Abstract
Ions crossing biological membranes are described as a concentration of charge flowing through a selective open channel of one conformation and analyzed by a combination of Poisson and Nernst-Planck equations and boundary conditions, called the PNP theory for short. The ion fluxes in this theory interact much as ion fluxes interact in biological channels and mediated transporters, provided the theoretical channel contains permanent charge and has selectivity created by (electro-chemical) resistance at its ends. Interaction occurs because the flux of different ionic species depends on the same electric field. That electric field is a variable, changing with experimental conditions because the screening (i.e., shielding) of the permanent charge within the channel changes with experimental conditions. For example, the screening of charge and the shape of the electric field depend on the concentration of all ionic species on both sides of the channel. As experimental interventions vary the screening, the electric field varies, and thus the flux of each ionic species varies conjointly, and is, in that sense, coupled. Interdependence and interaction are the rule, independence is the exception, in this channel.
Full text
PDF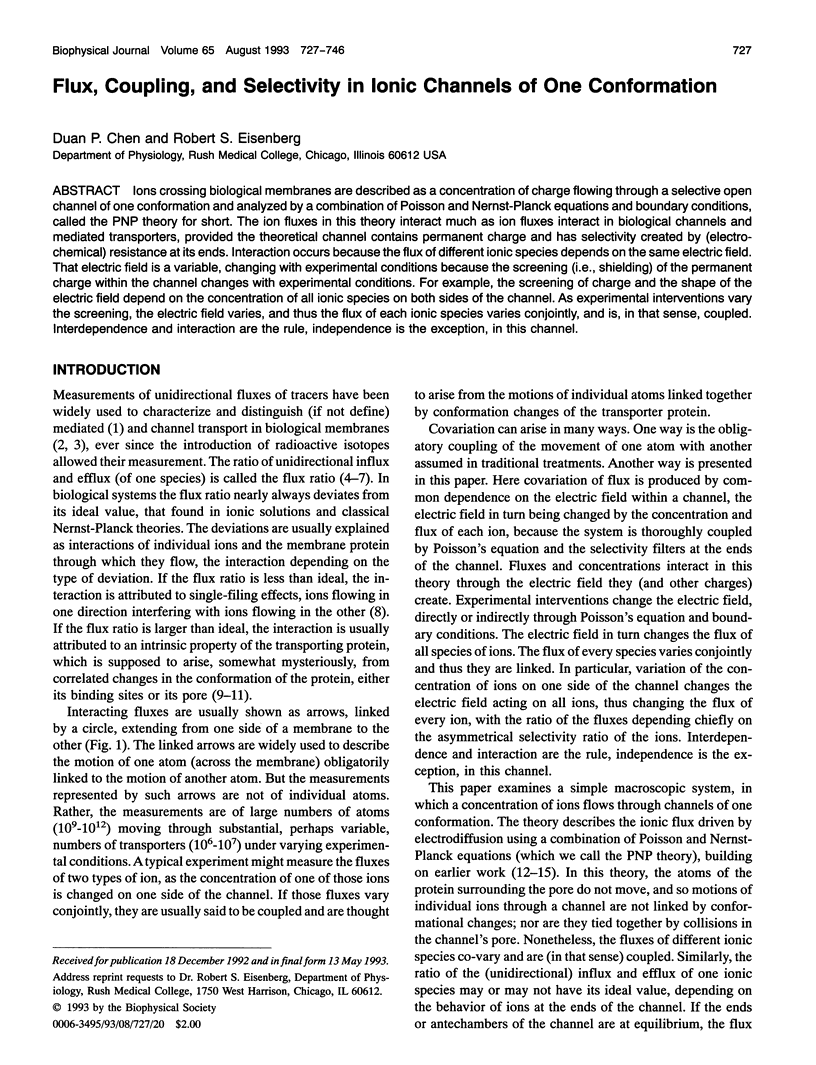
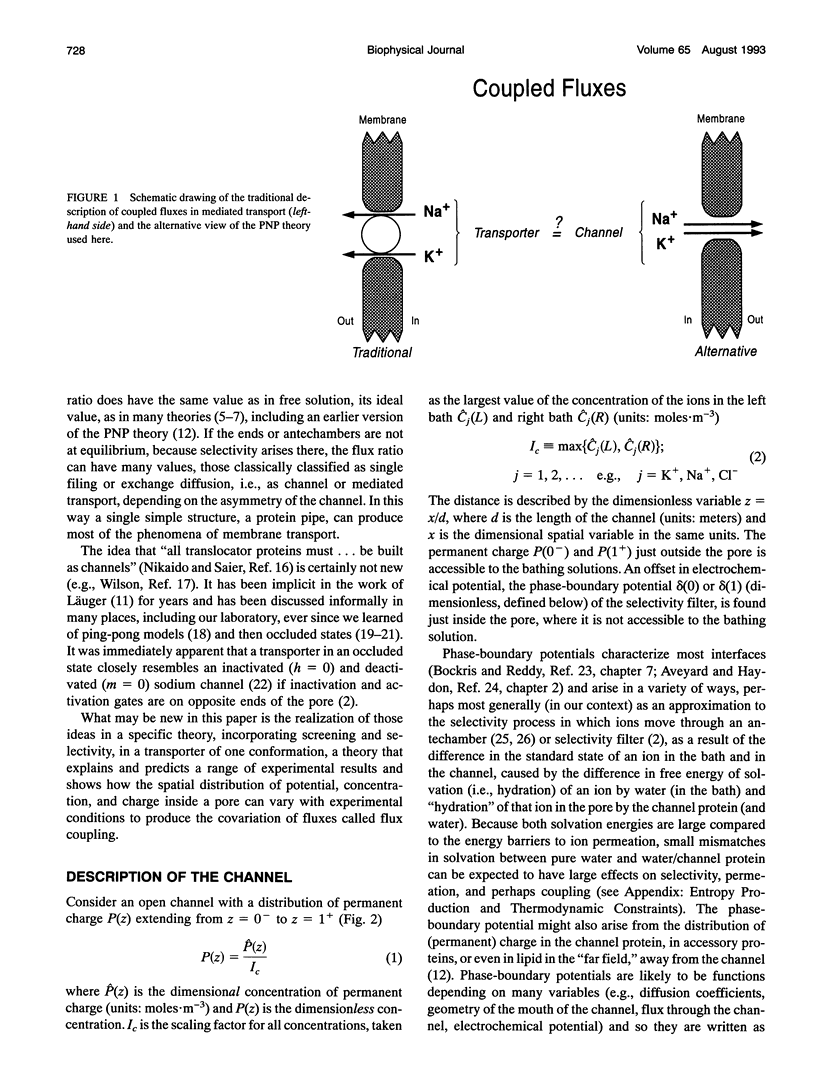
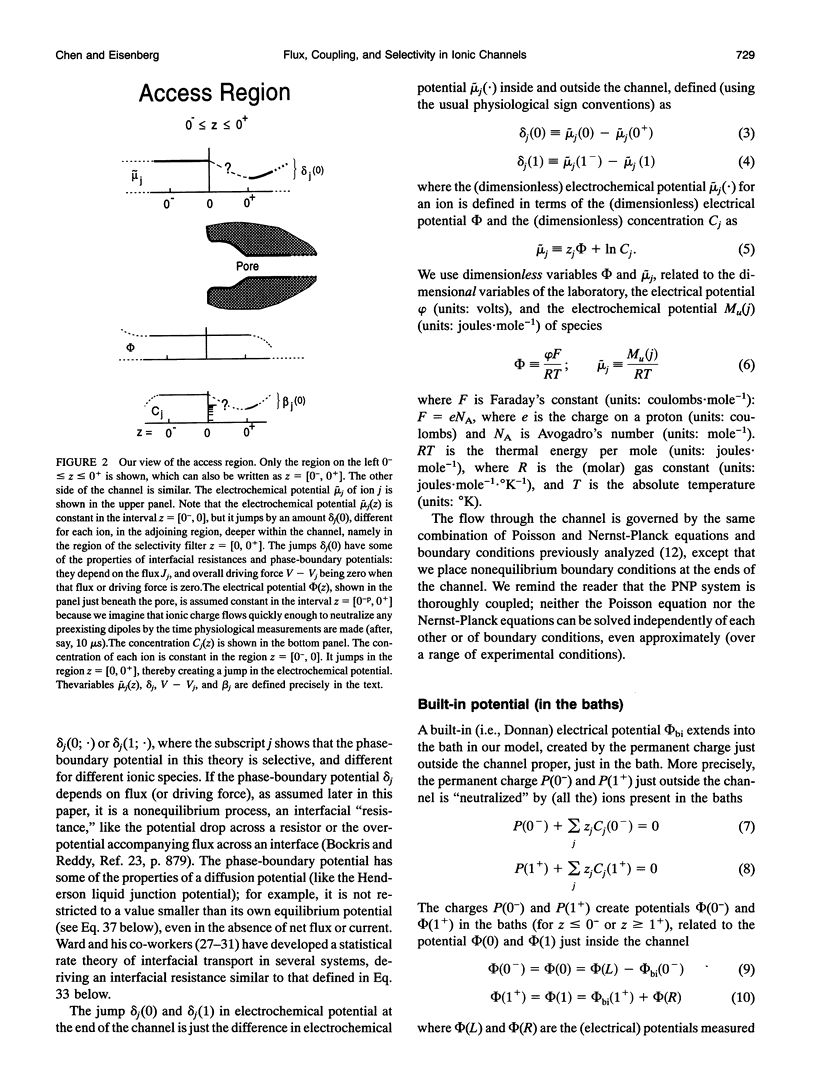
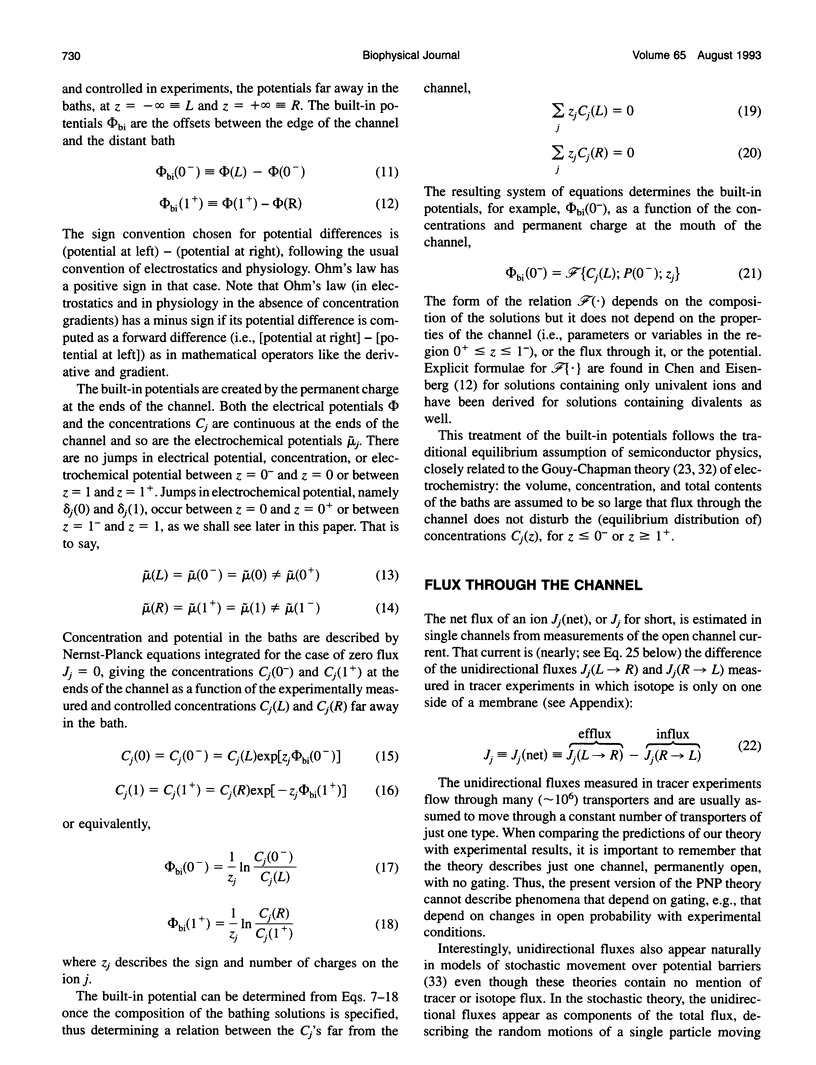
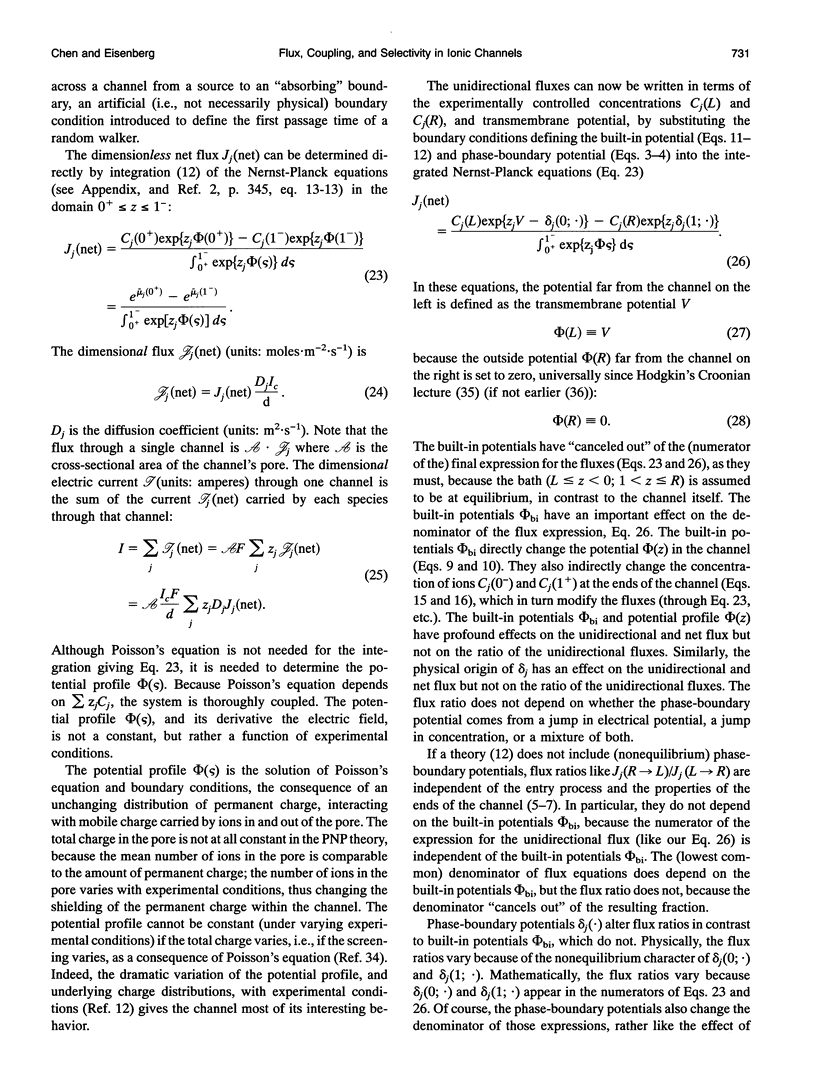
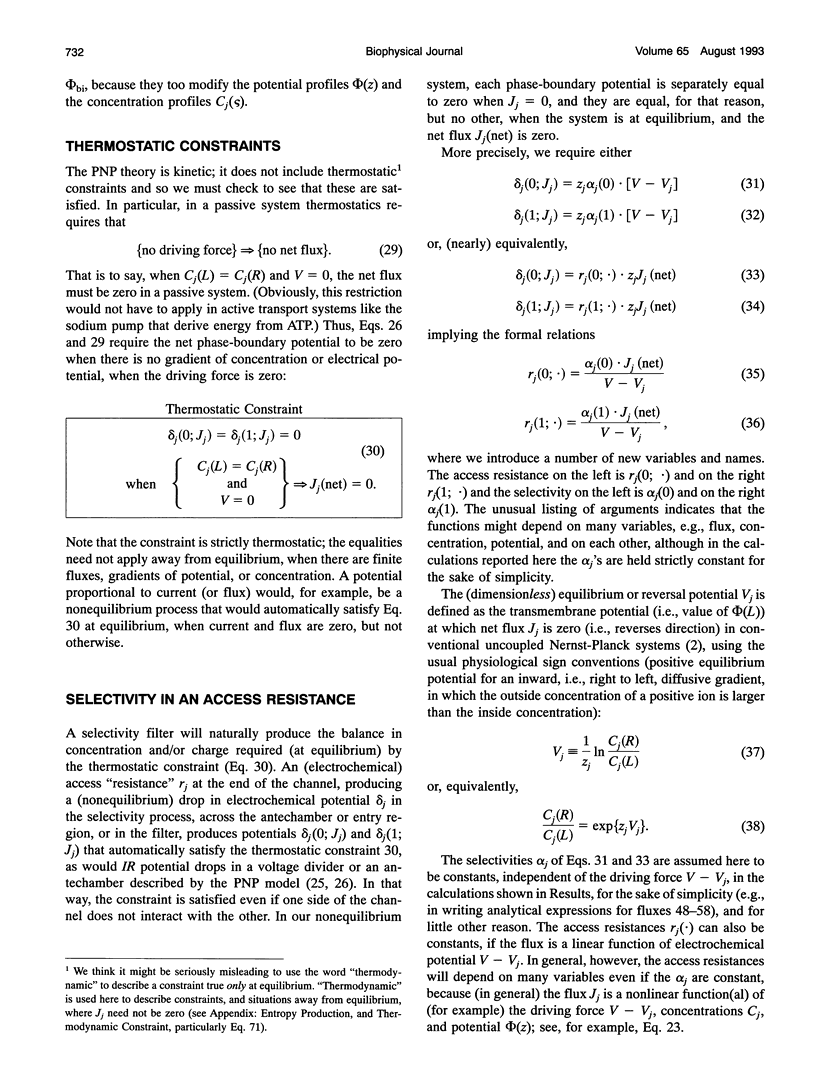
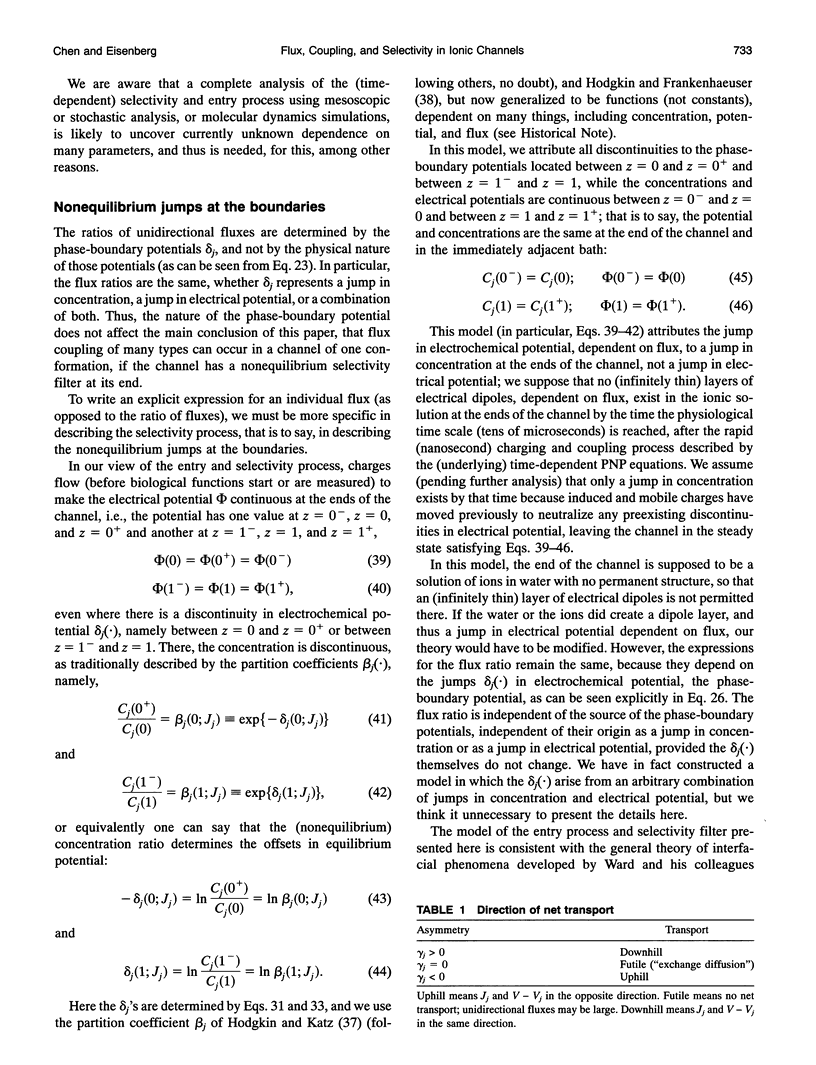
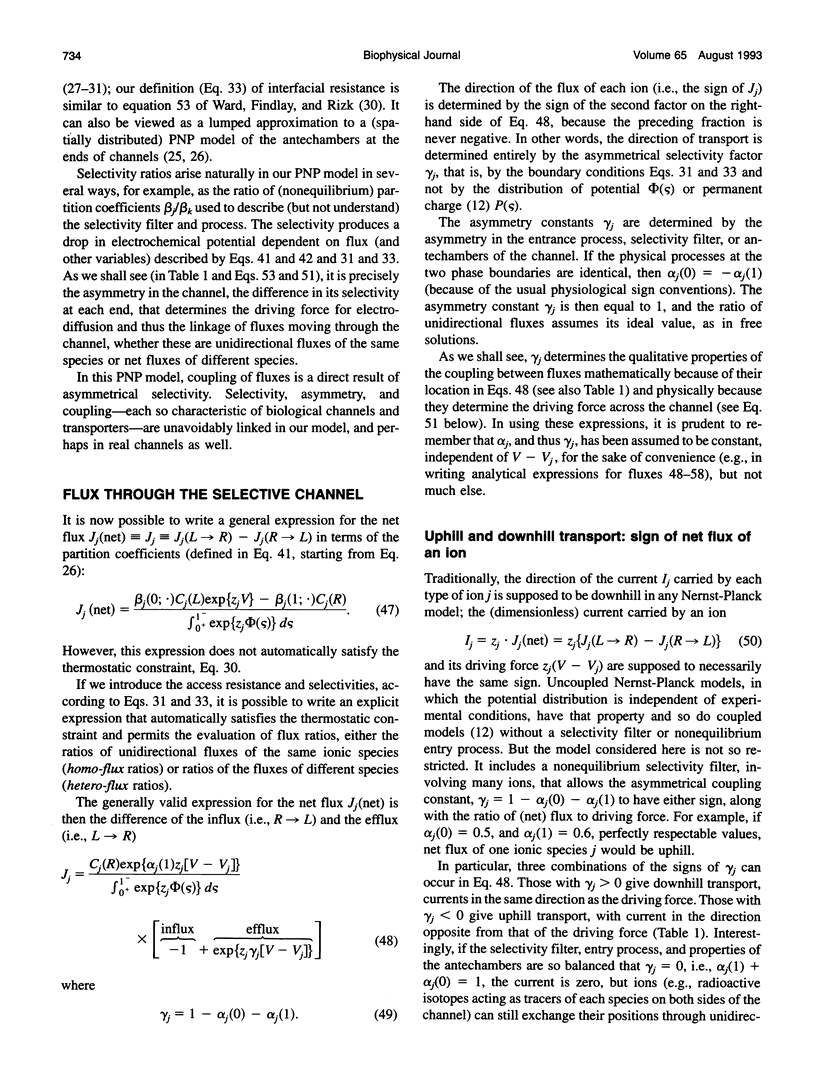
