Abstract
Fiber isometric tension redevelopment rate (kTR) was measured during submaximal and maximal activations in glycerinated fibers from rabbit psoas muscle. In fibers either containing endogenous skeletal troponin C (sTnC) or reconstituted with either purified cardiac troponin C (cTnC) or sTnC, graded activation was achieved by varying [Ca2+]. Some fibers were first partially, then fully, reconstituted with a modified form of cTnC (aTnC) that enables active force generation and shortening in the absence of Ca2+. kTR was derived from the half-time of tension redevelopment. In control fibers with endogenous sTnC, kTR increased nonlinearly with [Ca2+], and maximal kTR was 15.3 +/- 3.6 s-1 (mean +/- SD; n = 26 determinations on 25 fibers) at pCa 4.0. During submaximal activations by Ca2+, kTR in cTnC reconstituted fibers was approximately threefold faster than control, despite the lower (60%) maximum Ca(2+)-activated force after reconstitution. To obtain submaximal force with aTnC, eight fibers were treated to fully extract endogenous sTnC, then reconstituted with a mixture of a TnC and cTnC (aTnC:cTnC molar ratio 1:8.5). A second extraction selectively removed cTnC. In such fibers containing aTnC only, neither force nor kTR was affected by changes in [Ca2+]. Force was 22 +/- 7% of maximum control (mean +/- SD; n = 15) at pCa 9.2 vs. 24 +/- 8% (mean +/- SD; n = 8) at pCa 4.0, whereas kTR was 98 +/- 14% of maximum control (mean +/- SD; n = 15) at pCa 9.2 