Abstract
We report on an extension of a previously described method to measure the hydrodynamic force to separate doublets of fixed, sphered and swollen red cells cross-linked by antibody (S. P. Tha, J. Shuster, and H. L. Goldsmith. 1986. Biophys. J. 50:1117-1126). With a traveling microtube apparatus, doublets are tracked and videotaped in a slowly accelerating Poiseuille flow in 150-microns-diameter tubes, and the hydrodynamic normal force at break-up, Fn, is computed from the measured doublet velocity and radial position. Previous results showed a large range of Fn, the mean of which increased with [antiserum], and an absence of clustering at discrete values of Fn. Since it was assumed that the cells separate the instant a critical force to break all crossbridges was reached, lack of clustering could have been due to the use of a polyclonal antiserum. We therefore studied the effect of monoclonal IgM or IgA antibody on the distribution of Fn. The results showed that the data are as scattered as ever, with Fn varying from 2 to 200 pN, and exhibit no evidence of clustering. However, the scatter in Fn could be due to the stochastic nature of intercellular bonds (E. Evans, D. Berk, and A. Leung. 1991a. Biophys. J. 59:838-848). We therefore studied the force dependence of the time to break-up under constant shear stress (Fn from 30 to 200 pN), both in Poiseuille and Couette flow, the latter by using a counter-rotating cone and plate rheoscope. When 280 doublets were rapidly accelerated in the traveling microtube and then allowed to coast in steady flow for up to 180 s, 91% survived into the constant force region; 16% of these broke up after time