Abstract
Cystic fibrosis transmembrane conductance regulator (CFTR) Cl- channels appear to be regulated by hydrolysis of ATP and are inhibited by a product of hydrolysis, ADP. We assessed the effect of the other product of hydrolysis, inorganic phosphate (P(i)), on CFTR Cl- channel activity using the excised inside-out configuration of the patch-clamp technique. Millimolar concentrations of P(i) caused a dose-dependent stimulation of CFTR Cl- channel activity. Single-channel analysis demonstrated that the increase in macroscopic current was due to an increase in single-channel open-state probability (po) and not single-channel conductance. Kinetic modeling of the effect of P(i) using a linear three-state model indicated that the effect on po was predominantly the result of an increase in the rate at which the channel passed from the long closed state to the bursting state. P(i) also potentiated activity of channels studied in the presence of 10 mM ATP and stimulated Cl- currents in CFTR mutants lacking much of the R domain. Binding studies with a photoactivatable ATP analog indicated that Pi decreased the amount of bound nucleotide. These results suggest that P(i) increased CFTR Cl- channel activity by stimulating a rate-limiting step in channel opening that may occur by an interaction of P(i) at one or both nucleotide-binding domains.
Full text
PDF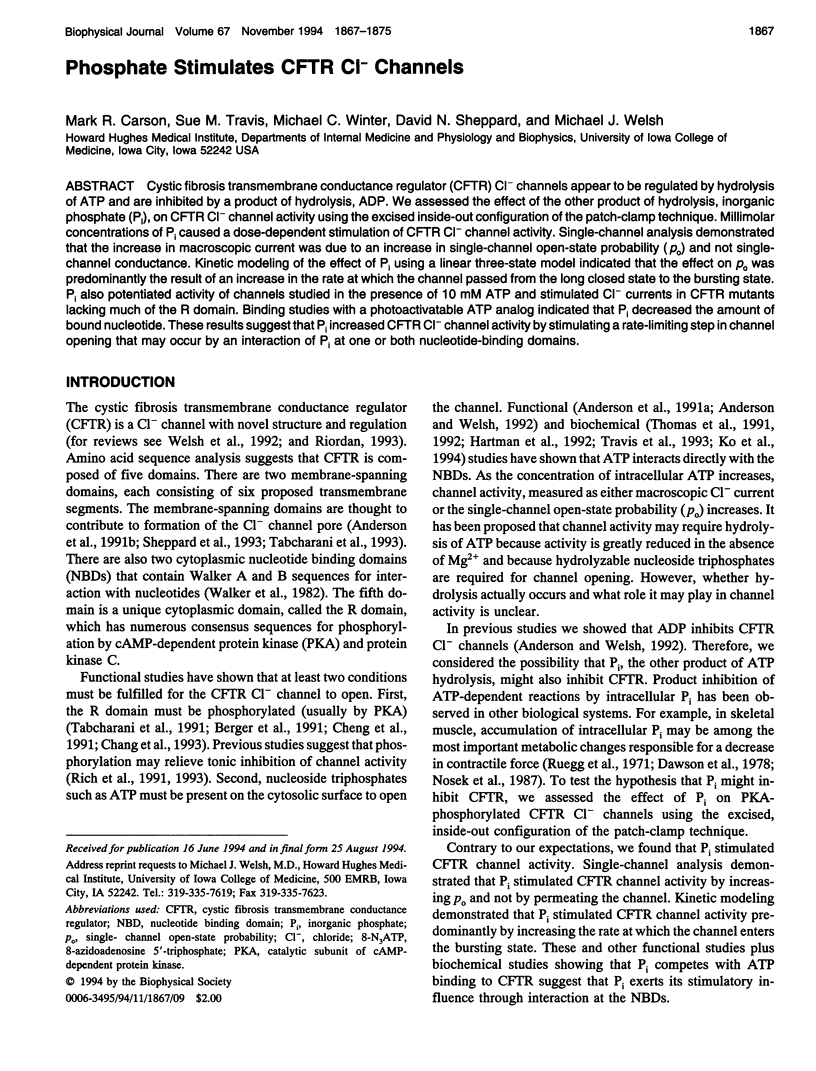
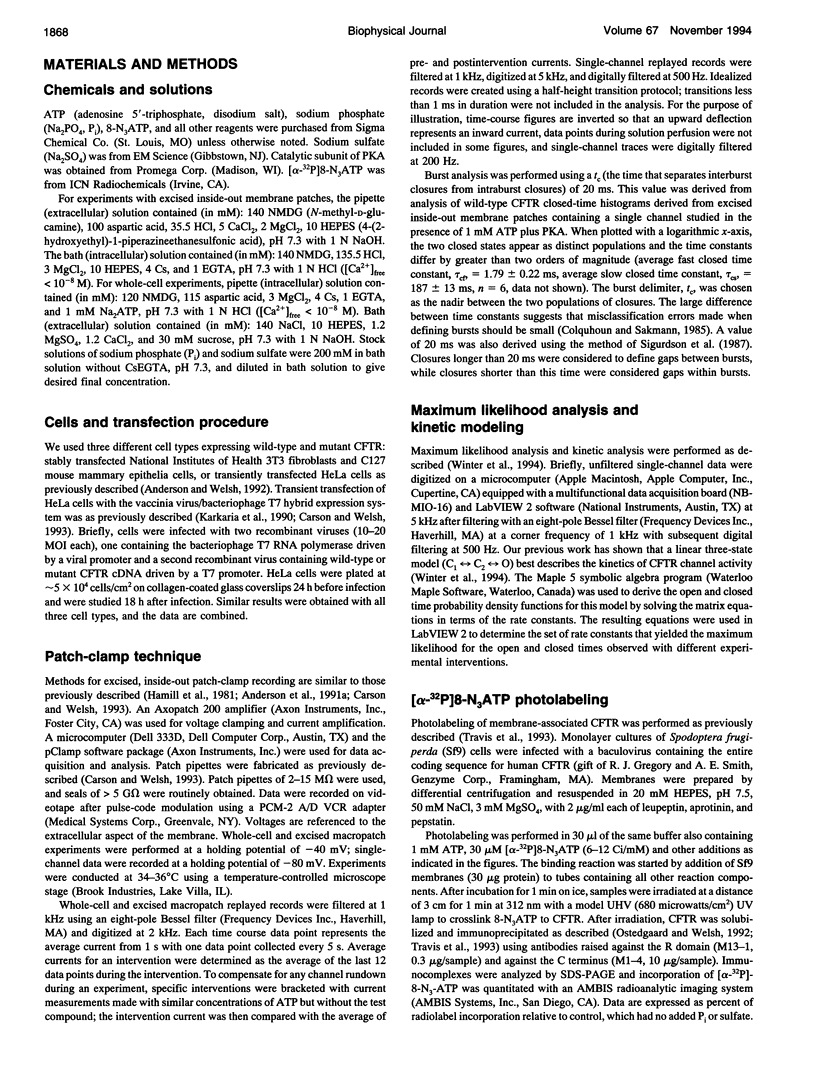
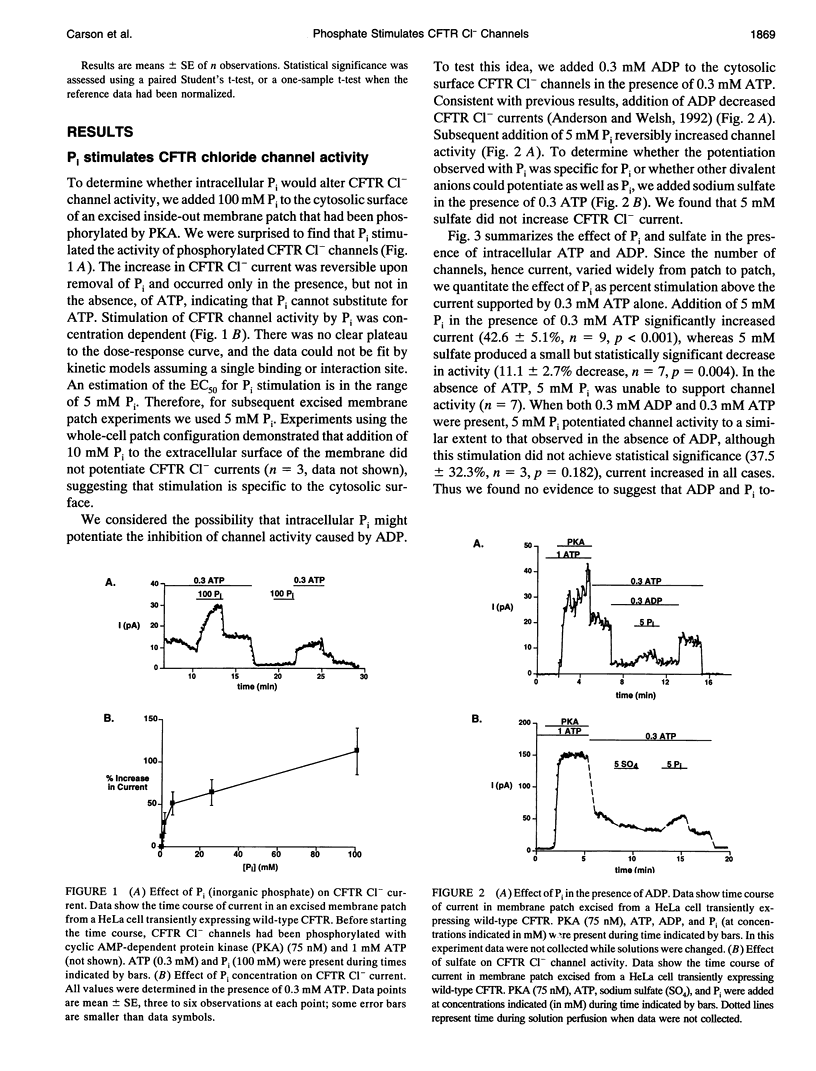
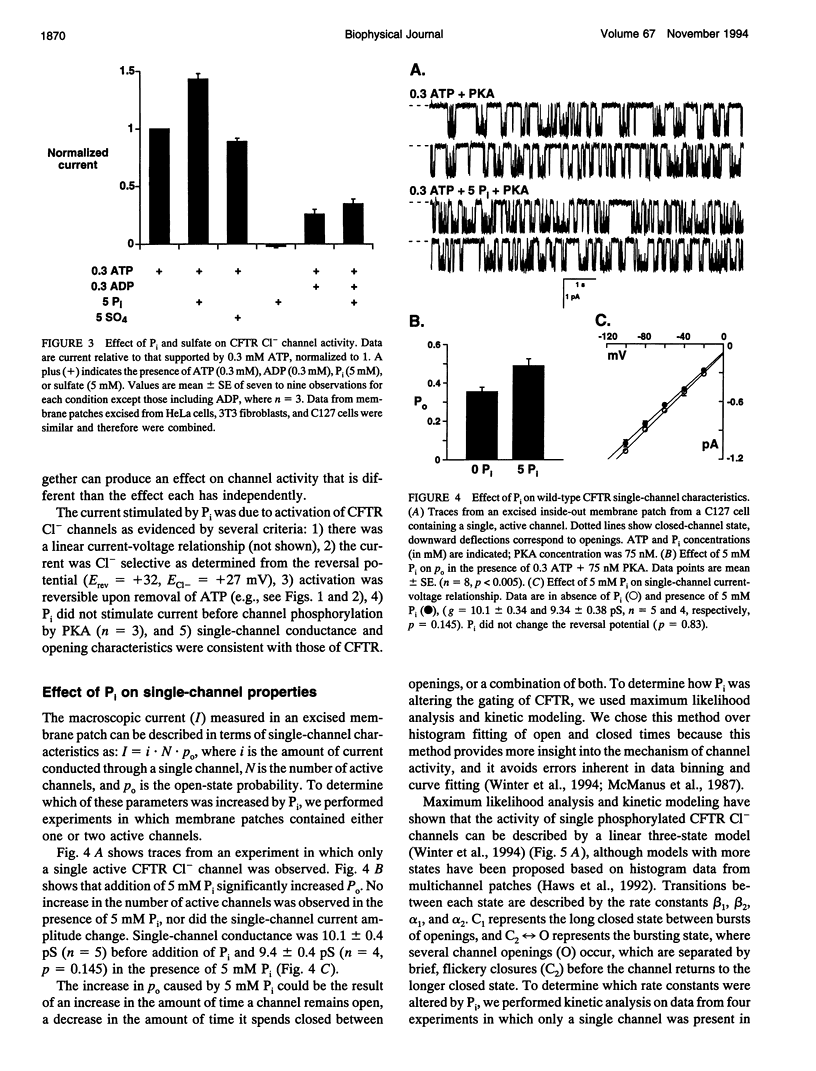
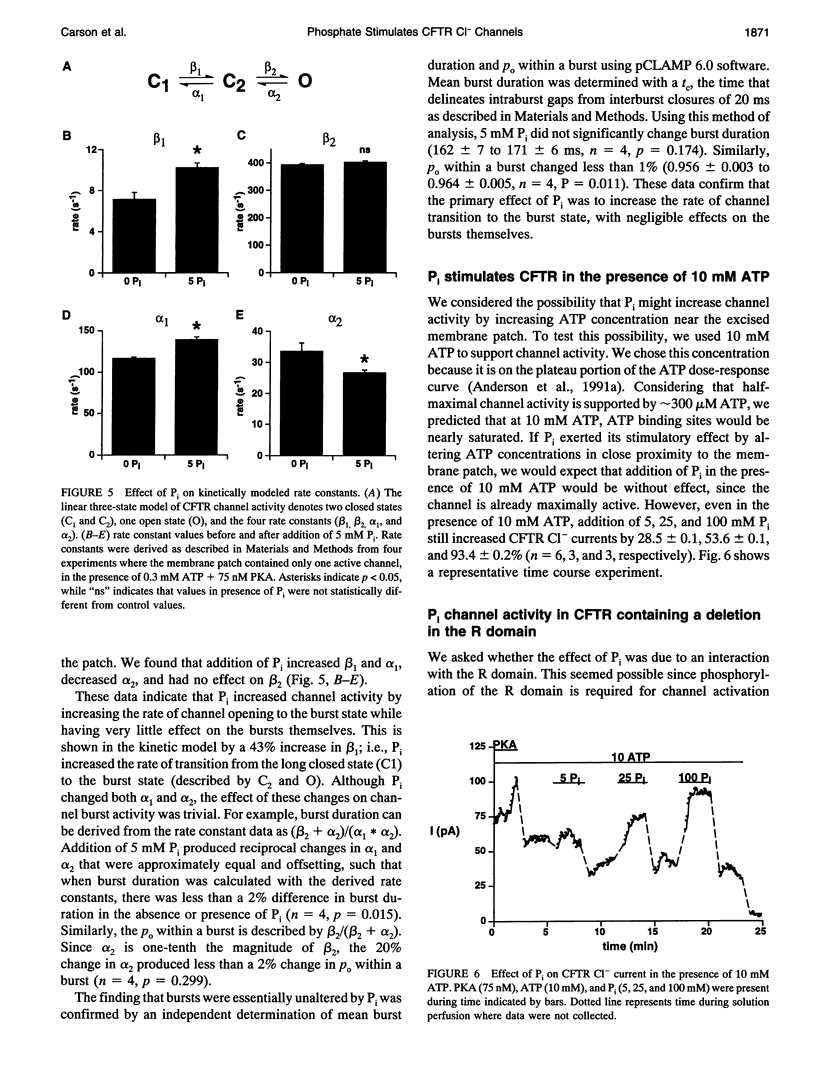
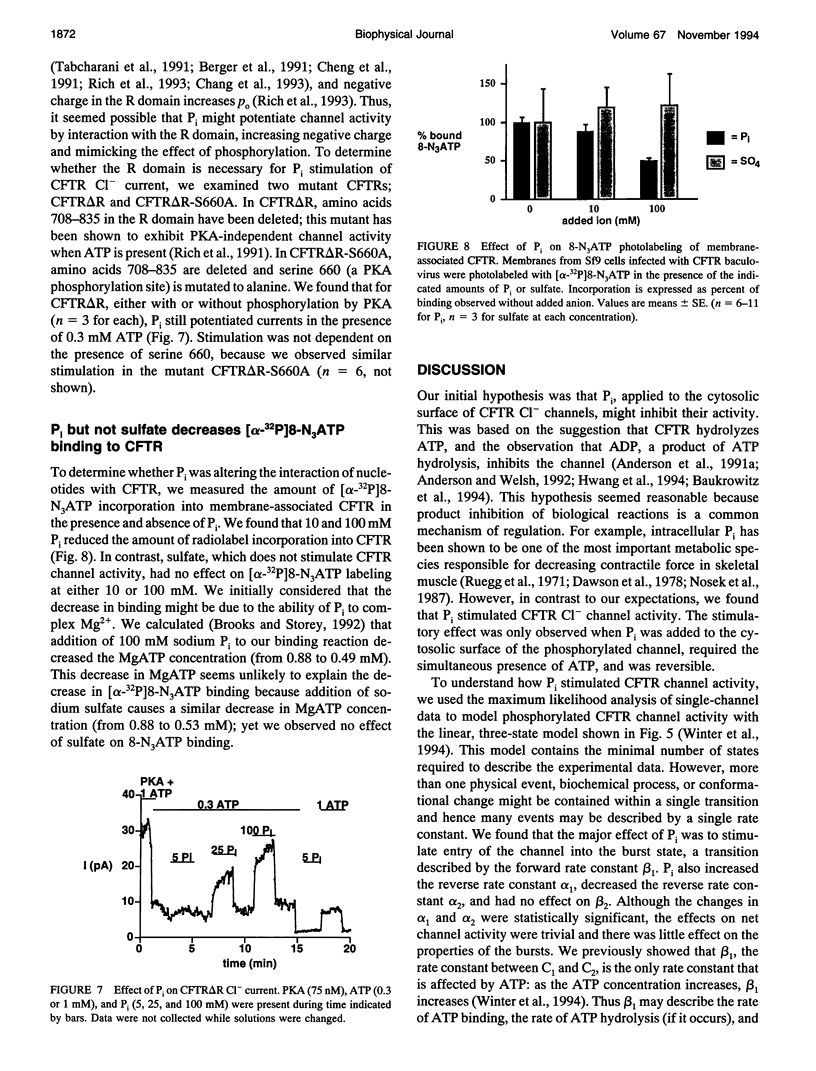