vs. 96 +/- 15% (mean +/- SD; n = 8) at pCa 4.0. Maximal reconstitution of fibers with aTnC alone increased force at pCa 9.2 to 69 +/- 5% of maximum control (mean + SD; n = 22 determinations on 13 fibers) and caused a small but significant reduction of kTR to 78 +/- 8% of maximum control (mean +/- SD; n = 22 determinations on 13 fibers); neither force nor krR was significantly affected by Ca>2(pCa 4.0). Taken together, we interpret our results to indicate that kTR reflects the dynamics of activation of individual thin filament regulatory units and that modulation of kTR by Ca> is effected primarily by Ca>+ binding to TnC.
Full text
PDF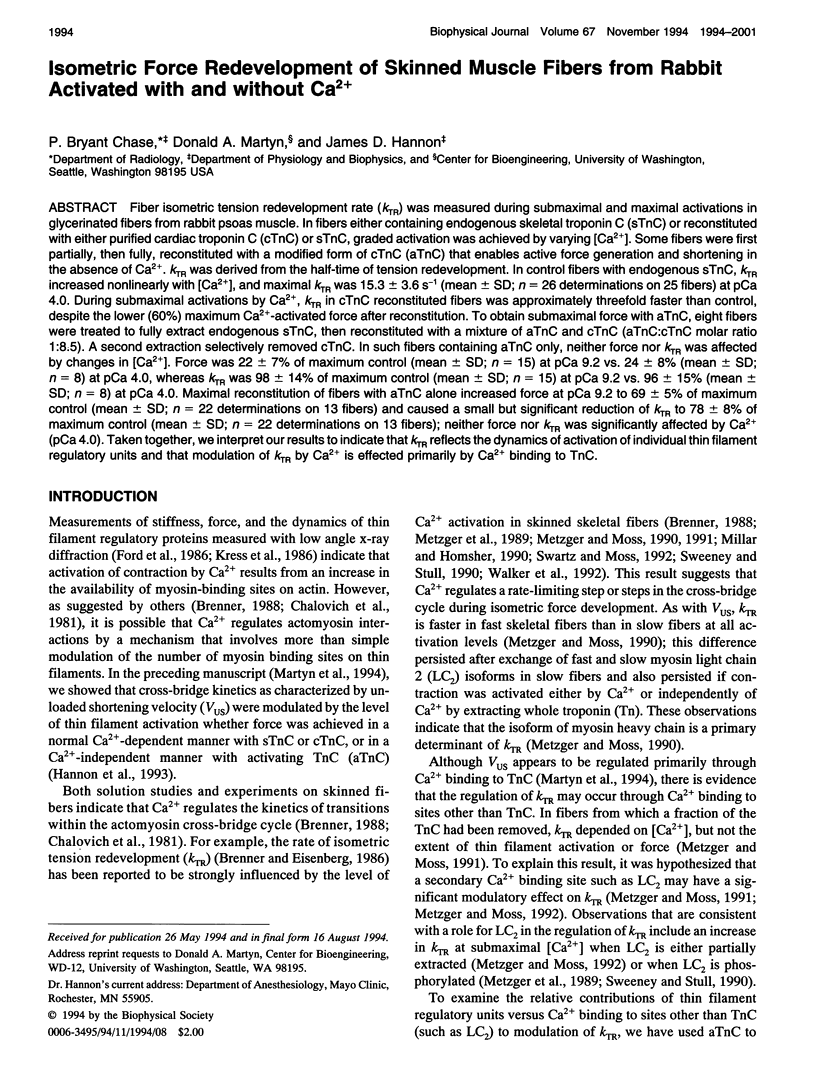
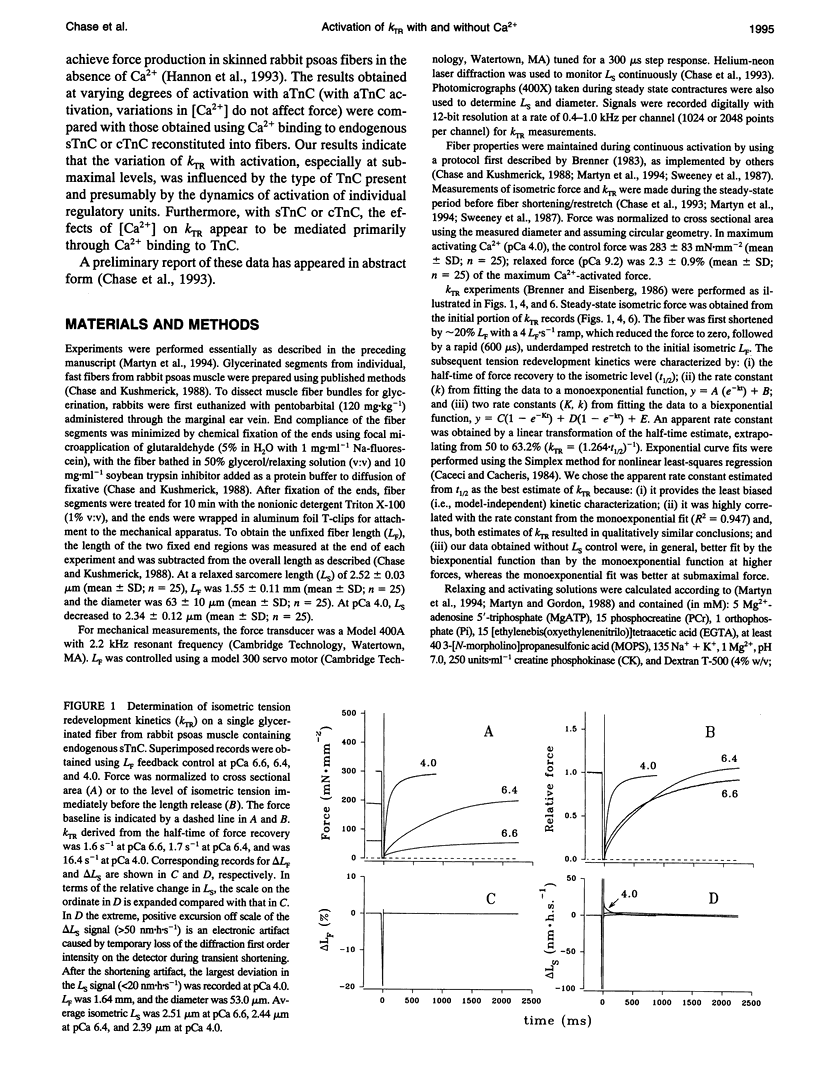
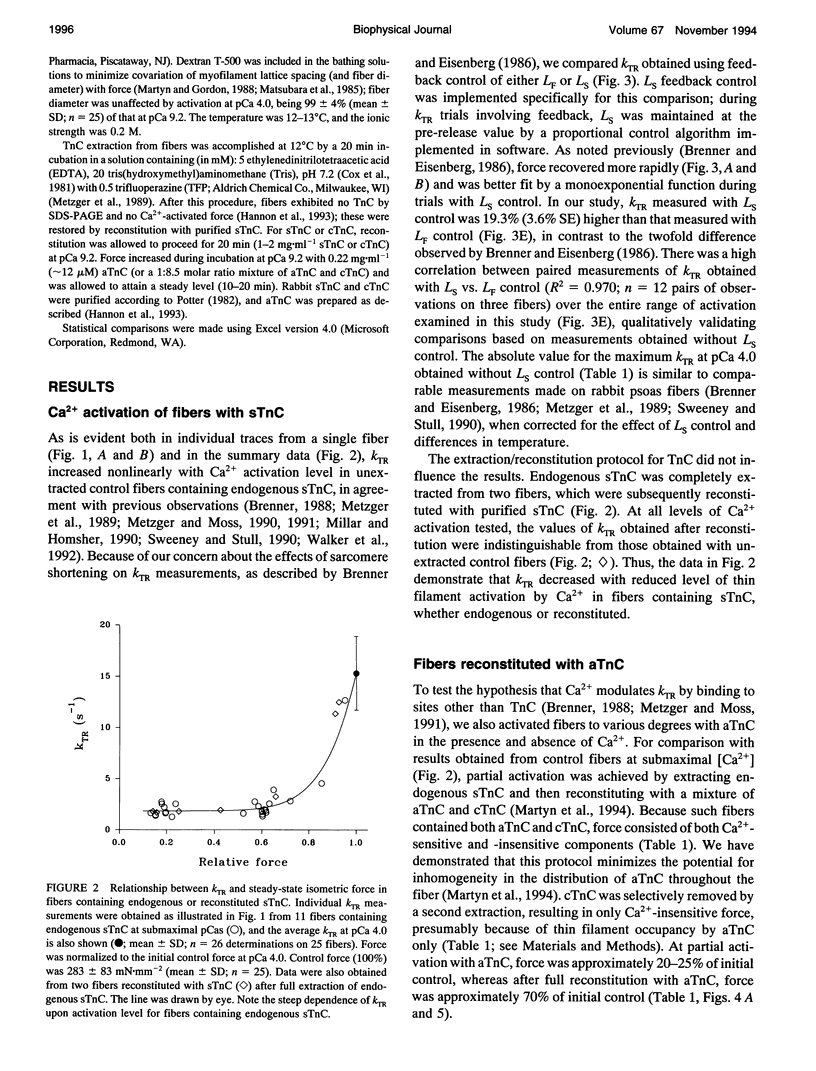
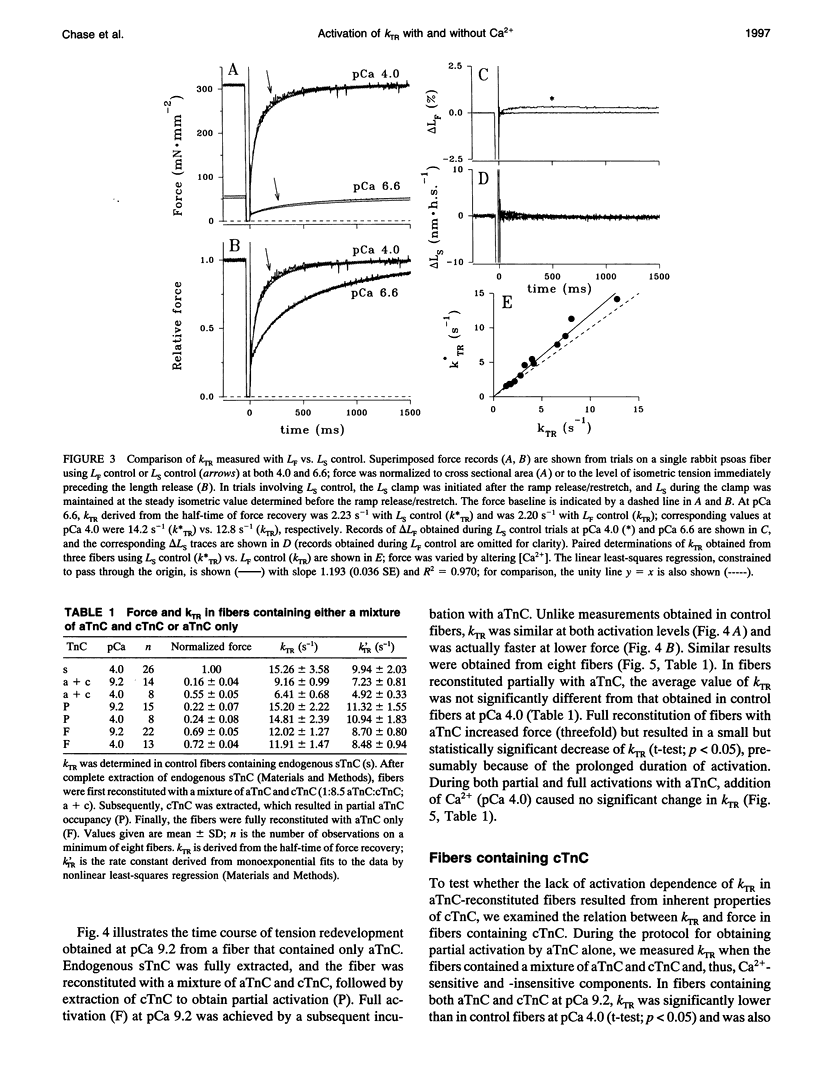
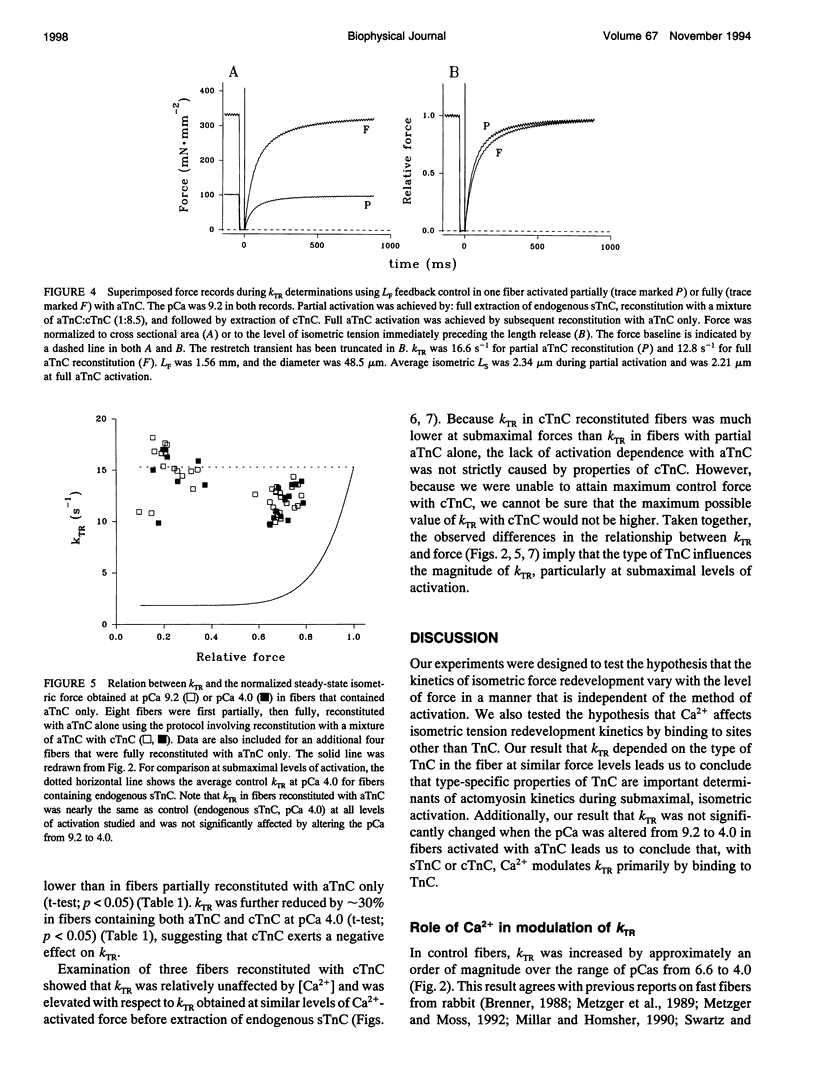
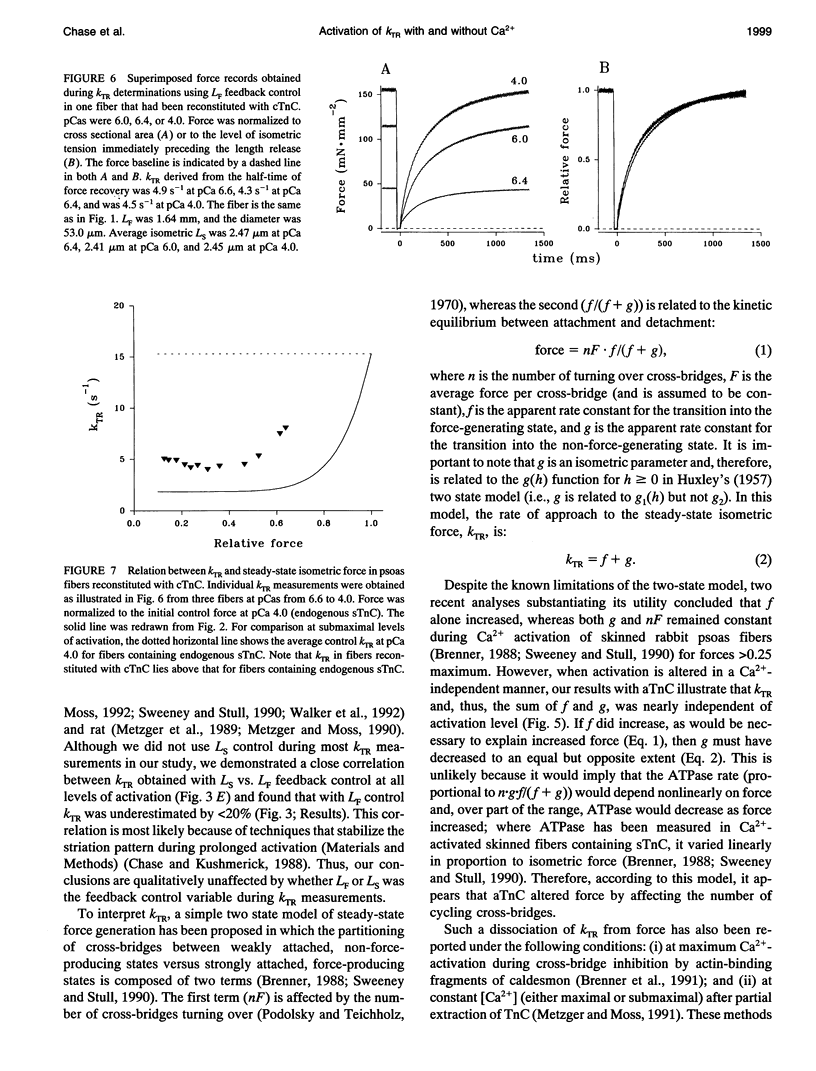
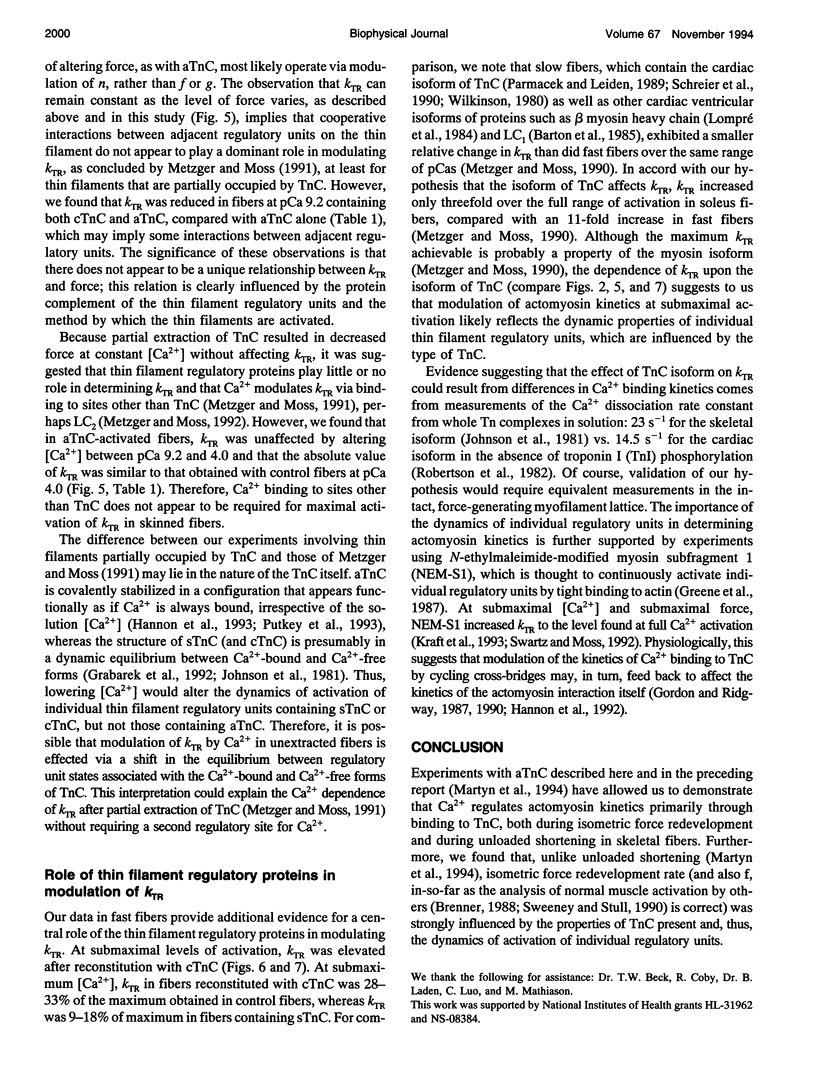
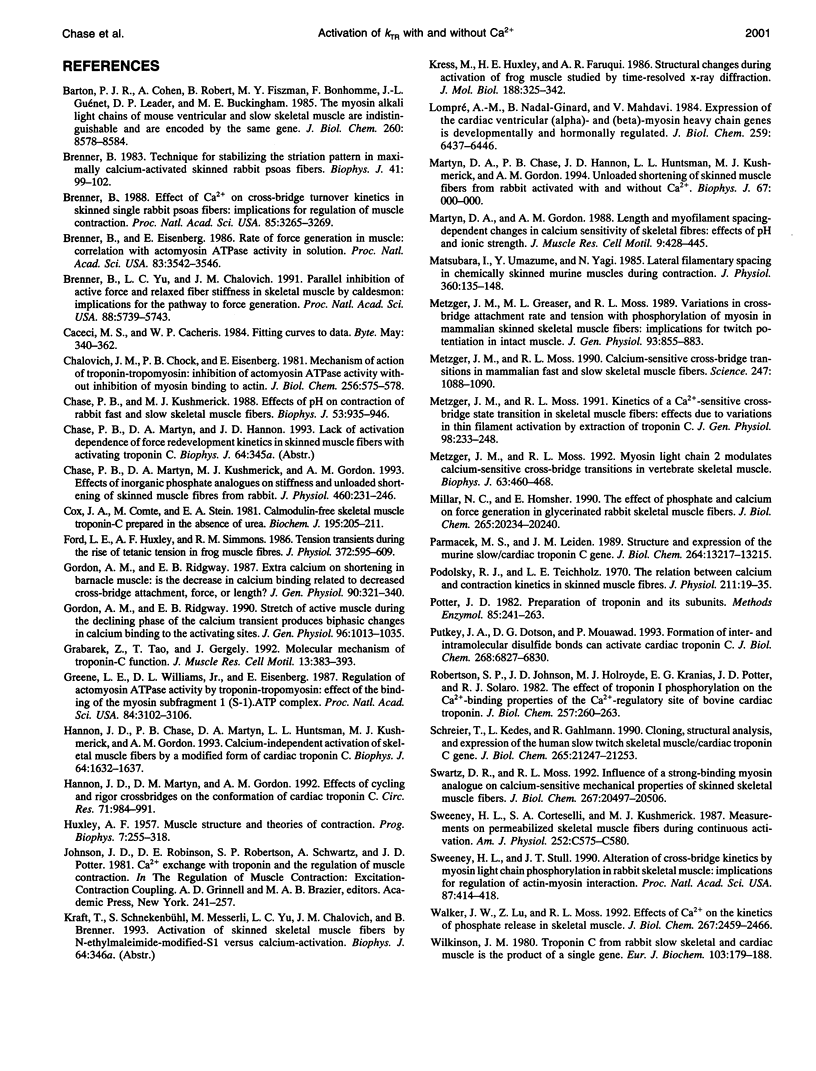
Selected References
These references are in PubMed. This may not be the complete list of references from this article.
- Barton P. J., Cohen A., Robert B., Fiszman M. Y., Bonhomme F., Guénet J. L., Leader D. P., Buckingham M. E. The myosin alkali light chains of mouse ventricular and slow skeletal muscle are indistinguishable and are encoded by the same gene. J Biol Chem. 1985 Jul 15;260(14):8578–8584. [PubMed] [Google Scholar]
