Abstract
Gigaohm seals made between patch pipettes and hydrophobic substrates have a finite conductance which are cation-selective and capable of producing quantized gating indistinguishable from the gating of biological ion channels. The selectivity sequence and streaming potentials of these seals suggests the existence of a pore of similar dimensions to the nicotinic acetylcholine channel. The ionic selectivity of these seals appears similar to the seal selectivity observed with membrane patches (Fischmeister, R., R. K. Ayer, and R. L. DeHann. 1986. Pfluegers Arch. 406:73-82) and the possibility of discrete gating within the seal region suggests caution when interpreting patch clamp data from unfamiliar preparations. The data suggests that the permeation pathway is the narrow space between the hydrophobic substrate and the pipette. Since this space has one hydrophobic wall, a hydrophilic channel lining may not be essential for channel permeation and gating.
Full text
PDF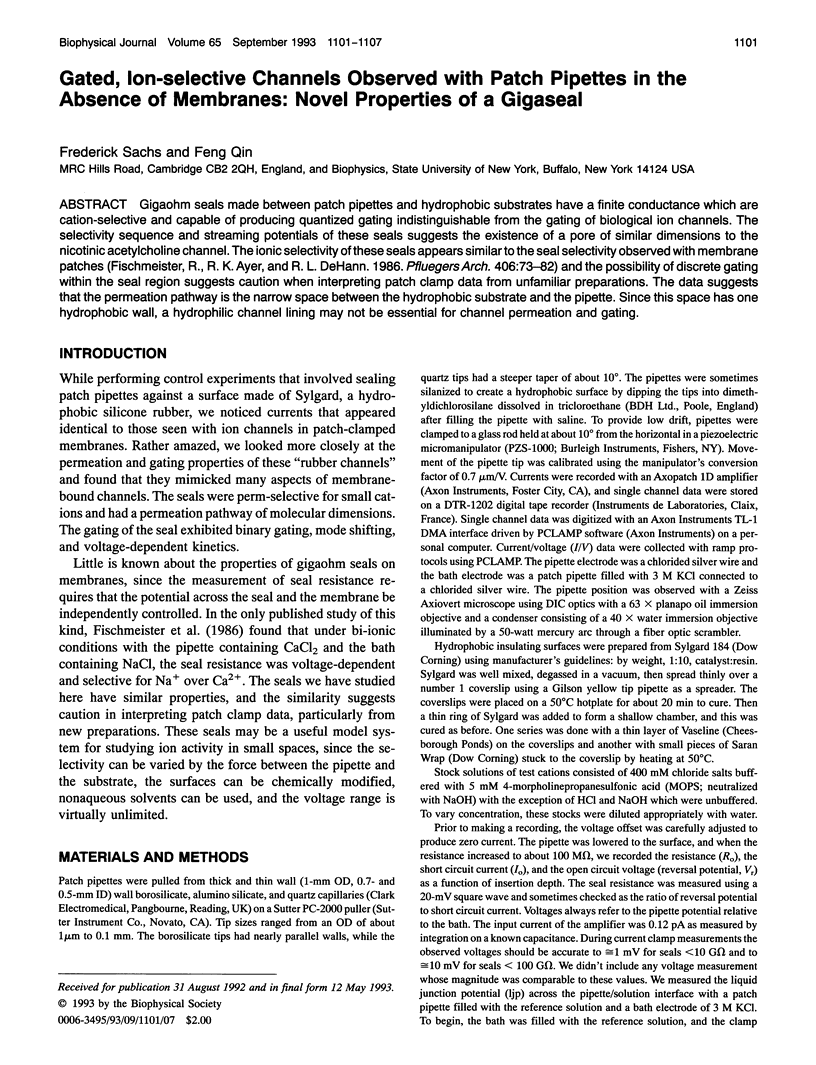
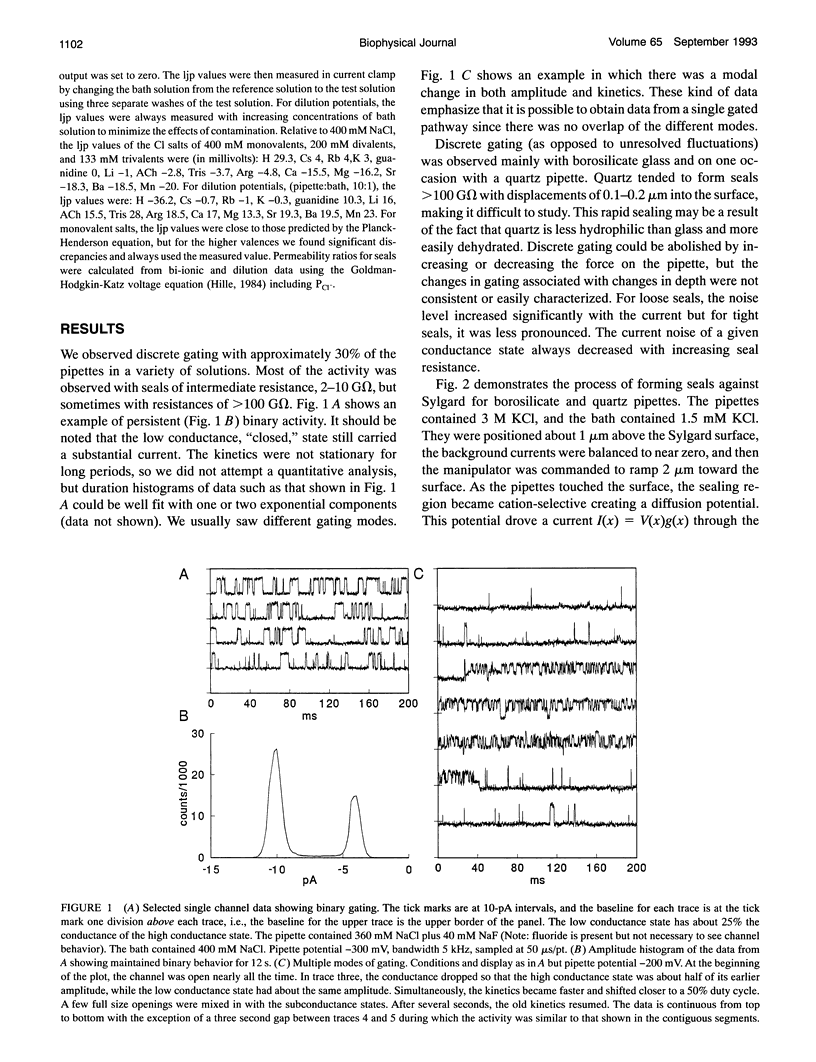
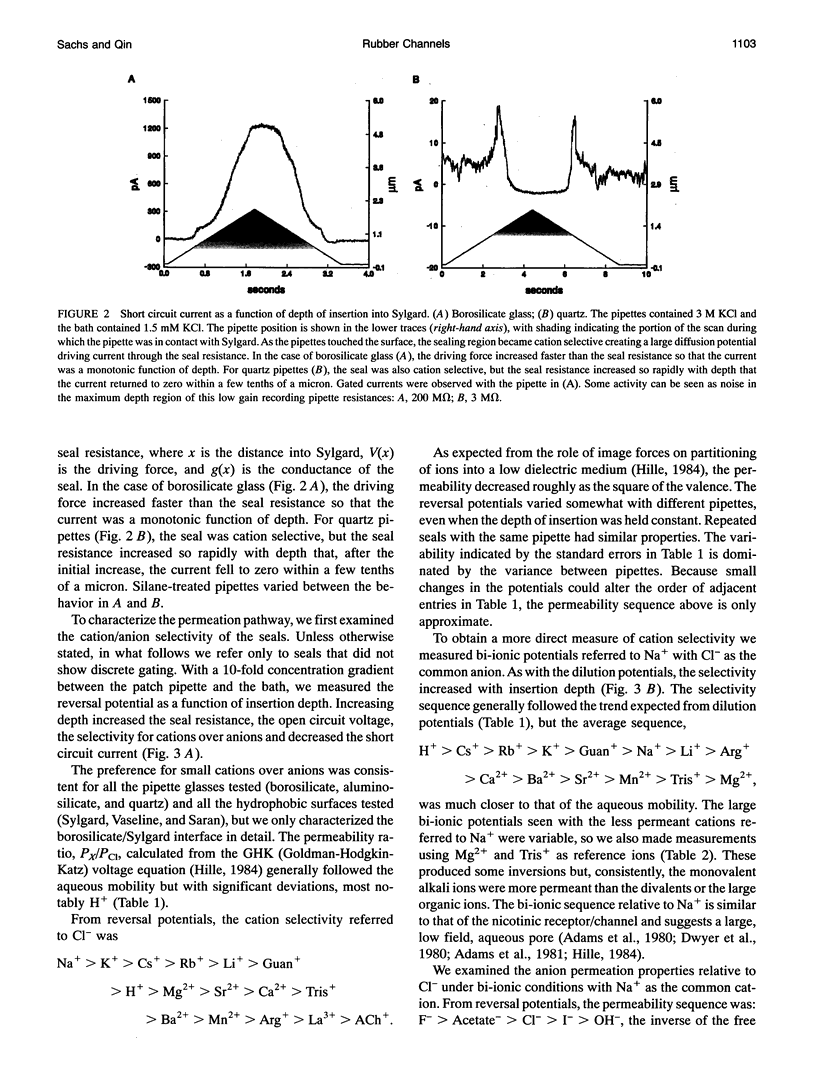
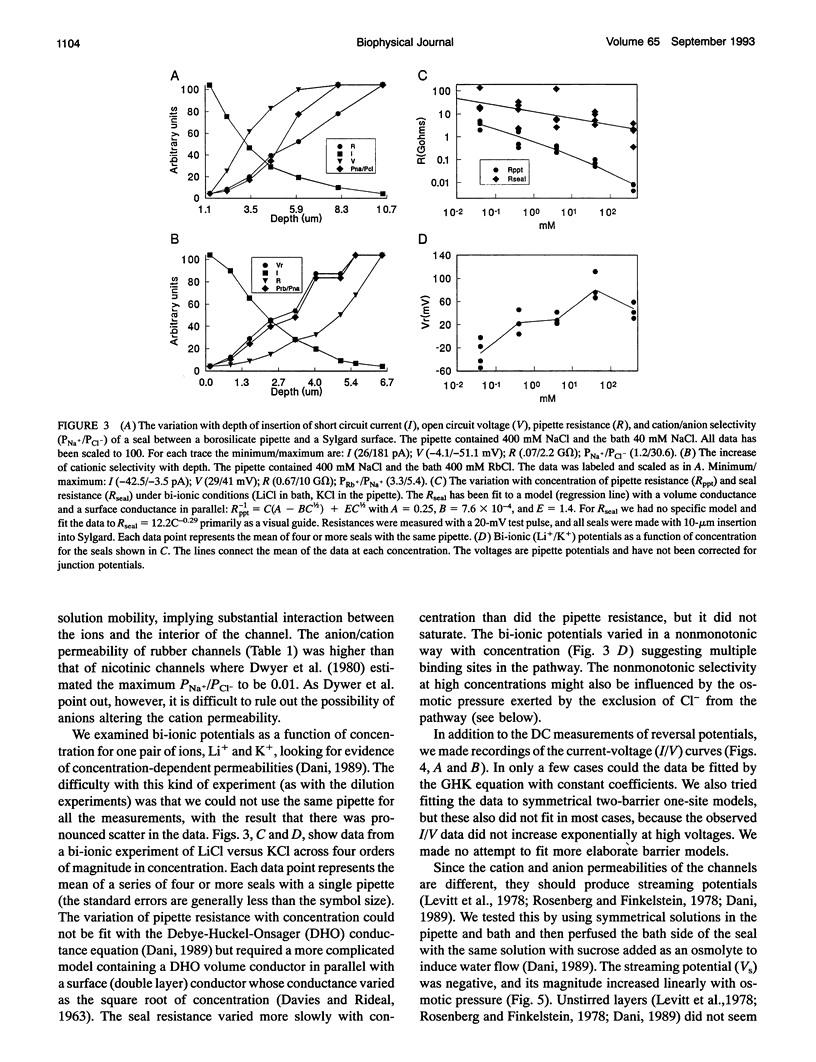
