Abstract
With few exceptions, membrane lipids are usually regarded as a kind of filler or passive solvent for membrane proteins. Yet, cells exquisitely control membrane composition. Many phospholipids found in plasma membrane bilayers favor packing into inverted hexagonal bulk phases. It was suggested that the strain of forcing such lipids into a bilayer may affect membrane protein function, such as the operation of transmembrane channels. To investigate this, we have inserted the peptide alamethicin into bilayer membranes composed of lipids of empirically determined inverted hexagonal phase "spontaneous radii" Ro, which will have expectably different degrees of strain when forced into bilayer form. We observe a correlation between measured Ro and the relative probabilities of different conductance states. States of higher conductance are more probable in dioleoylphosphatidylethanolamine, the lipid of highest curvature, 1/Ro, than in dioleoylphosphatidylcholine, the lipid of lowest curvature.
Full text
PDF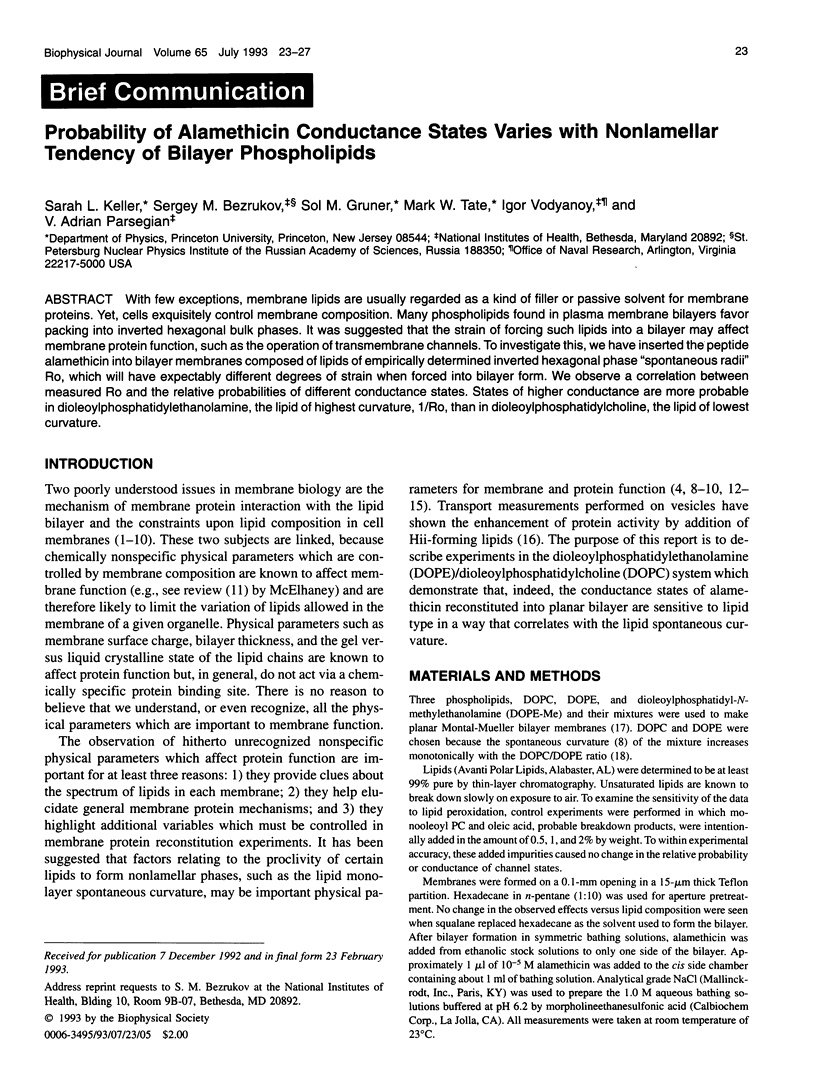
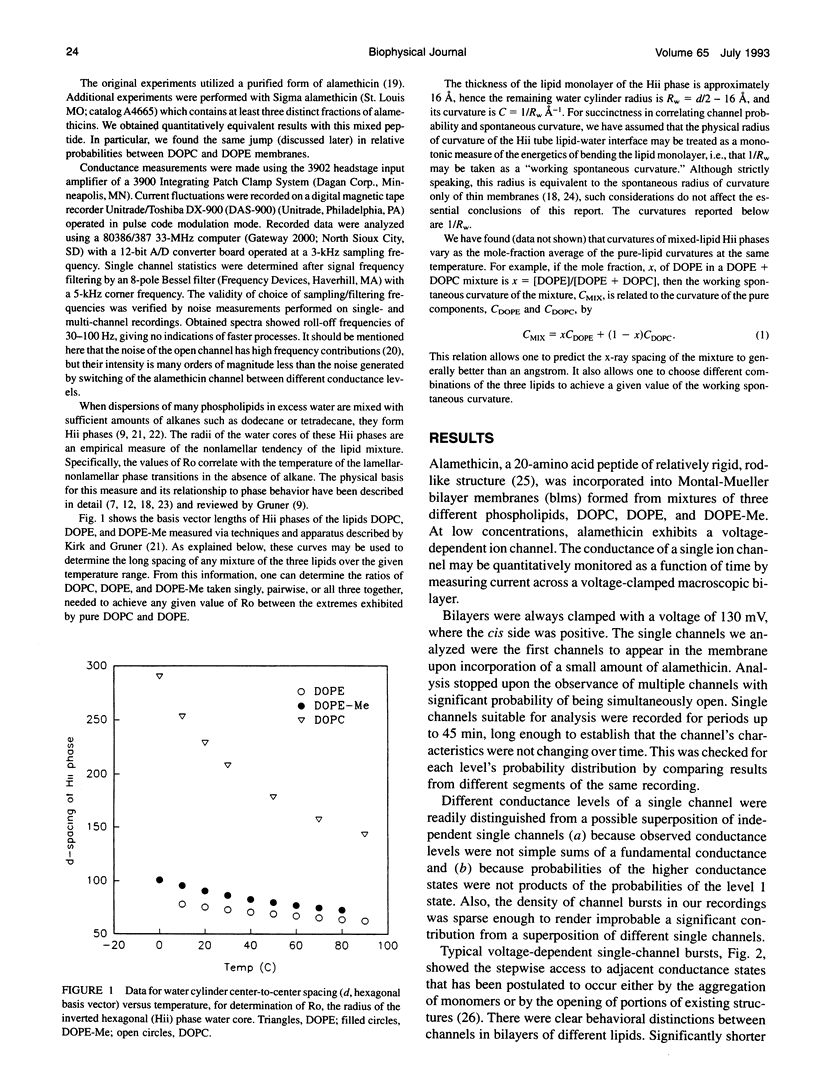
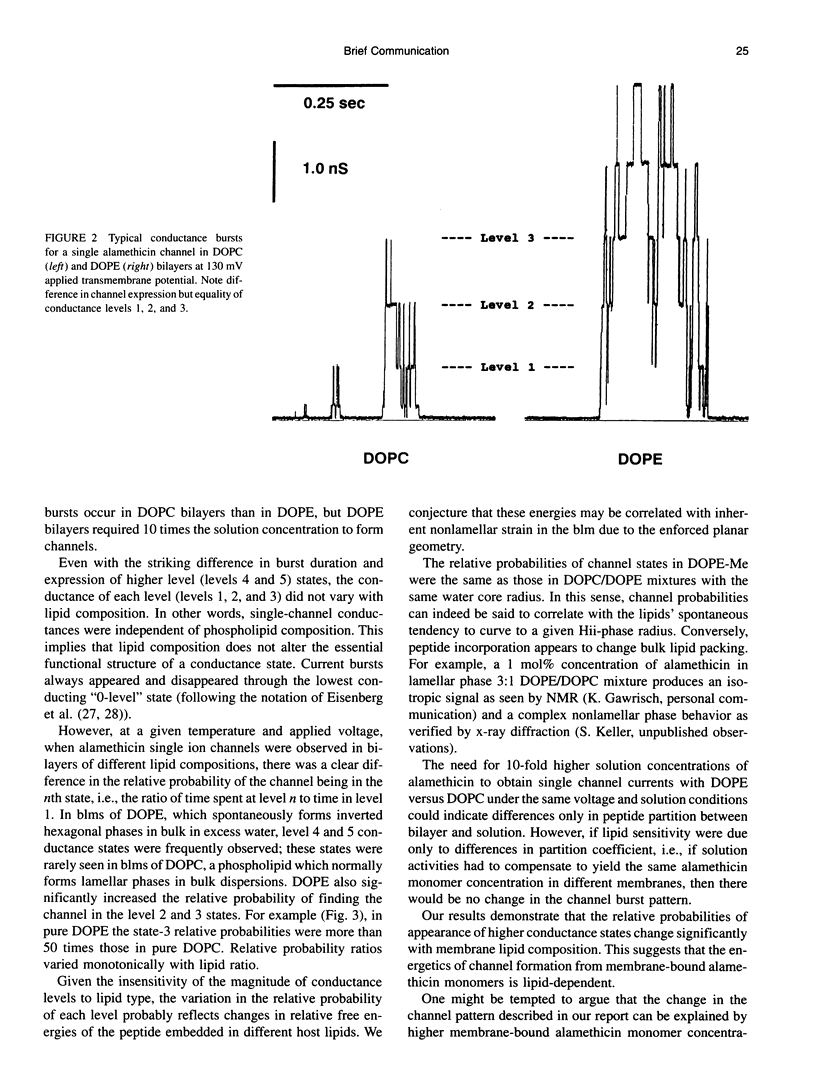
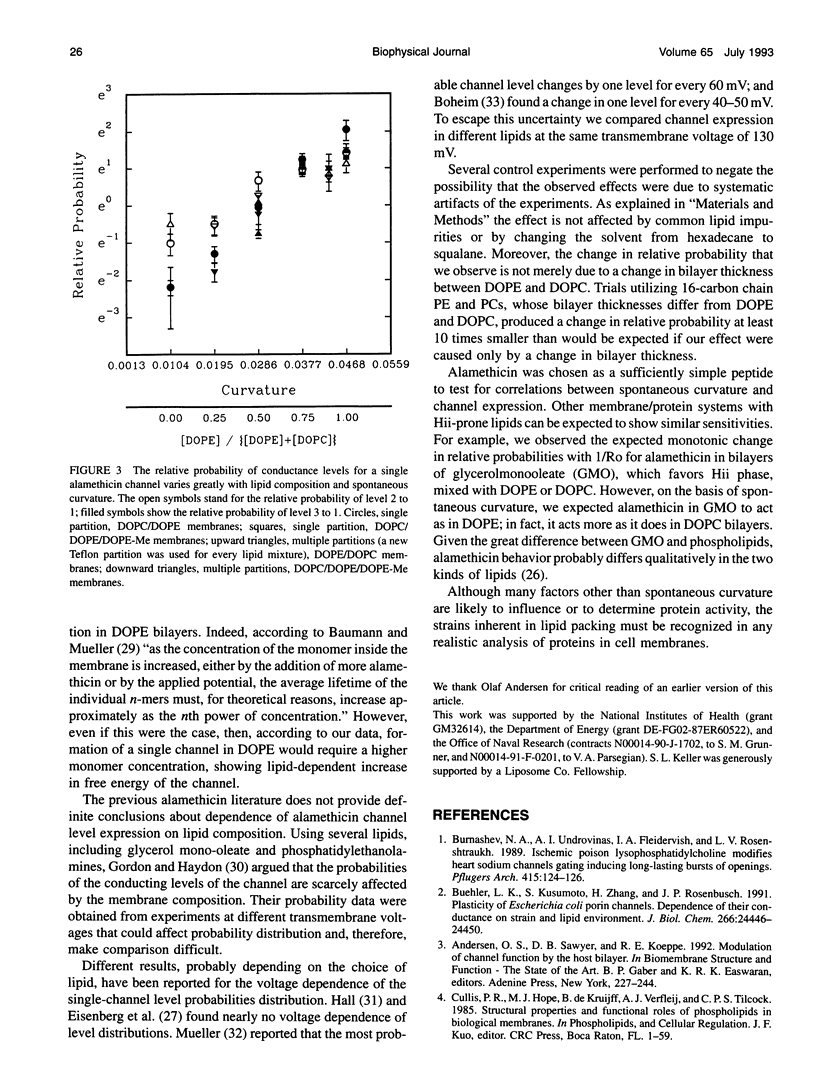
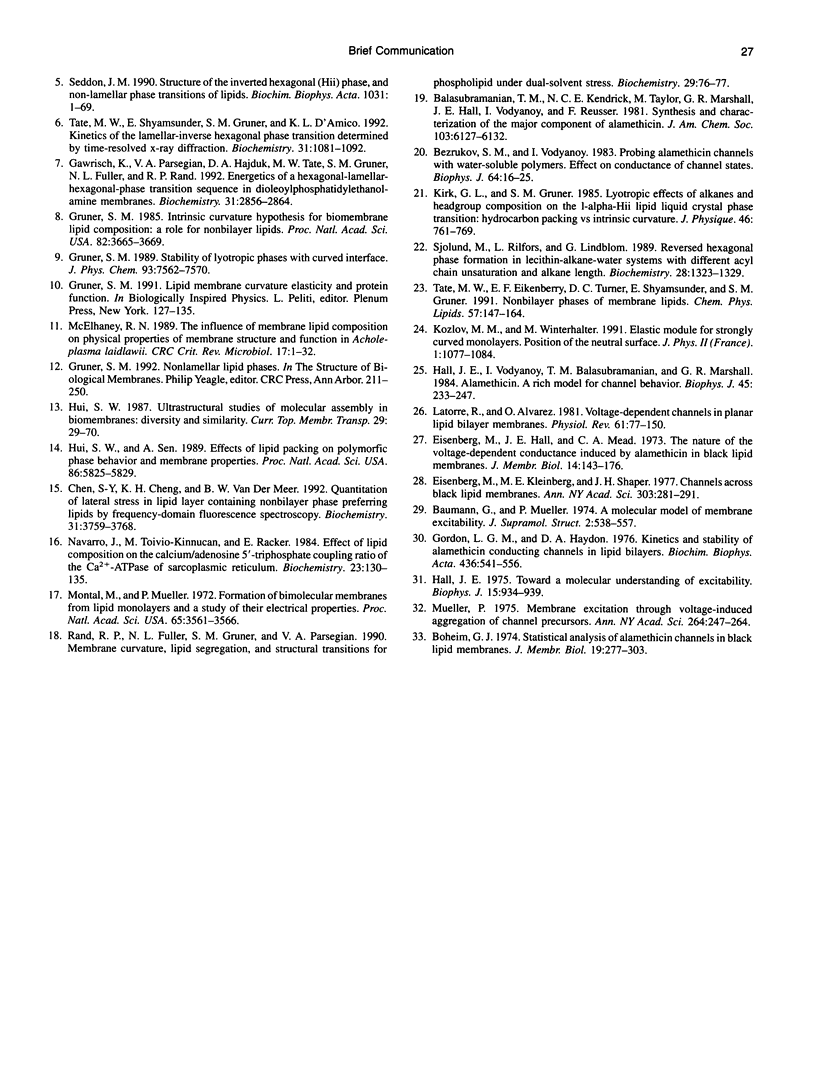
Selected References
These references are in PubMed. This may not be the complete list of references from this article.