intervals, tP, of 2-30s. Of 340 doublets immediately exposed to constant shear in the rheoscope, 37% broke after time intervals, tc, from < 1 to 10 s. Thus, doublets do indeed break up under a constant shear stress, if given time. The average time to break-up decreased significantly with increasing force, while the fraction of doublets broken up increased. At a given Fn, the fraction of break-ups decreased with increasing [IgM], suggesting that the average number of bonds had also increased. Using a stochastic model of break-up (G. I. Bell. 1978. Science (Washington DC). 200:618-627; E. Evans, D. Berk,and A. Leung. 1991. Biophys. J. 59:838-848) and a Poisson distribution for the number of bonds, Nb, break-up in slowly accelerating Poiseuille flow and in immediate shear application in Couette flow was simulated. In Poiseuille flow, the observed range and scatter in Fn could be reproduced assuming (Nb) > 5. In the rheoscope, the time intervals and number of rotations to break-up, tc, were quite well reproduced assuming (Nb) = 4. The similarity of (Fn) for monoclonal IgM and IgA for doublet break-up under constant slow acceleration is compatible with the conclusion of Evans et al. (1991 a) for normal red cells and Xia et al. (manuscript submitted for publication) for sphered and swollen red cells, that the applied force extracts the antigen from the cell membrane.
Full text
PDF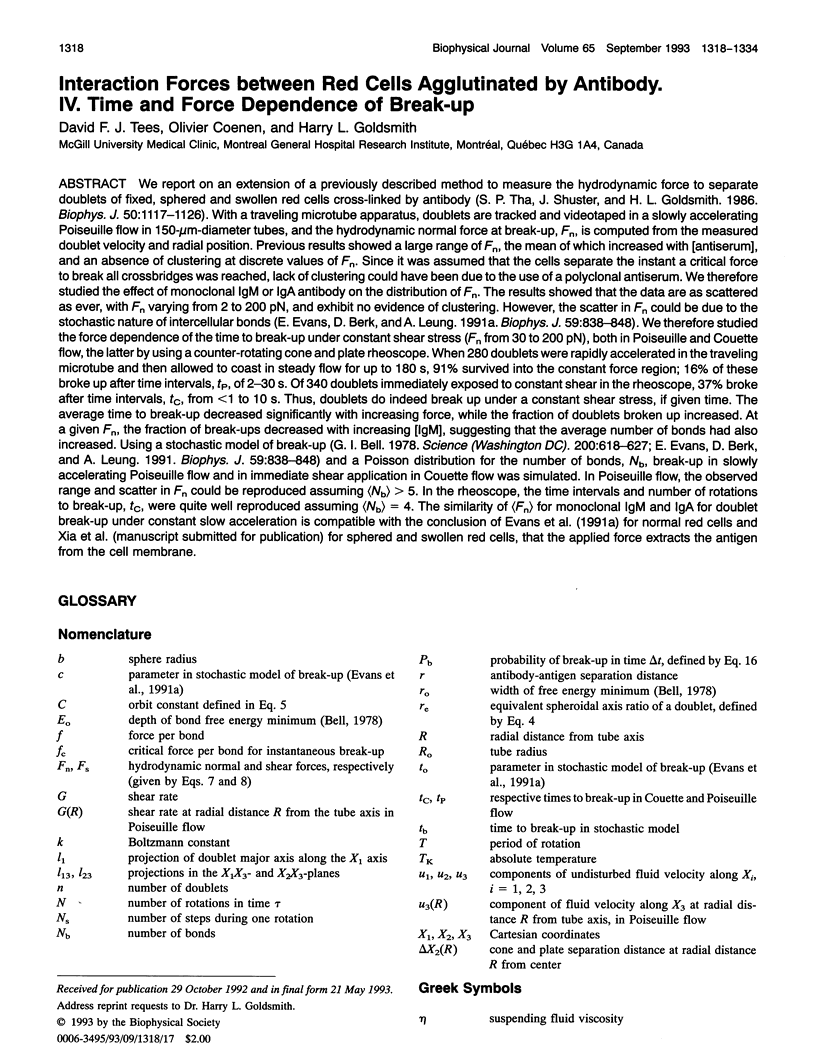
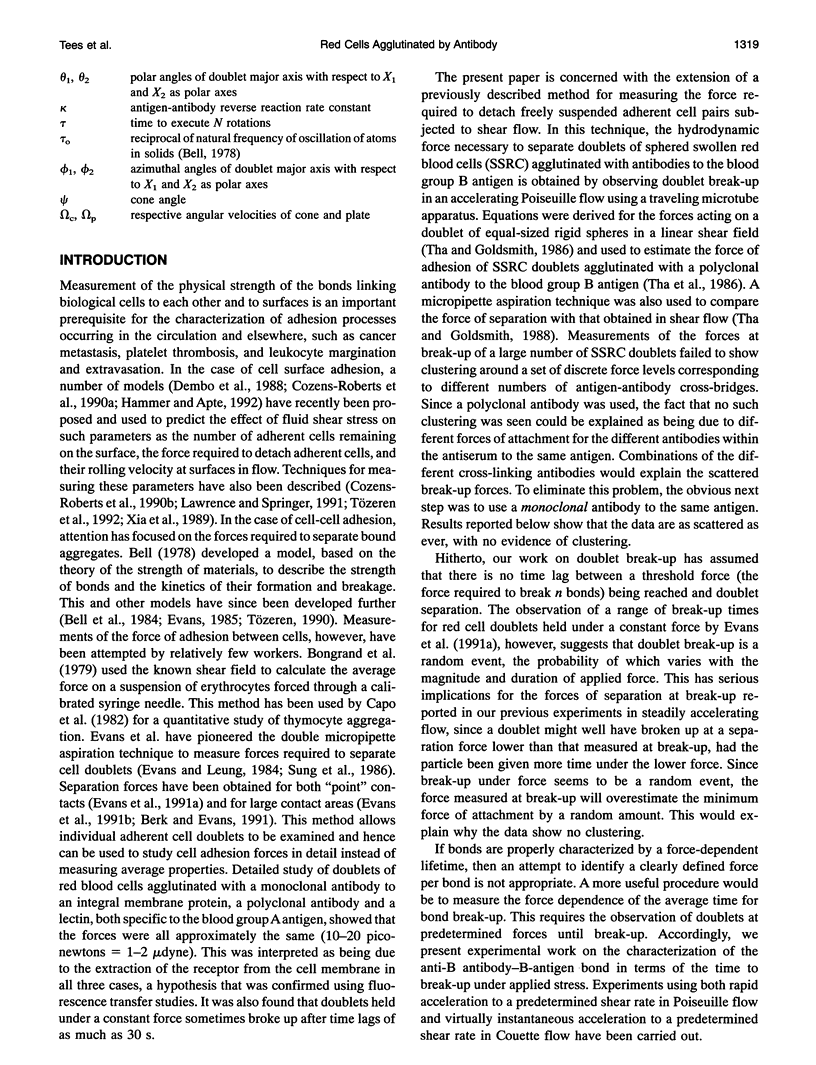
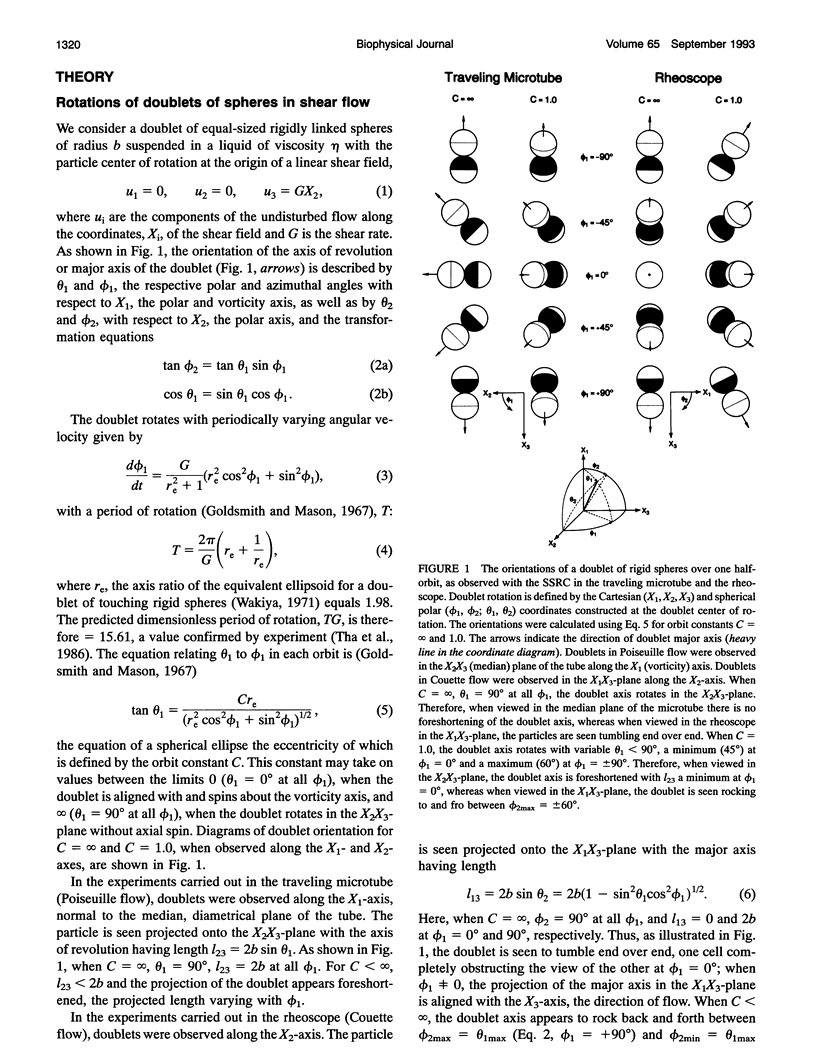
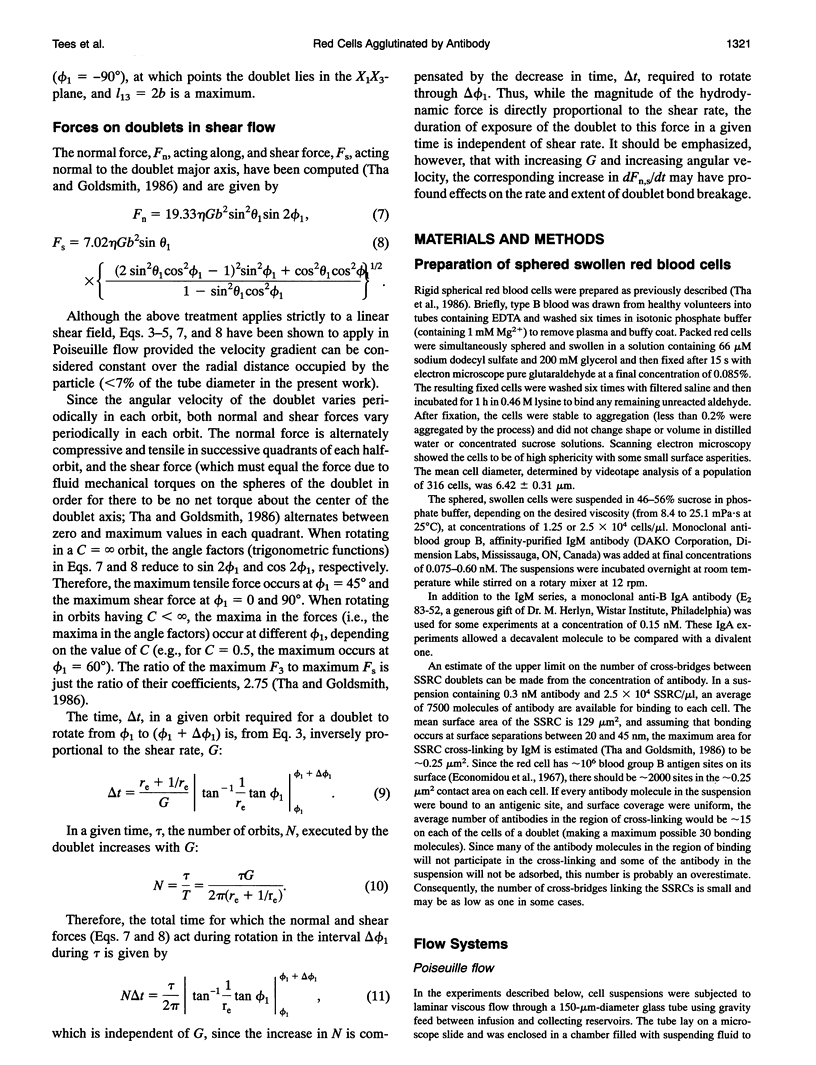
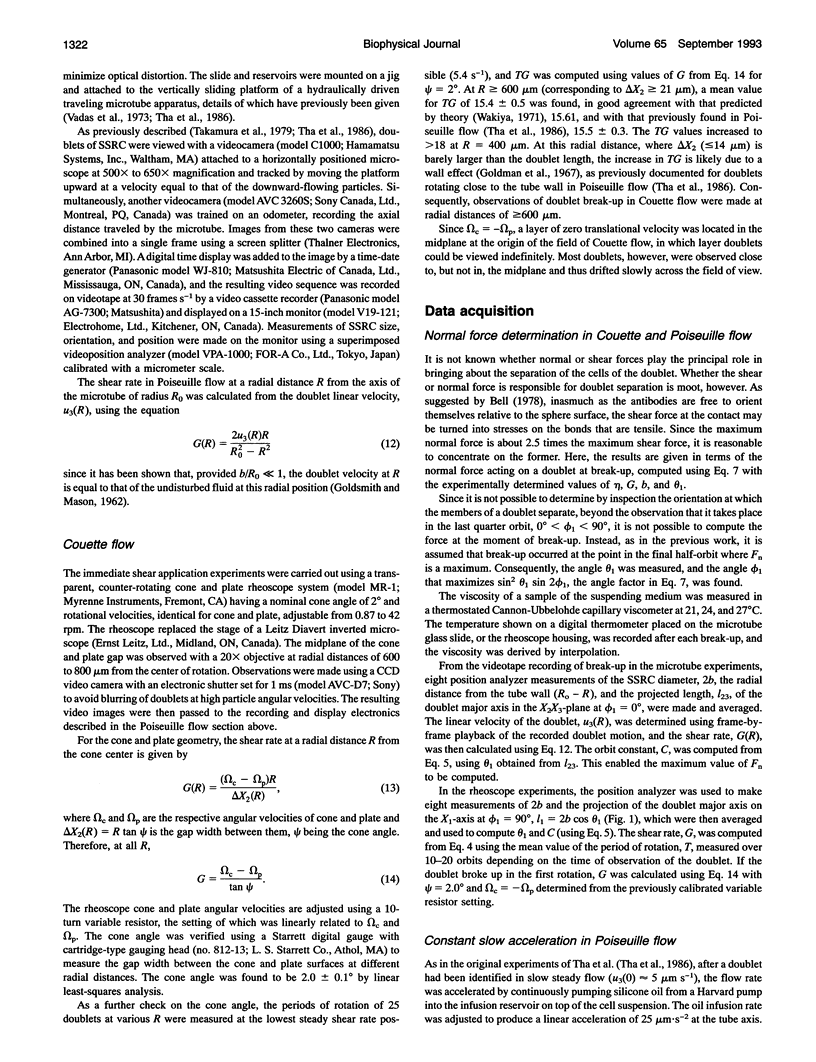
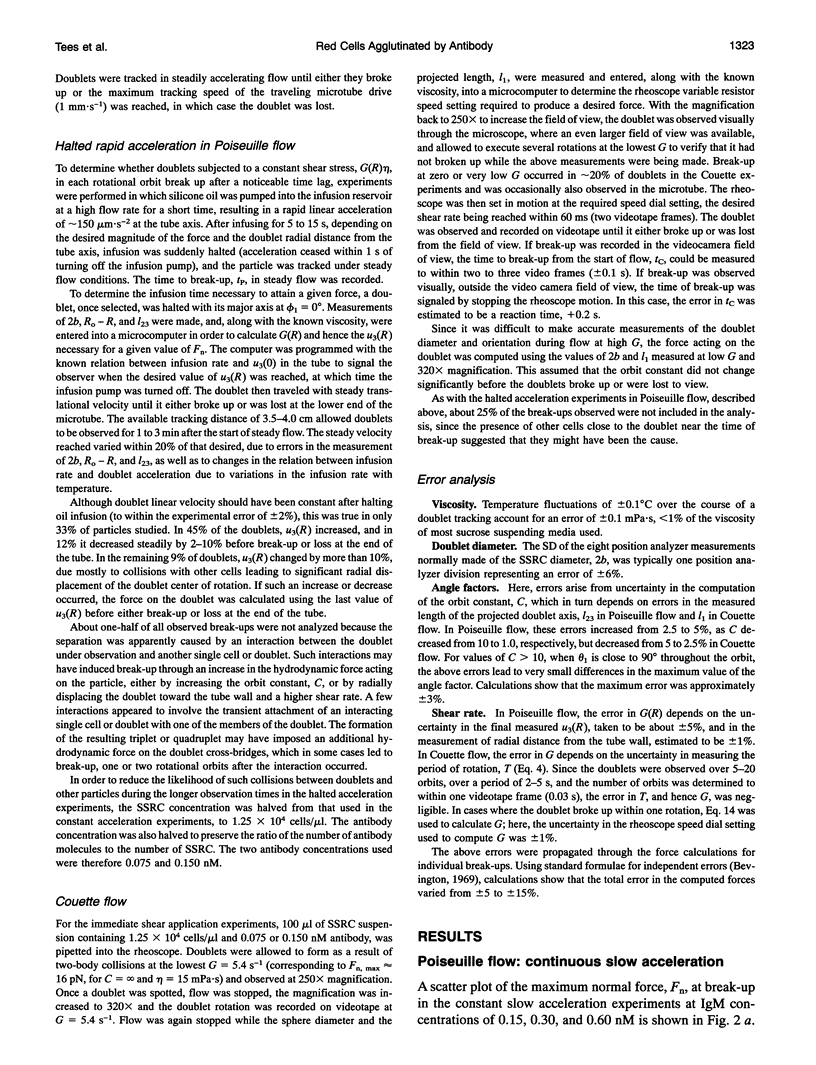
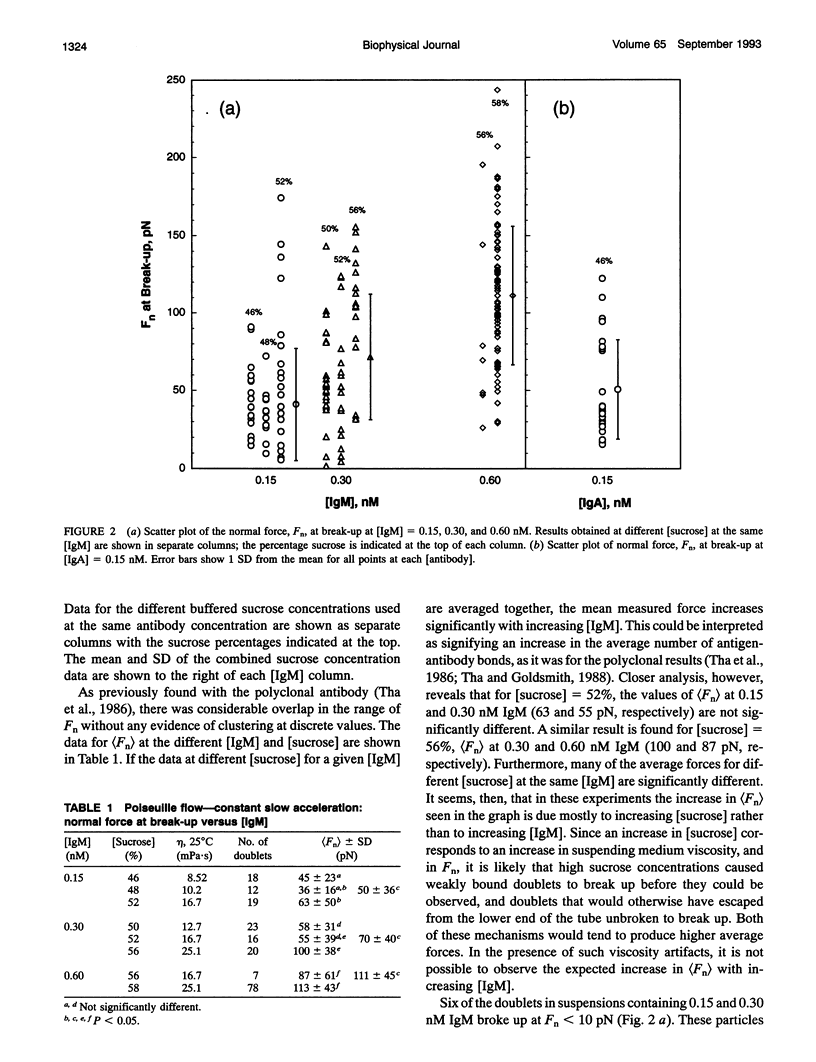