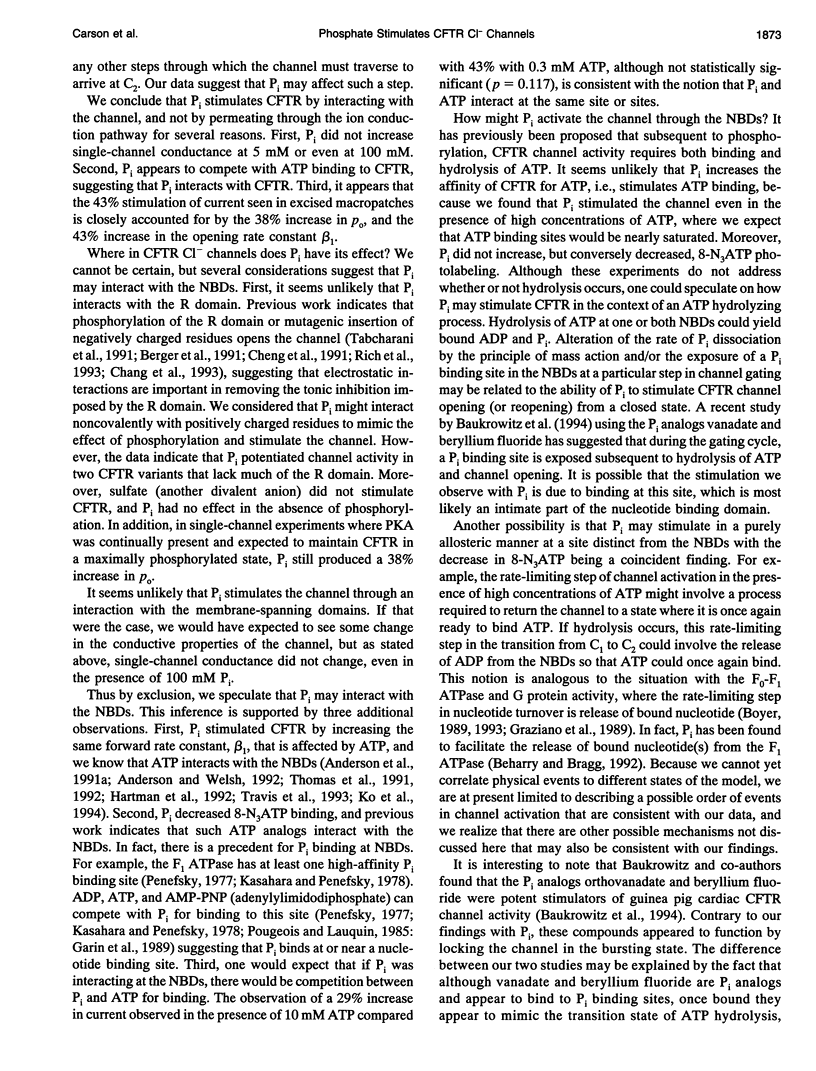
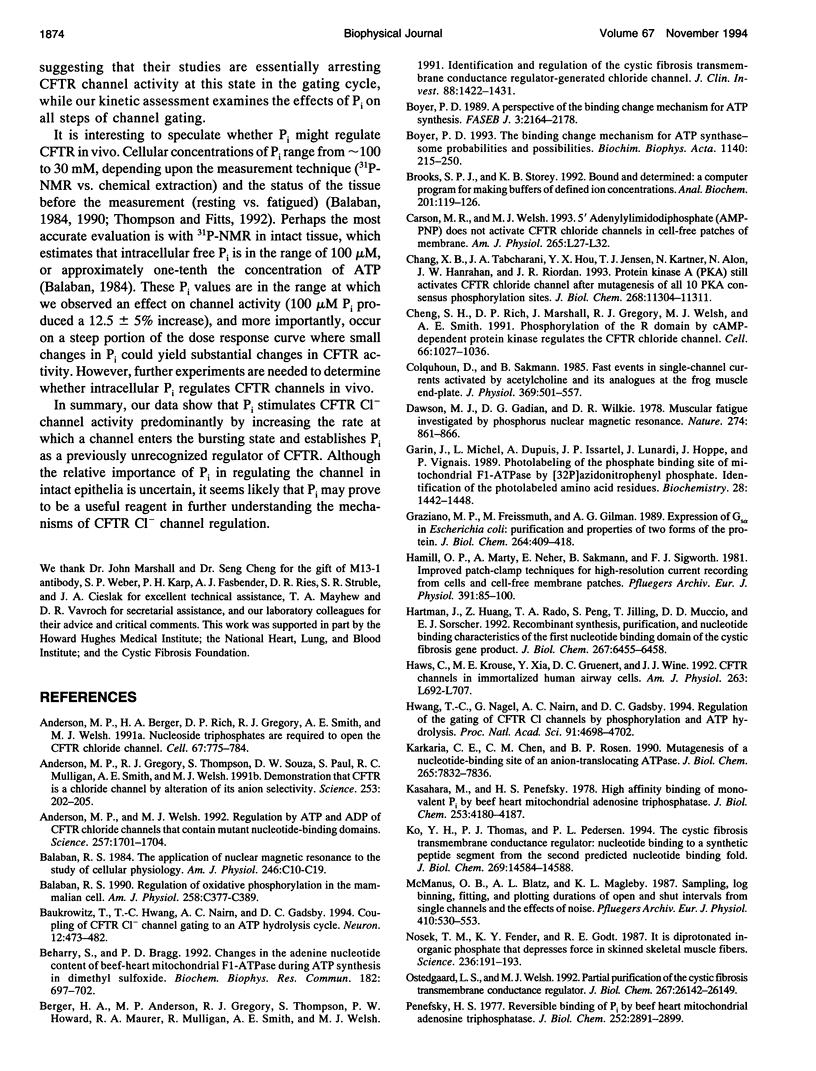
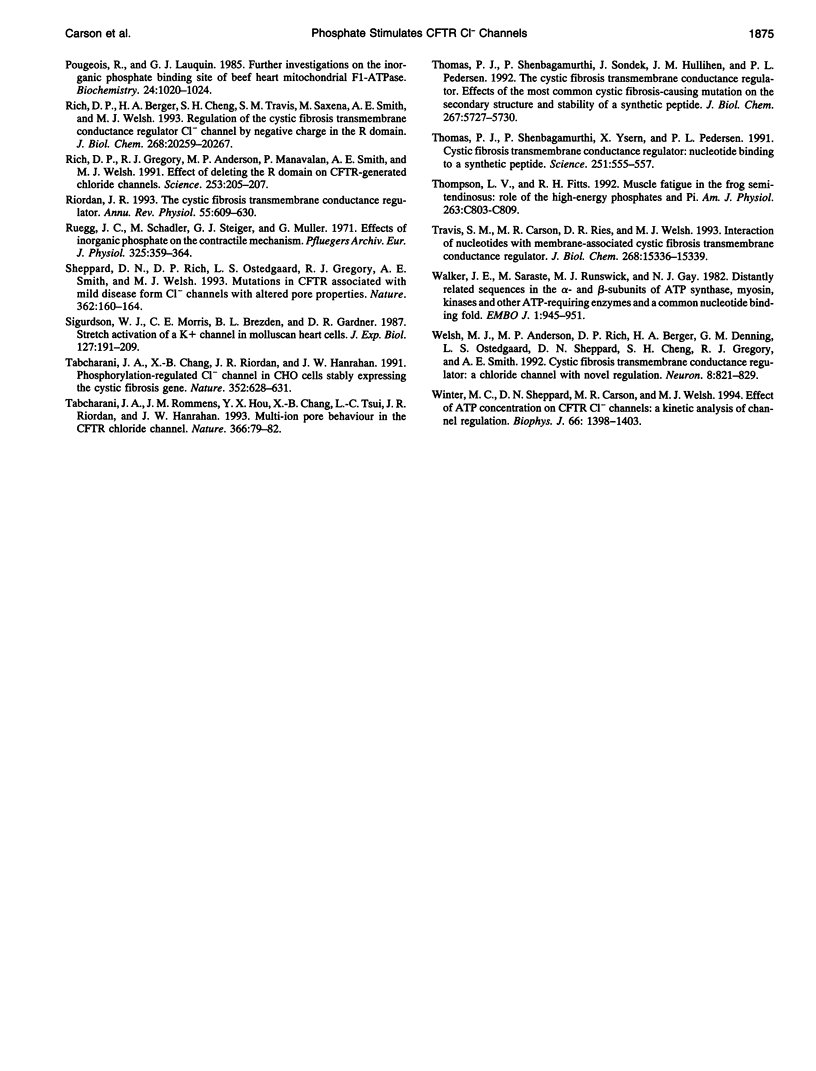
Images in this article
Selected References
These references are in PubMed. This may not be the complete list of references from this article.
- Anderson M. P., Berger H. A., Rich D. P., Gregory R. J., Smith A. E., Welsh M. J. Nucleoside triphosphates are required to open the CFTR chloride channel. Cell. 1991 Nov 15;67(4):775–784. doi: 10.1016/0092-8674(91)90072-7. [DOI] [PubMed] [Google Scholar]
- Anderson M. P., Gregory R. J., Thompson S., Souza D. W., Paul S., Mulligan R. C., Smith A. E., Welsh M. J. Demonstration that CFTR is a chloride channel by alteration of its anion selectivity. Science. 1991 Jul 12;253(5016):202–205. doi: 10.1126/science.1712984. [DOI] [PubMed] [Google Scholar]
- Anderson M. P., Welsh M. J. Regulation by ATP and ADP of CFTR chloride channels that contain mutant nucleotide-binding domains. Science. 1992 Sep 18;257(5077):1701–1704. doi: 10.1126/science.1382316. [DOI] [PubMed] [Google Scholar]
- Balaban R. S. Regulation of oxidative phosphorylation in the mammalian cell. Am J Physiol. 1990 Mar;258(3 Pt 1):C377–C389. doi: 10.1152/ajpcell.1990.258.3.C377. [DOI] [PubMed] [Google Scholar]
- Balaban R. S. The application of nuclear magnetic resonance to the study of cellular physiology. Am J Physiol. 1984 Jan;246(1 Pt 1):C10–C19. doi: 10.1152/ajpcell.1984.246.1.C10. [DOI] [PubMed] [Google Scholar]
- Baukrowitz T., Hwang T. C., Nairn A. C., Gadsby D. C. Coupling of CFTR Cl- channel gating to an ATP hydrolysis cycle. Neuron. 1994 Mar;12(3):473–482. doi: 10.1016/0896-6273(94)90206-2. [DOI] [PubMed] [Google Scholar]