- Baumann G., Mueller P. A molecular model of membrane excitability. J Supramol Struct. 1974;2(5-6):538–557. doi: 10.1002/jss.400020504. [DOI] [PubMed] [Google Scholar]
- Bezrukov S. M., Vodyanoy I. Probing alamethicin channels with water-soluble polymers. Effect on conductance of channel states. Biophys J. 1993 Jan;64(1):16–25. doi: 10.1016/S0006-3495(93)81336-5. [DOI] [PMC free article] [PubMed] [Google Scholar]
- Boheim G. Statistical analysis of alamethicin channels in black lipid membranes. J Membr Biol. 1974;19(3):277–303. doi: 10.1007/BF01869983. [DOI] [PubMed] [Google Scholar]
- Buehler L. K., Kusumoto S., Zhang H., Rosenbusch J. P. Plasticity of Escherichia coli porin channels. Dependence of their conductance on strain and lipid environment. J Biol Chem. 1991 Dec 25;266(36):24446–24450. [PubMed] [Google Scholar]
- Burnashev N. A., Undrovinas A. I., Fleidervish I. A., Rosenshtraukh L. V. Ischemic poison lysophosphatidylcholine modifies heart sodium channels gating inducing long-lasting bursts of openings. Pflugers Arch. 1989 Oct;415(1):124–126. doi: 10.1007/BF00373151. [DOI] [PubMed] [Google Scholar]
- Chen S. Y., Cheng K. H., Van der Meer B. W. Quantitation of lateral stress in lipid layer containing nonbilayer phase preferring lipids by frequency-domain fluorescence spectroscopy. Biochemistry. 1992 Apr 21;31(15):3759–3768. doi: 10.1021/bi00130a005. [DOI] [PubMed] [Google Scholar]
