Abstract
Phasic block of rat cardiac Na+ current by saxitoxin was assessed using pulse trains and two-pulse voltage clamp protocols, and the results were fit to several kinetic models. For brief depolarizations (5 to 50 ms) the depolarization duration did not affect the rate of development or the amplitude of phasic block for pulse trains. The pulse train data were well described by a recurrence relation based upon the guarded receptor model, and it provided rate constants that accurately predicted first-pulse block as well as recovery time constants in response to two-pulse protocols. However, the amplitudes and rates of phasic block development at rapid rates (> 5 Hz) were less than the model predicted. For two pulse protocols with a short (10 ms) conditioning step to -30 mV, block developed only after repolarization to -150 mV and then recovered as the interpulse interval was increased. This suggested that phasic block under these conditions was caused by binding with increased affinity to a state that exists transiently after repolarization to -150 mV. This "post-repolarization block" was fit to a three-state model consisting of a transient state with high affinity for the toxin, the toxin bound state, and the ultimate resting state of the channel. This model accounted for the biphasic post-repolarization block development and recovery observed in two-pulse protocols, and it more accurately described phasic block in pulse trains. The transient state after repolarization was predicted to have a dwell time of 570 ms, an on rate for saxitoxin of 16 s-1 micro M-1, and an off rate of 0.2 s-1 (KD = 12 nM). These results and the proposed model suggest a novel variation on phasic block mechanisms and suggest a long-lived transient Na+ channel conformation during recovery.
Full text
PDF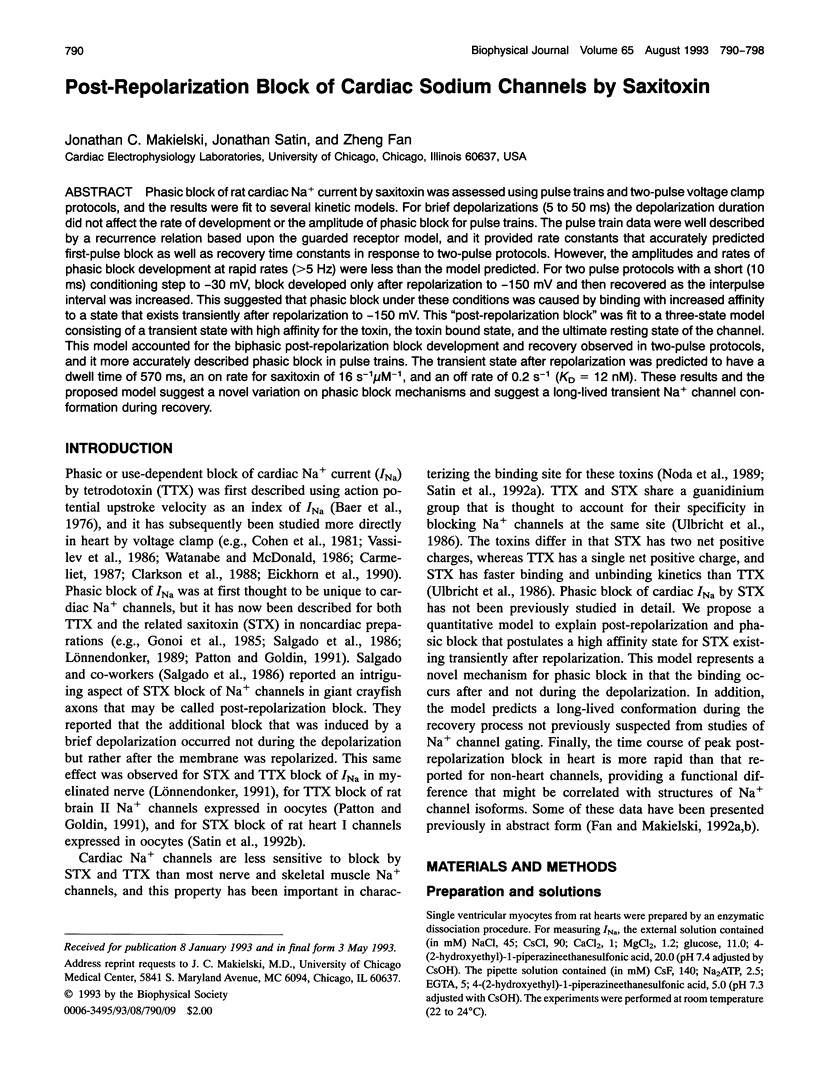
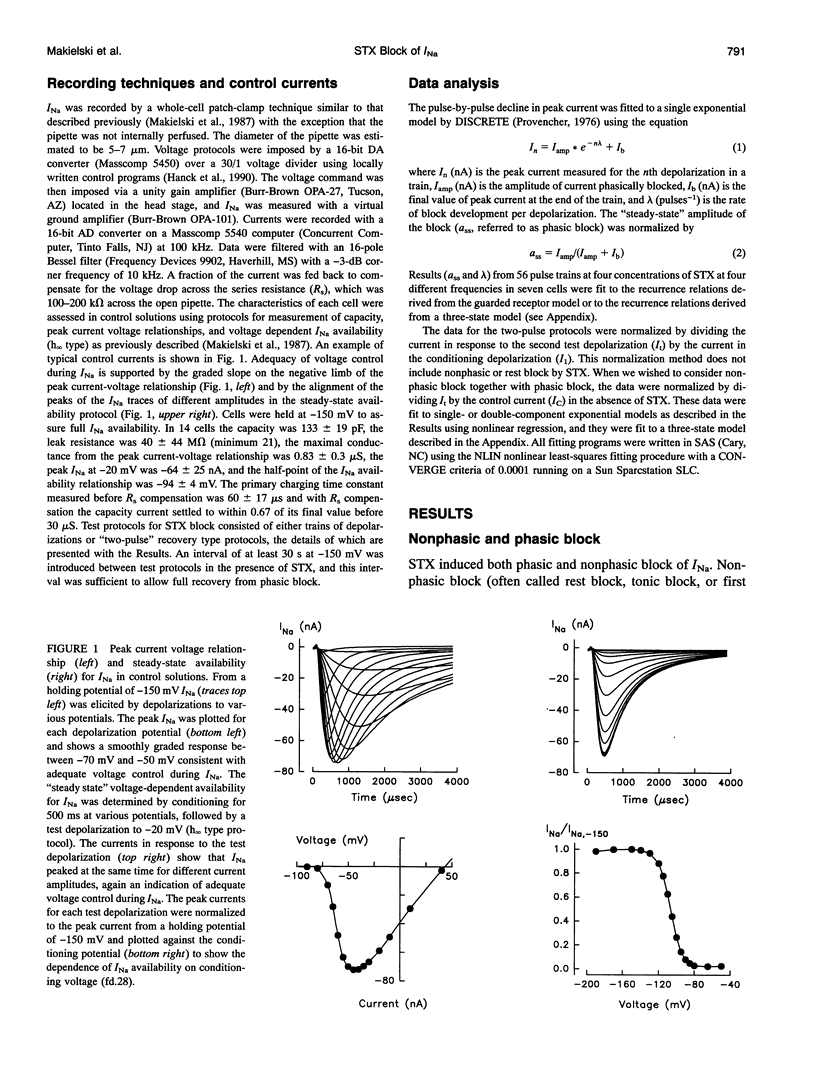
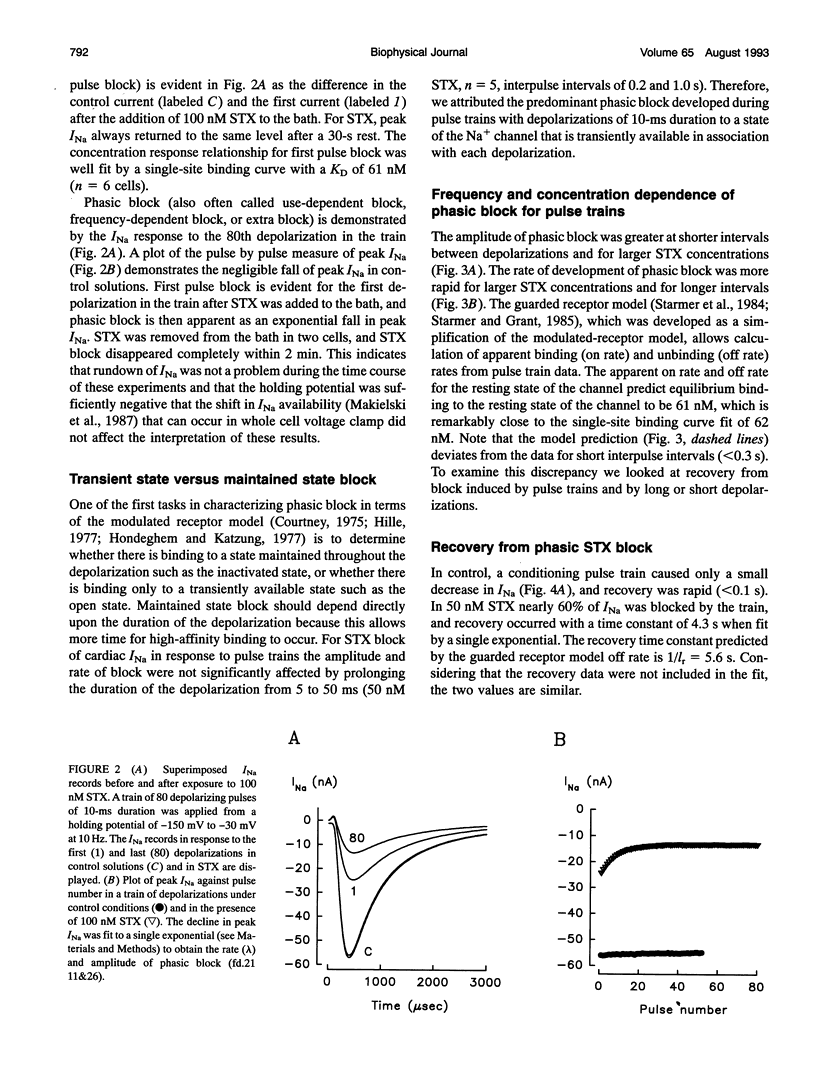