- Beharry S., Bragg P. D. Changes in the adenine nucleotide content of beef-heart mitochondrial F1 ATPase during ATP synthesis in dimethyl sulfoxide. Biochem Biophys Res Commun. 1992 Jan 31;182(2):697–702. doi: 10.1016/0006-291x(92)91788-r. [DOI] [PubMed] [Google Scholar]
- Berger H. A., Anderson M. P., Gregory R. J., Thompson S., Howard P. W., Maurer R. A., Mulligan R., Smith A. E., Welsh M. J. Identification and regulation of the cystic fibrosis transmembrane conductance regulator-generated chloride channel. J Clin Invest. 1991 Oct;88(4):1422–1431. doi: 10.1172/JCI115450. [DOI] [PMC free article] [PubMed] [Google Scholar]
- Boyer P. D. A perspective of the binding change mechanism for ATP synthesis. FASEB J. 1989 Aug;3(10):2164–2178. doi: 10.1096/fasebj.3.10.2526771. [DOI] [PubMed] [Google Scholar]
- Boyer P. D. The binding change mechanism for ATP synthase--some probabilities and possibilities. Biochim Biophys Acta. 1993 Jan 8;1140(3):215–250. doi: 10.1016/0005-2728(93)90063-l. [DOI] [PubMed] [Google Scholar]
- Brooks S. P., Storey K. B. Bound and determined: a computer program for making buffers of defined ion concentrations. Anal Biochem. 1992 Feb 14;201(1):119–126. doi: 10.1016/0003-2697(92)90183-8. [DOI] [PubMed] [Google Scholar]
- Carson M. R., Welsh M. J. 5'-Adenylylimidodiphosphate does not activate CFTR chloride channels in cell-free patches of membrane. Am J Physiol. 1993 Jul;265(1 Pt 1):L27–L32. doi: 10.1152/ajplung.1993.265.1.L27. [DOI] [PubMed] [Google Scholar]