- Eisenberg M., Hall J. E., Mead C. A. The nature of the voltage-dependent conductance induced by alamethicin in black lipid membranes. J Membr Biol. 1973 Dec 31;14(2):143–176. doi: 10.1007/BF01868075. [DOI] [PubMed] [Google Scholar]
- Eisenberg M., Kleinberg M. E., Shaper J. H. Channels across black lipid membranes. Ann N Y Acad Sci. 1977 Dec 30;303:281–294. [PubMed] [Google Scholar]
- Gawrisch K., Parsegian V. A., Hajduk D. A., Tate M. W., Graner S. M., Fuller N. L., Rand R. P. Energetics of a hexagonal-lamellar-hexagonal-phase transition sequence in dioleoylphosphatidylethanolamine membranes. Biochemistry. 1992 Mar 24;31(11):2856–2864. doi: 10.1021/bi00126a003. [DOI] [PubMed] [Google Scholar]
- Gordon L. G., Haydon D. A. Kinetics and stability of alamethicin conducting channels in lipid bilayers. Biochim Biophys Acta. 1976 Jul 1;436(3):541–556. doi: 10.1016/0005-2736(76)90439-9. [DOI] [PubMed] [Google Scholar]
- Gruner S. M. Intrinsic curvature hypothesis for biomembrane lipid composition: a role for nonbilayer lipids. Proc Natl Acad Sci U S A. 1985 Jun;82(11):3665–3669. doi: 10.1073/pnas.82.11.3665. [DOI] [PMC free article] [PubMed] [Google Scholar]
- Hall J. E. Toward a molecular understanding of excitability. Alamethicin in black lipid films. Biophys J. 1975 Sep;15(9):934–939. doi: 10.1016/S0006-3495(75)85869-3. [DOI] [PMC free article] [PubMed] [Google Scholar]