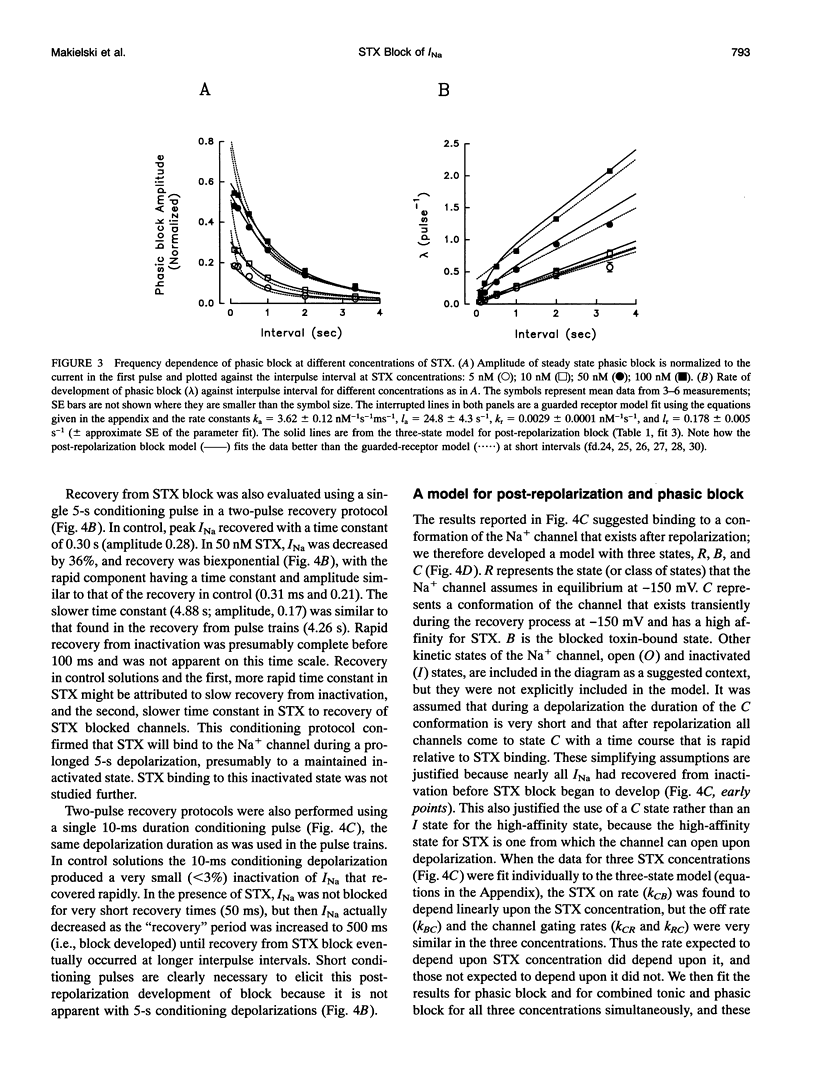
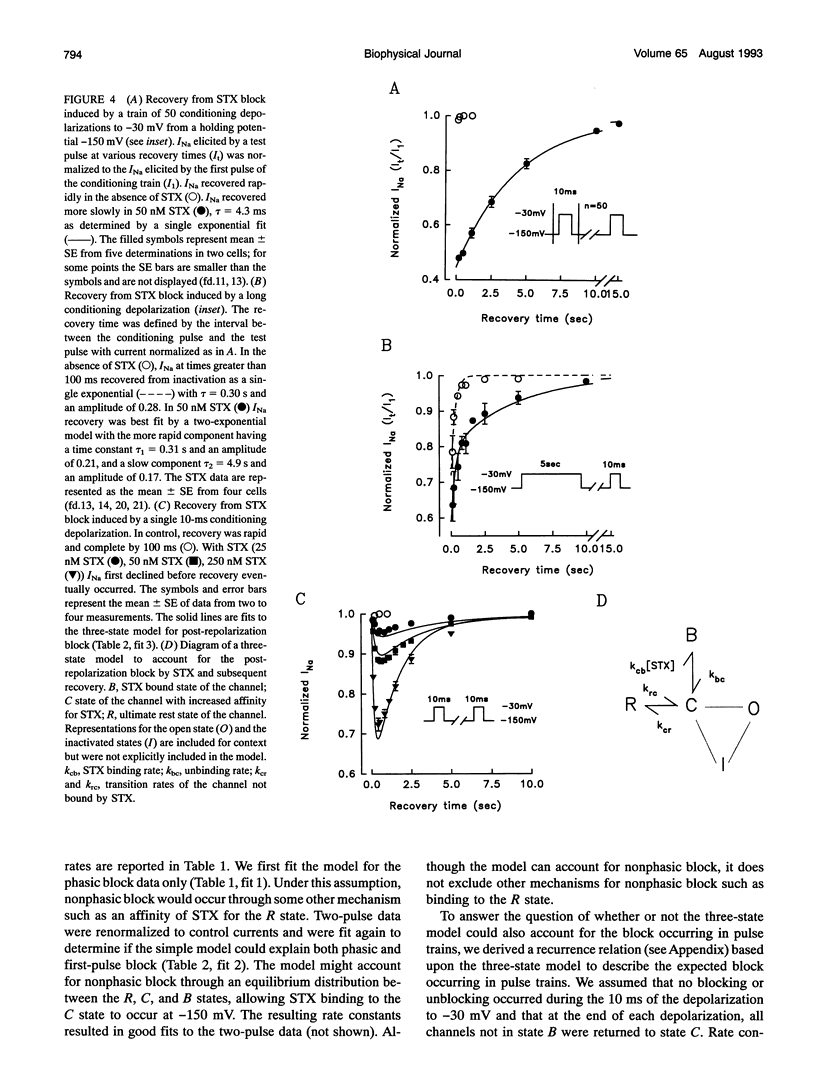
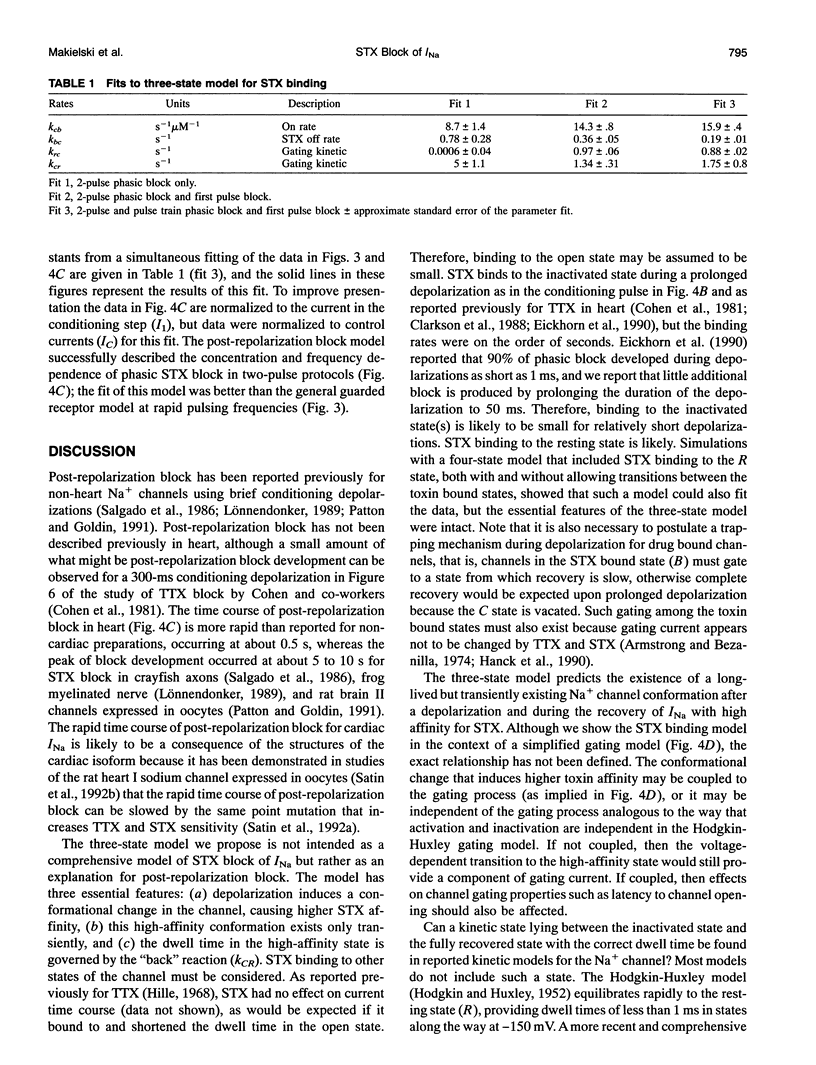
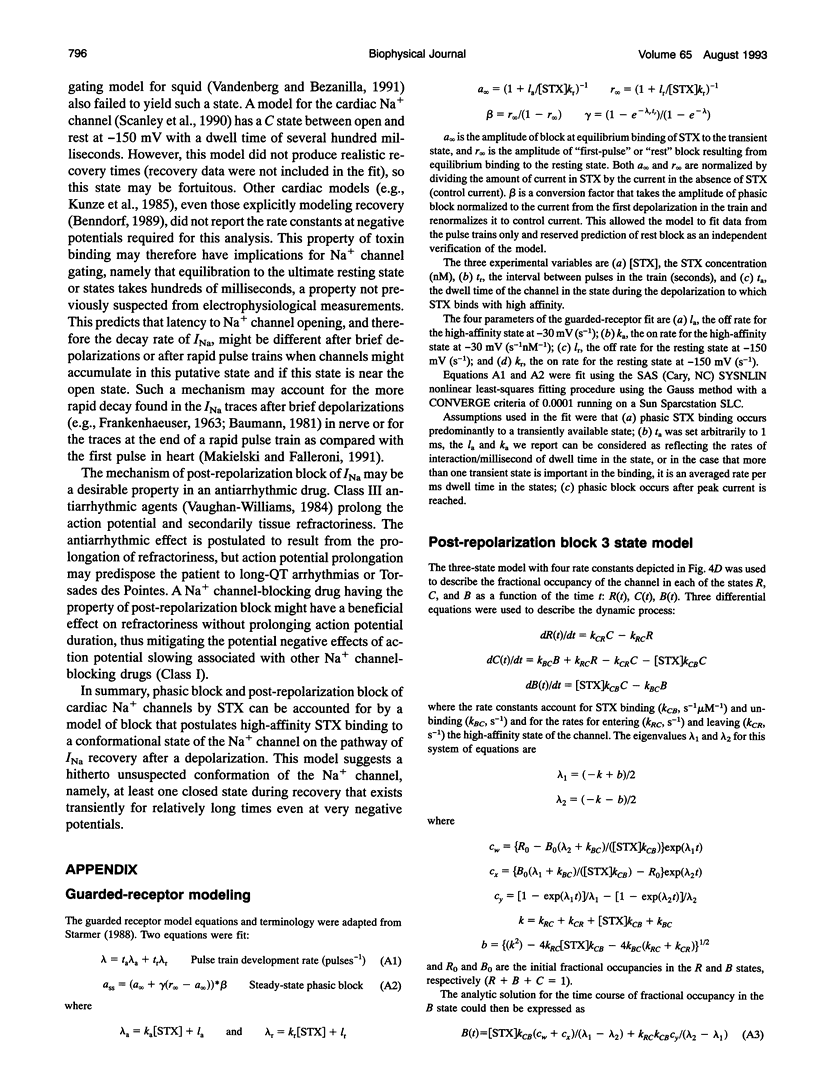
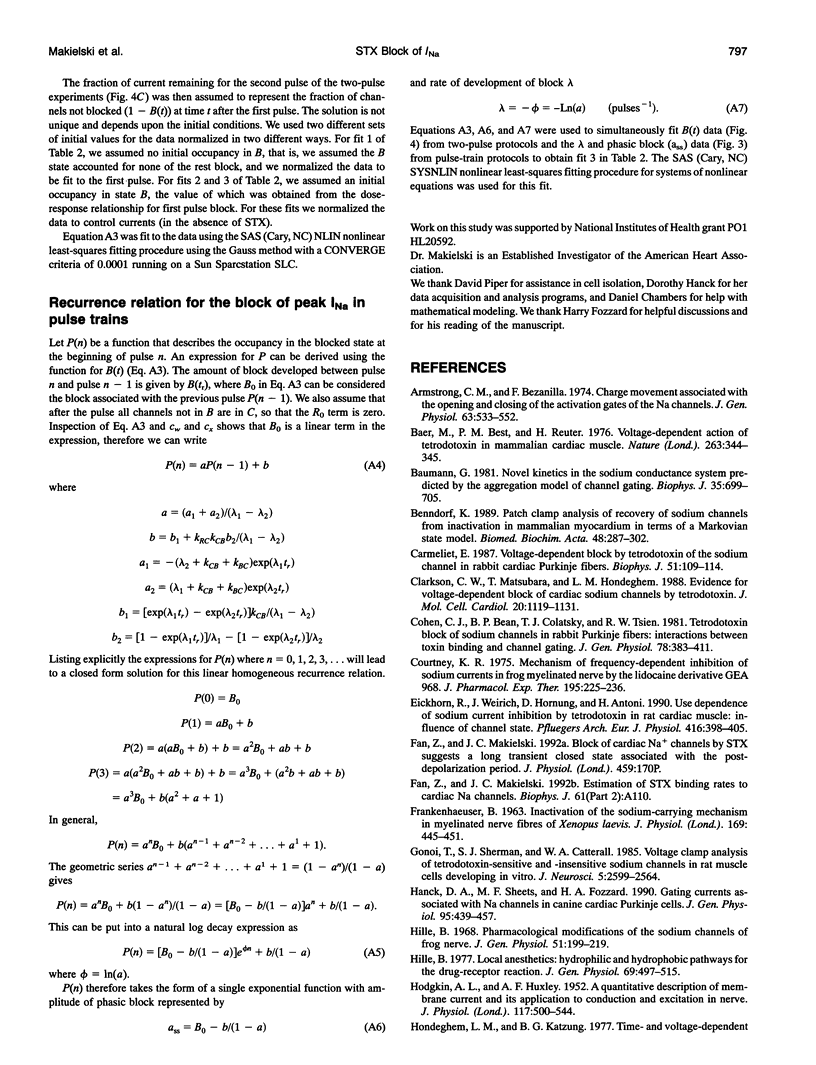
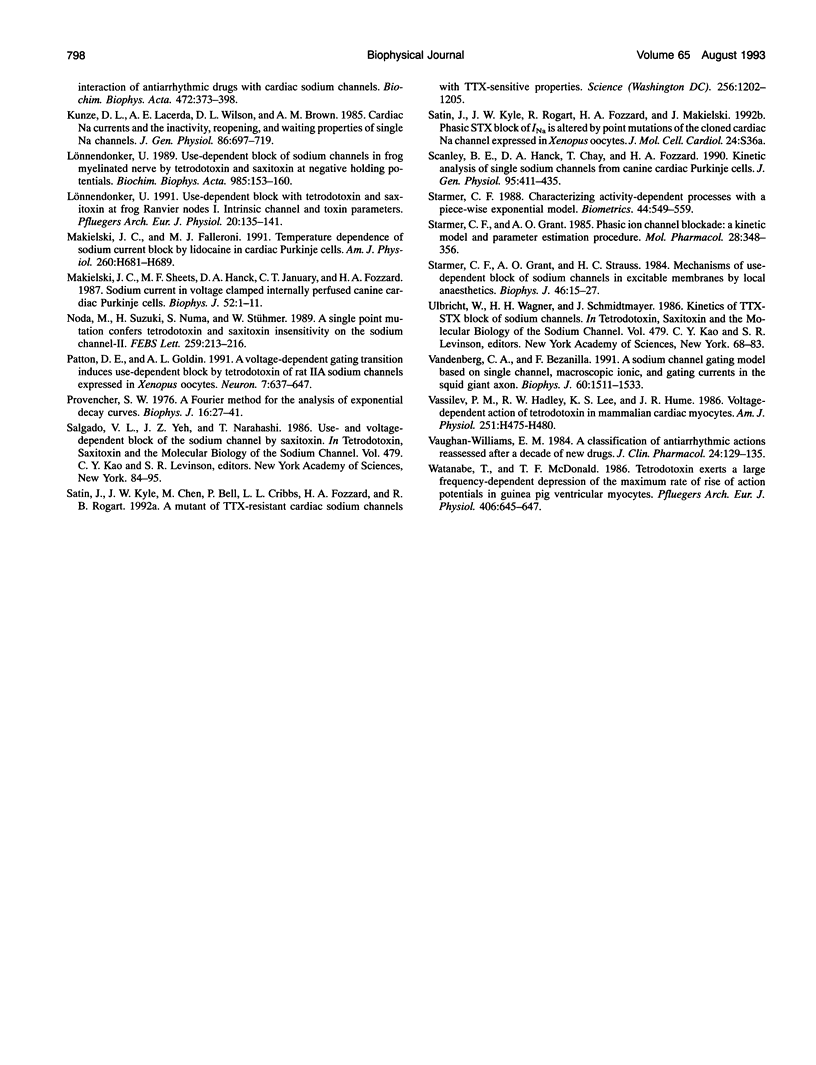
Selected References
These references are in PubMed. This may not be the complete list of references from this article.
