Abstract
Although the central role of the signal sequence in protein export is well established, the molecular details underlying signal sequence in vivo function remain unclear. As part of our continuing effort to relate signal sequence phenotypes to specific biophysical properties, we have carried out an extensive characterization of the secondary structure and lipid interactions for a family of peptides corresponding to the wild-type E. coli LamB signal sequence, and mutants that harbor charged residue point mutations in the hydrophobic core region. We used membrane-resident fluorescence quenching according to the parallax method to determine the relative depth of insertion of tryptophan-labeled analogs of these peptides into the acyl chain region of bilayer vesicles composed of 1-palmitoyl-2-oleoyl-sn-glycero-3-phosphoethanolamine and 1-palmitoyl-2-oleoyl-sn-glycero-3-phosphoglycerol. Also, restriction of acyl chain motion upon peptide binding was evaluated using steady-state fluorescence anisotropy of 1,6-diphenyl-1,3,5-hexatriene. Each of these peptides showed evidence of insertion into the acyl chain region, although most likely not in a transmembrane orientation. The mutant peptides were shown to have a reduced insertion potential relative to the wild-type peptide. Furthermore, tryptophan spectral properties indicated that insertion of the wild-type and mutant peptides enhances bilayer hydration. This effect was particularly pronounced with peptides harboring negatively charged aspartate