Abstract
To clarify the extensibility of thin actin and thick myosin filaments in muscle, we examined the spacings of actin and myosin filament-based reflections in x-ray diffraction patterns at high resolution during isometric contraction of frog skeletal muscles and steady lengthening of the active muscles using synchrotron radiation as an intense x-ray source and a storage phosphor plate as a high sensitivity, high resolution area detector. Spacing of the actin meridional reflection at approximately 1/2.7 nm-1, which corresponds to the axial rise per actin subunit in the thin filament, increased about 0.25% during isometric contraction of muscles at full overlap length of thick and thin filaments. The changes in muscles stretched to approximately half overlap of the filaments, when they were scaled linearly up to the full isometric tension, gave an increase of approximately 0.3%. Conversely, the spacing decreased by approximately 0.1% upon activation of muscles at nonoverlap length. Slow stretching of a contracting muscle increased tension and increased this spacing over the isometric contraction value. Scaled up to a 100% tension increase, this corresponds to a approximately 0.26% additional change, consistent with that of the initial isometric contraction. Taken together, the extensibility of the actin filament amounts to 3-4 nm of elongation when a muscle switches from relaxation to maximum isometric contraction. Axial spacings of the layer-line reflections at approximately 1/5.1 nm-1 and approximately 1/5.9 nm-1 corresponding to the pitches of the right- and left-handed genetic helices of the actin filament, showed similar changes to that of the meridional reflection during isometric contraction of muscles at full overlap. The spacing changes of these reflections, which also depend on the mechanical load on the muscle, indicate that elongation is accompanied by slight changes of the actin helical structure possibly because of the axial