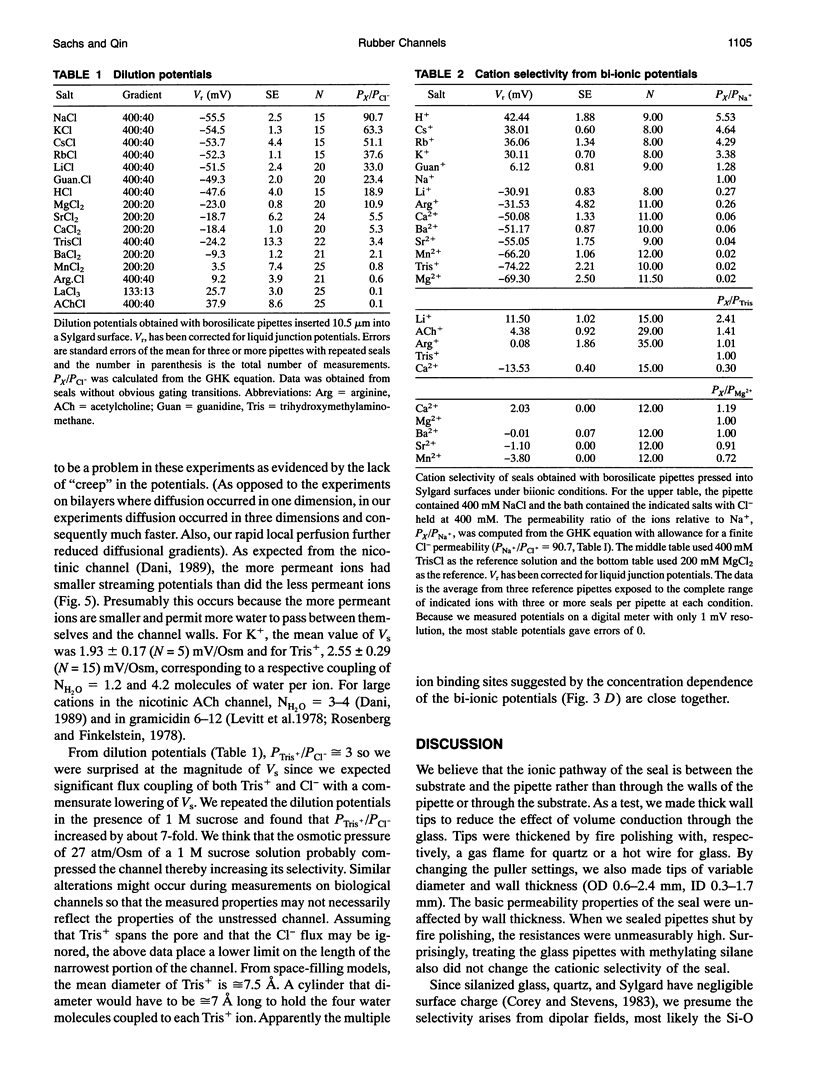
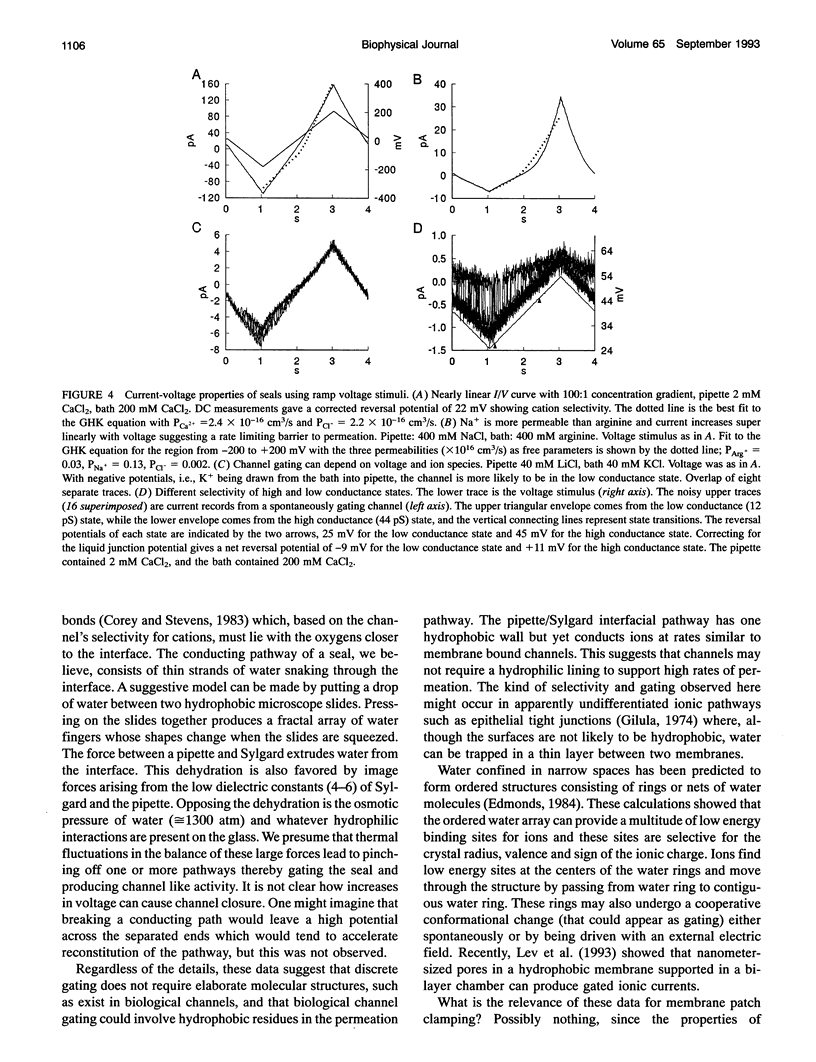
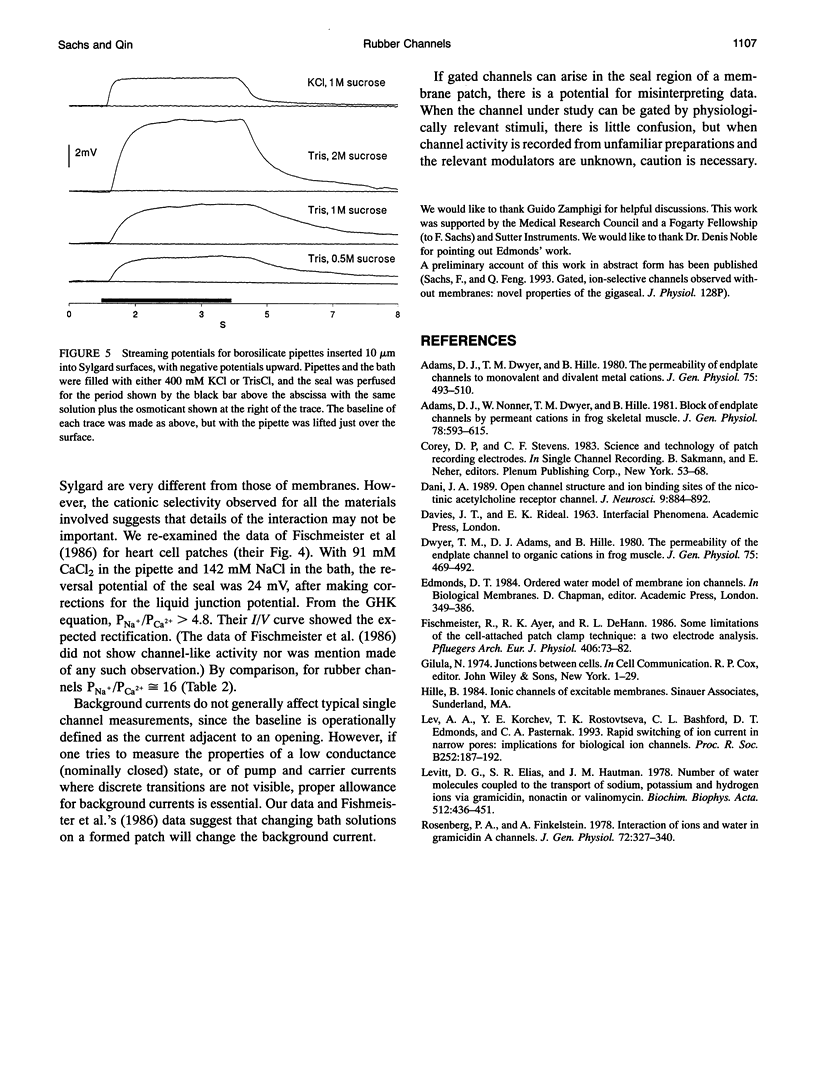
Images in this article
Selected References
These references are in PubMed. This may not be the complete list of references from this article.
- Adams D. J., Dwyer T. M., Hille B. The permeability of endplate channels to monovalent and divalent metal cations. J Gen Physiol. 1980 May;75(5):493–510. doi: 10.1085/jgp.75.5.493. [DOI] [PMC free article] [PubMed] [Google Scholar]