point substitutions. The results are discussed in relation to the potential roles of signal sequences in mediating protein translocation.
Full text
PDF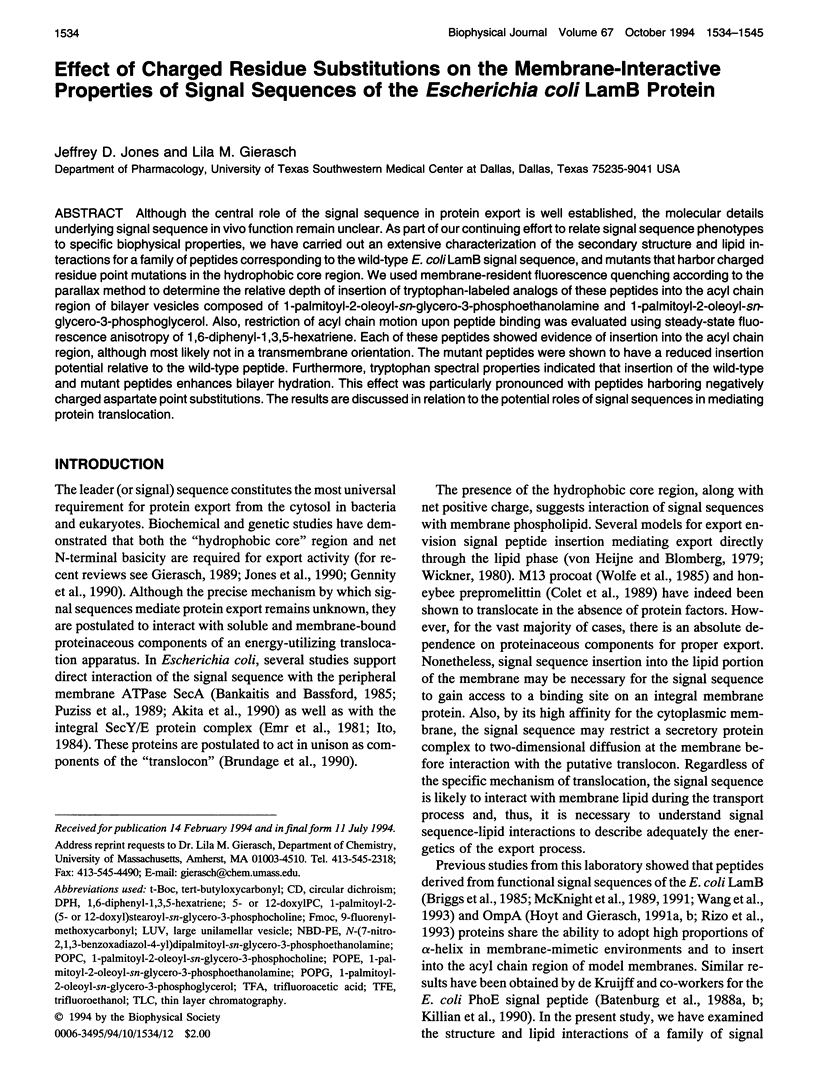
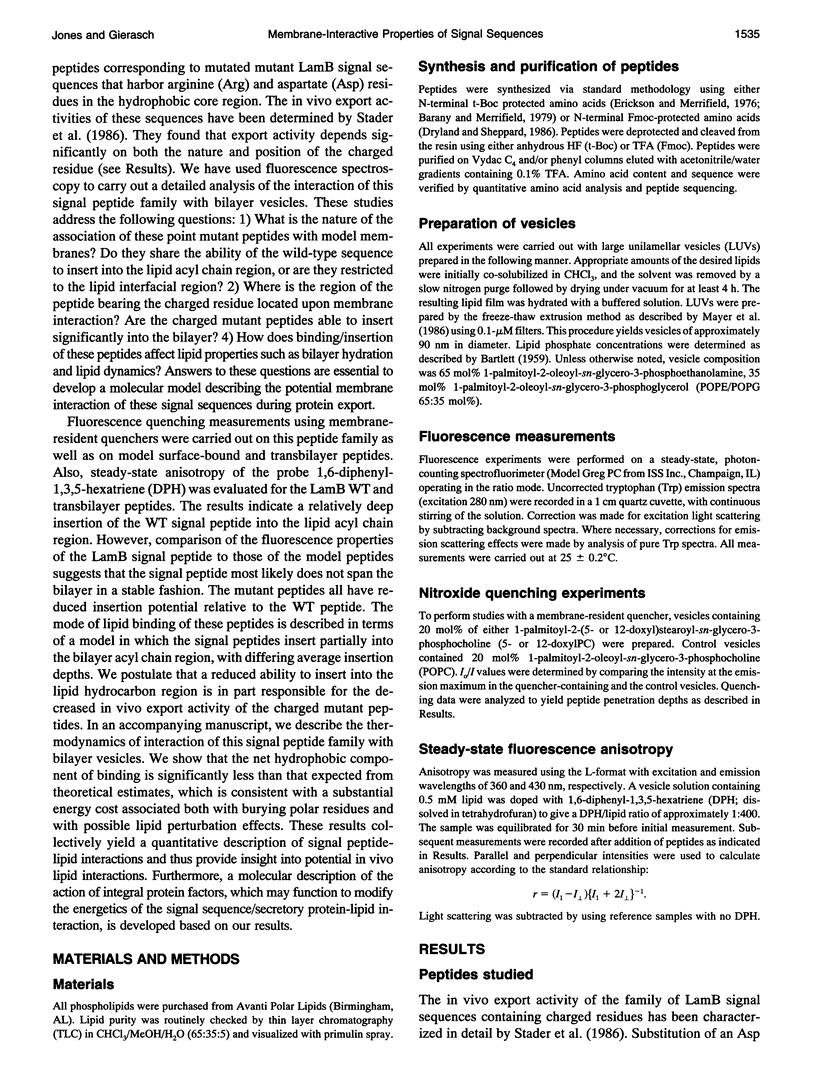
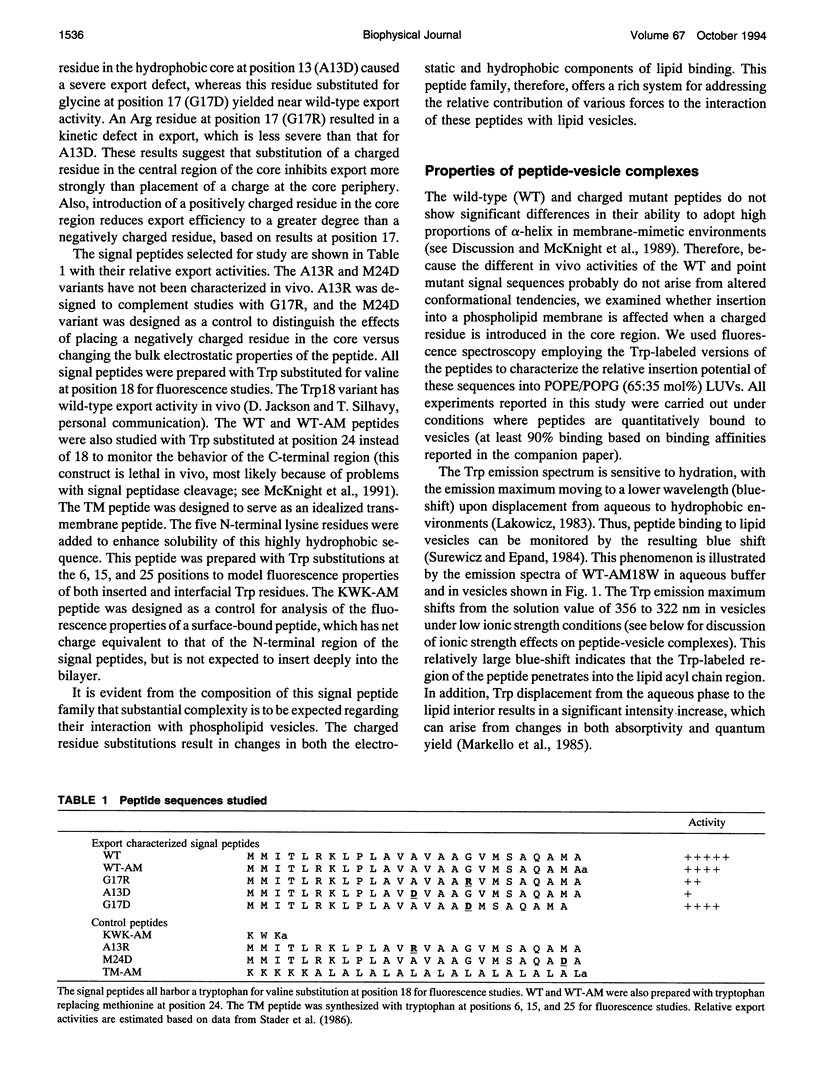
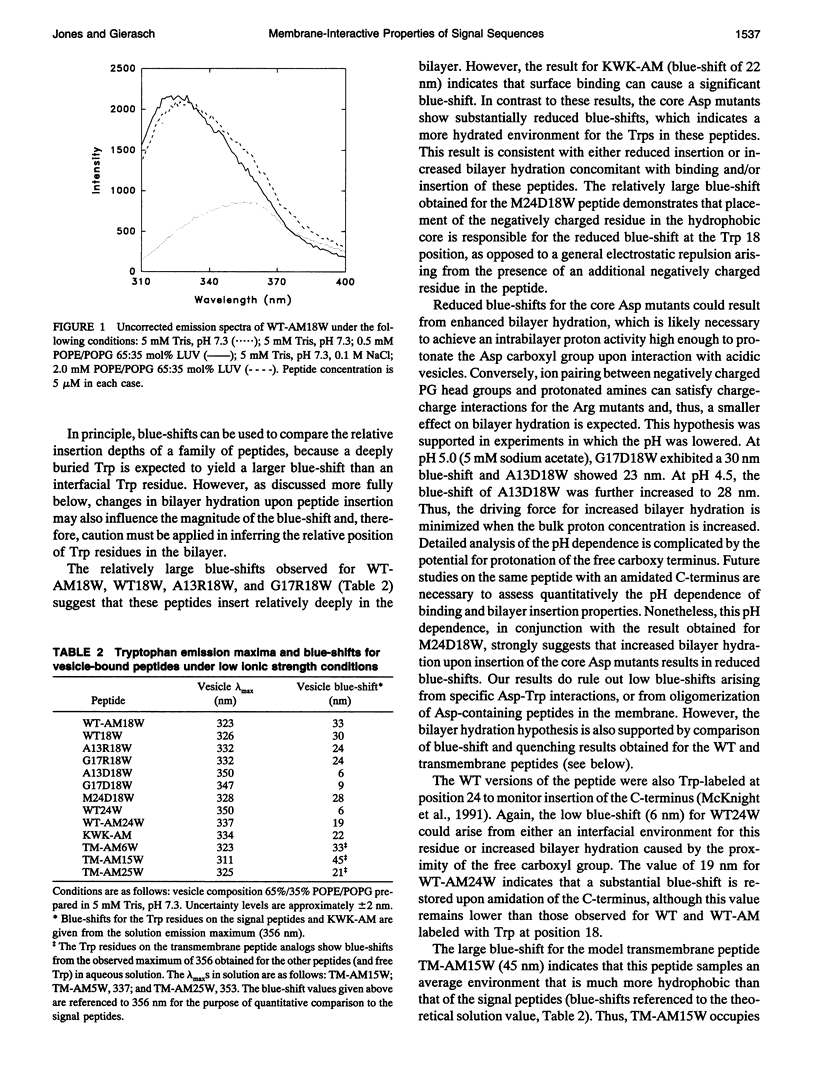
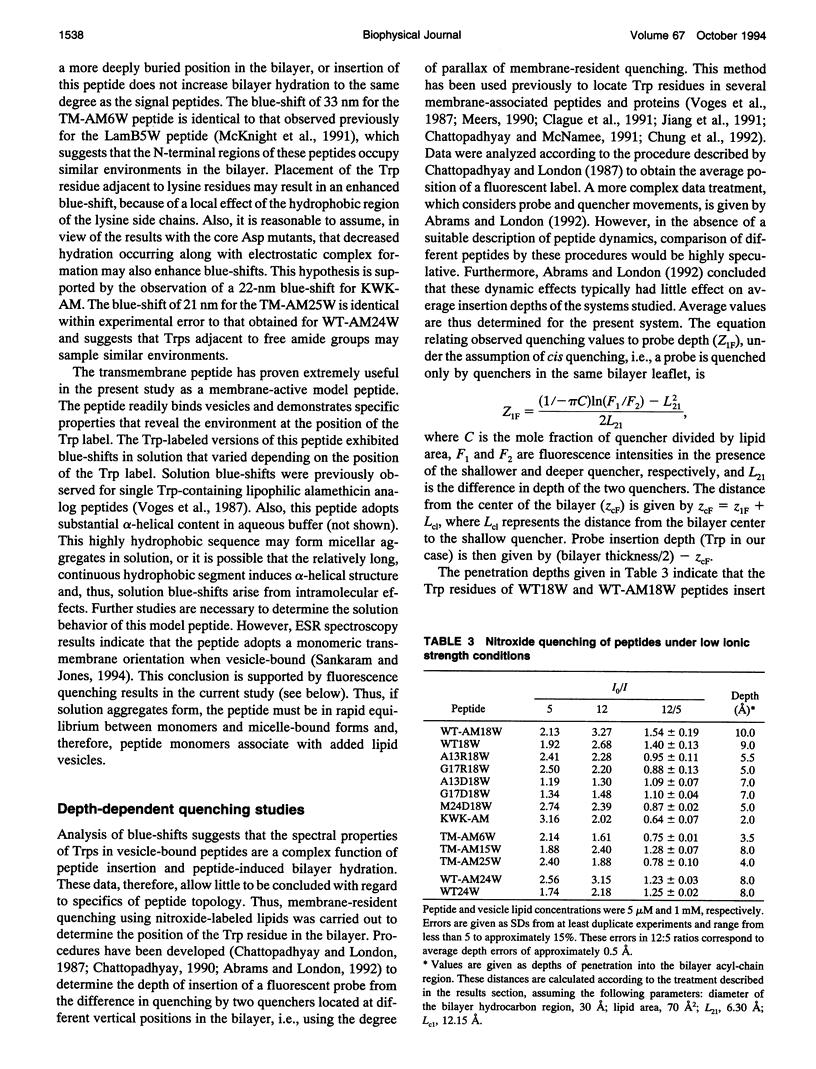
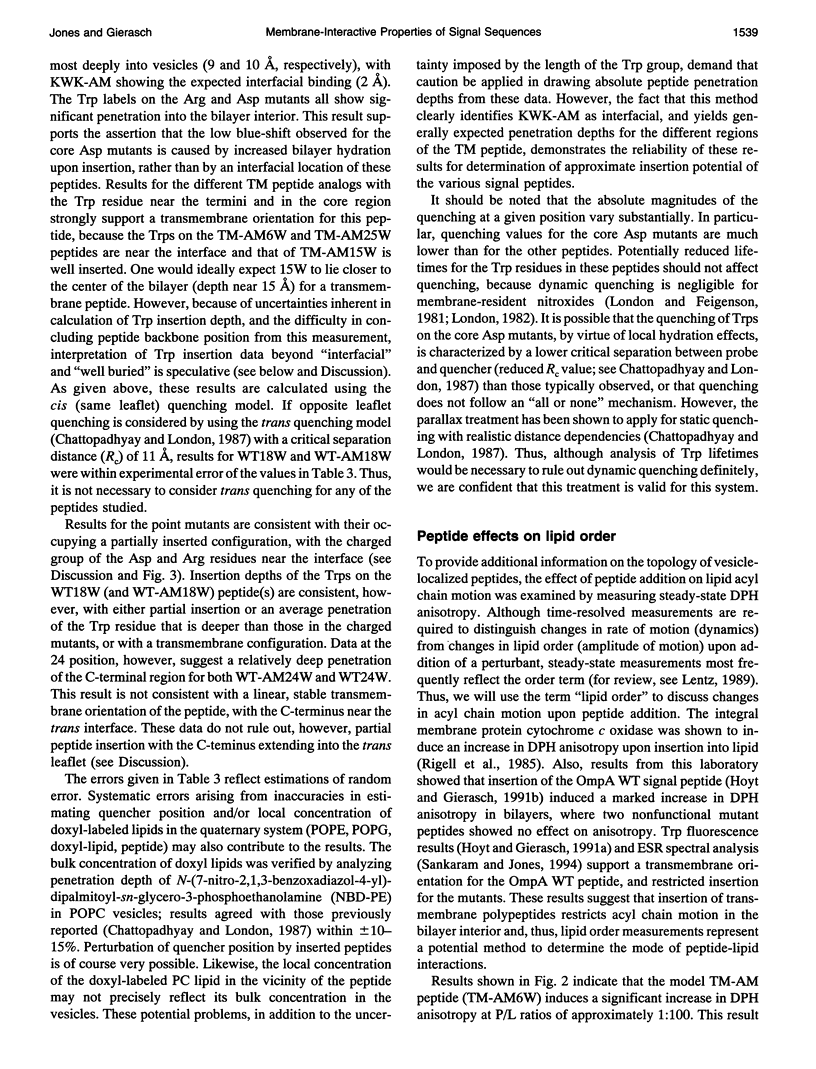