- Brenner B. Effect of Ca2+ on cross-bridge turnover kinetics in skinned single rabbit psoas fibers: implications for regulation of muscle contraction. Proc Natl Acad Sci U S A. 1988 May;85(9):3265–3269. doi: 10.1073/pnas.85.9.3265. [DOI] [PMC free article] [PubMed] [Google Scholar]
- Brenner B., Eisenberg E. Rate of force generation in muscle: correlation with actomyosin ATPase activity in solution. Proc Natl Acad Sci U S A. 1986 May;83(10):3542–3546. doi: 10.1073/pnas.83.10.3542. [DOI] [PMC free article] [PubMed] [Google Scholar]
- Brenner B. Technique for stabilizing the striation pattern in maximally calcium-activated skinned rabbit psoas fibers. Biophys J. 1983 Jan;41(1):99–102. doi: 10.1016/S0006-3495(83)84411-7. [DOI] [PMC free article] [PubMed] [Google Scholar]
- Brenner B., Yu L. C., Chalovich J. M. Parallel inhibition of active force and relaxed fiber stiffness in skeletal muscle by caldesmon: implications for the pathway to force generation. Proc Natl Acad Sci U S A. 1991 Jul 1;88(13):5739–5743. doi: 10.1073/pnas.88.13.5739. [DOI] [PMC free article] [PubMed] [Google Scholar]
- Chalovich J. M., Chock P. B., Eisenberg E. Mechanism of action of troponin . tropomyosin. Inhibition of actomyosin ATPase activity without inhibition of myosin binding to actin. J Biol Chem. 1981 Jan 25;256(2):575–578. [PMC free article] [PubMed] [Google Scholar]
- Chase P. B., Kushmerick M. J. Effects of pH on contraction of rabbit fast and slow skeletal muscle fibers. Biophys J. 1988 Jun;53(6):935–946. doi: 10.1016/S0006-3495(88)83174-6. [DOI] [PMC free article] [PubMed] [Google Scholar]