- Chang X. B., Tabcharani J. A., Hou Y. X., Jensen T. J., Kartner N., Alon N., Hanrahan J. W., Riordan J. R. Protein kinase A (PKA) still activates CFTR chloride channel after mutagenesis of all 10 PKA consensus phosphorylation sites. J Biol Chem. 1993 May 25;268(15):11304–11311. [PubMed] [Google Scholar]
- Cheng S. H., Rich D. P., Marshall J., Gregory R. J., Welsh M. J., Smith A. E. Phosphorylation of the R domain by cAMP-dependent protein kinase regulates the CFTR chloride channel. Cell. 1991 Sep 6;66(5):1027–1036. doi: 10.1016/0092-8674(91)90446-6. [DOI] [PubMed] [Google Scholar]
- Colquhoun D., Sakmann B. Fast events in single-channel currents activated by acetylcholine and its analogues at the frog muscle end-plate. J Physiol. 1985 Dec;369:501–557. doi: 10.1113/jphysiol.1985.sp015912. [DOI] [PMC free article] [PubMed] [Google Scholar]
- Dawson M. J., Gadian D. G., Wilkie D. R. Muscular fatigue investigated by phosphorus nuclear magnetic resonance. Nature. 1978 Aug 31;274(5674):861–866. doi: 10.1038/274861a0. [DOI] [PubMed] [Google Scholar]
- Garin J., Michel L., Dupuis A., Issartel J. P., Lunardi J., Hoppe J., Vignais P. Photolabeling of the phosphate binding site of mitochondrial F1-ATPase by [32P]azidonitrophenyl phosphate. Identification of the photolabeled amino acid residues. Biochemistry. 1989 Feb 21;28(4):1442–1448. doi: 10.1021/bi00430a003. [DOI] [PubMed] [Google Scholar]
- Graziano M. P., Freissmuth M., Gilman A. G. Expression of Gs alpha in Escherichia coli. Purification and properties of two forms of the protein. J Biol Chem. 1989 Jan 5;264(1):409–418. [PubMed] [Google Scholar]