- Adams D. J., Nonner W., Dwyer T. M., Hille B. Block of endplate channels by permeant cations in frog skeletal muscle. J Gen Physiol. 1981 Dec;78(6):593–615. doi: 10.1085/jgp.78.6.593. [DOI] [PMC free article] [PubMed] [Google Scholar]
- Dani J. A. Open channel structure and ion binding sites of the nicotinic acetylcholine receptor channel. J Neurosci. 1989 Mar;9(3):884–892. doi: 10.1523/JNEUROSCI.09-03-00884.1989. [DOI] [PMC free article] [PubMed] [Google Scholar]
- Dwyer T. M., Adams D. J., Hille B. The permeability of the endplate channel to organic cations in frog muscle. J Gen Physiol. 1980 May;75(5):469–492. doi: 10.1085/jgp.75.5.469. [DOI] [PMC free article] [PubMed] [Google Scholar]
- Fischmeister R., Ayer R. K., Jr, DeHaan R. L. Some limitations of the cell-attached patch clamp technique: a two-electrode analysis. Pflugers Arch. 1986 Jan;406(1):73–82. doi: 10.1007/BF00582957. [DOI] [PubMed] [Google Scholar]
- Lev A. A., Korchev Y. E., Rostovtseva T. K., Bashford C. L., Edmonds D. T., Pasternak C. A. Rapid switching of ion current in narrow pores: implications for biological ion channels. Proc Biol Sci. 1993 Jun 22;252(1335):187–192. doi: 10.1098/rspb.1993.0064. [DOI] [PubMed] [Google Scholar]
- Levitt D. G., Elias S. R., Hautman J. M. Number of water molecules coupled to the transport of sodium, potassium and hydrogen ions via gramicidin, nonactin or valinomycin. Biochim Biophys Acta. 1978 Sep 22;512(2):436–451. doi: 10.1016/0005-2736(78)90266-3. [DOI] [PubMed] [Google Scholar]
- Rosenberg P. A., Finkelstein A. Interaction of ions and water in gramicidin A channels: streaming potentials across lipid bilayer membranes. J Gen Physiol. 1978 Sep;72(3):327–340. doi: 10.1085/jgp.72.3.327. [DOI] [PMC free article] [PubMed] [Google Scholar]