- Chase P. B., Martyn D. A., Kushmerick M. J., Gordon A. M. Effects of inorganic phosphate analogues on stiffness and unloaded shortening of skinned muscle fibres from rabbit. J Physiol. 1993 Jan;460:231–246. doi: 10.1113/jphysiol.1993.sp019469. [DOI] [PMC free article] [PubMed] [Google Scholar]
- Cox J. A., Comte M., Stein E. A. Calmodulin-free skeletal-muscle troponin C prepared in the absence of urea. Biochem J. 1981 Apr 1;195(1):205–211. doi: 10.1042/bj1950205. [DOI] [PMC free article] [PubMed] [Google Scholar]
- Ford L. E., Huxley A. F., Simmons R. M. Tension transients during the rise of tetanic tension in frog muscle fibres. J Physiol. 1986 Mar;372:595–609. doi: 10.1113/jphysiol.1986.sp016027. [DOI] [PMC free article] [PubMed] [Google Scholar]
- Gordon A. M., Ridgway E. B. Extra calcium on shortening in barnacle muscle. Is the decrease in calcium binding related to decreased cross-bridge attachment, force, or length? J Gen Physiol. 1987 Sep;90(3):321–340. doi: 10.1085/jgp.90.3.321. [DOI] [PMC free article] [PubMed] [Google Scholar]
- Gordon A. M., Ridgway E. B. Stretch of active muscle during the declining phase of the calcium transient produces biphasic changes in calcium binding to the activating sites. J Gen Physiol. 1990 Nov;96(5):1013–1035. doi: 10.1085/jgp.96.5.1013. [DOI] [PMC free article] [PubMed] [Google Scholar]
