Abstract
The exquisite sensitivity of elasmobranch fishes to electric fields is thought to reside in electroreceptive organs called ampullae of Lorenzini. We measured the stimulus-response behavior of ampullary organs excised from skates. Under open-circuit conditions, the ampullary organ showed three distinct response states: spontaneous repetitive spikes, evoked spikes, and small, damped oscillatory responses. Under short-circuit conditions, the amplitude range for a linear current response to a sinusoidal (0.5 Hz) voltage clamp of an organ (assessed by spectral analysis of the harmonics generated) was 7-200 microV rms. Changes in the spike firing rate of the afferent nerve innervating the organ were evident for voltage clamps of the ampullary epithelium of 3 microV and the spike rate saturated for clamp steps exceeding 100 microV. Thus, the linear response range of the ampullary epithelium exceeded the range in spike firing rate of the afferent nerve. The steady-state transorgan electrical properties under voltage clamp conditions were obtained by analysis of complex admittance determinations in the frequency range 0.05-20 Hz for perturbations (< 100 microV rms) in the linear range. Admittance functions were distinctly related to the preparation states observed under open-circuit conditions. A negative real part in the organ admittance (i.e., a steady-state negative conductance generated by the preparation) was a common characteristic of the two (open-circuit) excitable states. The negative conductance was also confirmed by the direction of current flow through the ampullary epithelium in response to step voltage clamps. We conclude that the steady state-negative conductance is an essential property of the ampullary epithelium,and we suggest that the interplay of negative