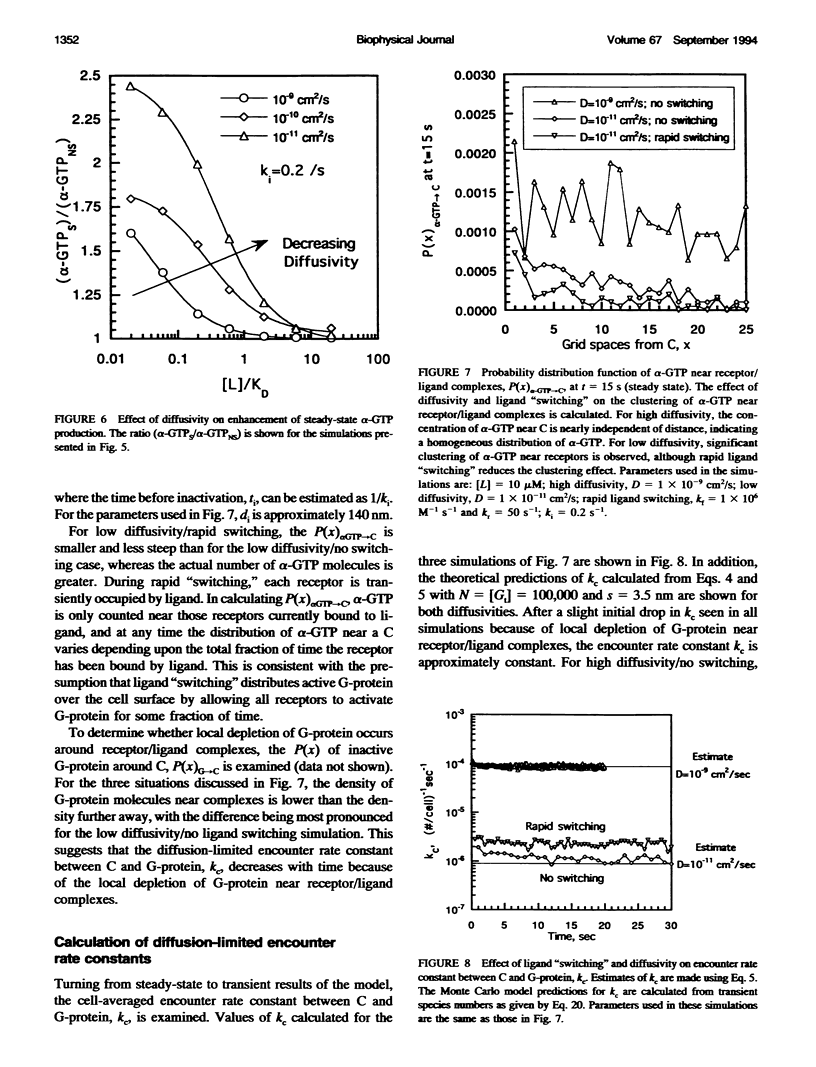
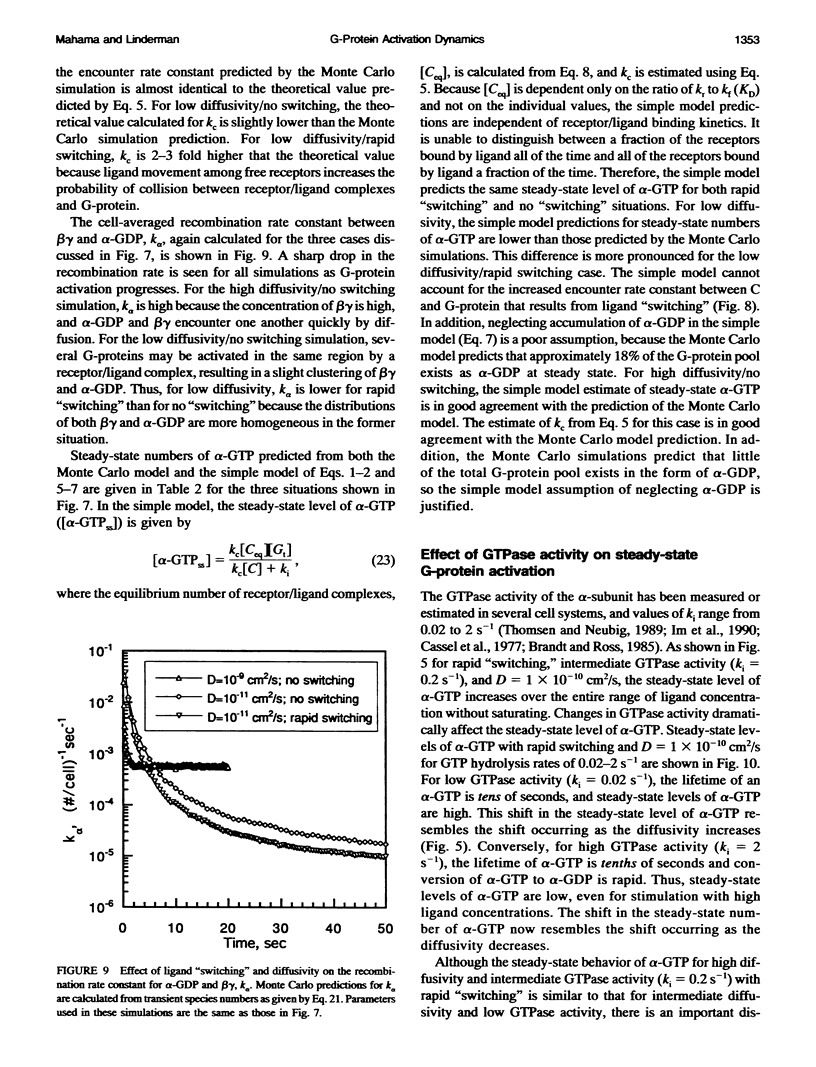