- Grabarek Z., Tao T., Gergely J. Molecular mechanism of troponin-C function. J Muscle Res Cell Motil. 1992 Aug;13(4):383–393. doi: 10.1007/BF01738034. [DOI] [PubMed] [Google Scholar]
- Greene L. E., Williams D. L., Jr, Eisenberg E. Regulation of actomyosin ATPase activity by troponin-tropomyosin: effect of the binding of the myosin subfragment 1 (S-1).ATP complex. Proc Natl Acad Sci U S A. 1987 May;84(10):3102–3106. doi: 10.1073/pnas.84.10.3102. [DOI] [PMC free article] [PubMed] [Google Scholar]
- HUXLEY A. F. Muscle structure and theories of contraction. Prog Biophys Biophys Chem. 1957;7:255–318. [PubMed] [Google Scholar]
- Hannon J. D., Chase P. B., Martyn D. A., Huntsman L. L., Kushmerick M. J., Gordon A. M. Calcium-independent activation of skeletal muscle fibers by a modified form of cardiac troponin C. Biophys J. 1993 May;64(5):1632–1637. doi: 10.1016/S0006-3495(93)81517-0. [DOI] [PMC free article] [PubMed] [Google Scholar]
- Hannon J. D., Martyn D. A., Gordon A. M. Effects of cycling and rigor crossbridges on the conformation of cardiac troponin C. Circ Res. 1992 Oct;71(4):984–991. doi: 10.1161/01.res.71.4.984. [DOI] [PubMed] [Google Scholar]
- Kress M., Huxley H. E., Faruqi A. R., Hendrix J. Structural changes during activation of frog muscle studied by time-resolved X-ray diffraction. J Mol Biol. 1986 Apr 5;188(3):325–342. doi: 10.1016/0022-2836(86)90158-0. [DOI] [PubMed] [Google Scholar]
- Lompré A. M., Nadal-Ginard B., Mahdavi V. Expression of the cardiac ventricular alpha- and beta-myosin heavy chain genes is developmentally and hormonally regulated. J Biol Chem. 1984 May 25;259(10):6437–6446. [PubMed] [Google Scholar]