- Armstrong C. M., Bezanilla F. Charge movement associated with the opening and closing of the activation gates of the Na channels. J Gen Physiol. 1974 May;63(5):533–552. doi: 10.1085/jgp.63.5.533. [DOI] [PMC free article] [PubMed] [Google Scholar]
- Baer M., Best P. M., Reuter H. Voltage-dependent action of tetrodotoxin in mammalian cardiac muscle. Nature. 1976 Sep 23;263(5575):344–345. doi: 10.1038/263344a0. [DOI] [PubMed] [Google Scholar]
- Baumann G. Novel kinetics in the sodium conductance system predicted by the aggregation model of channel gating. Biophys J. 1981 Sep;35(3):699–705. doi: 10.1016/S0006-3495(81)84821-7. [DOI] [PMC free article] [PubMed] [Google Scholar]
- Benndorf K. Patch clamp analysis of recovery of sodium channels from inactivation in mammalian myocardium in terms of a Markovian state model. Biomed Biochim Acta. 1989;48(4):287–302. [PubMed] [Google Scholar]
- Carmeliet E. Voltage-dependent block by tetrodotoxin of the sodium channel in rabbit cardiac Purkinje fibers. Biophys J. 1987 Jan;51(1):109–114. doi: 10.1016/S0006-3495(87)83315-5. [DOI] [PMC free article] [PubMed] [Google Scholar]
- Clarkson C. W., Matsubara T., Hondeghem L. M. Evidence for voltage-dependent block of cardiac sodium channels by tetrodotoxin. J Mol Cell Cardiol. 1988 Dec;20(12):1119–1131. doi: 10.1016/0022-2828(88)90592-5. [DOI] [PubMed] [Google Scholar]
- Cohen C. J., Bean B. P., Colatsky T. J., Tsien R. W. Tetrodotoxin block of sodium channels in rabbit Purkinje fibers. Interactions between toxin binding and channel gating. J Gen Physiol. 1981 Oct;78(4):383–411. doi: 10.1085/jgp.78.4.383. [DOI] [PMC free article] [PubMed] [Google Scholar]
- Courtney K. R. Mechanism of frequency-dependent inhibition of sodium currents in frog myelinated nerve by the lidocaine derivative GEA. J Pharmacol Exp Ther. 1975 Nov;195(2):225–236. [PubMed] [Google Scholar]
- Eickhorn R., Weirich J., Hornung D., Antoni H. Use dependence of sodium current inhibition by tetrodotoxin in rat cardiac muscle: influence of channel state. Pflugers Arch. 1990 Jun;416(4):398–405. doi: 10.1007/BF00370746. [DOI] [PubMed] [Google Scholar]
- FRANKENHAEUSER B. INACTIVATION OF THE SODIUM-CARRYING MECHANISM IN MYELINATED NERVE FIBRES OF XENOPUS LAEVIS. J Physiol. 1963 Nov;169:445–451. doi: 10.1113/jphysiol.1963.sp007271. [DOI] [PMC free article] [PubMed] [Google Scholar]
- Gonoi T., Sherman S. J., Catterall W. A. Voltage clamp analysis of tetrodotoxin-sensitive and -insensitive sodium channels in rat muscle cells developing in vitro. J Neurosci. 1985 Sep;5(9):2559–2564. doi: 10.1523/JNEUROSCI.05-09-02559.1985. [DOI] [PMC free article] [PubMed] [Google Scholar]
- HODGKIN A. L., HUXLEY A. F. A quantitative description of membrane current and its application to conduction and excitation in nerve. J Physiol. 1952 Aug;117(4):500–544. doi: 10.1113/jphysiol.1952.sp004764. [DOI] [PMC free article] [PubMed] [Google Scholar]
- Hanck D. A., Sheets M. F., Fozzard H. A. Gating currents associated with Na channels in canine cardiac Purkinje cells. J Gen Physiol. 1990 Mar;95(3):439–457. doi: 10.1085/jgp.95.3.439. [DOI] [PMC free article] [PubMed] [Google Scholar]
- Hille B. Local anesthetics: hydrophilic and hydrophobic pathways for the drug-receptor reaction. J Gen Physiol. 1977 Apr;69(4):497–515. doi: 10.1085/jgp.69.4.497. [DOI] [PMC free article] [PubMed] [Google Scholar]
- Hille B. Pharmacological modifications of the sodium channels of frog nerve. J Gen Physiol. 1968 Feb;51(2):199–219. doi: 10.1085/jgp.51.2.199. [DOI] [PMC free article] [PubMed] [Google Scholar]
- Hondeghem L. M., Katzung B. G. Time- and voltage-dependent interactions of antiarrhythmic drugs with cardiac sodium channels. Biochim Biophys Acta. 1977 Nov 14;472(3-4):373–398. doi: 10.1016/0304-4157(77)90003-x. [DOI] [PubMed] [Google Scholar]
- Kunze D. L., Lacerda A. E., Wilson D. L., Brown A. M. Cardiac Na currents and the inactivating, reopening, and waiting properties of single cardiac Na channels. J Gen Physiol. 1985 Nov;86(5):691–719. doi: 10.1085/jgp.86.5.691. [DOI] [PMC free article] [PubMed] [Google Scholar]