and positive conductances generated by ion channels in apical and basal membranes of receptor cells results in signal amplification that may contribute significantly to the electric field sensitivity of ampullary organs.
Full text
PDF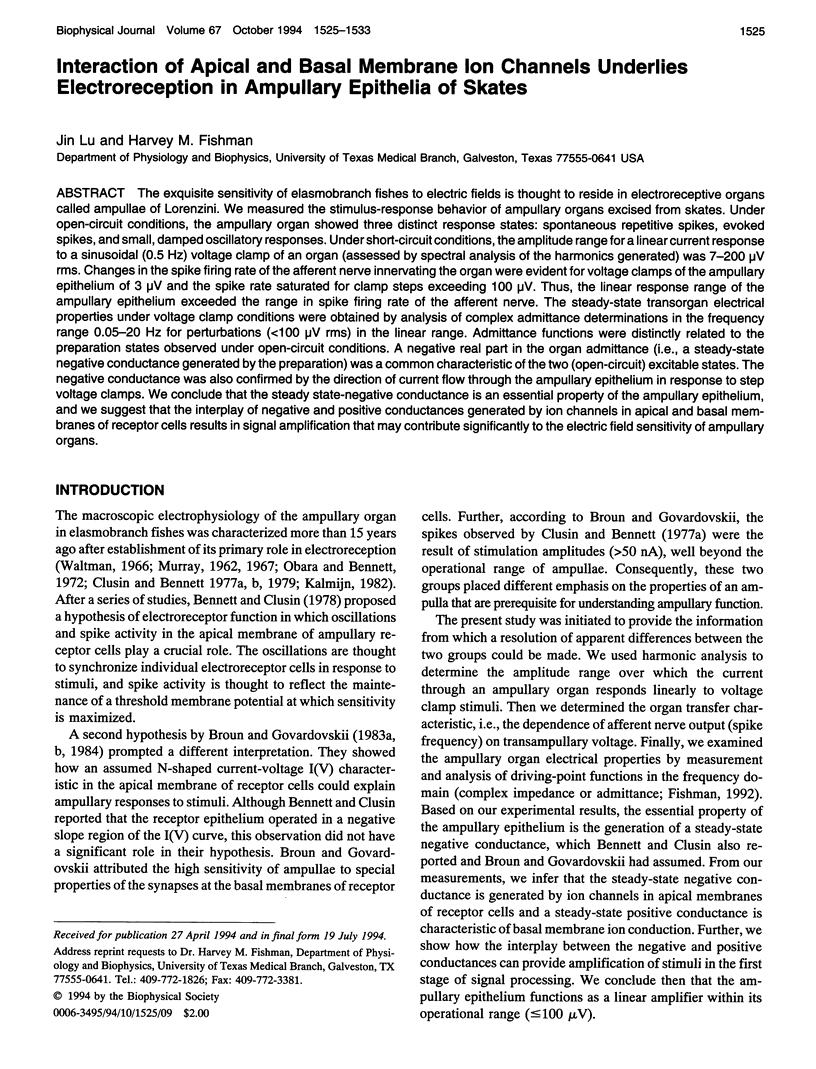
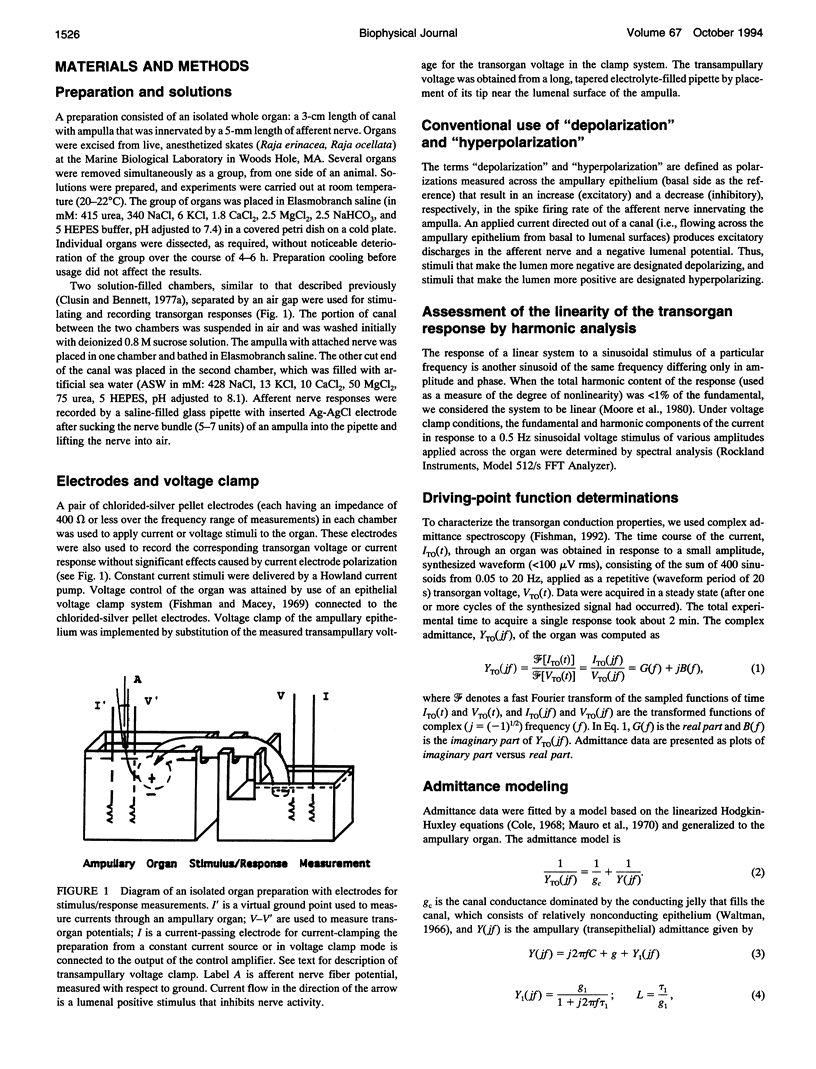
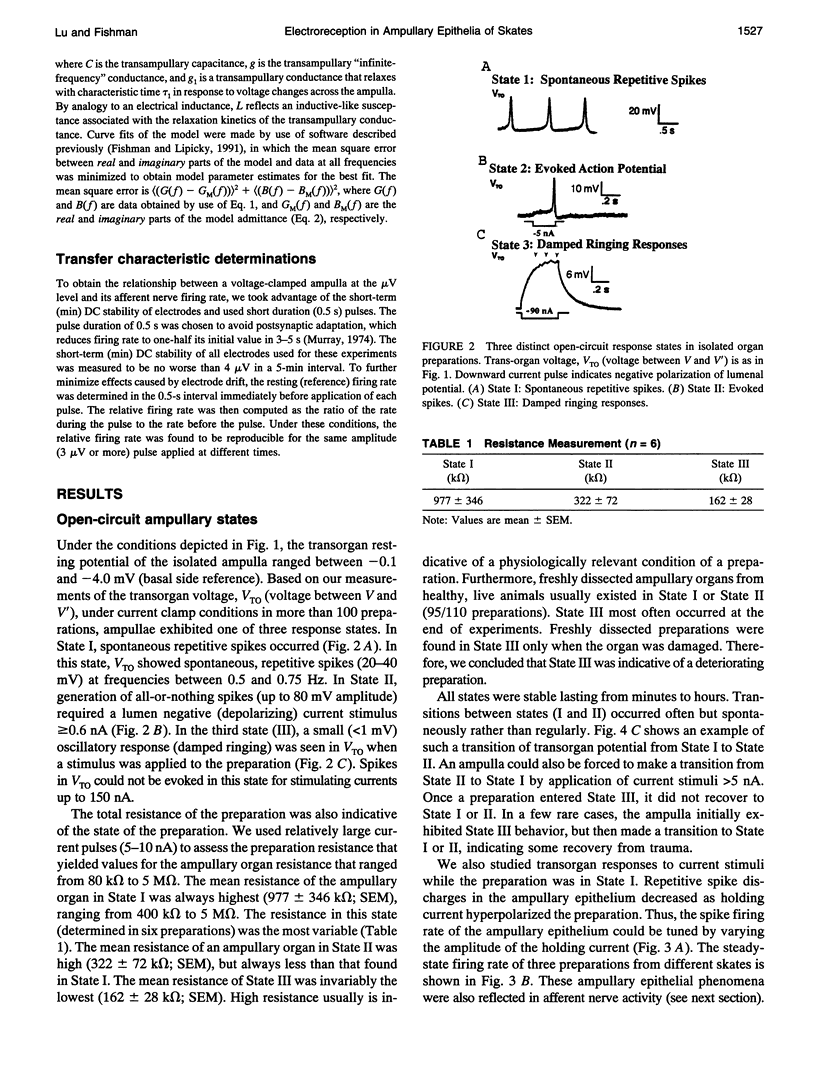