- Martyn D. A., Gordon A. M. Length and myofilament spacing-dependent changes in calcium sensitivity of skeletal fibres: effects of pH and ionic strength. J Muscle Res Cell Motil. 1988 Oct;9(5):428–445. doi: 10.1007/BF01774069. [DOI] [PubMed] [Google Scholar]
- Matsubara I., Umazume Y., Yagi N. Lateral filamentary spacing in chemically skinned murine muscles during contraction. J Physiol. 1985 Mar;360:135–148. doi: 10.1113/jphysiol.1985.sp015608. [DOI] [PMC free article] [PubMed] [Google Scholar]
- Metzger J. M., Greaser M. L., Moss R. L. Variations in cross-bridge attachment rate and tension with phosphorylation of myosin in mammalian skinned skeletal muscle fibers. Implications for twitch potentiation in intact muscle. J Gen Physiol. 1989 May;93(5):855–883. doi: 10.1085/jgp.93.5.855. [DOI] [PMC free article] [PubMed] [Google Scholar]
- Metzger J. M., Moss R. L. Calcium-sensitive cross-bridge transitions in mammalian fast and slow skeletal muscle fibers. Science. 1990 Mar 2;247(4946):1088–1090. doi: 10.1126/science.2309121. [DOI] [PubMed] [Google Scholar]
- Metzger J. M., Moss R. L. Kinetics of a Ca(2+)-sensitive cross-bridge state transition in skeletal muscle fibers. Effects due to variations in thin filament activation by extraction of troponin C. J Gen Physiol. 1991 Aug;98(2):233–248. doi: 10.1085/jgp.98.2.233. [DOI] [PMC free article] [PubMed] [Google Scholar]