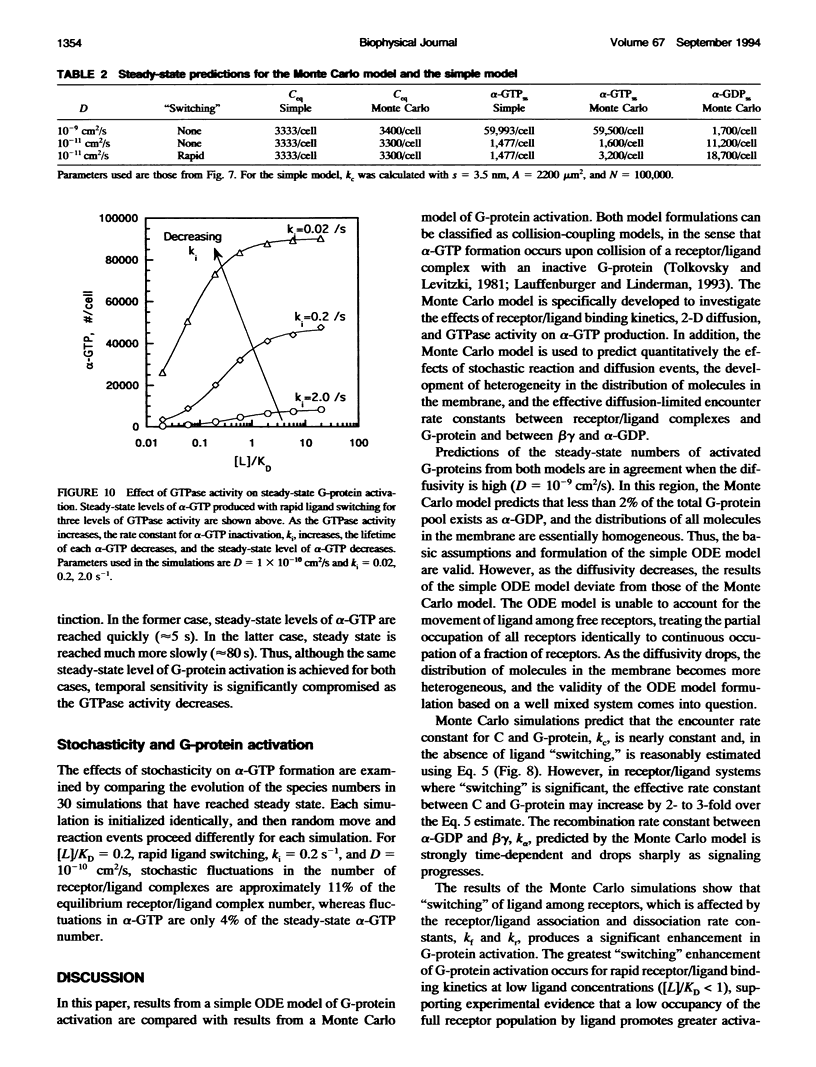
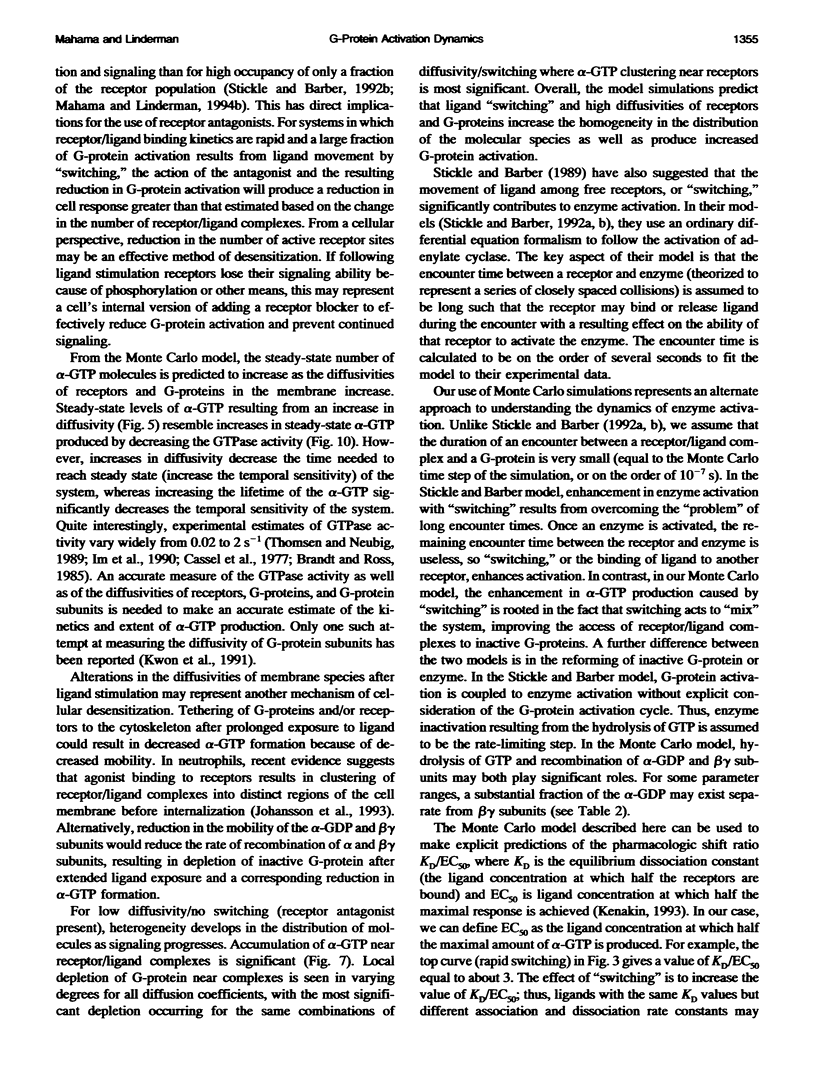