- Hall J. E., Vodyanoy I., Balasubramanian T. M., Marshall G. R. Alamethicin. A rich model for channel behavior. Biophys J. 1984 Jan;45(1):233–247. doi: 10.1016/S0006-3495(84)84151-X. [DOI] [PMC free article] [PubMed] [Google Scholar]
- Hui S. W., Sen A. Effects of lipid packing on polymorphic phase behavior and membrane properties. Proc Natl Acad Sci U S A. 1989 Aug;86(15):5825–5829. doi: 10.1073/pnas.86.15.5825. [DOI] [PMC free article] [PubMed] [Google Scholar]
- Latorre R., Alvarez O. Voltage-dependent channels in planar lipid bilayer membranes. Physiol Rev. 1981 Jan;61(1):77–150. doi: 10.1152/physrev.1981.61.1.77. [DOI] [PubMed] [Google Scholar]
- McElhaney R. N. The influence of membrane lipid composition and physical properties of membrane structure and function in Acholeplasma laidlawii. Crit Rev Microbiol. 1989;17(1):1–32. doi: 10.3109/10408418909105720. [DOI] [PubMed] [Google Scholar]
- Montal M., Mueller P. Formation of bimolecular membranes from lipid monolayers and a study of their electrical properties. Proc Natl Acad Sci U S A. 1972 Dec;69(12):3561–3566. doi: 10.1073/pnas.69.12.3561. [DOI] [PMC free article] [PubMed] [Google Scholar]
- Mueller P. Membrane excitation through voltage-induced aggregation of channel precursors. Ann N Y Acad Sci. 1975 Dec 30;264:247–264. doi: 10.1111/j.1749-6632.1975.tb31487.x. [DOI] [PubMed] [Google Scholar]