- Metzger J. M., Moss R. L. Myosin light chain 2 modulates calcium-sensitive cross-bridge transitions in vertebrate skeletal muscle. Biophys J. 1992 Aug;63(2):460–468. doi: 10.1016/S0006-3495(92)81614-4. [DOI] [PMC free article] [PubMed] [Google Scholar]
- Millar N. C., Homsher E. The effect of phosphate and calcium on force generation in glycerinated rabbit skeletal muscle fibers. A steady-state and transient kinetic study. J Biol Chem. 1990 Nov 25;265(33):20234–20240. [PubMed] [Google Scholar]
- Parmacek M. S., Leiden J. M. Structure and expression of the murine slow/cardiac troponin C gene. J Biol Chem. 1989 Aug 5;264(22):13217–13225. [PubMed] [Google Scholar]
- Podolsky R. J., Teichholz L. E. The relation between calcium and contraction kinetics in skinned muscle fibres. J Physiol. 1970 Nov;211(1):19–35. doi: 10.1113/jphysiol.1970.sp009263. [DOI] [PMC free article] [PubMed] [Google Scholar]
- Potter J. D. Preparation of troponin and its subunits. Methods Enzymol. 1982;85(Pt B):241–263. doi: 10.1016/0076-6879(82)85024-6. [DOI] [PubMed] [Google Scholar]
- Putkey J. A., Dotson D. G., Mouawad P. Formation of inter- and intramolecular disulfide bonds can activate cardiac troponin C. J Biol Chem. 1993 Apr 5;268(10):6827–6830. [PubMed] [Google Scholar]
- Robertson S. P., Johnson J. D., Holroyde M. J., Kranias E. G., Potter J. D., Solaro R. J. The effect of troponin I phosphorylation on the Ca2+-binding properties of the Ca2+-regulatory site of bovine cardiac troponin. J Biol Chem. 1982 Jan 10;257(1):260–263. [PubMed] [Google Scholar]