- Hamill O. P., Marty A., Neher E., Sakmann B., Sigworth F. J. Improved patch-clamp techniques for high-resolution current recording from cells and cell-free membrane patches. Pflugers Arch. 1981 Aug;391(2):85–100. doi: 10.1007/BF00656997. [DOI] [PubMed] [Google Scholar]
- Hartman J., Huang Z., Rado T. A., Peng S., Jilling T., Muccio D. D., Sorscher E. J. Recombinant synthesis, purification, and nucleotide binding characteristics of the first nucleotide binding domain of the cystic fibrosis gene product. J Biol Chem. 1992 Apr 5;267(10):6455–6458. [PubMed] [Google Scholar]
- Haws C., Krouse M. E., Xia Y., Gruenert D. C., Wine J. J. CFTR channels in immortalized human airway cells. Am J Physiol. 1992 Dec;263(6 Pt 1):L692–L707. doi: 10.1152/ajplung.1992.263.6.L692. [DOI] [PubMed] [Google Scholar]
- Hwang T. C., Nagel G., Nairn A. C., Gadsby D. C. Regulation of the gating of cystic fibrosis transmembrane conductance regulator C1 channels by phosphorylation and ATP hydrolysis. Proc Natl Acad Sci U S A. 1994 May 24;91(11):4698–4702. doi: 10.1073/pnas.91.11.4698. [DOI] [PMC free article] [PubMed] [Google Scholar]
- Karkaria C. E., Chen C. M., Rosen B. P. Mutagenesis of a nucleotide-binding site of an anion-translocating ATPase. J Biol Chem. 1990 May 15;265(14):7832–7836. [PubMed] [Google Scholar]
- Kasahara M., Penefsky H. S. High affinity binding of monovalent Pi by beef heart mitochondrial adenosine triphosphatase. J Biol Chem. 1978 Jun 25;253(12):4180–4187. [PubMed] [Google Scholar]
- Ko Y. H., Thomas P. J., Pedersen P. L. The cystic fibrosis transmembrane conductance regulator. Nucleotide binding to a synthetic peptide segment from the second predicted nucleotide binding fold. J Biol Chem. 1994 May 20;269(20):14584–14588. [PubMed] [Google Scholar]