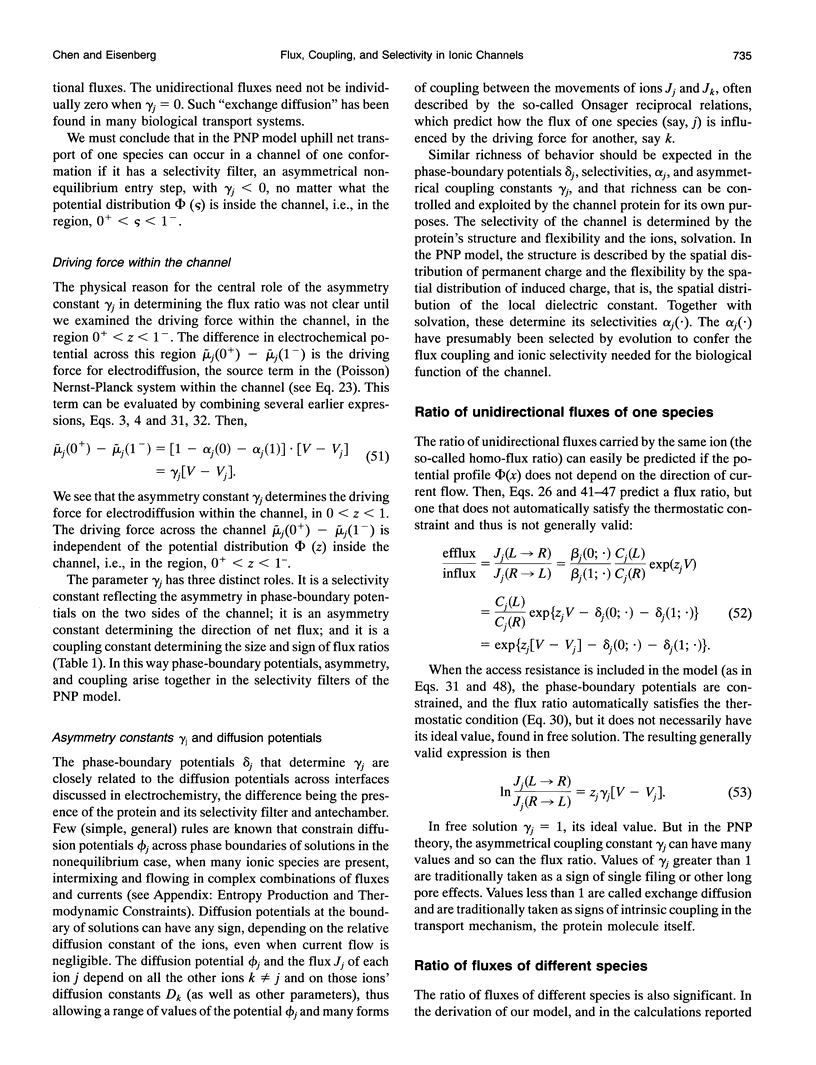
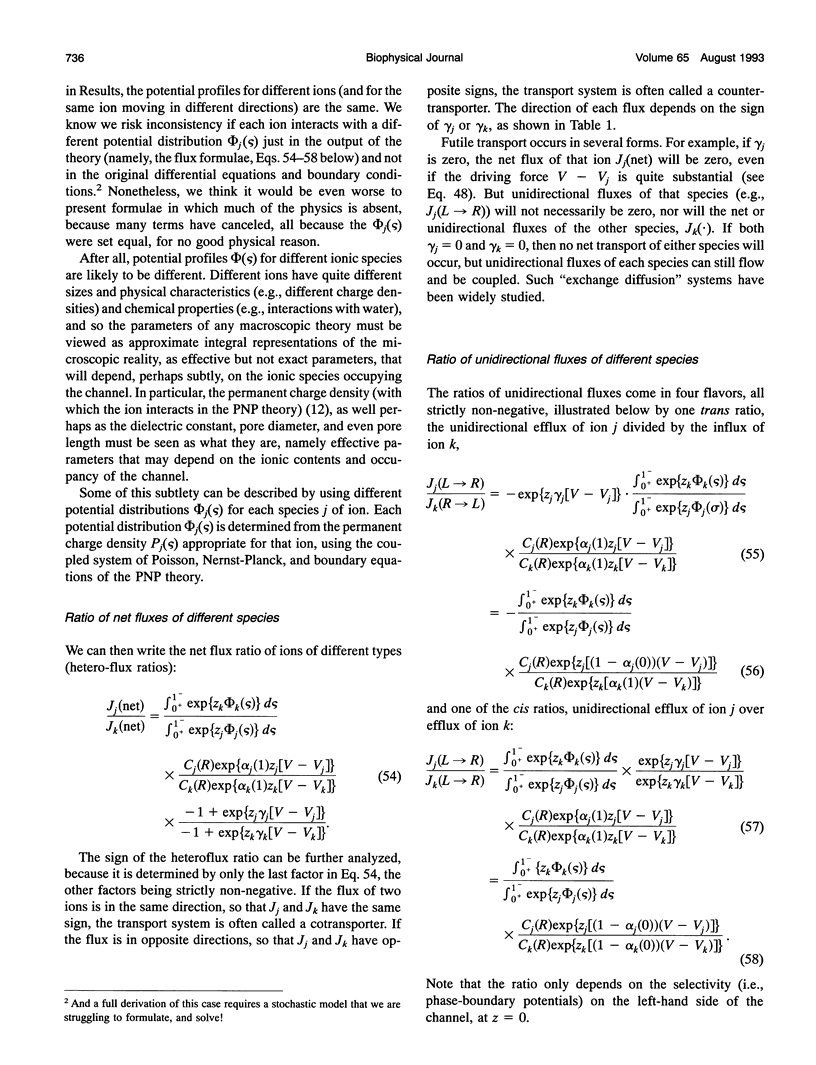
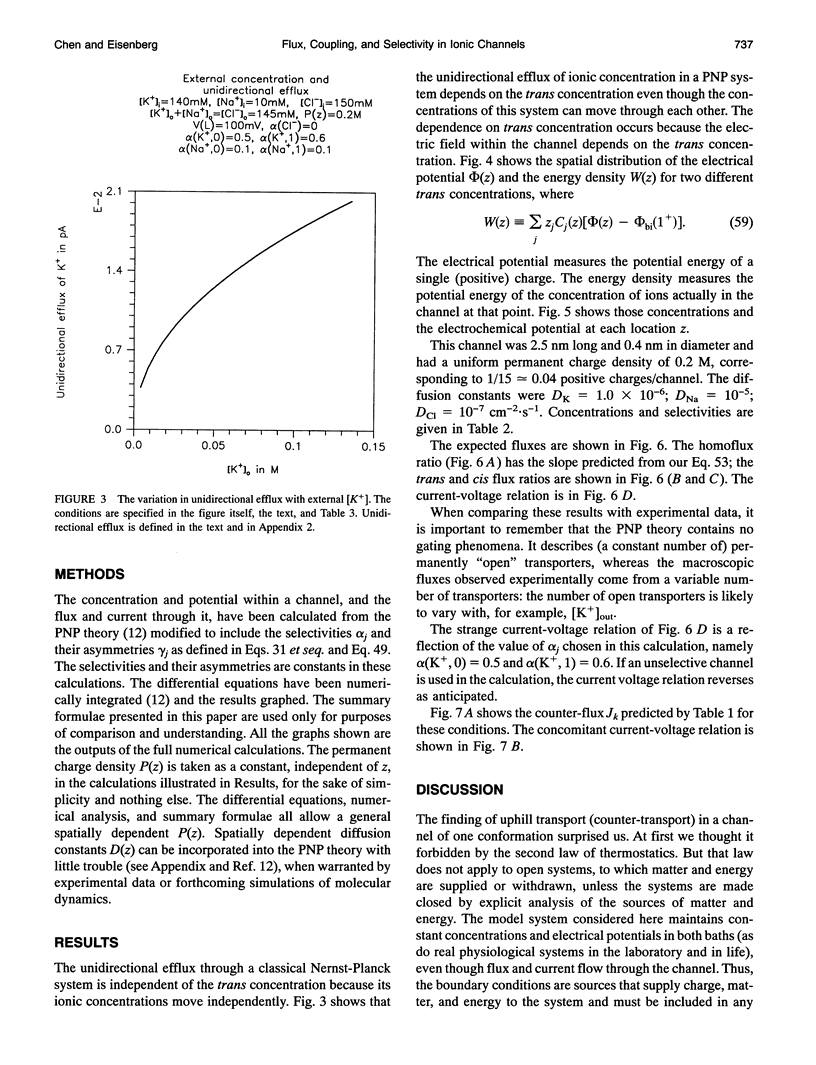
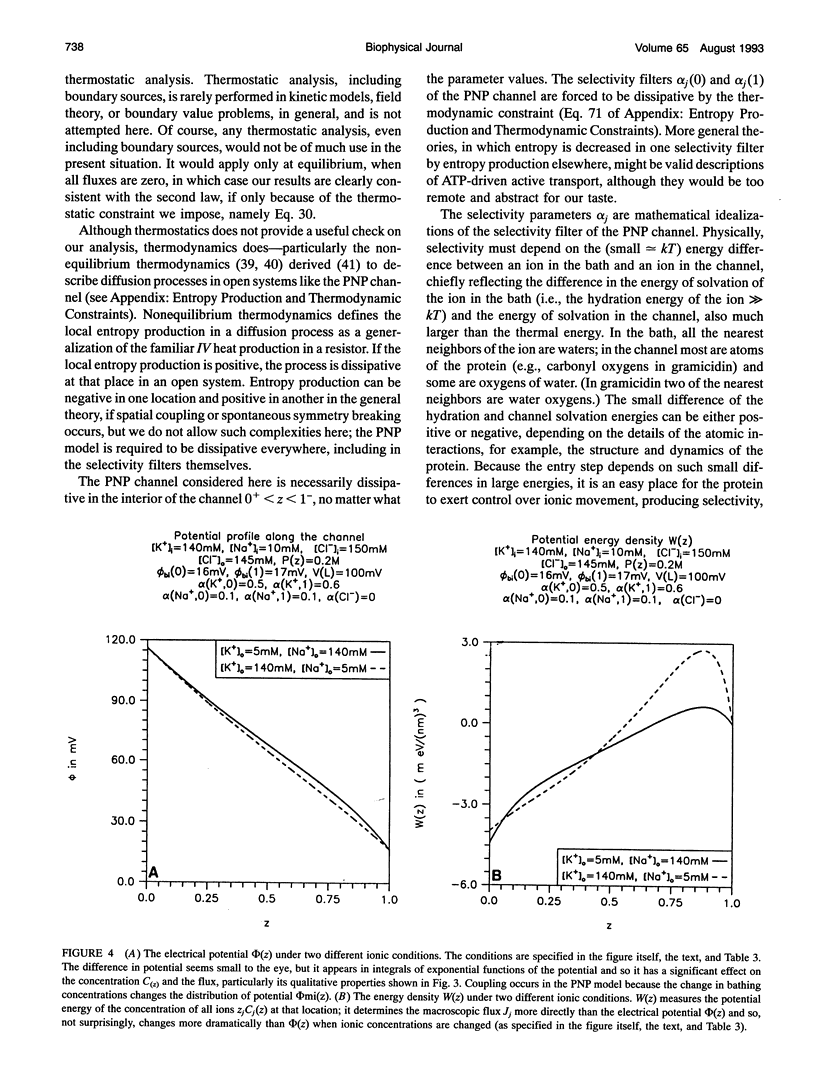
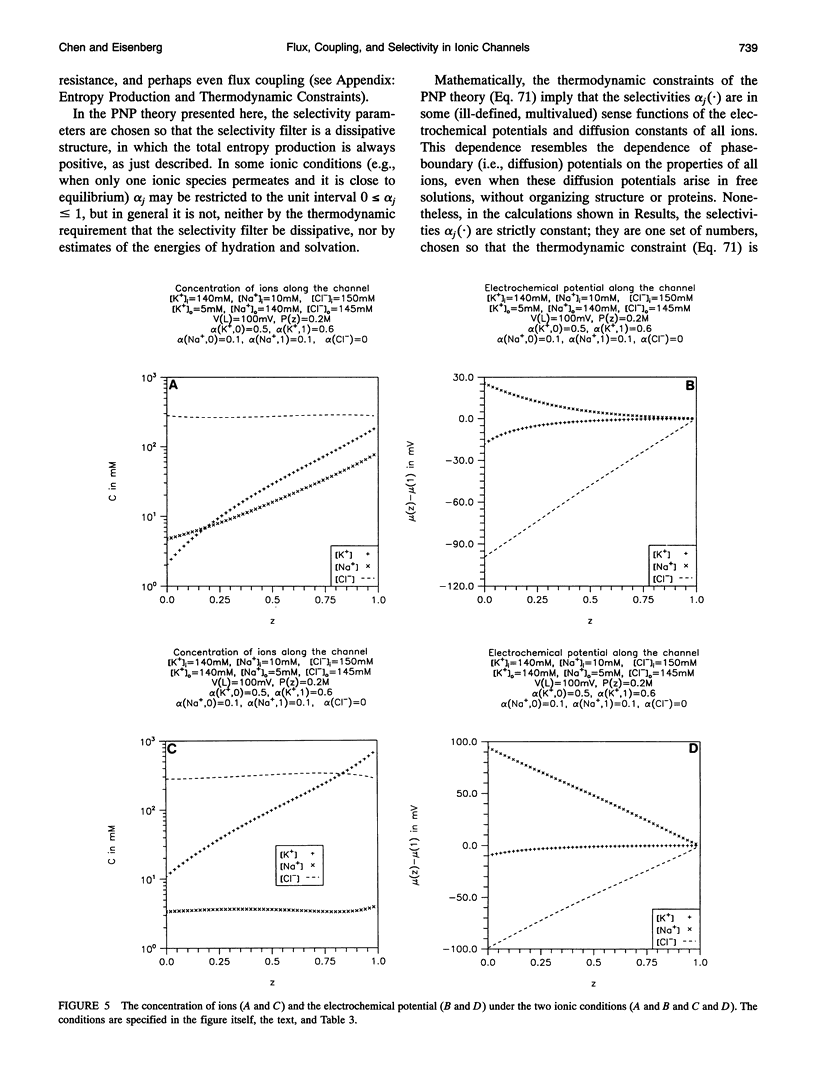
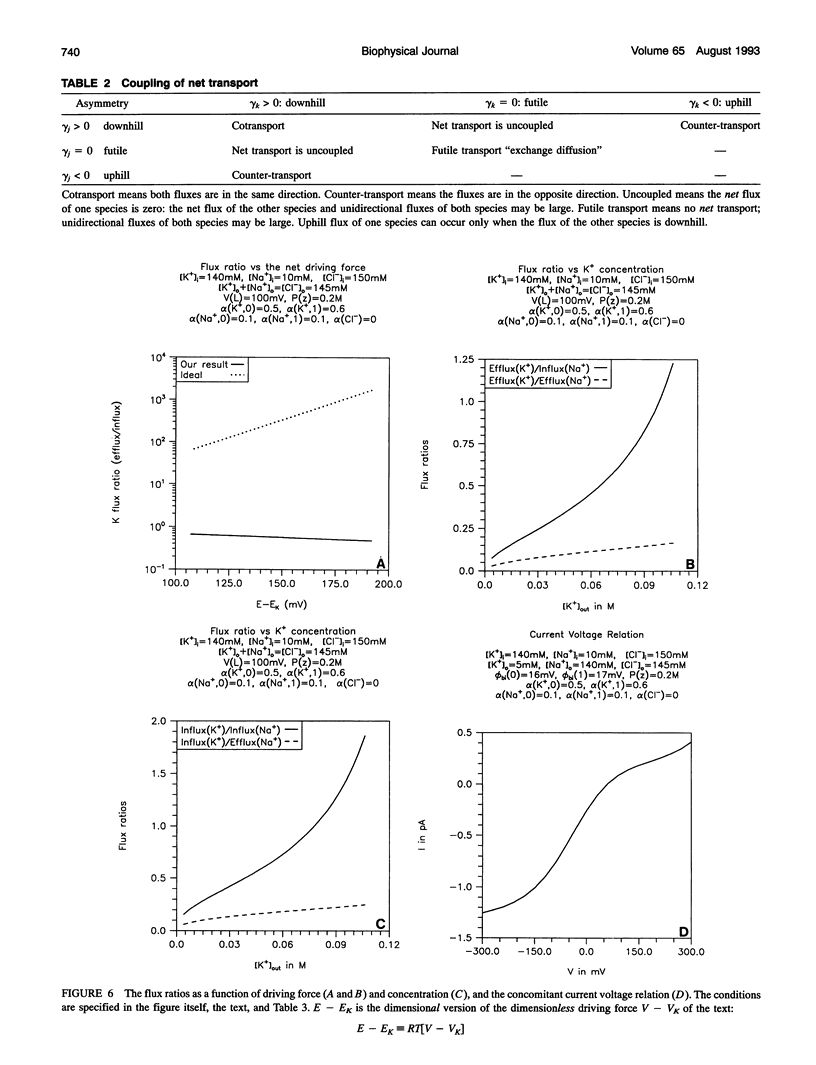
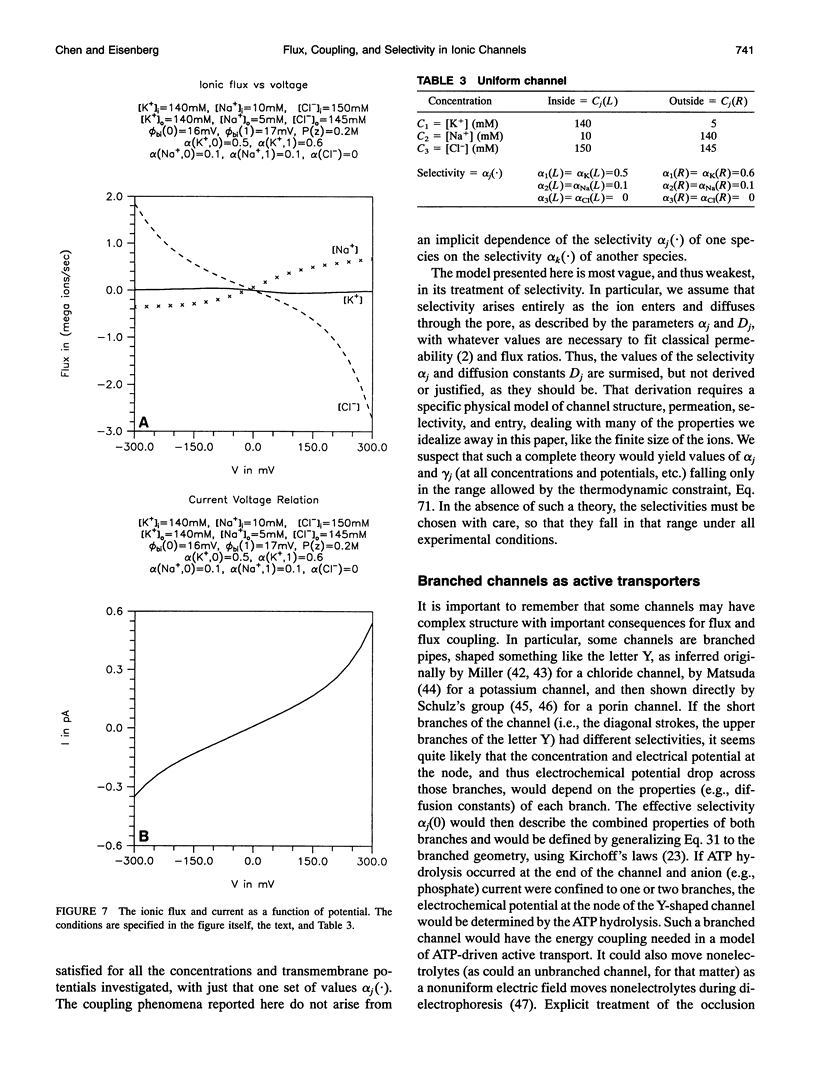