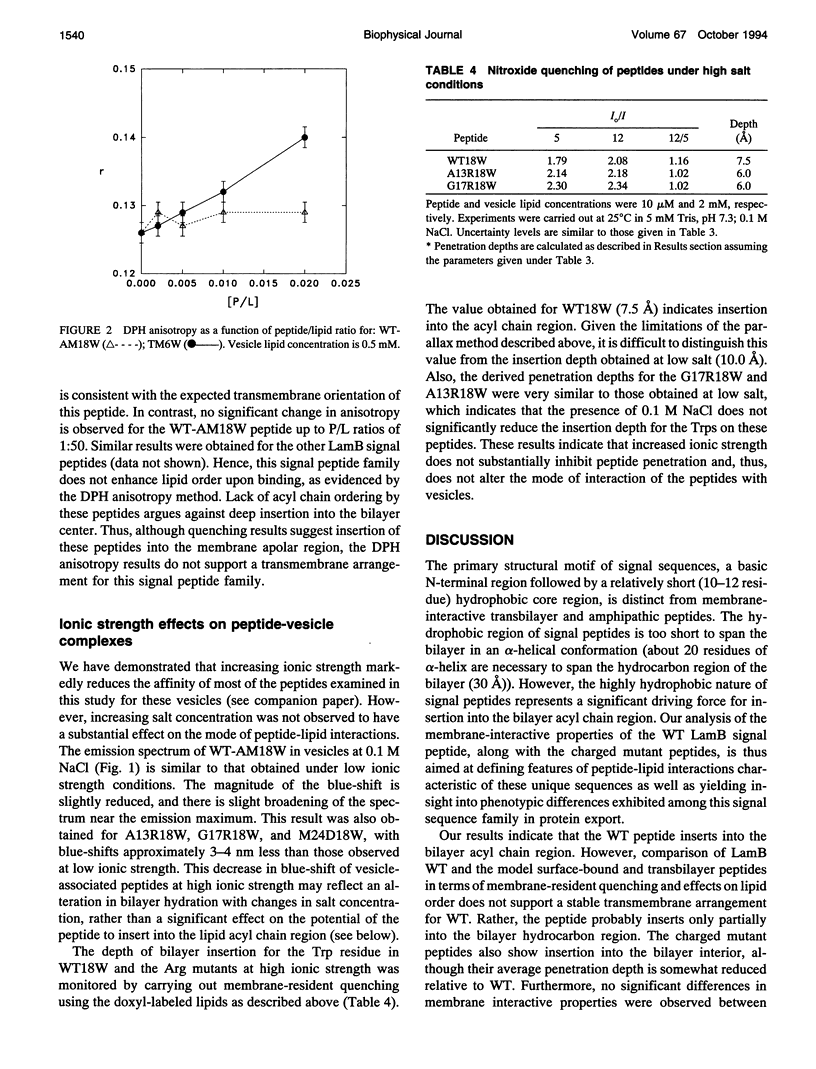
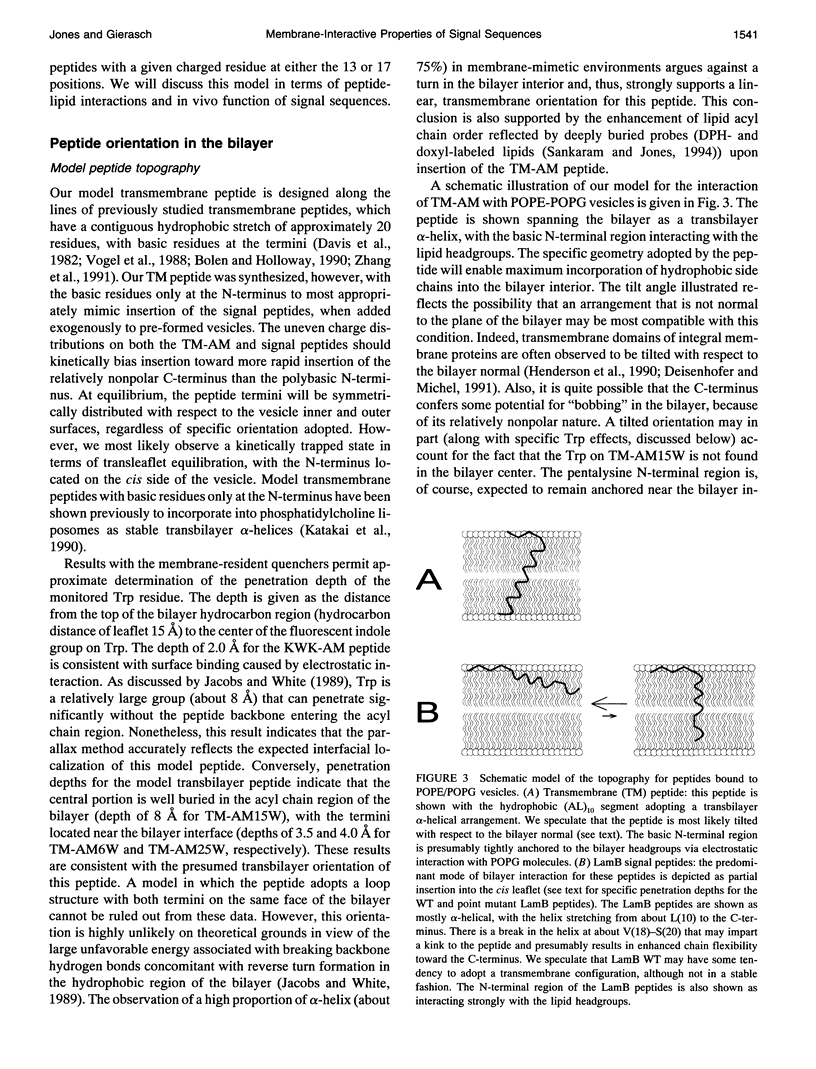
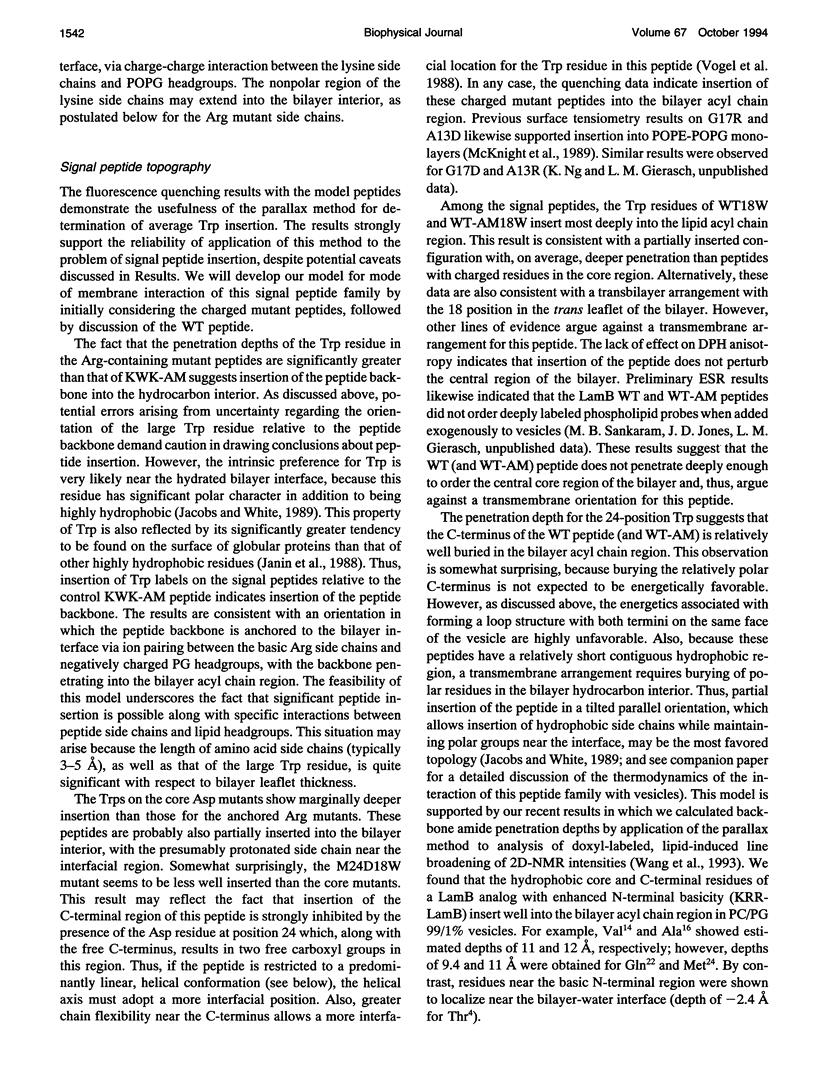
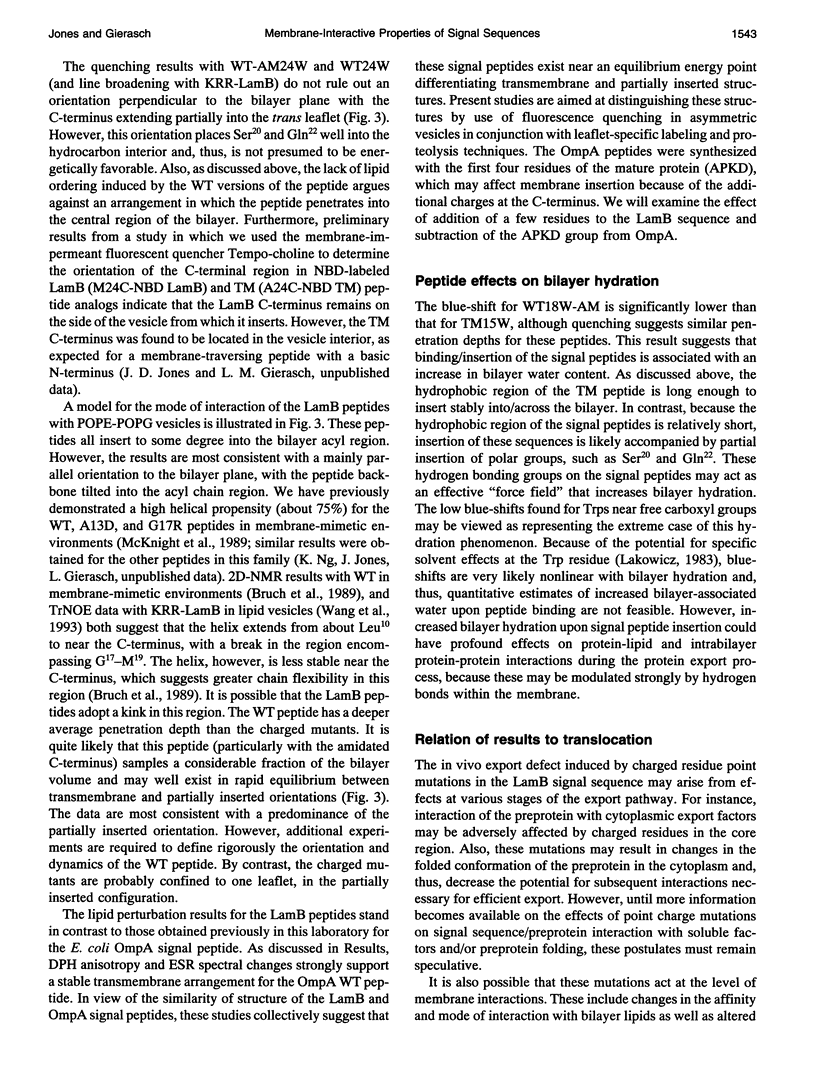
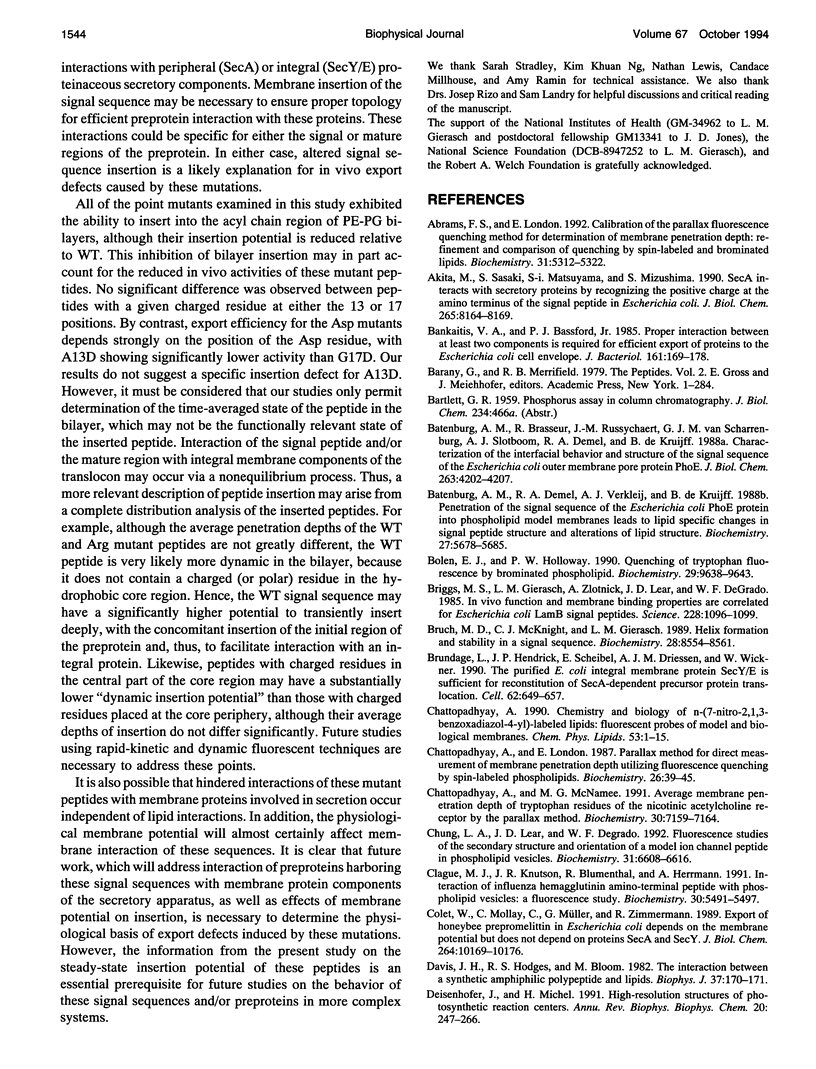
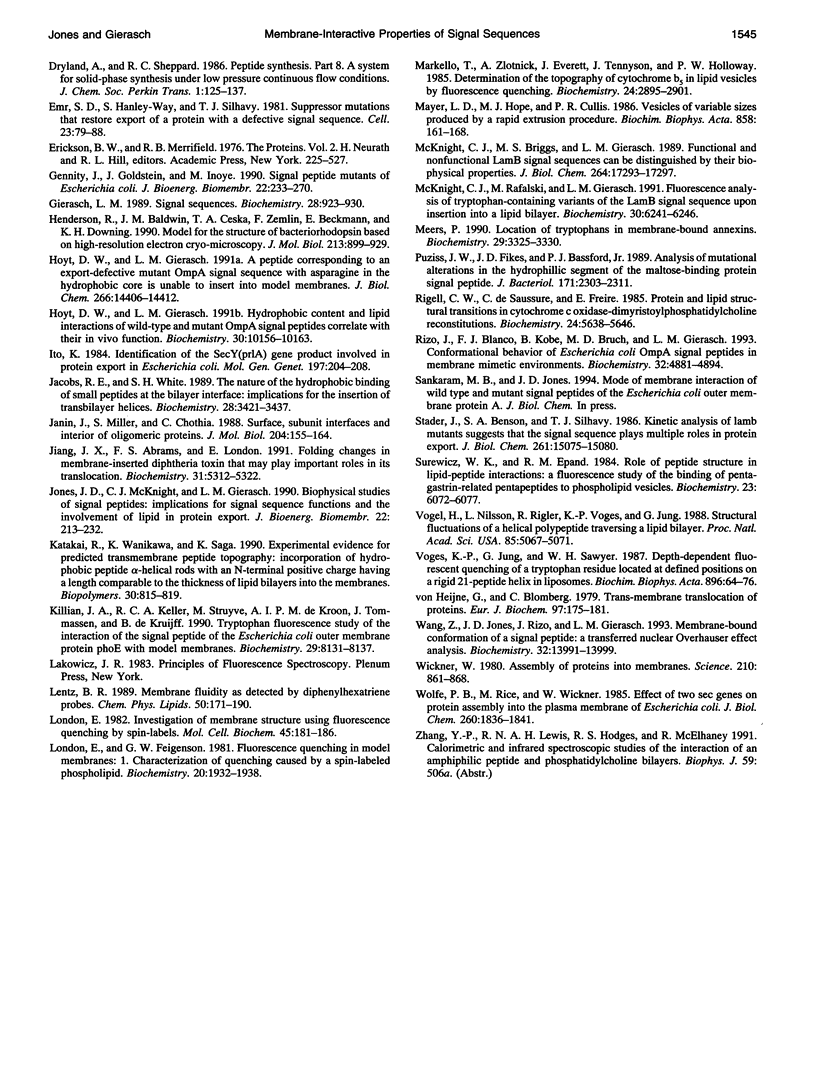
Selected References
These references are in PubMed. This may not be the complete list of references from this article.