force exerted by the actomyosin cross-bridges. Additional small spacing changes of the myosin meridional reflections during length changes applied to contracting muscles represented an increase of approximately 0.26% (scaled up to a 100% tension increase) in the myosin periodicity, suggesting that such spacing changes correspond to a tension-related extension of the myosin filaments. Elongation of the myosin filament backbone amounts to approximately 2.1 nm per half sarcomere. The results indicate that a large part (approximately 70%) of the sarcomere compliance of an active muscle is caused by the extensibility of the actin and myosin filaments; 42% of the compliance resides in the actin filaments, and 27% of it is in the myosin filaments.
Full text
PDF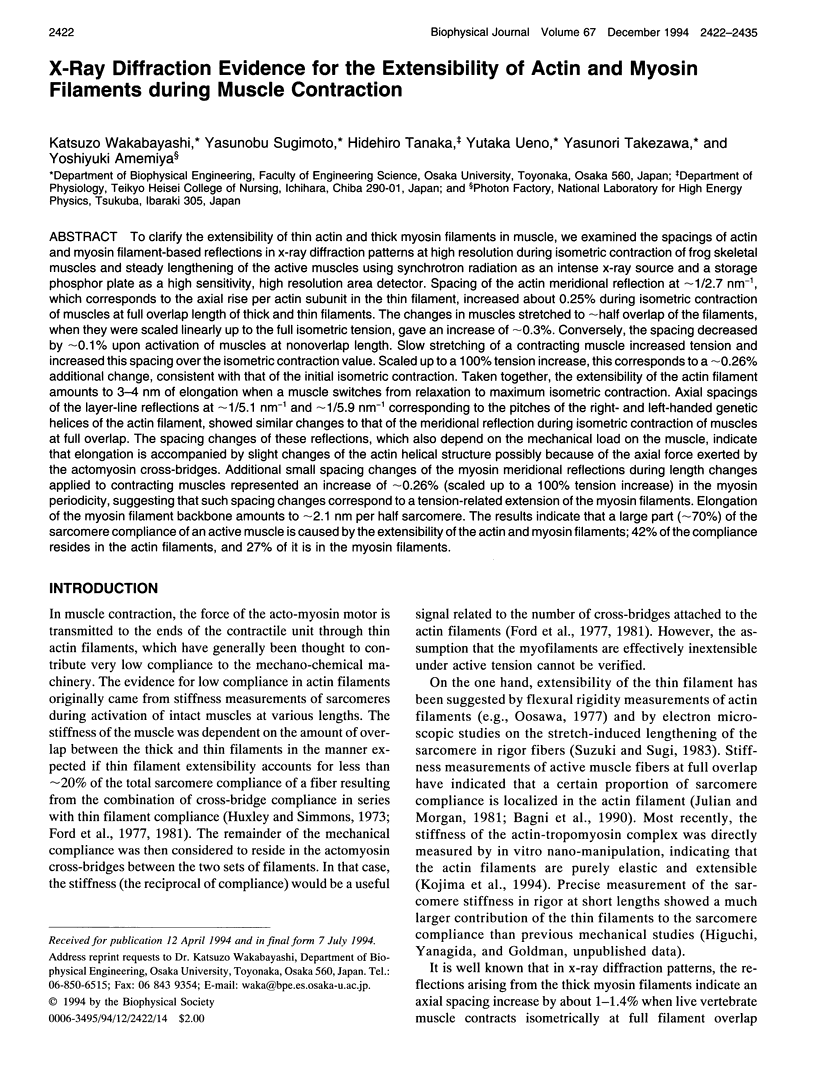
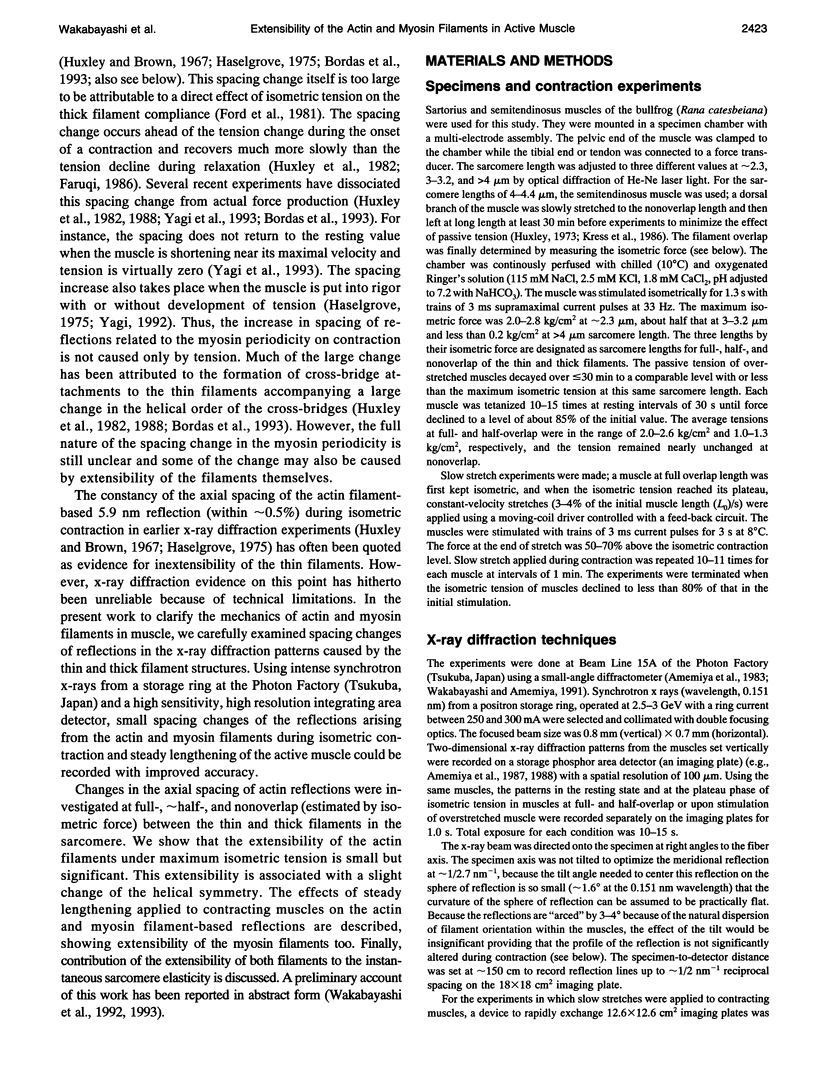
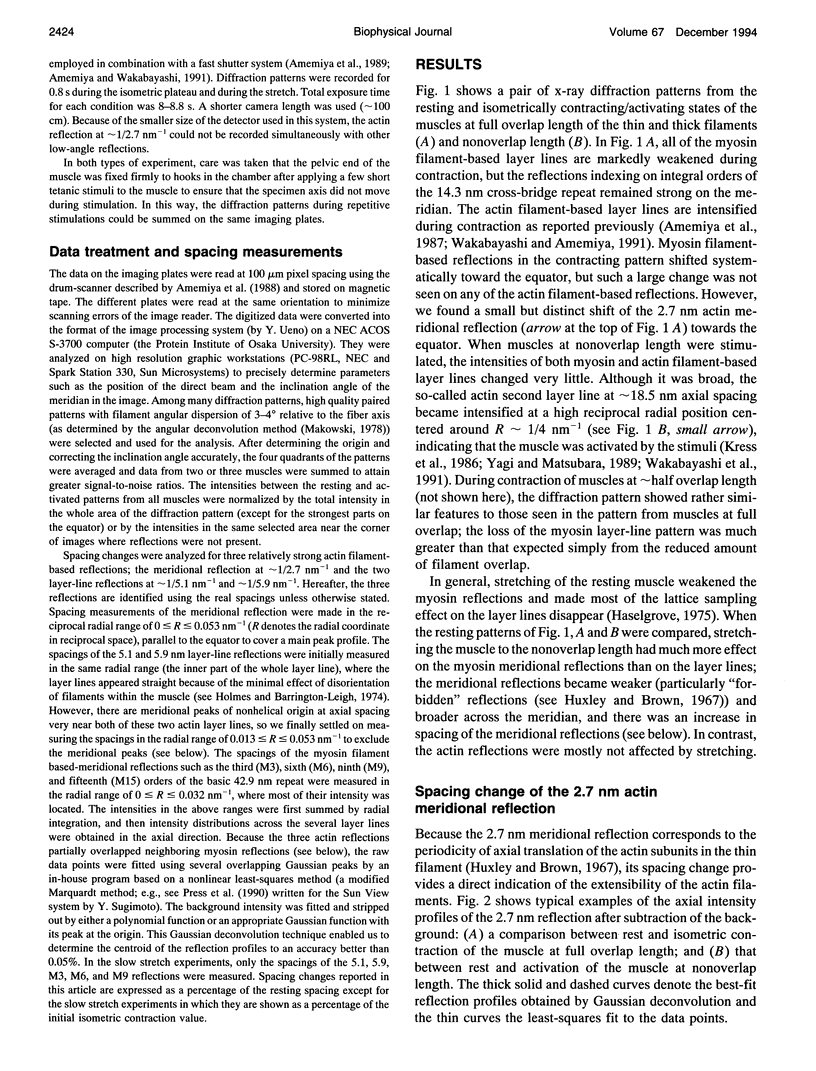
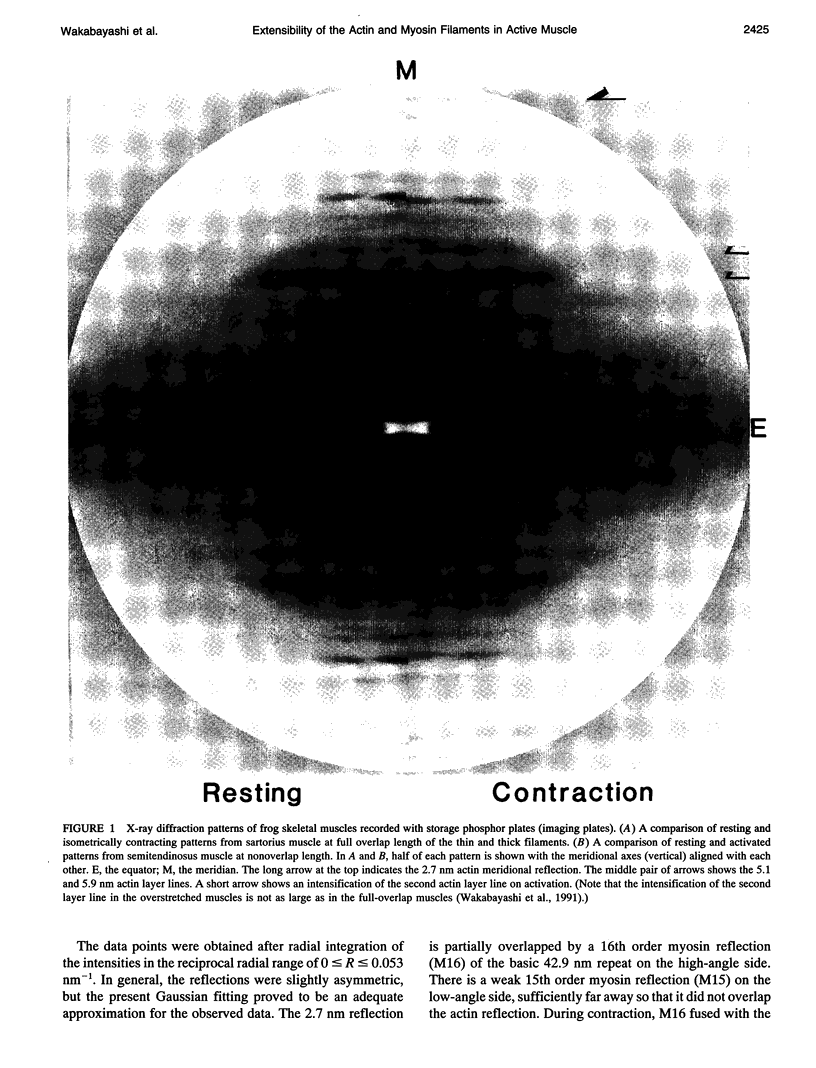
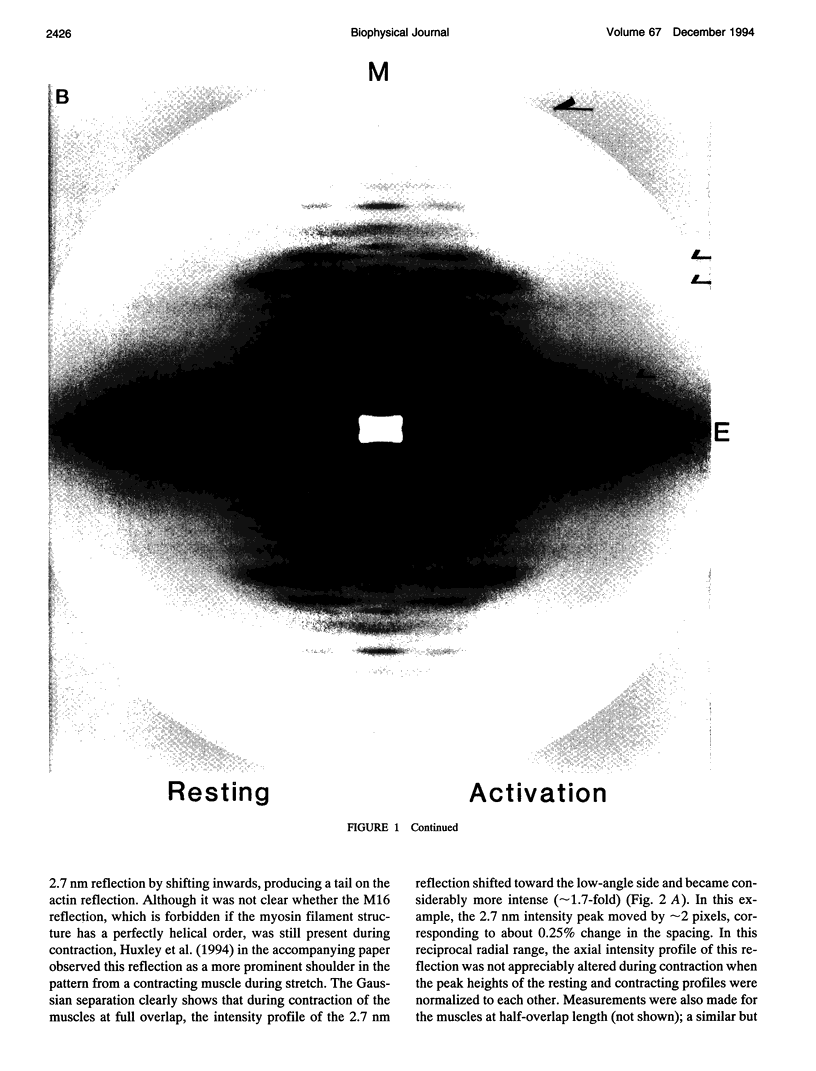