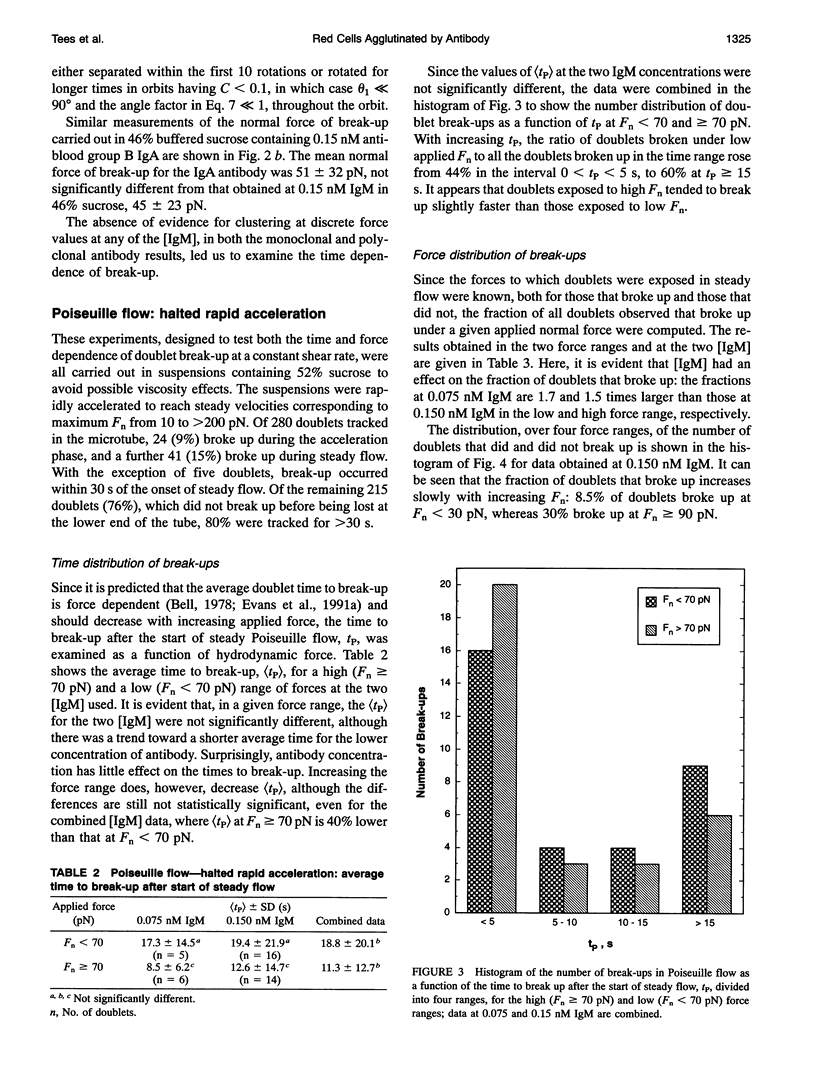
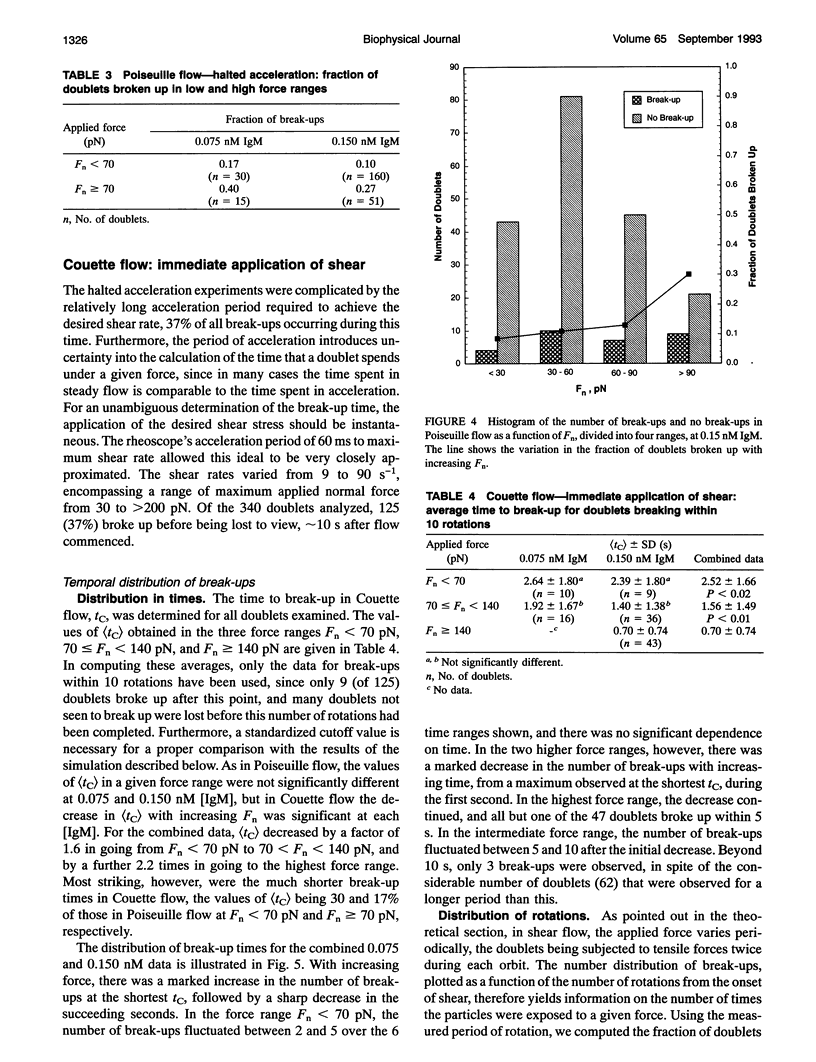
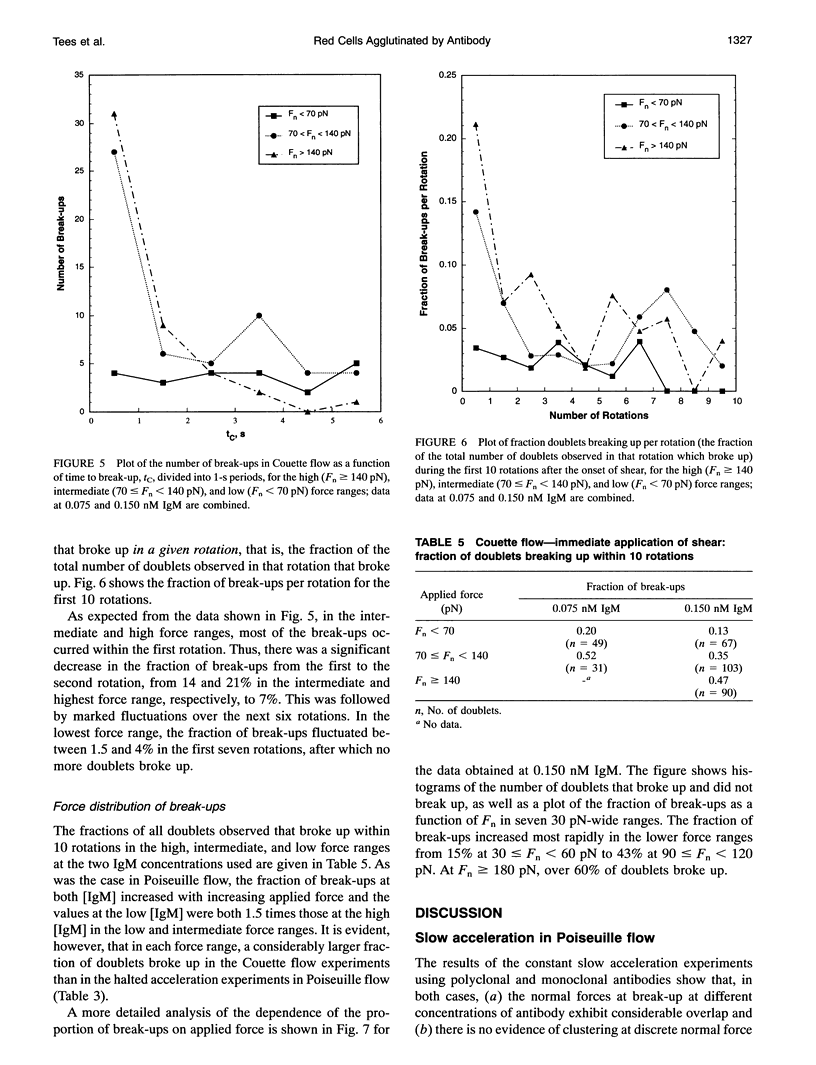
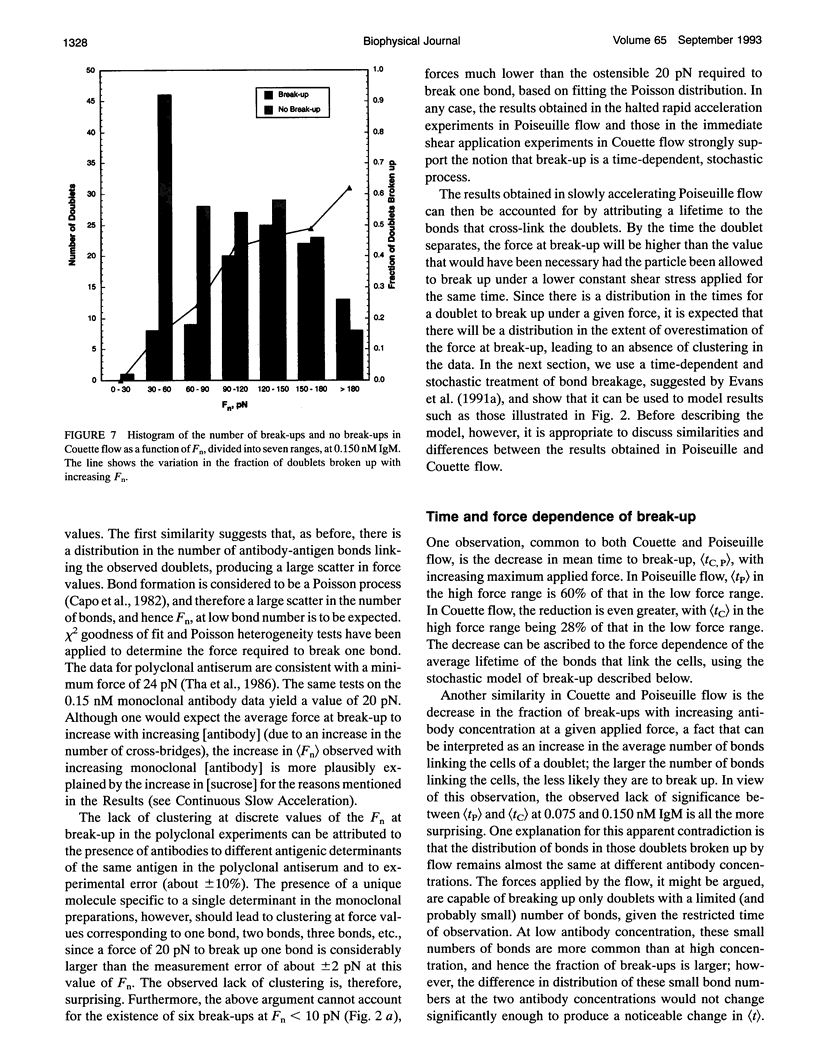
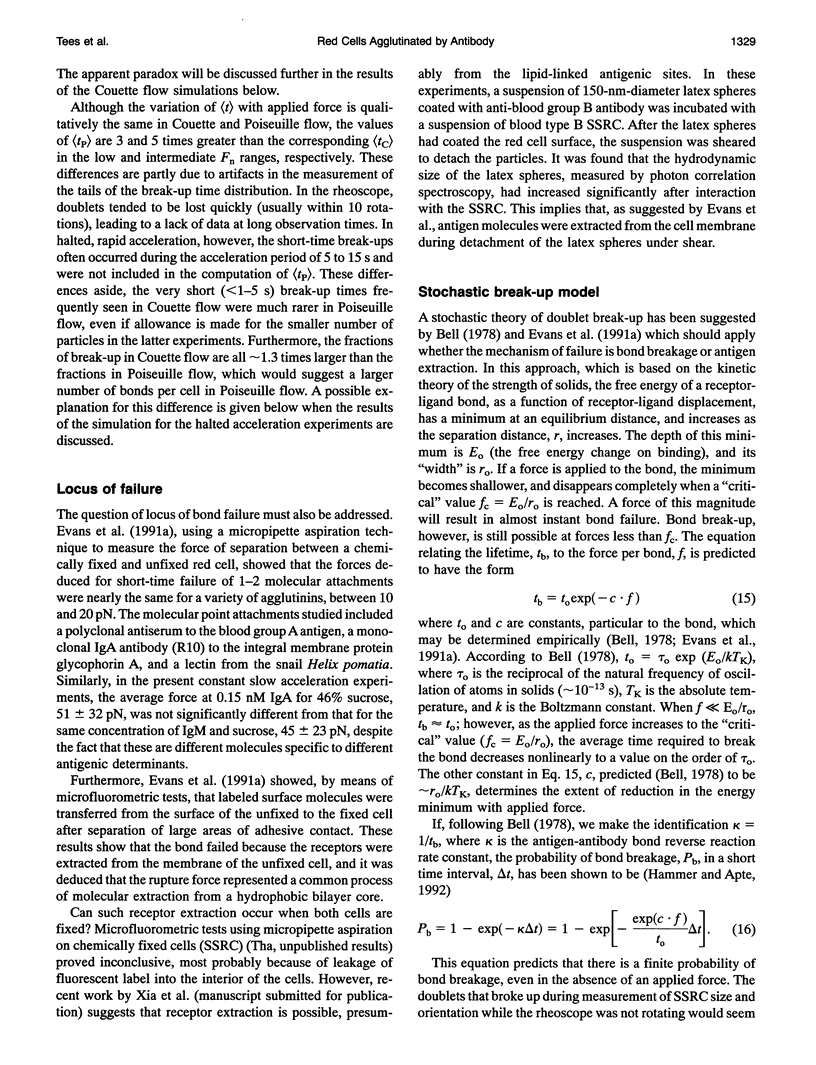
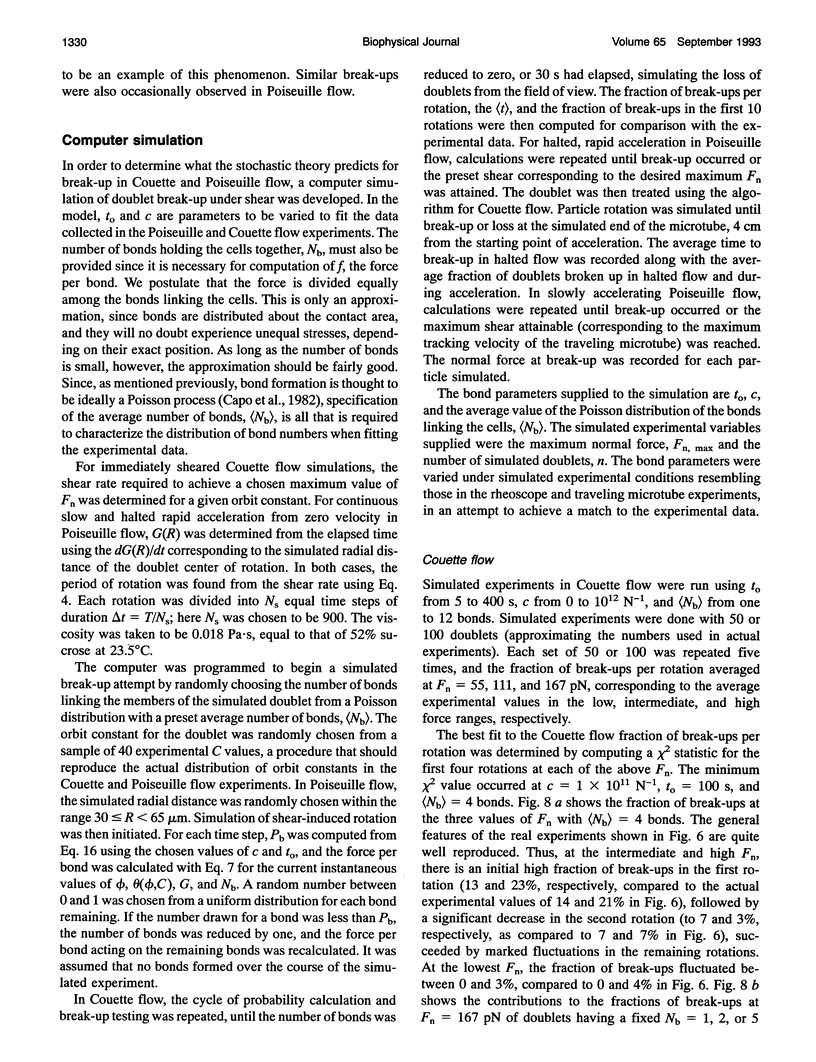
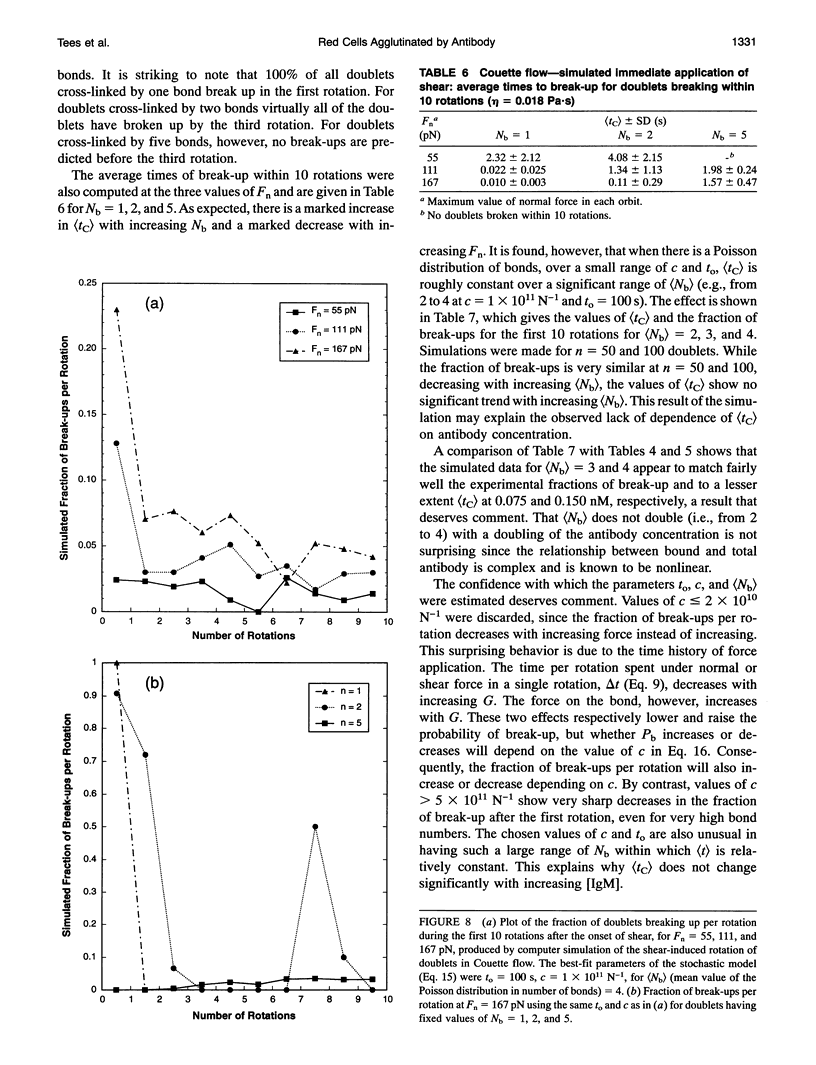
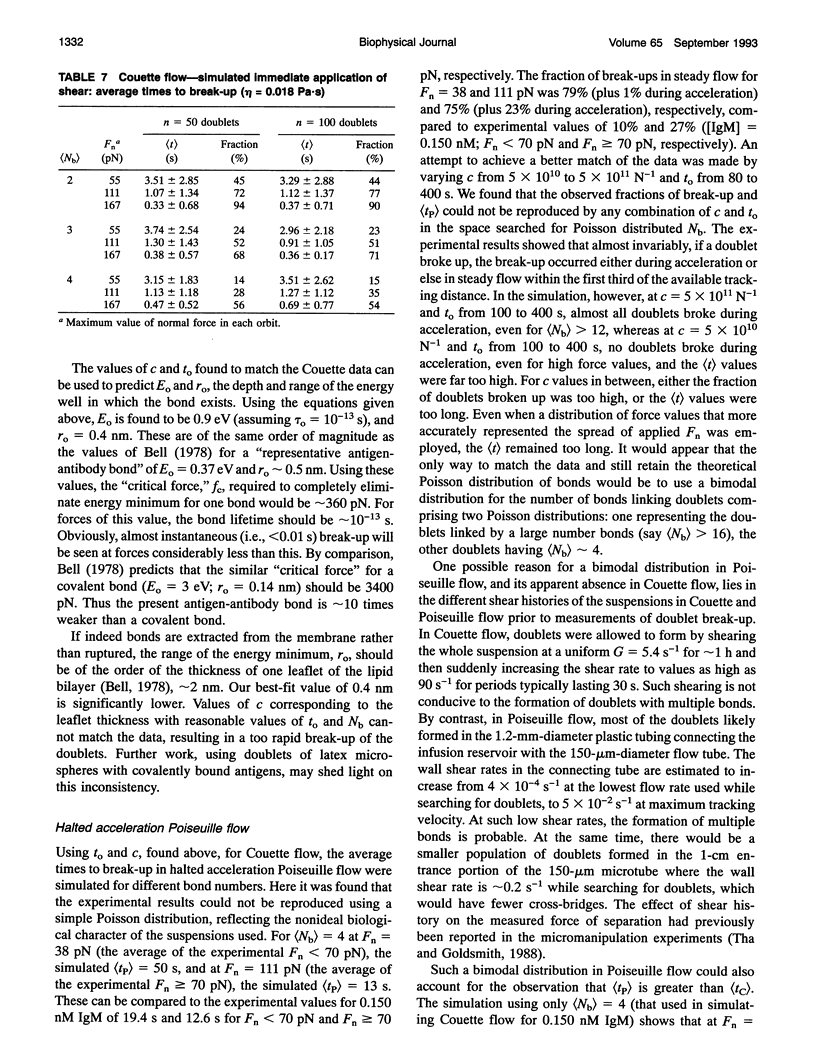
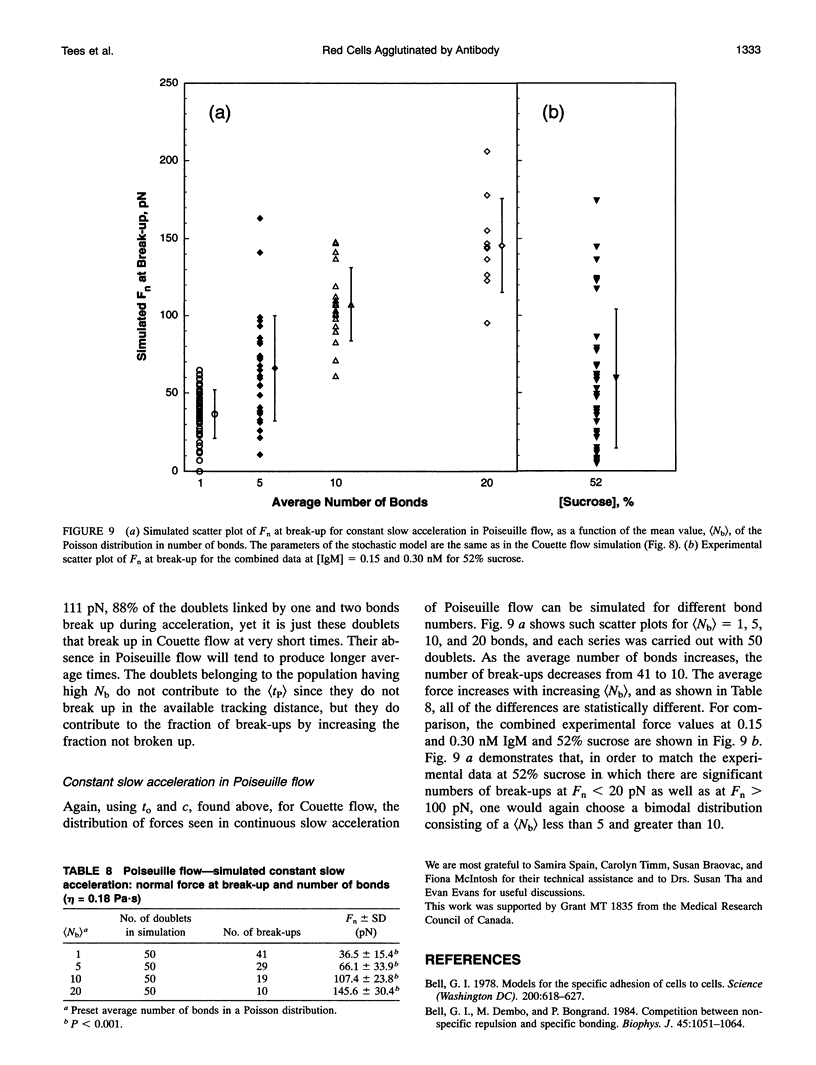
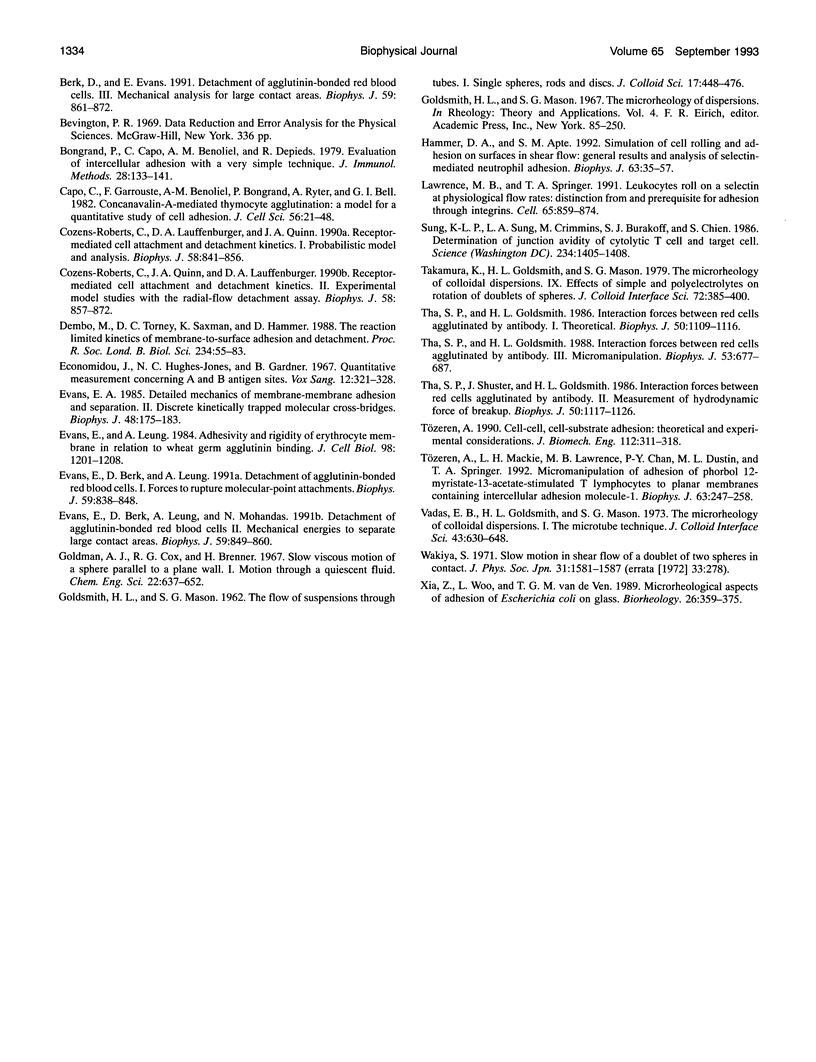
Images in this article
Selected References
These references are in PubMed. This may not be the complete list of references from this article.