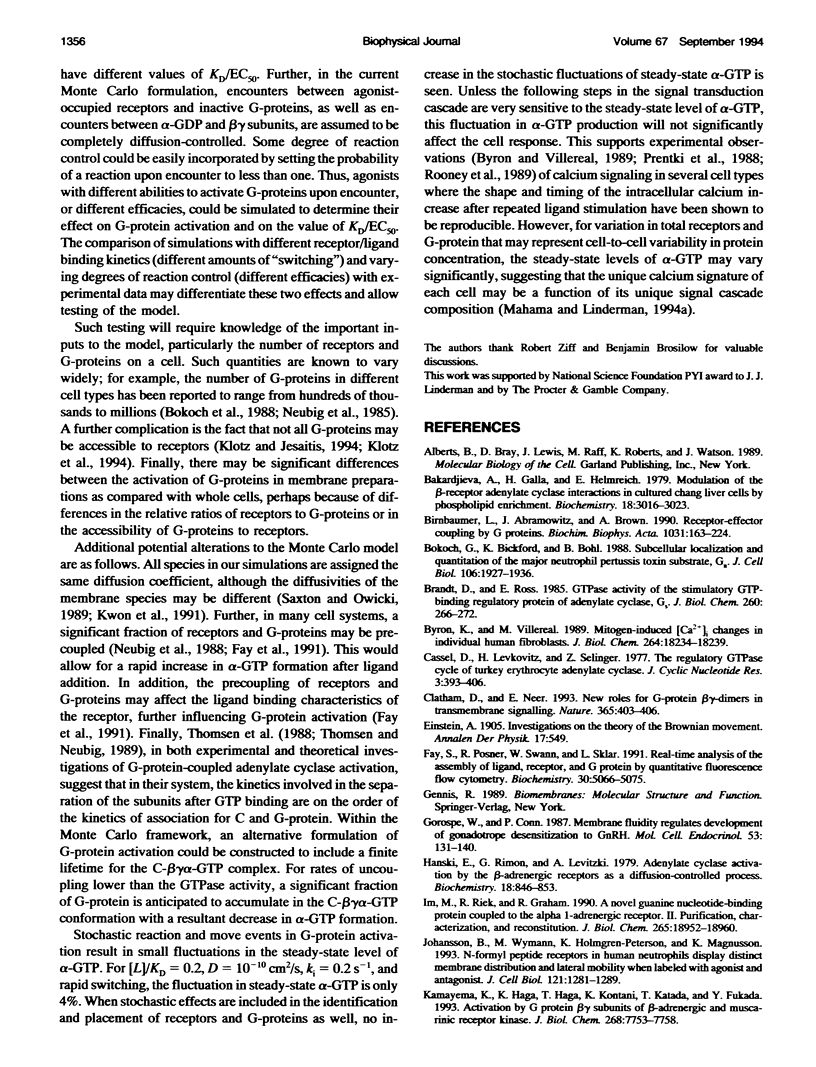
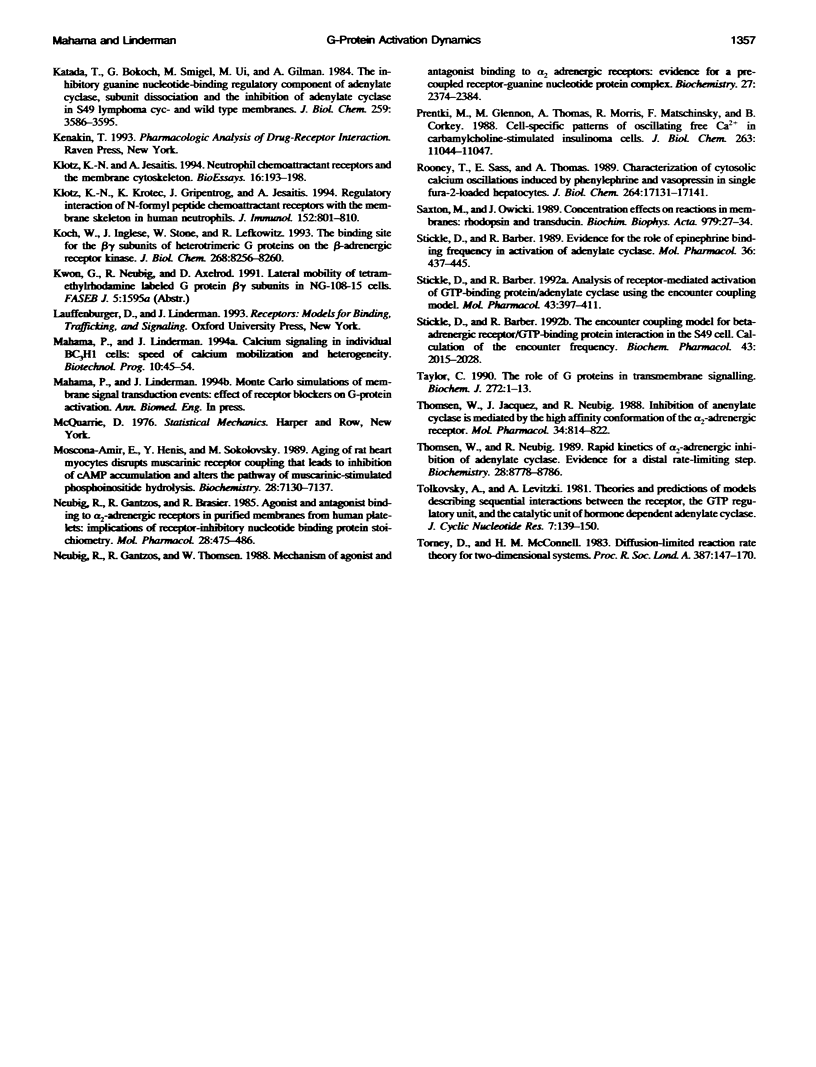
Selected References
These references are in PubMed. This may not be the complete list of references from this article.