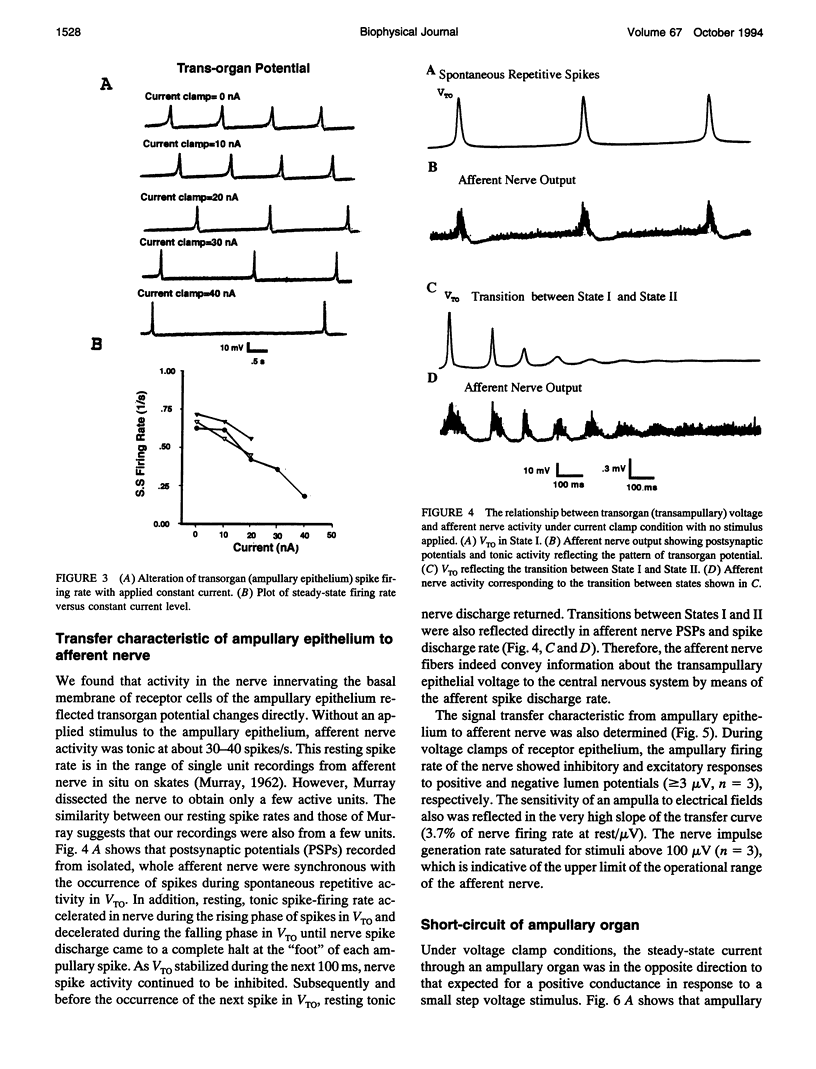
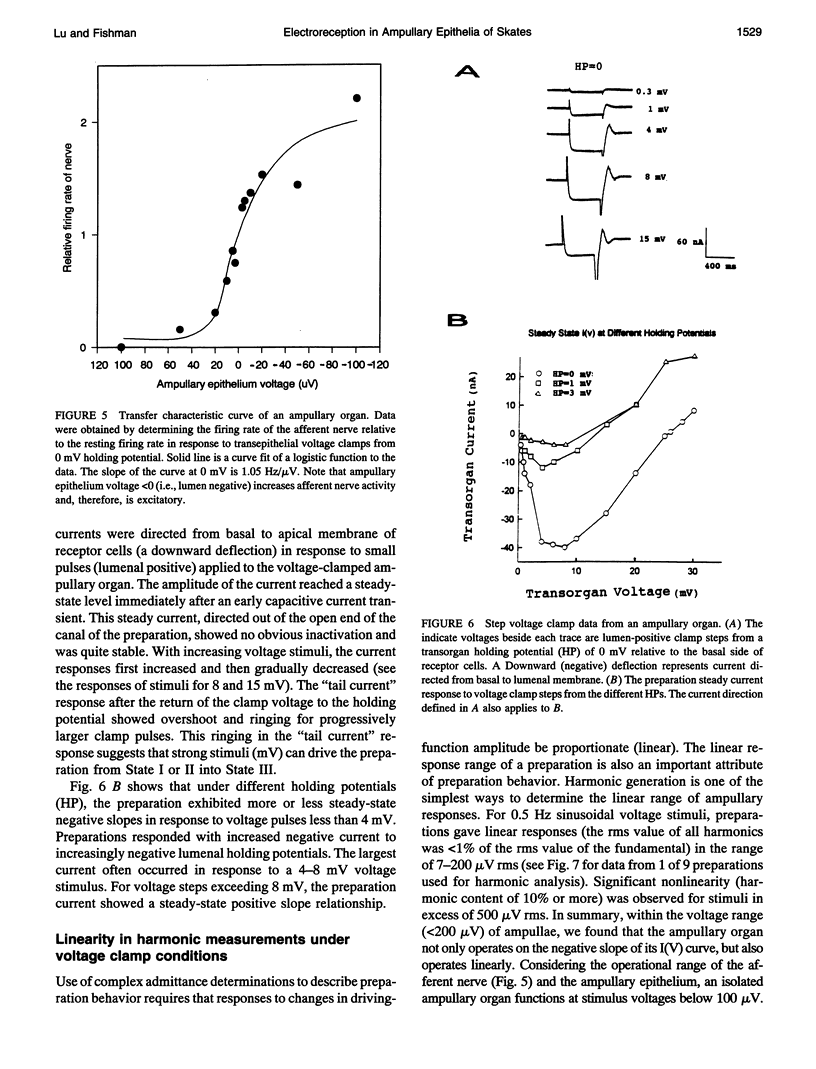
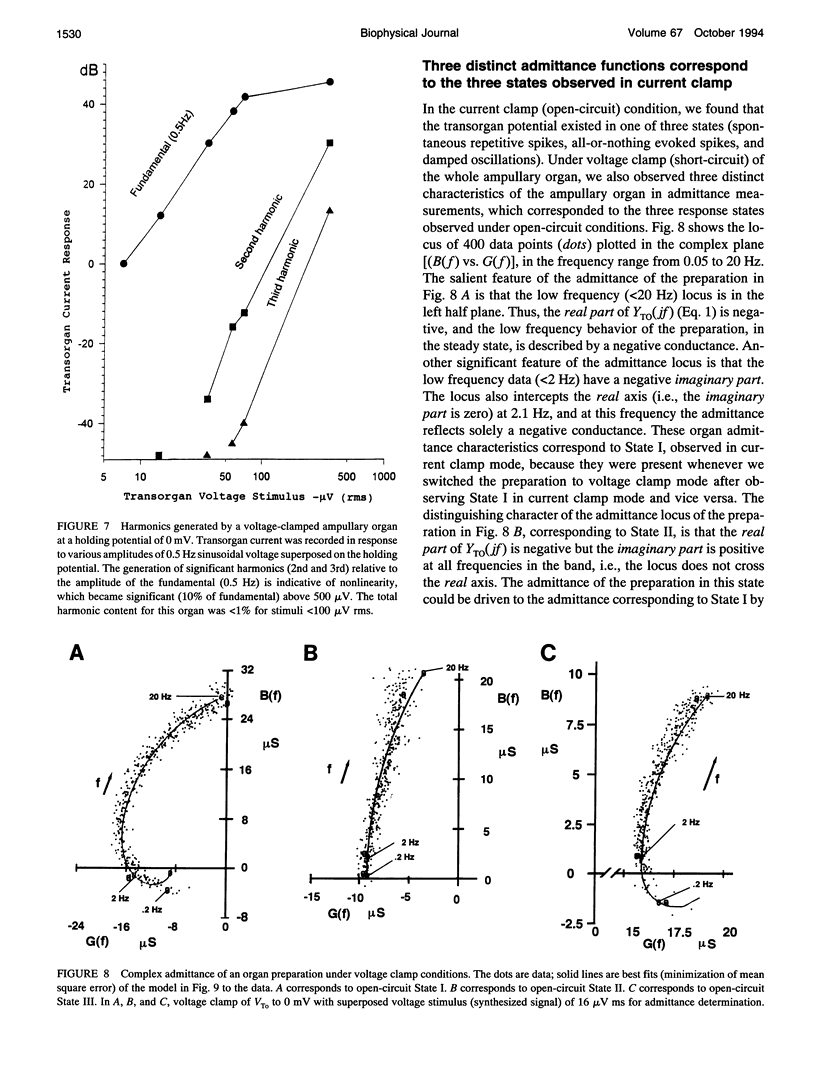
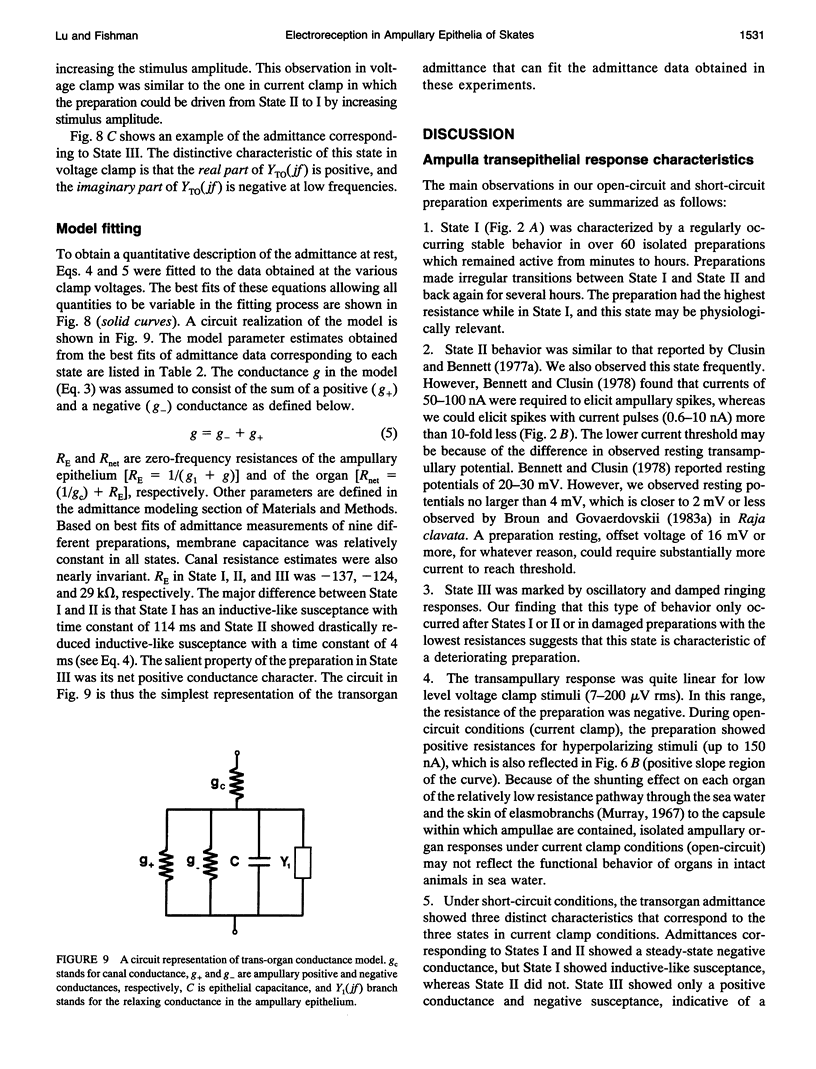
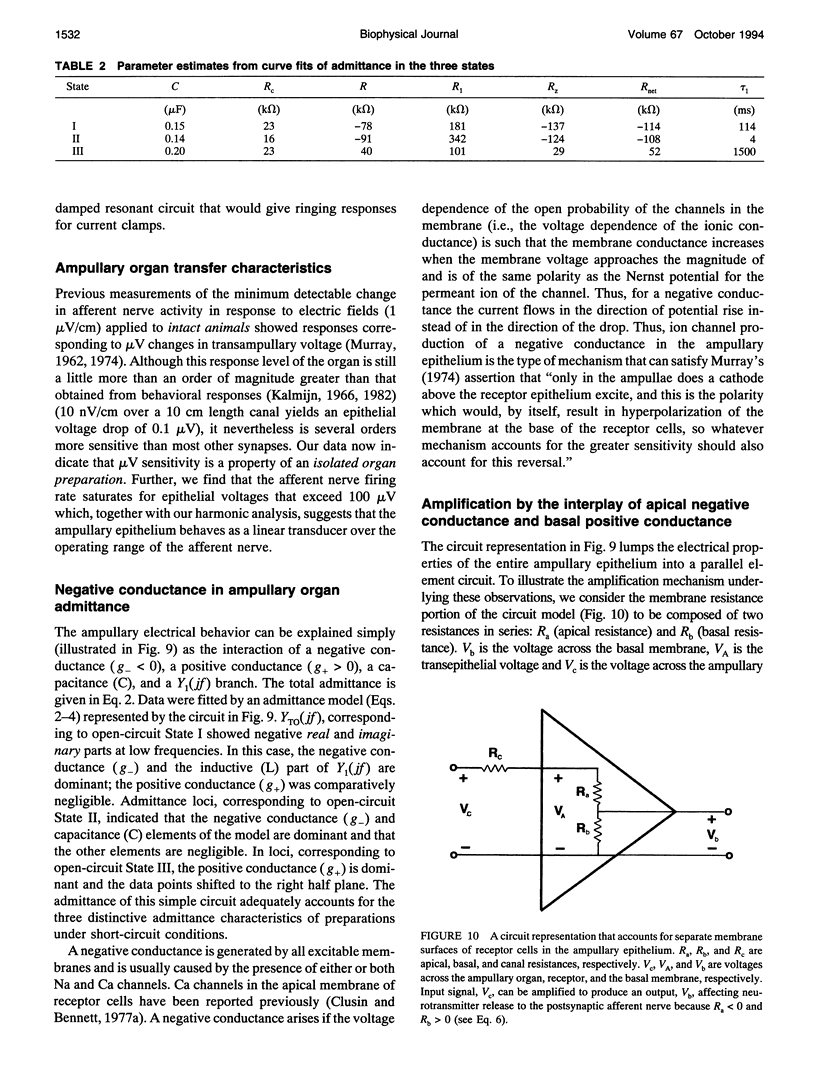
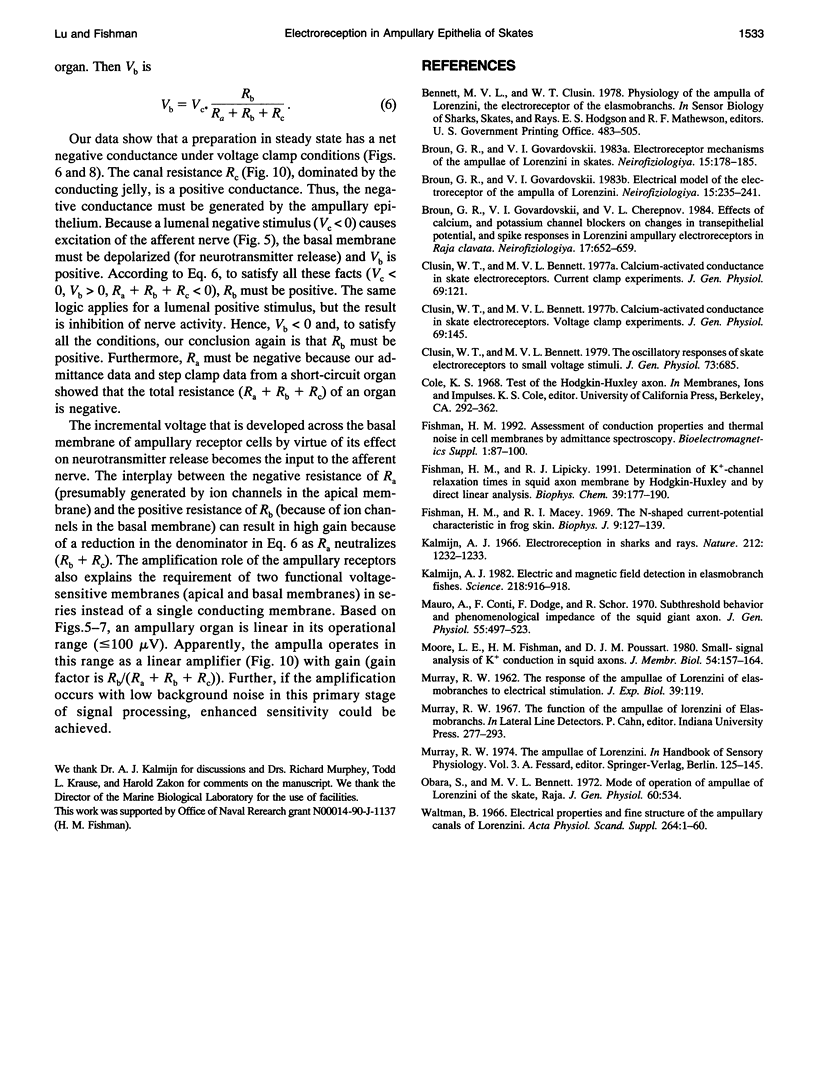
Selected References
These references are in PubMed. This may not be the complete list of references from this article.