- Abrams F. S., London E. Calibration of the parallax fluorescence quenching method for determination of membrane penetration depth: refinement and comparison of quenching by spin-labeled and brominated lipids. Biochemistry. 1992 Jun 16;31(23):5312–5322. doi: 10.1021/bi00138a010. [DOI] [PubMed] [Google Scholar]
- Akita M., Sasaki S., Matsuyama S., Mizushima S. SecA interacts with secretory proteins by recognizing the positive charge at the amino terminus of the signal peptide in Escherichia coli. J Biol Chem. 1990 May 15;265(14):8164–8169. [PubMed] [Google Scholar]
- Bankaitis V. A., Bassford P. J., Jr Proper interaction between at least two components is required for efficient export of proteins to the Escherichia coli cell envelope. J Bacteriol. 1985 Jan;161(1):169–178. doi: 10.1128/jb.161.1.169-178.1985. [DOI] [PMC free article] [PubMed] [Google Scholar]
- Batenburg A. M., Brasseur R., Ruysschaert J. M., van Scharrenburg G. J., Slotboom A. J., Demel R. A., de Kruijff B. Characterization of the interfacial behavior and structure of the signal sequence of Escherichia coli outer membrane pore protein PhoE. J Biol Chem. 1988 Mar 25;263(9):4202–4207. [PubMed] [Google Scholar]
- Batenburg A. M., Demel R. A., Verkleij A. J., de Kruijff B. Penetration of the signal sequence of Escherichia coli PhoE protein into phospholipid model membranes leads to lipid-specific changes in signal peptide structure and alterations of lipid organization. Biochemistry. 1988 Jul 26;27(15):5678–5685. doi: 10.1021/bi00415a043. [DOI] [PubMed] [Google Scholar]
- Bolen E. J., Holloway P. W. Quenching of tryptophan fluorescence by brominated phospholipid. Biochemistry. 1990 Oct 16;29(41):9638–9643. doi: 10.1021/bi00493a019. [DOI] [PubMed] [Google Scholar]
- Briggs M. S., Gierasch L. M., Zlotnick A., Lear J. D., DeGrado W. F. In vivo function and membrane binding properties are correlated for Escherichia coli lamB signal peptides. Science. 1985 May 31;228(4703):1096–1099. doi: 10.1126/science.3158076. [DOI] [PubMed] [Google Scholar]
- Bruch M. D., McKnight C. J., Gierasch L. M. Helix formation and stability in a signal sequence. Biochemistry. 1989 Oct 17;28(21):8554–8561. doi: 10.1021/bi00447a043. [DOI] [PubMed] [Google Scholar]
- Brundage L., Hendrick J. P., Schiebel E., Driessen A. J., Wickner W. The purified E. coli integral membrane protein SecY/E is sufficient for reconstitution of SecA-dependent precursor protein translocation. Cell. 1990 Aug 24;62(4):649–657. doi: 10.1016/0092-8674(90)90111-q. [DOI] [PubMed] [Google Scholar]
- Chattopadhyay A. Chemistry and biology of N-(7-nitrobenz-2-oxa-1,3-diazol-4-yl)-labeled lipids: fluorescent probes of biological and model membranes. Chem Phys Lipids. 1990 Mar;53(1):1–15. doi: 10.1016/0009-3084(90)90128-e. [DOI] [PubMed] [Google Scholar]
- Chattopadhyay A., London E. Parallax method for direct measurement of membrane penetration depth utilizing fluorescence quenching by spin-labeled phospholipids. Biochemistry. 1987 Jan 13;26(1):39–45. doi: 10.1021/bi00375a006. [DOI] [PubMed] [Google Scholar]
- Chattopadhyay A., McNamee M. G. Average membrane penetration depth of tryptophan residues of the nicotinic acetylcholine receptor by the parallax method. Biochemistry. 1991 Jul 23;30(29):7159–7164. doi: 10.1021/bi00243a017. [DOI] [PubMed] [Google Scholar]
- Chung L. A., Lear J. D., DeGrado W. F. Fluorescence studies of the secondary structure and orientation of a model ion channel peptide in phospholipid vesicles. Biochemistry. 1992 Jul 21;31(28):6608–6616. doi: 10.1021/bi00143a035. [DOI] [PubMed] [Google Scholar]
- Clague M. J., Knutson J. R., Blumenthal R., Herrmann A. Interaction of influenza hemagglutinin amino-terminal peptide with phospholipid vesicles: a fluorescence study. Biochemistry. 1991 Jun 4;30(22):5491–5497. doi: 10.1021/bi00236a023. [DOI] [PubMed] [Google Scholar]
- Cobet W. W., Mollay C., Müller G., Zimmermann R. Export of honeybee prepromelittin in Escherichia coli depends on the membrane potential but does not depend on proteins secA and secY. J Biol Chem. 1989 Jun 15;264(17):10169–10176. [PubMed] [Google Scholar]
- Davis J. H., Hodges R. S., Bloom M. The Interaction between a Synthetic Amphiphilic Polypeptide and Lipids. Biophys J. 1982 Jan;37(1):170–171. doi: 10.1016/S0006-3495(82)84655-9. [DOI] [PMC free article] [PubMed] [Google Scholar]
- Deisenhofer J., Michel H. High-resolution structures of photosynthetic reaction centers. Annu Rev Biophys Biophys Chem. 1991;20:247–266. doi: 10.1146/annurev.bb.20.060191.001335. [DOI] [PubMed] [Google Scholar]
- Emr S. D., Hanley-Way S., Silhavy T. J. Suppressor mutations that restore export of a protein with a defective signal sequence. Cell. 1981 Jan;23(1):79–88. doi: 10.1016/0092-8674(81)90272-5. [DOI] [PubMed] [Google Scholar]
- Gennity J., Goldstein J., Inouye M. Signal peptide mutants of Escherichia coli. J Bioenerg Biomembr. 1990 Jun;22(3):233–269. doi: 10.1007/BF00763167. [DOI] [PubMed] [Google Scholar]
- Gierasch L. M. Signal sequences. Biochemistry. 1989 Feb 7;28(3):923–930. doi: 10.1021/bi00429a001. [DOI] [PubMed] [Google Scholar]
- Henderson R., Baldwin J. M., Ceska T. A., Zemlin F., Beckmann E., Downing K. H. Model for the structure of bacteriorhodopsin based on high-resolution electron cryo-microscopy. J Mol Biol. 1990 Jun 20;213(4):899–929. doi: 10.1016/S0022-2836(05)80271-2. [DOI] [PubMed] [Google Scholar]
- Hoyt D. W., Gierasch L. M. A peptide corresponding to an export-defective mutant OmpA signal sequence with asparagine in the hydrophobic core is unable to insert into model membranes. J Biol Chem. 1991 Aug 5;266(22):14406–14412. [PubMed] [Google Scholar]
- Hoyt D. W., Gierasch L. M. Hydrophobic content and lipid interactions of wild-type and mutant OmpA signal peptides correlate with their in vivo function. Biochemistry. 1991 Oct 22;30(42):10155–10163. doi: 10.1021/bi00106a012. [DOI] [PubMed] [Google Scholar]