- Lönnendonker U. Use-dependent block with tetrodotoxin and saxitoxin at frog Ranvier nodes. I. Intrinsic channel and toxin parameters. Eur Biophys J. 1991;20(3):135–141. doi: 10.1007/BF01561135. [DOI] [PubMed] [Google Scholar]
- Makielski J. C., Falleroni M. J. Temperature dependence of sodium current block by lidocaine in cardiac Purkinje cells. Am J Physiol. 1991 Mar;260(3 Pt 2):H681–H689. doi: 10.1152/ajpheart.1991.260.3.H681. [DOI] [PubMed] [Google Scholar]
- Makielski J. C., Sheets M. F., Hanck D. A., January C. T., Fozzard H. A. Sodium current in voltage clamped internally perfused canine cardiac Purkinje cells. Biophys J. 1987 Jul;52(1):1–11. doi: 10.1016/S0006-3495(87)83182-X. [DOI] [PMC free article] [PubMed] [Google Scholar]
- Noda M., Suzuki H., Numa S., Stühmer W. A single point mutation confers tetrodotoxin and saxitoxin insensitivity on the sodium channel II. FEBS Lett. 1989 Dec 18;259(1):213–216. doi: 10.1016/0014-5793(89)81531-5. [DOI] [PubMed] [Google Scholar]
- Patton D. E., Goldin A. L. A voltage-dependent gating transition induces use-dependent block by tetrodotoxin of rat IIA sodium channels expressed in Xenopus oocytes. Neuron. 1991 Oct;7(4):637–647. doi: 10.1016/0896-6273(91)90376-b. [DOI] [PubMed] [Google Scholar]
- Provencher S. W. A Fourier method for the analysis of exponential decay curves. Biophys J. 1976 Jan;16(1):27–41. doi: 10.1016/S0006-3495(76)85660-3. [DOI] [PMC free article] [PubMed] [Google Scholar]
- Salgado V. L., Yeh J. Z., Narahashi T. Use- and voltage-dependent block of the sodium channel by saxitoxin. Ann N Y Acad Sci. 1986;479:84–95. doi: 10.1111/j.1749-6632.1986.tb15563.x. [DOI] [PubMed] [Google Scholar]
- Satin J., Kyle J. W., Chen M., Bell P., Cribbs L. L., Fozzard H. A., Rogart R. B. A mutant of TTX-resistant cardiac sodium channels with TTX-sensitive properties. Science. 1992 May 22;256(5060):1202–1205. doi: 10.1126/science.256.5060.1202. [DOI] [PubMed] [Google Scholar]
- Scanley B. E., Hanck D. A., Chay T., Fozzard H. A. Kinetic analysis of single sodium channels from canine cardiac Purkinje cells. J Gen Physiol. 1990 Mar;95(3):411–437. doi: 10.1085/jgp.95.3.411. [DOI] [PMC free article] [PubMed] [Google Scholar]
- Starmer C. F. Characterizing activity-dependent processes with a piecewise exponential model. Biometrics. 1988 Jun;44(2):549–559. [PubMed] [Google Scholar]
- Starmer C. F., Grant A. O. Phasic ion channel blockade. A kinetic model and parameter estimation procedure. Mol Pharmacol. 1985 Oct;28(4):348–356. [PubMed] [Google Scholar]
- Starmer C. F., Grant A. O., Strauss H. C. Mechanisms of use-dependent block of sodium channels in excitable membranes by local anesthetics. Biophys J. 1984 Jul;46(1):15–27. doi: 10.1016/S0006-3495(84)83994-6. [DOI] [PMC free article] [PubMed] [Google Scholar]
- Ulbricht W., Wagner H. H., Schmidtmayer J. Kinetics of TTX-STX block of sodium channels. Ann N Y Acad Sci. 1986;479:68–83. doi: 10.1111/j.1749-6632.1986.tb15562.x. [DOI] [PubMed] [Google Scholar]
- Vandenberg C. A., Bezanilla F. A sodium channel gating model based on single channel, macroscopic ionic, and gating currents in the squid giant axon. Biophys J. 1991 Dec;60(6):1511–1533. doi: 10.1016/S0006-3495(91)82186-5. [DOI] [PMC free article] [PubMed] [Google Scholar]
- Vassilev P. M., Hadley R. W., Lee K. S., Hume J. R. Voltage-dependent action of tetrodotoxin in mammalian cardiac myocytes. Am J Physiol. 1986 Aug;251(2 Pt 2):H475–H480. doi: 10.1152/ajpheart.1986.251.2.H475. [DOI] [PubMed] [Google Scholar]
- Vaughan Williams E. M. A classification of antiarrhythmic actions reassessed after a decade of new drugs. J Clin Pharmacol. 1984 Apr;24(4):129–147. doi: 10.1002/j.1552-4604.1984.tb01822.x. [DOI] [PubMed] [Google Scholar]
- Watanabe T., McDonald T. F. Tetrodotoxin exerts a large frequency-dependent depression of the maximum rate of rise of action potentials in guinea pig ventricular myocytes. Pflugers Arch. 1986 Jun;406(6):645–647. doi: 10.1007/BF00584034. [DOI] [PubMed] [Google Scholar]