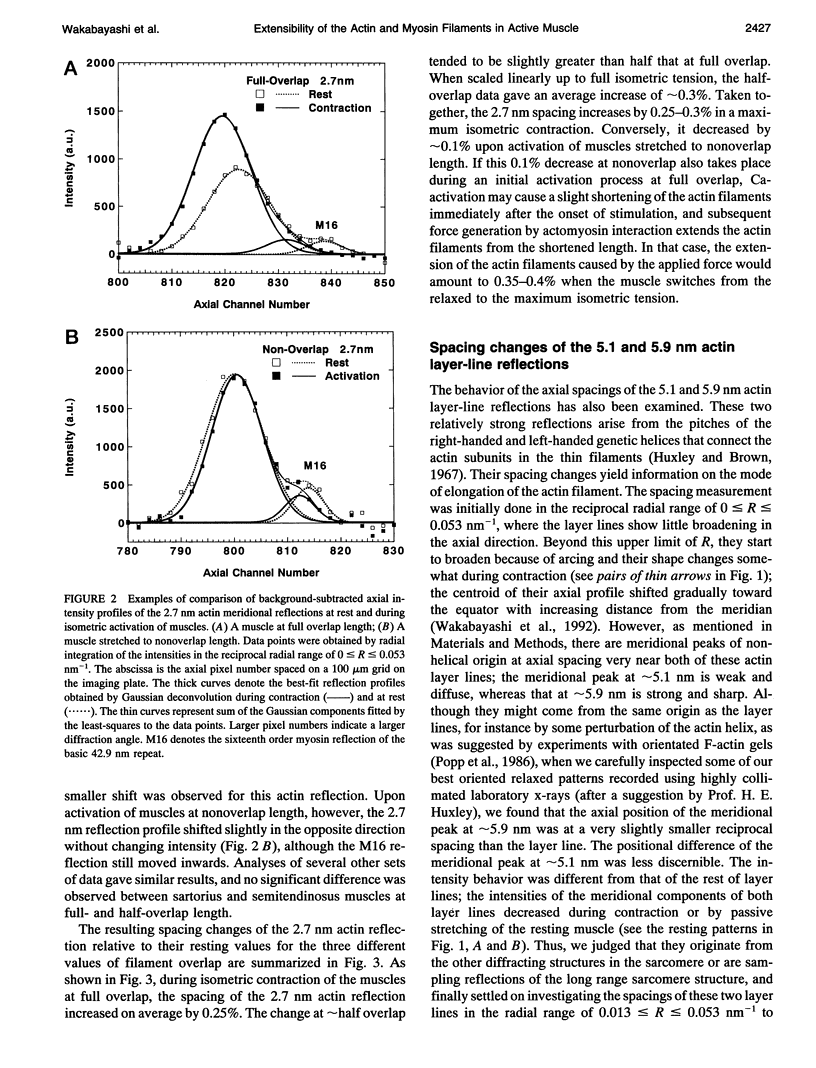
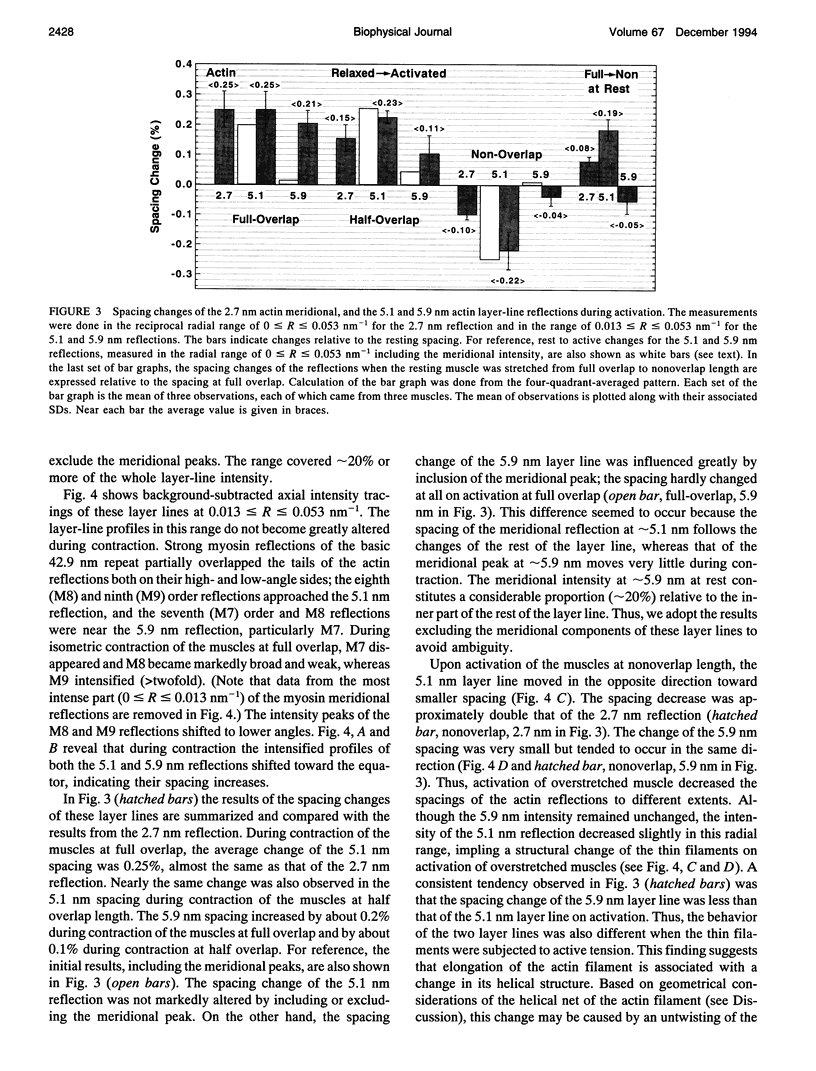
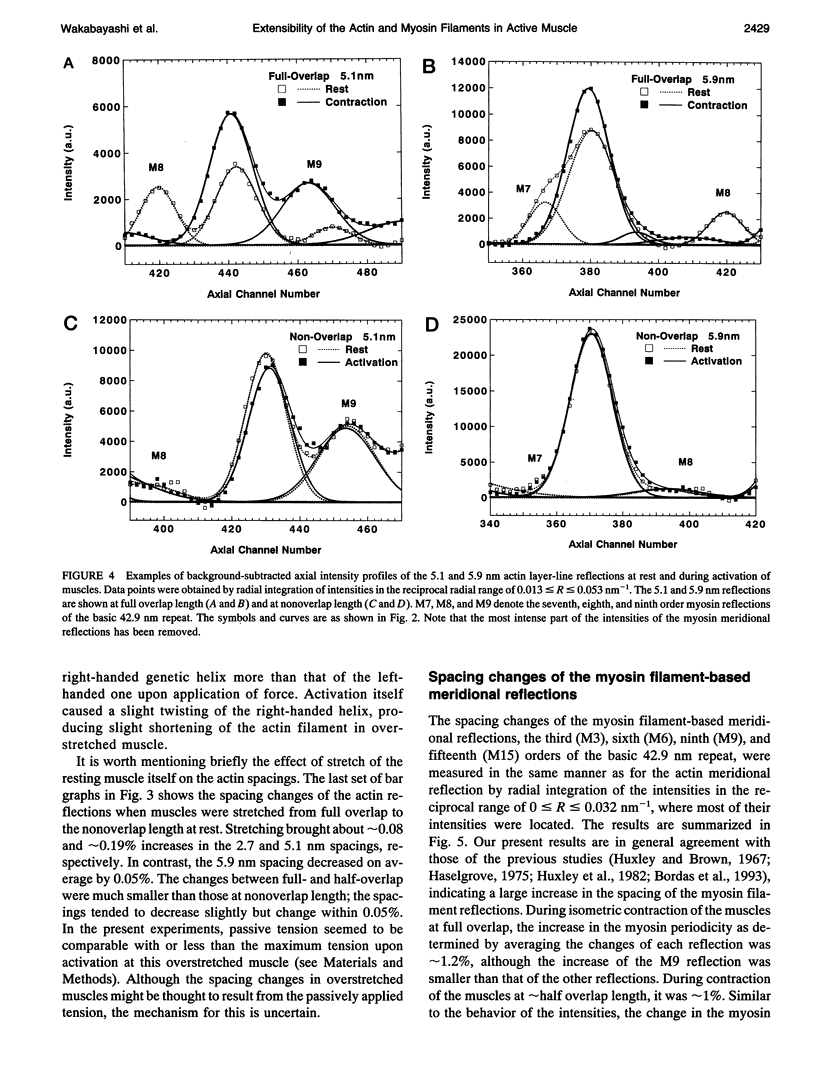
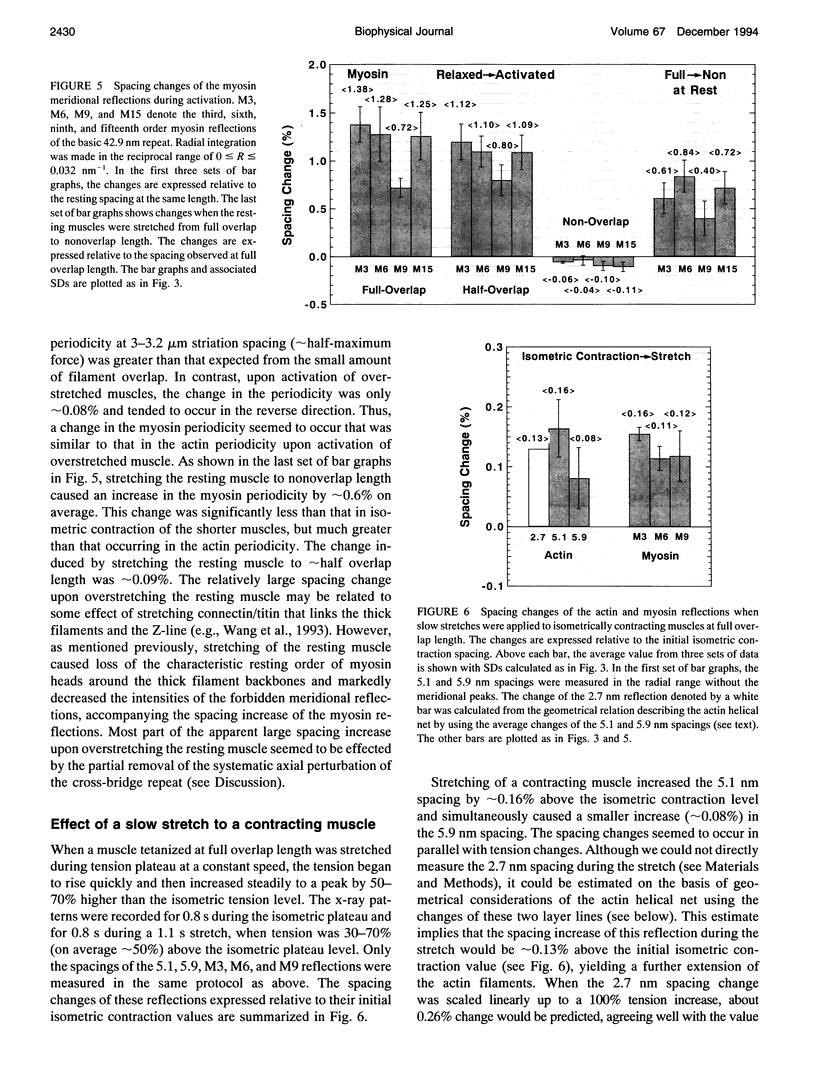
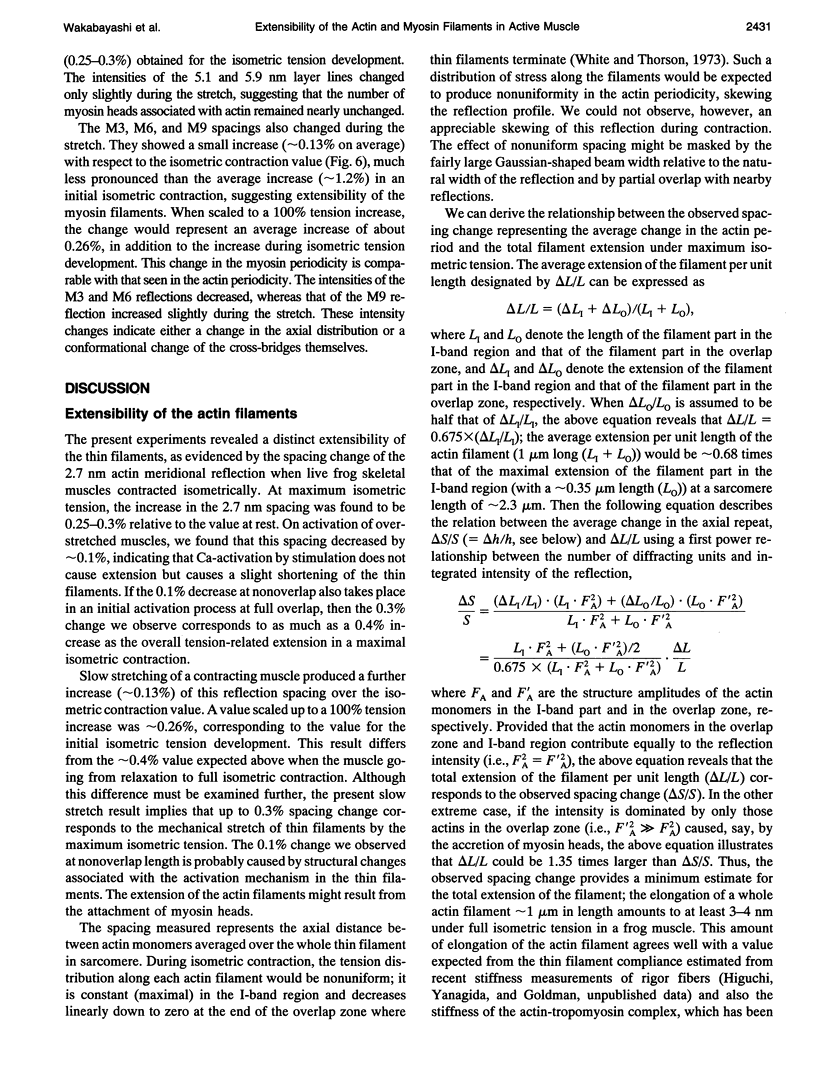
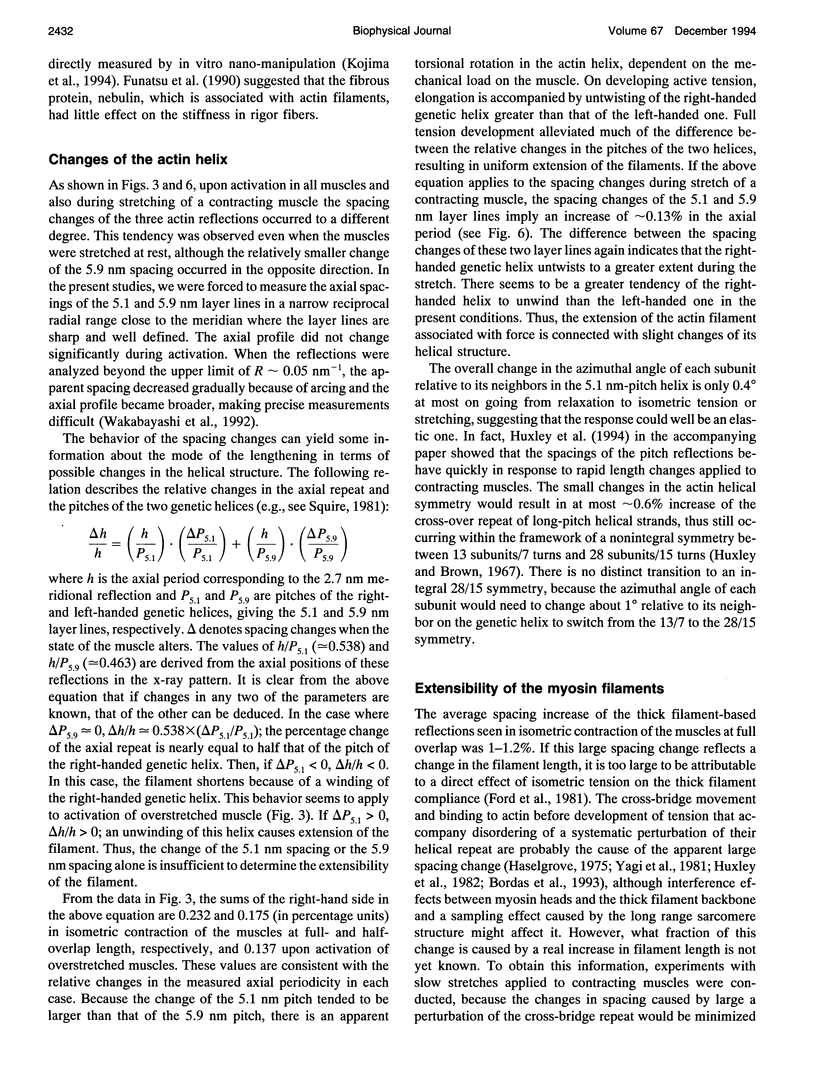
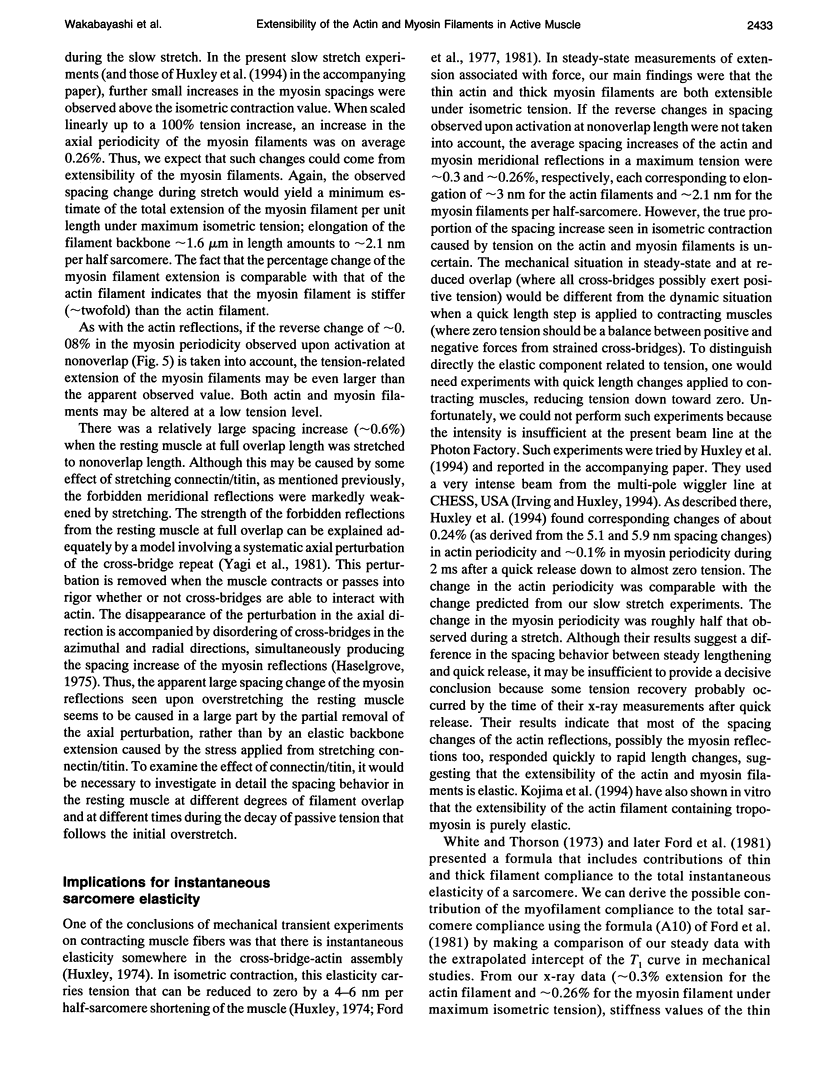
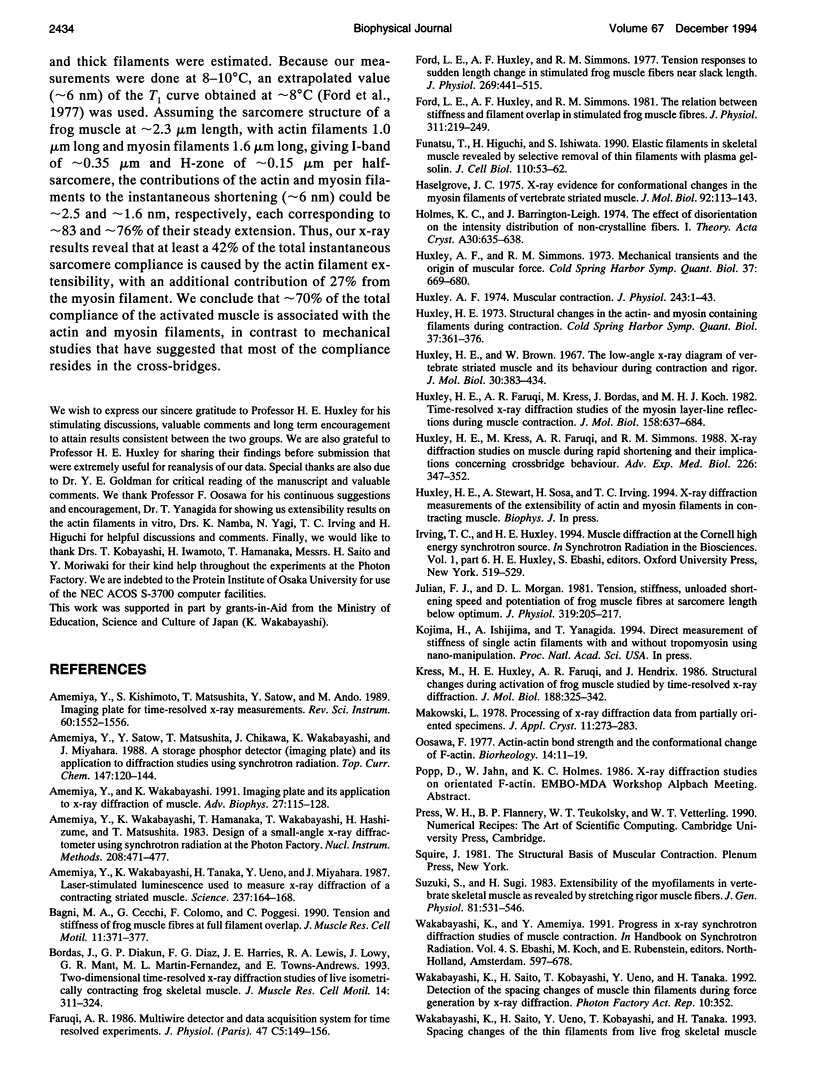
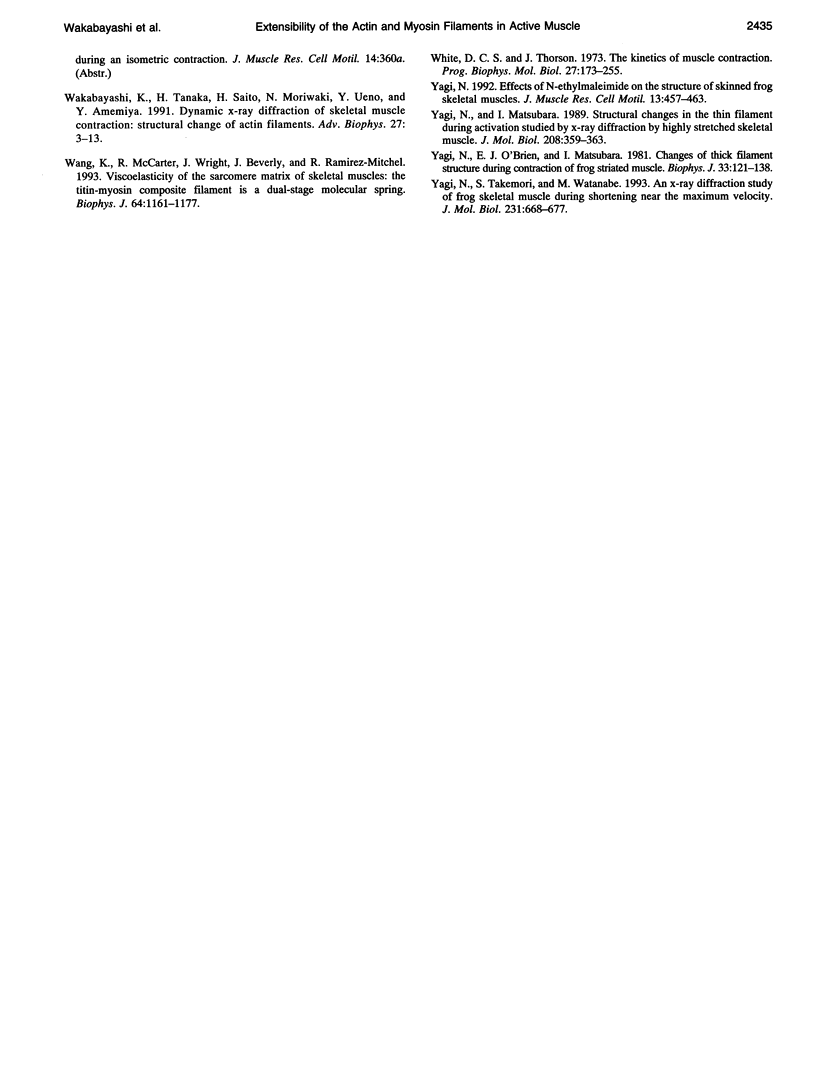
Images in this article
Selected References
These references are in PubMed. This may not be the complete list of references from this article.