- Schreier T., Kedes L., Gahlmann R. Cloning, structural analysis, and expression of the human slow twitch skeletal muscle/cardiac troponin C gene. J Biol Chem. 1990 Dec 5;265(34):21247–21253. [PubMed] [Google Scholar]
- Swartz D. R., Moss R. L. Influence of a strong-binding myosin analogue on calcium-sensitive mechanical properties of skinned skeletal muscle fibers. J Biol Chem. 1992 Oct 5;267(28):20497–20506. [PubMed] [Google Scholar]
- Sweeney H. L., Corteselli S. A., Kushmerick M. J. Measurements on permeabilized skeletal muscle fibers during continuous activation. Am J Physiol. 1987 May;252(5 Pt 1):C575–C580. doi: 10.1152/ajpcell.1987.252.5.C575. [DOI] [PubMed] [Google Scholar]
- Sweeney H. L., Stull J. T. Alteration of cross-bridge kinetics by myosin light chain phosphorylation in rabbit skeletal muscle: implications for regulation of actin-myosin interaction. Proc Natl Acad Sci U S A. 1990 Jan;87(1):414–418. doi: 10.1073/pnas.87.1.414. [DOI] [PMC free article] [PubMed] [Google Scholar]
- Walker J. W., Lu Z., Moss R. L. Effects of Ca2+ on the kinetics of phosphate release in skeletal muscle. J Biol Chem. 1992 Feb 5;267(4):2459–2466. [PubMed] [Google Scholar]
- Wilkinson J. M. Troponin C from rabbit slow skeletal and cardiac muscle is the product of a single gene. Eur J Biochem. 1980 Jan;103(1):179–188. doi: 10.1111/j.1432-1033.1980.tb04302.x. [DOI] [PubMed] [Google Scholar]