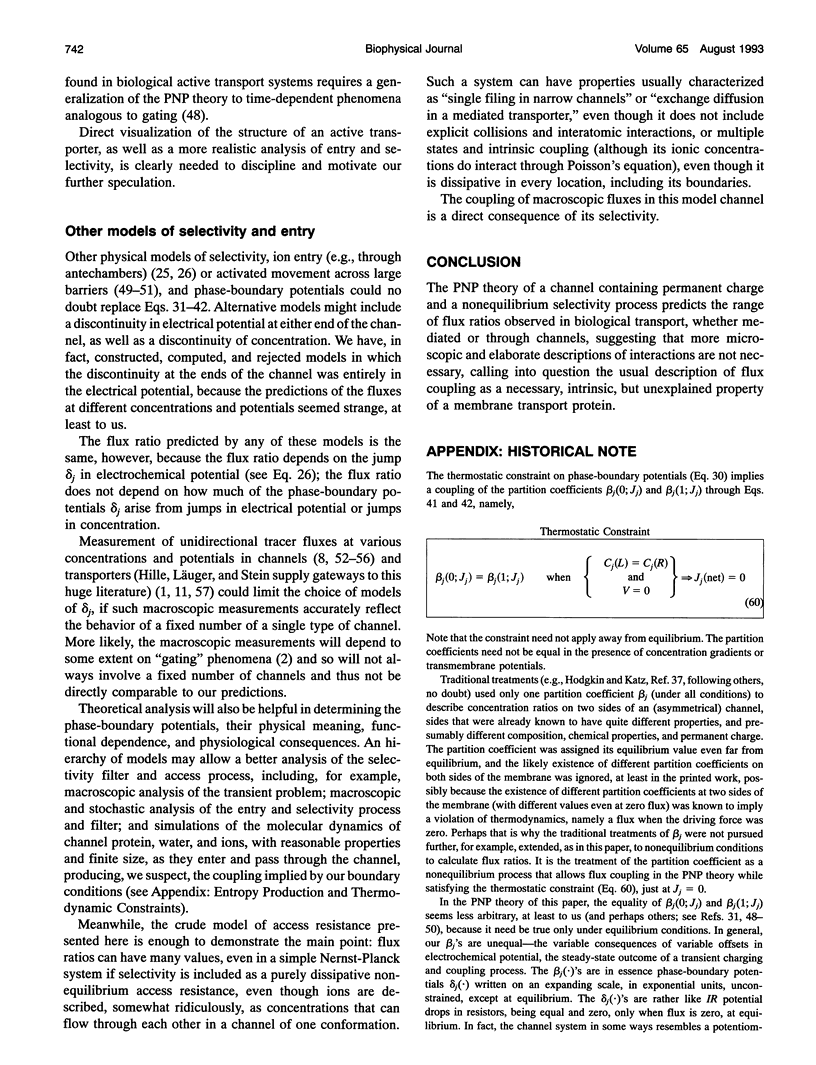
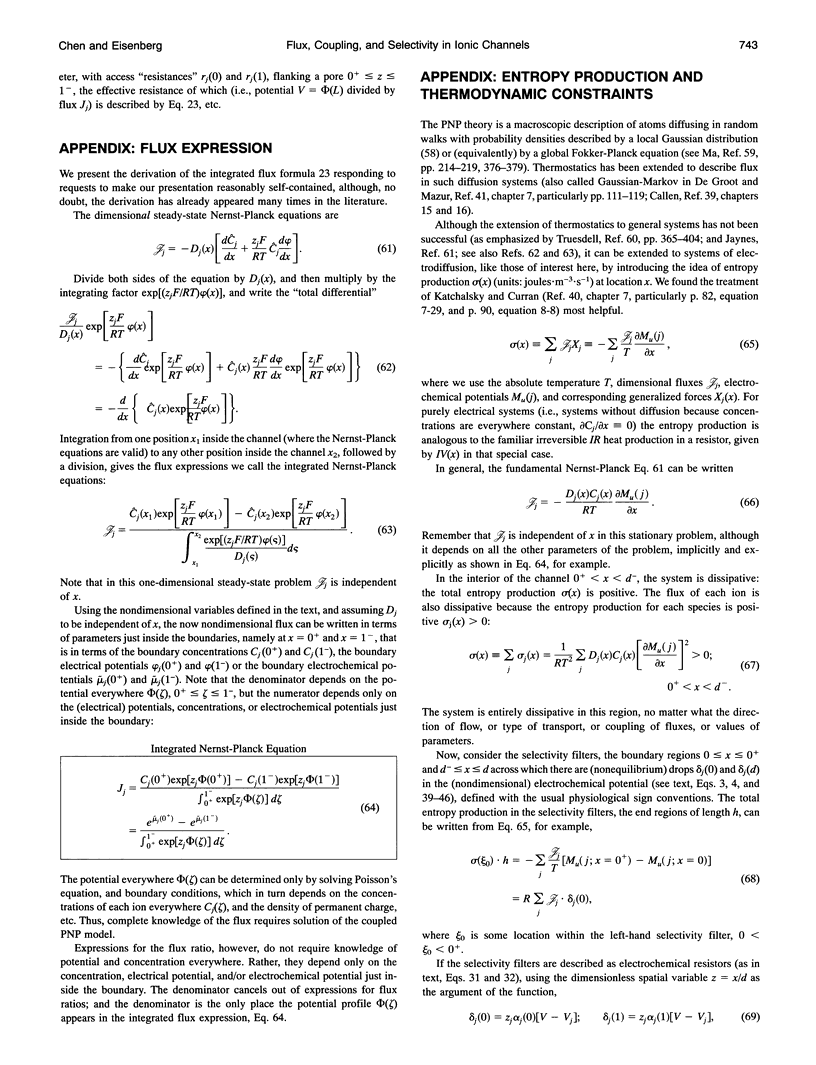
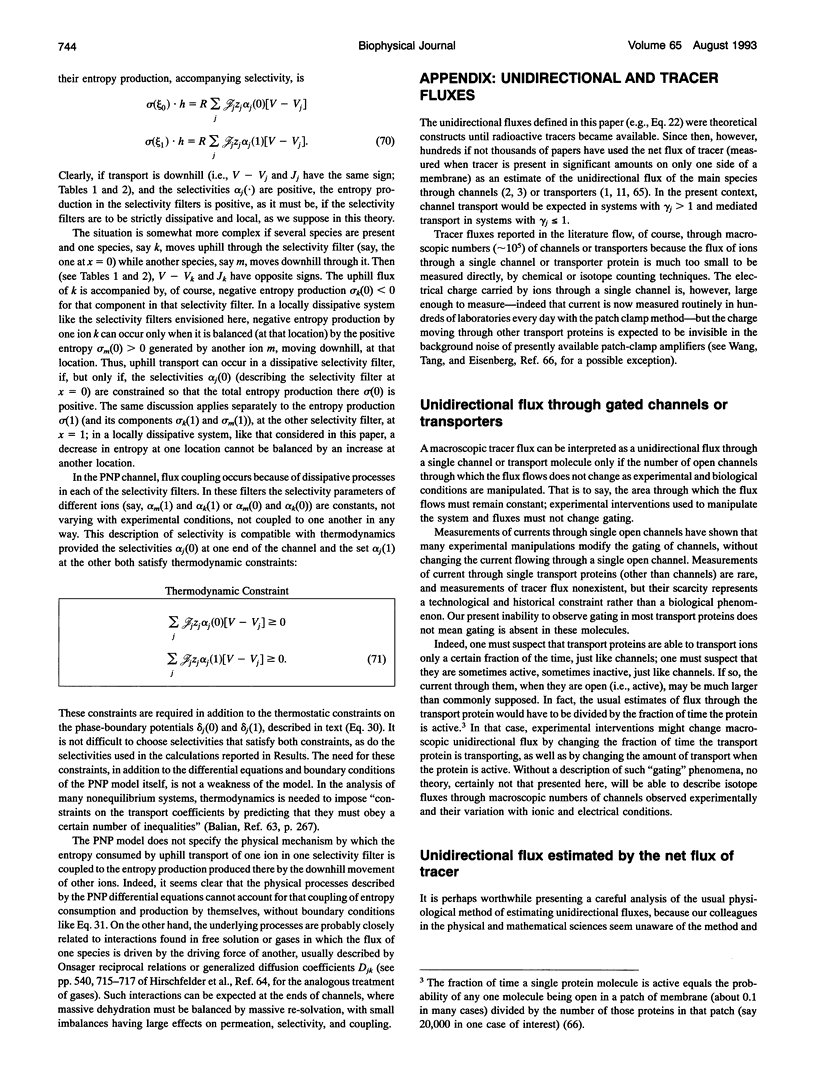
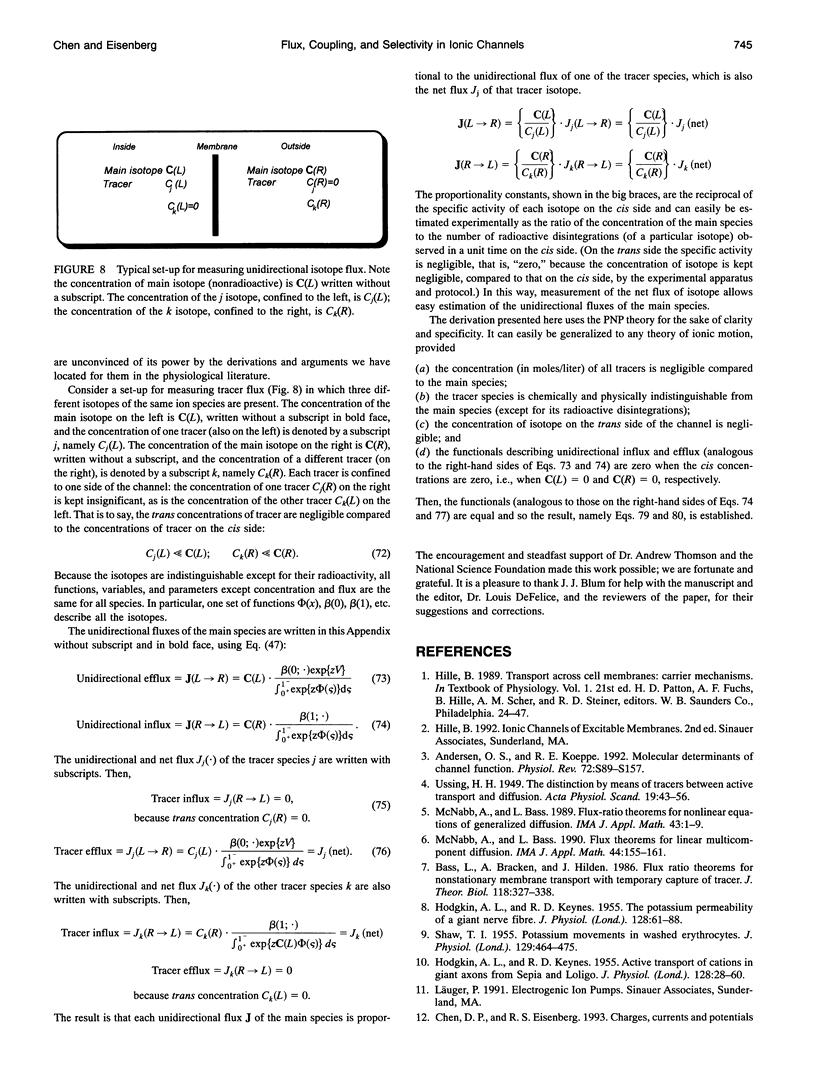
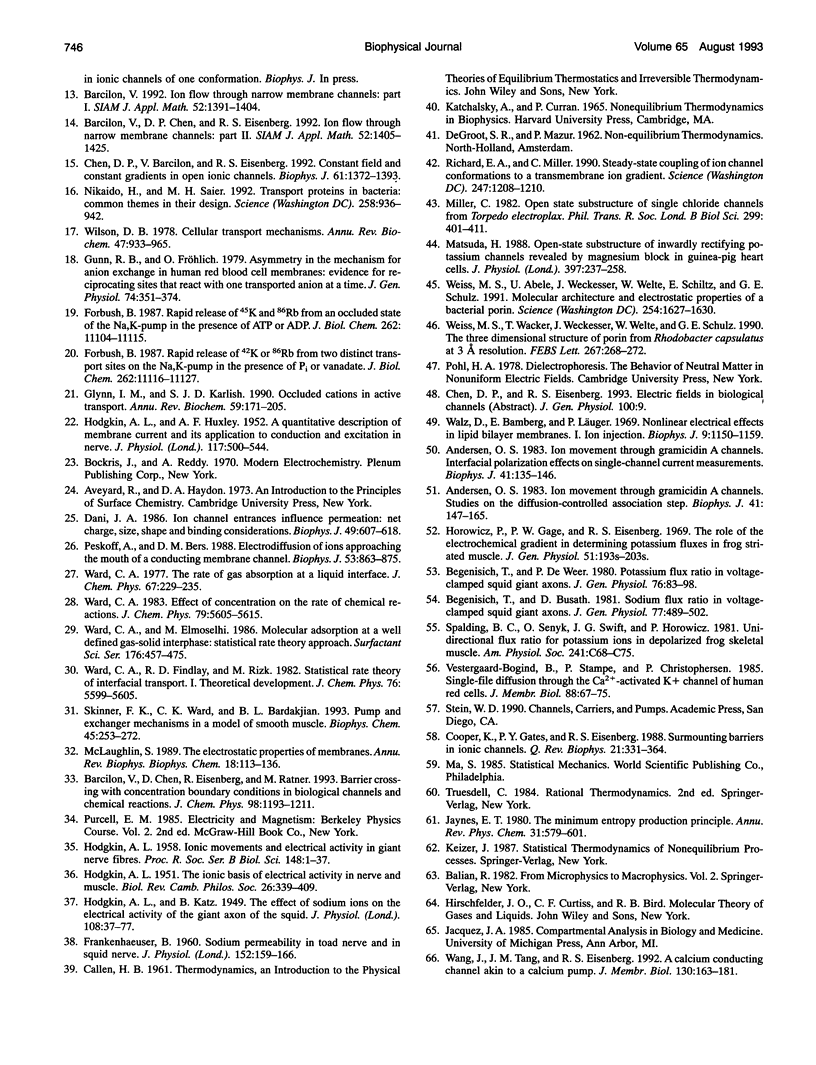
Selected References
These references are in PubMed. This may not be the complete list of references from this article.