- Bakardjieva A., Galla H. J., Helmreich E. J. Modulation of the beta-receptor adenylate cyclase interactions in cultured Chang liver cells by phospholipid enrichment. Biochemistry. 1979 Jul 10;18(14):3016–3023. doi: 10.1021/bi00581a017. [DOI] [PubMed] [Google Scholar]
- Birnbaumer L., Abramowitz J., Brown A. M. Receptor-effector coupling by G proteins. Biochim Biophys Acta. 1990 May 7;1031(2):163–224. doi: 10.1016/0304-4157(90)90007-y. [DOI] [PubMed] [Google Scholar]
- Bokoch G. M., Bickford K., Bohl B. P. Subcellular localization and quantitation of the major neutrophil pertussis toxin substrate, Gn. J Cell Biol. 1988 Jun;106(6):1927–1936. doi: 10.1083/jcb.106.6.1927. [DOI] [PMC free article] [PubMed] [Google Scholar]
- Brandt D. R., Ross E. M. GTPase activity of the stimulatory GTP-binding regulatory protein of adenylate cyclase, Gs. Accumulation and turnover of enzyme-nucleotide intermediates. J Biol Chem. 1985 Jan 10;260(1):266–272. [PubMed] [Google Scholar]
- Byron K. L., Villereal M. L. Mitogen-induced [Ca2+]i changes in individual human fibroblasts. Image analysis reveals asynchronous responses which are characteristic for different mitogens. J Biol Chem. 1989 Oct 25;264(30):18234–18239. [PubMed] [Google Scholar]
- Cassel D., Levkovitz H., Selinger Z. The regulatory GTPase cycle of turkey erythrocyte adenylate cyclase. J Cyclic Nucleotide Res. 1977 Dec;3(6):393–406. [PubMed] [Google Scholar]
- Clapham D. E., Neer E. J. New roles for G-protein beta gamma-dimers in transmembrane signalling. Nature. 1993 Sep 30;365(6445):403–406. doi: 10.1038/365403a0. [DOI] [PubMed] [Google Scholar]
- Fay S. P., Posner R. G., Swann W. N., Sklar L. A. Real-time analysis of the assembly of ligand, receptor, and G protein by quantitative fluorescence flow cytometry. Biochemistry. 1991 May 21;30(20):5066–5075. doi: 10.1021/bi00234a033. [DOI] [PubMed] [Google Scholar]
- Gorospe W. C., Conn P. M. Membrane fluidity regulates development of gonadotrope desensitization to GnRH. Mol Cell Endocrinol. 1987 Sep;53(1-2):131–140. doi: 10.1016/0303-7207(87)90199-7. [DOI] [PubMed] [Google Scholar]
- Hanski E., Rimon G., Levitzki A. Adenylate cyclase activation by the beta-adrenergic receptors as a diffusion-controlled process. Biochemistry. 1979 Mar 6;18(5):846–853. doi: 10.1021/bi00572a017. [DOI] [PubMed] [Google Scholar]
- Im M. J., Riek R. P., Graham R. M. A novel guanine nucleotide-binding protein coupled to the alpha 1-adrenergic receptor. II. Purification, characterization, and reconstitution. J Biol Chem. 1990 Nov 5;265(31):18952–18960. [PubMed] [Google Scholar]
- Johansson B., Wymann M. P., Holmgren-Peterson K., Magnusson K. E. N-formyl peptide receptors in human neutrophils display distinct membrane distribution and lateral mobility when labeled with agonist and antagonist. J Cell Biol. 1993 Jun;121(6):1281–1289. doi: 10.1083/jcb.121.6.1281. [DOI] [PMC free article] [PubMed] [Google Scholar]
- Kameyama K., Haga K., Haga T., Kontani K., Katada T., Fukada Y. Activation by G protein beta gamma subunits of beta-adrenergic and muscarinic receptor kinase. J Biol Chem. 1993 Apr 15;268(11):7753–7758. [PubMed] [Google Scholar]
- Katada T., Bokoch G. M., Smigel M. D., Ui M., Gilman A. G. The inhibitory guanine nucleotide-binding regulatory component of adenylate cyclase. Subunit dissociation and the inhibition of adenylate cyclase in S49 lymphoma cyc- and wild type membranes. J Biol Chem. 1984 Mar 25;259(6):3586–3595. [PubMed] [Google Scholar]
- Klotz K. N., Jesaitis A. J. Neutrophil chemoattractant receptors and the membrane skeleton. Bioessays. 1994 Mar;16(3):193–198. doi: 10.1002/bies.950160310. [DOI] [PubMed] [Google Scholar]