- McManus O. B., Blatz A. L., Magleby K. L. Sampling, log binning, fitting, and plotting durations of open and shut intervals from single channels and the effects of noise. Pflugers Arch. 1987 Nov;410(4-5):530–553. doi: 10.1007/BF00586537. [DOI] [PubMed] [Google Scholar]
- Nosek T. M., Fender K. Y., Godt R. E. It is diprotonated inorganic phosphate that depresses force in skinned skeletal muscle fibers. Science. 1987 Apr 10;236(4798):191–193. doi: 10.1126/science.3563496. [DOI] [PubMed] [Google Scholar]
- Ostedgaard L. S., Welsh M. J. Partial purification of the cystic fibrosis transmembrane conductance regulator. J Biol Chem. 1992 Dec 25;267(36):26142–26149. [PubMed] [Google Scholar]
- Penefsky H. S. Reversible binding of Pi by beef heart mitochondrial adenosine triphosphatase. J Biol Chem. 1977 May 10;252(9):2891–2899. [PubMed] [Google Scholar]
- Pougeois R., Lauquin G. J. Further investigations on the inorganic phosphate binding site of beef heart mitochondrial F1-ATPase. Biochemistry. 1985 Feb 12;24(4):1020–1024. doi: 10.1021/bi00325a032. [DOI] [PubMed] [Google Scholar]
- Rich D. P., Berger H. A., Cheng S. H., Travis S. M., Saxena M., Smith A. E., Welsh M. J. Regulation of the cystic fibrosis transmembrane conductance regulator Cl- channel by negative charge in the R domain. J Biol Chem. 1993 Sep 25;268(27):20259–20267. [PubMed] [Google Scholar]
- Rich D. P., Gregory R. J., Anderson M. P., Manavalan P., Smith A. E., Welsh M. J. Effect of deleting the R domain on CFTR-generated chloride channels. Science. 1991 Jul 12;253(5016):205–207. doi: 10.1126/science.1712985. [DOI] [PubMed] [Google Scholar]