- Ito K. Identification of the secY (prlA) gene product involved in protein export in Escherichia coli. Mol Gen Genet. 1984;197(2):204–208. doi: 10.1007/BF00330964. [DOI] [PubMed] [Google Scholar]
- Jacobs R. E., White S. H. The nature of the hydrophobic binding of small peptides at the bilayer interface: implications for the insertion of transbilayer helices. Biochemistry. 1989 Apr 18;28(8):3421–3437. doi: 10.1021/bi00434a042. [DOI] [PubMed] [Google Scholar]
- Janin J., Miller S., Chothia C. Surface, subunit interfaces and interior of oligomeric proteins. J Mol Biol. 1988 Nov 5;204(1):155–164. doi: 10.1016/0022-2836(88)90606-7. [DOI] [PubMed] [Google Scholar]
- Jones J. D., McKnight C. J., Gierasch L. M. Biophysical studies of signal peptides: implications for signal sequence functions and the involvement of lipid in protein export. J Bioenerg Biomembr. 1990 Jun;22(3):213–232. doi: 10.1007/BF00763166. [DOI] [PubMed] [Google Scholar]
- Katakai R., Wanikawa K., Saga K. Experimental evidence for predicted transmembrane peptide topography: incorporation of hydrophobic peptide alpha-helical rods with an N-terminal positive charge having a length comparable to the thickness of lipid bilayers into the membranes. Biopolymers. 1990;30(7-8):815–819. doi: 10.1002/bip.360300716. [DOI] [PubMed] [Google Scholar]
- Killian J. A., Keller R. C., Struyvé M., de Kroon A. I., Tommassen J., de Kruijff B. Tryptophan fluorescence study on the interaction of the signal peptide of the Escherichia coli outer membrane protein PhoE with model membranes. Biochemistry. 1990 Sep 4;29(35):8131–8137. doi: 10.1021/bi00487a021. [DOI] [PubMed] [Google Scholar]
- London E., Feigenson G. W. Fluorescence quenching in model membranes. 1. Characterization of quenching caused by a spin-labeled phospholipid. Biochemistry. 1981 Mar 31;20(7):1932–1938. doi: 10.1021/bi00510a032. [DOI] [PubMed] [Google Scholar]
- London E. Investigation of membrane structure using fluorescence quenching by spin-labels. A review of recent studies. Mol Cell Biochem. 1982 Jun 25;45(3):181–188. doi: 10.1007/BF00230086. [DOI] [PubMed] [Google Scholar]
- Markello T., Zlotnick A., Everett J., Tennyson J., Holloway P. W. Determination of the topography of cytochrome b5 in lipid vesicles by fluorescence quenching. Biochemistry. 1985 Jun 4;24(12):2895–2901. doi: 10.1021/bi00333a012. [DOI] [PubMed] [Google Scholar]
- Mayer L. D., Hope M. J., Cullis P. R. Vesicles of variable sizes produced by a rapid extrusion procedure. Biochim Biophys Acta. 1986 Jun 13;858(1):161–168. doi: 10.1016/0005-2736(86)90302-0. [DOI] [PubMed] [Google Scholar]
- McKnight C. J., Briggs M. S., Gierasch L. M. Functional and nonfunctional LamB signal sequences can be distinguished by their biophysical properties. J Biol Chem. 1989 Oct 15;264(29):17293–17297. [PubMed] [Google Scholar]
- McKnight C. J., Rafalski M., Gierasch L. M. Fluorescence analysis of tryptophan-containing variants of the LamB signal sequence upon insertion into a lipid bilayer. Biochemistry. 1991 Jun 25;30(25):6241–6246. doi: 10.1021/bi00239a023. [DOI] [PubMed] [Google Scholar]
- Meers P. Location of tryptophans in membrane-bound annexins. Biochemistry. 1990 Apr 3;29(13):3325–3330. doi: 10.1021/bi00465a025. [DOI] [PubMed] [Google Scholar]
- Puziss J. W., Fikes J. D., Bassford P. J., Jr Analysis of mutational alterations in the hydrophilic segment of the maltose-binding protein signal peptide. J Bacteriol. 1989 May;171(5):2303–2311. doi: 10.1128/jb.171.5.2303-2311.1989. [DOI] [PMC free article] [PubMed] [Google Scholar]
- Rigell C. W., de Saussure C., Freire E. Protein and lipid structural transitions in cytochrome c oxidase-dimyristoylphosphatidylcholine reconstitutions. Biochemistry. 1985 Sep 24;24(20):5638–5646. doi: 10.1021/bi00341a053. [DOI] [PubMed] [Google Scholar]
- Rizo J., Blanco F. J., Kobe B., Bruch M. D., Gierasch L. M. Conformational behavior of Escherichia coli OmpA signal peptides in membrane mimetic environments. Biochemistry. 1993 May 11;32(18):4881–4894. doi: 10.1021/bi00069a025. [DOI] [PubMed] [Google Scholar]
- Stader J., Benson S. A., Silhavy T. J. Kinetic analysis of lamB mutants suggests the signal sequence plays multiple roles in protein export. J Biol Chem. 1986 Nov 15;261(32):15075–15080. [PubMed] [Google Scholar]
- Surewicz W. K., Epand R. M. Role of peptide structure in lipid-peptide interactions: a fluorescence study of the binding of pentagastrin-related pentapeptides to phospholipid vesicles. Biochemistry. 1984 Dec 4;23(25):6072–6077. doi: 10.1021/bi00320a026. [DOI] [PubMed] [Google Scholar]
- Vogel H., Nilsson L., Rigler R., Voges K. P., Jung G. Structural fluctuations of a helical polypeptide traversing a lipid bilayer. Proc Natl Acad Sci U S A. 1988 Jul;85(14):5067–5071. doi: 10.1073/pnas.85.14.5067. [DOI] [PMC free article] [PubMed] [Google Scholar]
- Voges K. P., Jung G., Sawyer W. H. Depth-dependent fluorescent quenching of a tryptophan residue located at defined positions on a rigid 21-peptide helix in liposomes. Biochim Biophys Acta. 1987 Jan 9;896(1):64–76. doi: 10.1016/0005-2736(87)90357-9. [DOI] [PubMed] [Google Scholar]
- Wang Z., Jones J. D., Rizo J., Gierasch L. M. Membrane-bound conformation of a signal peptide: a transferred nuclear Overhauser effect analysis. Biochemistry. 1993 Dec 21;32(50):13991–13999. doi: 10.1021/bi00213a032. [DOI] [PubMed] [Google Scholar]
- Wickner W. Assembly of proteins into membranes. Science. 1980 Nov 21;210(4472):861–868. doi: 10.1126/science.7001628. [DOI] [PubMed] [Google Scholar]
- Wolfe P. B., Rice M., Wickner W. Effects of two sec genes on protein assembly into the plasma membrane of Escherichia coli. J Biol Chem. 1985 Feb 10;260(3):1836–1841. [PubMed] [Google Scholar]
- von Heijne G., Blomberg C. Trans-membrane translocation of proteins. The direct transfer model. Eur J Biochem. 1979 Jun;97(1):175–181. doi: 10.1111/j.1432-1033.1979.tb13100.x. [DOI] [PubMed] [Google Scholar]