- Broun G. R., Govardovskii V. I., Cherepnov V. L. Vliianie blokatorov kalievykh o kal'tsievykh kanalov na izmeneniia transépitelial'nogo potentsiala i impul'snye otvety élektroretseptorov ampul Lorentsini skata. Neirofiziologiia. 1985;17(5):652–660. [PubMed] [Google Scholar]
- Broun G. R., Govardovskii V. I. Elektricheskaia model' élektroretseptora ampuly Lorentsini. Neirofiziologiia. 1983;15(3):235–241. [PubMed] [Google Scholar]
- Broun G. R., Govardovskii V. I. Issledovanie retseptornykh mekhanizmov élektroretseptorov ampul Lorentsini skata. Neirofiziologiia. 1983;15(2):178–185. [PubMed] [Google Scholar]
- Clusin W. T., Bennett M. V. Calcium-activated conductance in skate electroreceptors: current clamp experiments. J Gen Physiol. 1977 Feb;69(2):121–143. doi: 10.1085/jgp.69.2.121. [DOI] [PMC free article] [PubMed] [Google Scholar]
- Clusin W. T., Bennett M. V. Calcium-activated conductance in skate electroreceptors: voltage clamp experiments. J Gen Physiol. 1977 Feb;69(2):145–182. doi: 10.1085/jgp.69.2.145. [DOI] [PMC free article] [PubMed] [Google Scholar]
- Clusin W. T., Bennett M. V. The oscillatory responses of skate electroreceptors to small voltage stimuli. J Gen Physiol. 1979 Jun;73(6):685–702. doi: 10.1085/jgp.73.6.685. [DOI] [PMC free article] [PubMed] [Google Scholar]
- Fishman H. M. Assessment of conduction properties and thermal noise in cell membranes by admittance spectroscopy. Bioelectromagnetics. 1992;Suppl 1:87–100. doi: 10.1002/bem.2250130709. [DOI] [PubMed] [Google Scholar]
- Fishman H. M., Lipicky R. J. Determination of K(+)-channel relaxation times in squid axon membrane by Hodgkin-Huxley and by direct linear analysis. Biophys Chem. 1991 Feb;39(2):177–190. doi: 10.1016/0301-4622(91)85020-q. [DOI] [PubMed] [Google Scholar]
- Fishman H. M., Macey R. I. The N-shaped current-potential characteristic in frog skin. I. Time development during step voltage clamp. Biophys J. 1969 Feb;9(2):127–139. doi: 10.1016/S0006-3495(69)86374-5. [DOI] [PMC free article] [PubMed] [Google Scholar]
- Kalmijn A. J. Electric and magnetic field detection in elasmobranch fishes. Science. 1982 Nov 26;218(4575):916–918. doi: 10.1126/science.7134985. [DOI] [PubMed] [Google Scholar]
- MURRAY R. W. The response of the ampullae of Lorenzini of elasmobranchs to electrical stimulation. J Exp Biol. 1962 Mar;39:119–128. doi: 10.1242/jeb.39.1.119. [DOI] [PubMed] [Google Scholar]
- Mauro A., Conti F., Dodge F., Schor R. Subthreshold behavior and phenomenological impedance of the squid giant axon. J Gen Physiol. 1970 Apr;55(4):497–523. doi: 10.1085/jgp.55.4.497. [DOI] [PMC free article] [PubMed] [Google Scholar]
- Moore L. E., Fishman H. M., Poussart D. J. Small-signal analysis of K+ conduction in squid axons. J Membr Biol. 1980 May 23;54(2):157–164. doi: 10.1007/BF01940569. [DOI] [PubMed] [Google Scholar]
- Obara S., Bennett M. V. Mode of operation of ampullae of Lorenzini of the skate, Raja. J Gen Physiol. 1972 Nov;60(5):534–557. doi: 10.1085/jgp.60.5.534. [DOI] [PMC free article] [PubMed] [Google Scholar]
- Waltman B. Electrical properties and fine structure of the ampullary canals of Lorenzini. Acta Physiol Scand Suppl. 1966;264:1–60. [PubMed] [Google Scholar]