- Andersen O. S. Ion movement through gramicidin A channels. Interfacial polarization effects on single-channel current measurements. Biophys J. 1983 Feb;41(2):135–146. doi: 10.1016/S0006-3495(83)84415-4. [DOI] [PMC free article] [PubMed] [Google Scholar]
- Andersen O. S. Ion movement through gramicidin A channels. Studies on the diffusion-controlled association step. Biophys J. 1983 Feb;41(2):147–165. doi: 10.1016/S0006-3495(83)84416-6. [DOI] [PMC free article] [PubMed] [Google Scholar]
- Andersen O. S., Koeppe R. E., 2nd Molecular determinants of channel function. Physiol Rev. 1992 Oct;72(4 Suppl):S89–158. doi: 10.1152/physrev.1992.72.suppl_4.S89. [DOI] [PubMed] [Google Scholar]
- Bass L., Bracken A. J., Hilden J. Flux ratio theorems for nonstationary membrane transport with temporary capture of tracer. J Theor Biol. 1986 Feb 7;118(3):327–338. doi: 10.1016/s0022-5193(86)80063-7. [DOI] [PubMed] [Google Scholar]
- Begenisich T., Busath D. Sodium flux ratio in voltage-clamped squid giant axons. J Gen Physiol. 1981 May;77(5):489–502. doi: 10.1085/jgp.77.5.489. [DOI] [PMC free article] [PubMed] [Google Scholar]
- Begenisich T., De Weer P. Potassium flux ratio in voltage-clamped squid giant axons. J Gen Physiol. 1980 Jul;76(1):83–98. doi: 10.1085/jgp.76.1.83. [DOI] [PMC free article] [PubMed] [Google Scholar]
- Chen D. P., Barcilon V., Eisenberg R. S. Constant fields and constant gradients in open ionic channels. Biophys J. 1992 May;61(5):1372–1393. doi: 10.1016/S0006-3495(92)81944-6. [DOI] [PMC free article] [PubMed] [Google Scholar]
- Cooper K. E., Gates P. Y., Eisenberg R. S. Surmounting barriers in ionic channels. Q Rev Biophys. 1988 Aug;21(3):331–364. doi: 10.1017/s0033583500004480. [DOI] [PubMed] [Google Scholar]
- Dani J. A. Ion-channel entrances influence permeation. Net charge, size, shape, and binding considerations. Biophys J. 1986 Mar;49(3):607–618. doi: 10.1016/S0006-3495(86)83688-8. [DOI] [PMC free article] [PubMed] [Google Scholar]
- FRANKENHAEUSER B. Sodium permeability in toad nerve and in squid nerve. J Physiol. 1960 Jun;152:159–166. doi: 10.1113/jphysiol.1960.sp006477. [DOI] [PMC free article] [PubMed] [Google Scholar]
- Forbush B., 3rd Rapid release of 42K and 86Rb from an occluded state of the Na,K-pump in the presence of ATP or ADP. J Biol Chem. 1987 Aug 15;262(23):11104–11115. [PubMed] [Google Scholar]
- Forbush B., 3rd Rapid release of 42K or 86Rb from two distinct transport sites on the Na,K-pump in the presence of Pi or vanadate. J Biol Chem. 1987 Aug 15;262(23):11116–11127. [PubMed] [Google Scholar]
- Glynn I. M., Karlish S. J. Occluded cations in active transport. Annu Rev Biochem. 1990;59:171–205. doi: 10.1146/annurev.bi.59.070190.001131. [DOI] [PubMed] [Google Scholar]
- Gunn R. B., Fröhlich O. Asymmetry in the mechanism for anion exchange in human red blood cell membranes. Evidence for reciprocating sites that react with one transported anion at a time. J Gen Physiol. 1979 Sep;74(3):351–374. doi: 10.1085/jgp.74.3.351. [DOI] [PMC free article] [PubMed] [Google Scholar]
- HODGKIN A. L., HUXLEY A. F. A quantitative description of membrane current and its application to conduction and excitation in nerve. J Physiol. 1952 Aug;117(4):500–544. doi: 10.1113/jphysiol.1952.sp004764. [DOI] [PMC free article] [PubMed] [Google Scholar]
- HODGKIN A. L. Ionic movements and electrical activity in giant nerve fibres. Proc R Soc Lond B Biol Sci. 1958 Jan 1;148(930):1–37. doi: 10.1098/rspb.1958.0001. [DOI] [PubMed] [Google Scholar]
- HODGKIN A. L., KATZ B. The effect of sodium ions on the electrical activity of giant axon of the squid. J Physiol. 1949 Mar 1;108(1):37–77. doi: 10.1113/jphysiol.1949.sp004310. [DOI] [PMC free article] [PubMed] [Google Scholar]
- HODGKIN A. L., KEYNES R. D. Active transport of cations in giant axons from Sepia and Loligo. J Physiol. 1955 Apr 28;128(1):28–60. doi: 10.1113/jphysiol.1955.sp005290. [DOI] [PMC free article] [PubMed] [Google Scholar]
- HODGKIN A. L., KEYNES R. D. The potassium permeability of a giant nerve fibre. J Physiol. 1955 Apr 28;128(1):61–88. doi: 10.1113/jphysiol.1955.sp005291. [DOI] [PMC free article] [PubMed] [Google Scholar]
- Horowicz P., Gage P. W., Eisenberg R. S. The role of the electrochemical gradient in determining potassium fluxes in frog striated muscle. J Gen Physiol. 1968 May;51(5 Suppl):193S+–193S+. [PubMed] [Google Scholar]
- Matsuda H. Open-state substructure of inwardly rectifying potassium channels revealed by magnesium block in guinea-pig heart cells. J Physiol. 1988 Mar;397:237–258. doi: 10.1113/jphysiol.1988.sp016998. [DOI] [PMC free article] [PubMed] [Google Scholar]
- McLaughlin S. The electrostatic properties of membranes. Annu Rev Biophys Biophys Chem. 1989;18:113–136. doi: 10.1146/annurev.bb.18.060189.000553. [DOI] [PubMed] [Google Scholar]
- Miller C. Open-state substructure of single chloride channels from Torpedo electroplax. Philos Trans R Soc Lond B Biol Sci. 1982 Dec 1;299(1097):401–411. doi: 10.1098/rstb.1982.0140. [DOI] [PubMed] [Google Scholar]
- Nikaido H., Saier M. H., Jr Transport proteins in bacteria: common themes in their design. Science. 1992 Nov 6;258(5084):936–942. doi: 10.1126/science.1279804. [DOI] [PubMed] [Google Scholar]
- Peskoff A., Bers D. M. Electrodiffusion of ions approaching the mouth of a conducting membrane channel. Biophys J. 1988 Jun;53(6):863–875. doi: 10.1016/S0006-3495(88)83167-9. [DOI] [PMC free article] [PubMed] [Google Scholar]
- Richard E. A., Miller C. Steady-state coupling of ion-channel conformations to a transmembrane ion gradient. Science. 1990 Mar 9;247(4947):1208–1210. doi: 10.1126/science.2156338. [DOI] [PubMed] [Google Scholar]
- SHAW T. I. Potassium movements in washed erythrocytes. J Physiol. 1955 Sep 28;129(3):464–475. doi: 10.1113/jphysiol.1955.sp005371. [DOI] [PMC free article] [PubMed] [Google Scholar]
- Skinner F. K., Ward C. A., Bardakjian B. L. Pump and exchanger mechanisms in a model of smooth muscle. Biophys Chem. 1993 Jan;45(3):253–272. doi: 10.1016/0301-4622(93)80007-6. [DOI] [PubMed] [Google Scholar]
- Spalding B. C., Senyk O., Swift J. G., Horowicz P. Unidirectional flux ratio for potassium ions in depolarized frog skeletal muscle. Am J Physiol. 1981 Jul;241(1):C68–C75. doi: 10.1152/ajpcell.1981.241.1.C68. [DOI] [PubMed] [Google Scholar]
- Vestergaard-Bogind B., Stampe P., Christophersen P. Single-file diffusion through the Ca2+-activated K+ channel of human red cells. J Membr Biol. 1985;88(1):67–75. doi: 10.1007/BF01871214. [DOI] [PubMed] [Google Scholar]
- Walz D., Bamberg E., Läuger P. Nonlinear electrical effects in lipid bilayer membranes. I. Ion injection. Biophys J. 1969 Sep;9(9):1150–1159. doi: 10.1016/S0006-3495(69)86442-8. [DOI] [PMC free article] [PubMed] [Google Scholar]
- Wang J., Tang J. M., Eisenberg R. S. A calcium conducting channel akin to a calcium pump. J Membr Biol. 1992 Nov;130(2):163–181. doi: 10.1007/BF00231894. [DOI] [PubMed] [Google Scholar]
- Weiss M. S., Abele U., Weckesser J., Welte W., Schiltz E., Schulz G. E. Molecular architecture and electrostatic properties of a bacterial porin. Science. 1991 Dec 13;254(5038):1627–1630. doi: 10.1126/science.1721242. [DOI] [PubMed] [Google Scholar]
- Weiss M. S., Wacker T., Weckesser J., Welte W., Schulz G. E. The three-dimensional structure of porin from Rhodobacter capsulatus at 3 A resolution. FEBS Lett. 1990 Jul 16;267(2):268–272. doi: 10.1016/0014-5793(90)80942-c. [DOI] [PubMed] [Google Scholar]
- Wilson D. B. Cellular transport mechanisms. Annu Rev Biochem. 1978;47:933–965. doi: 10.1146/annurev.bi.47.070178.004441. [DOI] [PubMed] [Google Scholar]