- Amemiya Y., Wakabayashi K. Imaging plate and its application to X-ray diffraction of muscle. Adv Biophys. 1991;27:115–126. doi: 10.1016/0065-227x(91)90012-3. [DOI] [PubMed] [Google Scholar]
- Amemiya Y., Wakabayashi K., Tanaka H., Ueno Y., Miyahara J. Laser-stimulated luminescence used to measure x-ray diffraction of a contracting striated muscle. Science. 1987 Jul 10;237(4811):164–168. doi: 10.1126/science.3496662. [DOI] [PubMed] [Google Scholar]
- Bagni M. A., Cecchi G., Colomo F., Poggesi C. Tension and stiffness of frog muscle fibres at full filament overlap. J Muscle Res Cell Motil. 1990 Oct;11(5):371–377. doi: 10.1007/BF01739758. [DOI] [PubMed] [Google Scholar]
- Bordas J., Diakun G. P., Diaz F. G., Harries J. E., Lewis R. A., Lowy J., Mant G. R., Martin-Fernandez M. L., Towns-Andrews E. Two-dimensional time-resolved X-ray diffraction studies of live isometrically contracting frog sartorius muscle. J Muscle Res Cell Motil. 1993 Jun;14(3):311–324. doi: 10.1007/BF00123096. [DOI] [PubMed] [Google Scholar]
- Ford L. E., Huxley A. F., Simmons R. M. Tension responses to sudden length change in stimulated frog muscle fibres near slack length. J Physiol. 1977 Jul;269(2):441–515. doi: 10.1113/jphysiol.1977.sp011911. [DOI] [PMC free article] [PubMed] [Google Scholar]
- Ford L. E., Huxley A. F., Simmons R. M. The relation between stiffness and filament overlap in stimulated frog muscle fibres. J Physiol. 1981 Feb;311:219–249. doi: 10.1113/jphysiol.1981.sp013582. [DOI] [PMC free article] [PubMed] [Google Scholar]
- Funatsu T., Higuchi H., Ishiwata S. Elastic filaments in skeletal muscle revealed by selective removal of thin filaments with plasma gelsolin. J Cell Biol. 1990 Jan;110(1):53–62. doi: 10.1083/jcb.110.1.53. [DOI] [PMC free article] [PubMed] [Google Scholar]
- Haselgrove J. C. X-ray evidence for conformational changes in the myosin filaments of vertebrate striated muscle. J Mol Biol. 1975 Feb 15;92(1):113–143. doi: 10.1016/0022-2836(75)90094-7. [DOI] [PubMed] [Google Scholar]
- Huxley A. F. Muscular contraction. J Physiol. 1974 Nov;243(1):1–43. [PMC free article] [PubMed] [Google Scholar]
- Huxley H. E., Brown W. The low-angle x-ray diagram of vertebrate striated muscle and its behaviour during contraction and rigor. J Mol Biol. 1967 Dec 14;30(2):383–434. doi: 10.1016/s0022-2836(67)80046-9. [DOI] [PubMed] [Google Scholar]
- Huxley H. E., Faruqi A. R., Kress M., Bordas J., Koch M. H. Time-resolved X-ray diffraction studies of the myosin layer-line reflections during muscle contraction. J Mol Biol. 1982 Jul 15;158(4):637–684. doi: 10.1016/0022-2836(82)90253-4. [DOI] [PubMed] [Google Scholar]
- Huxley H. E., Kress M., Faruqi A. F., Simmons R. M. X-ray diffraction studies on muscle during rapid shortening and their implications concerning crossbridge behaviour. Adv Exp Med Biol. 1988;226:347–352. [PubMed] [Google Scholar]
- Julian F. J., Morgan D. L. Tension, stiffness, unloaded shortening speed and potentiation of frog muscle fibres at sarcomere lengths below optimum. J Physiol. 1981;319:205–217. doi: 10.1113/jphysiol.1981.sp013902. [DOI] [PMC free article] [PubMed] [Google Scholar]
- Kress M., Huxley H. E., Faruqi A. R., Hendrix J. Structural changes during activation of frog muscle studied by time-resolved X-ray diffraction. J Mol Biol. 1986 Apr 5;188(3):325–342. doi: 10.1016/0022-2836(86)90158-0. [DOI] [PubMed] [Google Scholar]
- Oosawa F. Actin-actin bond strength and the conformational change of F-actin. Biorheology. 1977;14(1):11–19. doi: 10.3233/bir-1977-14102. [DOI] [PubMed] [Google Scholar]
- Suzuki S., Sugi H. Extensibility of the myofilaments in vertebrate skeletal muscle as revealed by stretching rigor muscle fibers. J Gen Physiol. 1983 Apr;81(4):531–546. doi: 10.1085/jgp.81.4.531. [DOI] [PMC free article] [PubMed] [Google Scholar]
- Wakabayashi K., Tanaka H., Saito H., Moriwaki N., Ueno Y., Amemiya Y. Dynamic X-ray diffraction of skeletal muscle contraction: structural change of actin filaments. Adv Biophys. 1991;27:3–13. doi: 10.1016/0065-227x(91)90004-w. [DOI] [PubMed] [Google Scholar]
- Wang K., McCarter R., Wright J., Beverly J., Ramirez-Mitchell R. Viscoelasticity of the sarcomere matrix of skeletal muscles. The titin-myosin composite filament is a dual-stage molecular spring. Biophys J. 1993 Apr;64(4):1161–1177. doi: 10.1016/S0006-3495(93)81482-6. [DOI] [PMC free article] [PubMed] [Google Scholar]
- Yagi N. Effects of N-ethylmaleimide on the structure of skinned frog skeletal muscles. J Muscle Res Cell Motil. 1992 Aug;13(4):457–463. doi: 10.1007/BF01738040. [DOI] [PubMed] [Google Scholar]
- Yagi N., Matsubara I. Structural changes in the thin filament during activation studied by X-ray diffraction of highly stretched skeletal muscle. J Mol Biol. 1989 Jul 20;208(2):359–363. doi: 10.1016/0022-2836(89)90396-3. [DOI] [PubMed] [Google Scholar]
- Yagi N., O'Brien E. J., Matsubara I. Changes of thick filament structure during contraction of frog striated muscle. Biophys J. 1981 Jan;33(1):121–137. doi: 10.1016/S0006-3495(81)84876-X. [DOI] [PMC free article] [PubMed] [Google Scholar]
- Yagi N., Takemori S., Watanabe M. An X-ray diffraction study of frog skeletal muscle during shortening near the maximum velocity. J Mol Biol. 1993 Jun 5;231(3):668–677. doi: 10.1006/jmbi.1993.1318. [DOI] [PubMed] [Google Scholar]