- Navarro J., Toivio-Kinnucan M., Racker E. Effect of lipid composition on the calcium/adenosine 5'-triphosphate coupling ratio of the Ca2+-ATPase of sarcoplasmic reticulum. Biochemistry. 1984 Jan 3;23(1):130–135. doi: 10.1021/bi00296a021. [DOI] [PubMed] [Google Scholar]
- Rand R. P., Fuller N. L., Gruner S. M., Parsegian V. A. Membrane curvature, lipid segregation, and structural transitions for phospholipids under dual-solvent stress. Biochemistry. 1990 Jan 9;29(1):76–87. doi: 10.1021/bi00453a010. [DOI] [PubMed] [Google Scholar]
- Seddon J. M. Structure of the inverted hexagonal (HII) phase, and non-lamellar phase transitions of lipids. Biochim Biophys Acta. 1990 Feb 28;1031(1):1–69. doi: 10.1016/0304-4157(90)90002-t. [DOI] [PubMed] [Google Scholar]
- Sjölund M., Rilfors L., Lindblom G. Reversed hexagonal phase formation in lecithin-alkane-water systems with different acyl chain unsaturation and alkane length. Biochemistry. 1989 Feb 7;28(3):1323–1329. doi: 10.1021/bi00429a057. [DOI] [PubMed] [Google Scholar]
- Tate M. W., Eikenberry E. F., Turner D. C., Shyamsunder E., Gruner S. M. Nonbilayer phases of membrane lipids. Chem Phys Lipids. 1991 Mar;57(2-3):147–164. doi: 10.1016/0009-3084(91)90073-k. [DOI] [PubMed] [Google Scholar]
- Tate M. W., Shyamsunder E., Gruner S. M., D'Amico K. L. Kinetics of the lamellar-inverse hexagonal phase transition determined by time-resolved X-ray diffraction. Biochemistry. 1992 Feb 4;31(4):1081–1092. doi: 10.1021/bi00119a017. [DOI] [PubMed] [Google Scholar]