- Riordan J. R. The cystic fibrosis transmembrane conductance regulator. Annu Rev Physiol. 1993;55:609–630. doi: 10.1146/annurev.ph.55.030193.003141. [DOI] [PubMed] [Google Scholar]
- Rüegg J. C., Schädler M., Steiger G. J., Müller G. Effects of inorganic phosphate on the contractile mechanism. Pflugers Arch. 1971;325(4):359–364. doi: 10.1007/BF00592176. [DOI] [PubMed] [Google Scholar]
- Sheppard D. N., Rich D. P., Ostedgaard L. S., Gregory R. J., Smith A. E., Welsh M. J. Mutations in CFTR associated with mild-disease-form Cl- channels with altered pore properties. Nature. 1993 Mar 11;362(6416):160–164. doi: 10.1038/362160a0. [DOI] [PubMed] [Google Scholar]
- Tabcharani J. A., Chang X. B., Riordan J. R., Hanrahan J. W. Phosphorylation-regulated Cl- channel in CHO cells stably expressing the cystic fibrosis gene. Nature. 1991 Aug 15;352(6336):628–631. doi: 10.1038/352628a0. [DOI] [PubMed] [Google Scholar]
- Tabcharani J. A., Rommens J. M., Hou Y. X., Chang X. B., Tsui L. C., Riordan J. R., Hanrahan J. W. Multi-ion pore behaviour in the CFTR chloride channel. Nature. 1993 Nov 4;366(6450):79–82. doi: 10.1038/366079a0. [DOI] [PubMed] [Google Scholar]
- Thomas P. J., Shenbagamurthi P., Sondek J., Hullihen J. M., Pedersen P. L. The cystic fibrosis transmembrane conductance regulator. Effects of the most common cystic fibrosis-causing mutation on the secondary structure and stability of a synthetic peptide. J Biol Chem. 1992 Mar 25;267(9):5727–5730. [PubMed] [Google Scholar]