- Klotz K. N., Krotec K. L., Gripentrog J., Jesaitis A. J. Regulatory interaction of N-formyl peptide chemoattractant receptors with the membrane skeleton in human neutrophils. J Immunol. 1994 Jan 15;152(2):801–810. [PubMed] [Google Scholar]
- Koch W. J., Inglese J., Stone W. C., Lefkowitz R. J. The binding site for the beta gamma subunits of heterotrimeric G proteins on the beta-adrenergic receptor kinase. J Biol Chem. 1993 Apr 15;268(11):8256–8260. [PubMed] [Google Scholar]
- Moscona-Amir E., Henis Y. I., Sokolovsky M. Aging of rat heart myocytes disrupts muscarinic receptor coupling that leads to inhibition of cAMP accumulation and alters the pathway of muscarinic-stimulated phosphoinositide hydrolysis. Biochemistry. 1989 Aug 22;28(17):7130–7137. doi: 10.1021/bi00443a052. [DOI] [PubMed] [Google Scholar]
- Neubig R. R., Gantzos R. D., Brasier R. S. Agonist and antagonist binding to alpha 2-adrenergic receptors in purified membranes from human platelets. Implications of receptor-inhibitory nucleotide-binding protein stoichiometry. Mol Pharmacol. 1985 Nov;28(5):475–486. [PubMed] [Google Scholar]
- Neubig R. R., Gantzos R. D., Thomsen W. J. Mechanism of agonist and antagonist binding to alpha 2 adrenergic receptors: evidence for a precoupled receptor-guanine nucleotide protein complex. Biochemistry. 1988 Apr 5;27(7):2374–2384. doi: 10.1021/bi00407a019. [DOI] [PubMed] [Google Scholar]
- Prentki M., Glennon M. C., Thomas A. P., Morris R. L., Matschinsky F. M., Corkey B. E. Cell-specific patterns of oscillating free Ca2+ in carbamylcholine-stimulated insulinoma cells. J Biol Chem. 1988 Aug 15;263(23):11044–11047. [PubMed] [Google Scholar]
- Rooney T. A., Sass E. J., Thomas A. P. Characterization of cytosolic calcium oscillations induced by phenylephrine and vasopressin in single fura-2-loaded hepatocytes. J Biol Chem. 1989 Oct 15;264(29):17131–17141. [PubMed] [Google Scholar]
- Saxton M. J., Owicki J. C. Concentration effects on reactions in membranes: rhodopsin and transducin. Biochim Biophys Acta. 1989 Feb 13;979(1):27–34. doi: 10.1016/0005-2736(89)90519-1. [DOI] [PubMed] [Google Scholar]
- Stickle D., Barber R. Evidence for the role of epinephrine binding frequency in activation of adenylate cyclase. Mol Pharmacol. 1989 Sep;36(3):437–445. [PubMed] [Google Scholar]
- Stickle D., Barber R. The encounter coupling model for beta-adrenergic receptor/GTP-binding protein interaction in the S49 cell. Calculation of the encounter frequency. Biochem Pharmacol. 1992 May 8;43(9):2015–2028. doi: 10.1016/0006-2952(92)90645-y. [DOI] [PubMed] [Google Scholar]
- Taylor C. W. The role of G proteins in transmembrane signalling. Biochem J. 1990 Nov 15;272(1):1–13. doi: 10.1042/bj2720001. [DOI] [PMC free article] [PubMed] [Google Scholar]
- Thomsen W. J., Jacquez J. A., Neubig R. R. Inhibition of adenylate cyclase is mediated by the high affinity conformation of the alpha 2-adrenergic receptor. Mol Pharmacol. 1988 Dec;34(6):814–822. [PubMed] [Google Scholar]
- Thomsen W. J., Neubig R. R. Rapid kinetics of alpha 2-adrenergic inhibition of adenylate cyclase. Evidence for a distal rate-limiting step. Biochemistry. 1989 Oct 31;28(22):8778–8786. doi: 10.1021/bi00448a015. [DOI] [PubMed] [Google Scholar]
- Tolkovsky A. M., Levitzki A. Theories and predictions of models describing sequential interactions between the receptor, the GTP regulatory unit, and the catalytic unit of hormone dependent adenylate cyclases. J Cyclic Nucleotide Res. 1981;7(3):139–150. [PubMed] [Google Scholar]