- Thomas P. J., Shenbagamurthi P., Ysern X., Pedersen P. L. Cystic fibrosis transmembrane conductance regulator: nucleotide binding to a synthetic peptide. Science. 1991 Feb 1;251(4993):555–557. doi: 10.1126/science.1703660. [DOI] [PubMed] [Google Scholar]
- Thompson L. V., Fitts R. H. Muscle fatigue in the frog semitendinosus: role of the high-energy phosphates and Pi. Am J Physiol. 1992 Oct;263(4 Pt 1):C803–C809. doi: 10.1152/ajpcell.1992.263.4.C803. [DOI] [PubMed] [Google Scholar]
- Travis S. M., Carson M. R., Ries D. R., Welsh M. J. Interaction of nucleotides with membrane-associated cystic fibrosis transmembrane conductance regulator. J Biol Chem. 1993 Jul 25;268(21):15336–15339. [PubMed] [Google Scholar]
- Walker J. E., Saraste M., Runswick M. J., Gay N. J. Distantly related sequences in the alpha- and beta-subunits of ATP synthase, myosin, kinases and other ATP-requiring enzymes and a common nucleotide binding fold. EMBO J. 1982;1(8):945–951. doi: 10.1002/j.1460-2075.1982.tb01276.x. [DOI] [PMC free article] [PubMed] [Google Scholar]
- Welsh M. J., Anderson M. P., Rich D. P., Berger H. A., Denning G. M., Ostedgaard L. S., Sheppard D. N., Cheng S. H., Gregory R. J., Smith A. E. Cystic fibrosis transmembrane conductance regulator: a chloride channel with novel regulation. Neuron. 1992 May;8(5):821–829. doi: 10.1016/0896-6273(92)90196-k. [DOI] [PubMed] [Google Scholar]
- Winter M. C., Sheppard D. N., Carson M. R., Welsh M. J. Effect of ATP concentration on CFTR Cl- channels: a kinetic analysis of channel regulation. Biophys J. 1994 May;66(5):1398–1403. doi: 10.1016/S0006-3495(94)80930-0. [DOI] [PMC free article] [PubMed] [Google Scholar]