- Bell G. I., Dembo M., Bongrand P. Cell adhesion. Competition between nonspecific repulsion and specific bonding. Biophys J. 1984 Jun;45(6):1051–1064. doi: 10.1016/S0006-3495(84)84252-6. [DOI] [PMC free article] [PubMed] [Google Scholar]
- Bell G. I. Models for the specific adhesion of cells to cells. Science. 1978 May 12;200(4342):618–627. doi: 10.1126/science.347575. [DOI] [PubMed] [Google Scholar]
- Berk D., Evans E. Detachment of agglutinin-bonded red blood cells. III. Mechanical analysis for large contact areas. Biophys J. 1991 Apr;59(4):861–872. doi: 10.1016/S0006-3495(91)82298-6. [DOI] [PMC free article] [PubMed] [Google Scholar]
- Bongrand P., Capo C., Benoliel A. M., Depieds R. Evaluation of intercellular adhesion with a very simple technique. J Immunol Methods. 1979;28(1-2):133–141. doi: 10.1016/0022-1759(79)90335-1. [DOI] [PubMed] [Google Scholar]
- Capo C., Garrouste F., Benoliel A. M., Bongrand P., Ryter A., Bell G. I. Concanavalin-A-mediated thymocyte agglutination: a model for a quantitative study of cell adhesion. J Cell Sci. 1982 Aug;56:21–48. doi: 10.1242/jcs.56.1.21. [DOI] [PubMed] [Google Scholar]
- Cozens-Roberts C., Lauffenburger D. A., Quinn J. A. Receptor-mediated cell attachment and detachment kinetics. I. Probabilistic model and analysis. Biophys J. 1990 Oct;58(4):841–856. doi: 10.1016/S0006-3495(90)82430-9. [DOI] [PMC free article] [PubMed] [Google Scholar]
- Cozens-Roberts C., Quinn J. A., Lauffenburger D. A. Receptor-mediated cell attachment and detachment kinetics. II. Experimental model studies with the radial-flow detachment assay. Biophys J. 1990 Oct;58(4):857–872. doi: 10.1016/S0006-3495(90)82431-0. [DOI] [PMC free article] [PubMed] [Google Scholar]
- Dembo M., Torney D. C., Saxman K., Hammer D. The reaction-limited kinetics of membrane-to-surface adhesion and detachment. Proc R Soc Lond B Biol Sci. 1988 Jun 22;234(1274):55–83. doi: 10.1098/rspb.1988.0038. [DOI] [PubMed] [Google Scholar]
- Economidou J., Hughes-Jones N. C., Gardner B. Quantitative measurements concerning A and B antigen sites. Vox Sang. 1967 May;12(5):321–328. doi: 10.1111/j.1423-0410.1967.tb03362.x. [DOI] [PubMed] [Google Scholar]
- Evans E. A. Detailed mechanics of membrane-membrane adhesion and separation. I. Continuum of molecular cross-bridges. Biophys J. 1985 Jul;48(1):175–183. doi: 10.1016/S0006-3495(85)83770-X. [DOI] [PMC free article] [PubMed] [Google Scholar]
- Evans E., Berk D., Leung A. Detachment of agglutinin-bonded red blood cells. I. Forces to rupture molecular-point attachments. Biophys J. 1991 Apr;59(4):838–848. doi: 10.1016/S0006-3495(91)82296-2. [DOI] [PMC free article] [PubMed] [Google Scholar]
- Evans E., Berk D., Leung A., Mohandas N. Detachment of agglutinin-bonded red blood cells. II. Mechanical energies to separate large contact areas. Biophys J. 1991 Apr;59(4):849–860. doi: 10.1016/S0006-3495(91)82297-4. [DOI] [PMC free article] [PubMed] [Google Scholar]
- Evans E., Leung A. Adhesivity and rigidity of erythrocyte membrane in relation to wheat germ agglutinin binding. J Cell Biol. 1984 Apr;98(4):1201–1208. doi: 10.1083/jcb.98.4.1201. [DOI] [PMC free article] [PubMed] [Google Scholar]
- Hammer D. A., Apte S. M. Simulation of cell rolling and adhesion on surfaces in shear flow: general results and analysis of selectin-mediated neutrophil adhesion. Biophys J. 1992 Jul;63(1):35–57. doi: 10.1016/S0006-3495(92)81577-1. [DOI] [PMC free article] [PubMed] [Google Scholar]
- Lawrence M. B., Springer T. A. Leukocytes roll on a selectin at physiologic flow rates: distinction from and prerequisite for adhesion through integrins. Cell. 1991 May 31;65(5):859–873. doi: 10.1016/0092-8674(91)90393-d. [DOI] [PubMed] [Google Scholar]
- Sung K. L., Sung L. A., Crimmins M., Burakoff S. J., Chien S. Determination of junction avidity of cytolytic T cell and target cell. Science. 1986 Dec 12;234(4782):1405–1408. doi: 10.1126/science.3491426. [DOI] [PubMed] [Google Scholar]
- Tha S. P., Goldsmith H. L. Interaction forces between red cells agglutinated by antibody. I. Theoretical. Biophys J. 1986 Dec;50(6):1109–1116. doi: 10.1016/S0006-3495(86)83555-X. [DOI] [PMC free article] [PubMed] [Google Scholar]
- Tha S. P., Goldsmith H. L. Interaction forces between red cells agglutinated by antibody. III. Micromanipulation. Biophys J. 1988 May;53(5):677–687. doi: 10.1016/S0006-3495(88)83149-7. [DOI] [PMC free article] [PubMed] [Google Scholar]
- Tha S. P., Shuster J., Goldsmith H. L. Interaction forces between red cells agglutinated by antibody. II. Measurement of hydrodynamic force of breakup. Biophys J. 1986 Dec;50(6):1117–1126. doi: 10.1016/S0006-3495(86)83556-1. [DOI] [PMC free article] [PubMed] [Google Scholar]
- Tozeren A. Cell-cell, cell-substrate adhesion: theoretical and experimental considerations. J Biomech Eng. 1990 Aug;112(3):311–318. doi: 10.1115/1.2891189. [DOI] [PubMed] [Google Scholar]
- Tözeren A., Mackie L. H., Lawrence M. B., Chan P. Y., Dustin M. L., Springer T. A. Micromanipulation of adhesion of phorbol 12-myristate-13-acetate-stimulated T lymphocytes to planar membranes containing intercellular adhesion molecule-1. Biophys J. 1992 Jul;63(1):247–258. doi: 10.1016/S0006-3495(92)81578-3. [DOI] [PMC free article] [PubMed] [Google Scholar]
- Xia Z., Woo L., van de Ven T. G. Microrheological aspects of adhesion of Escherichia coli on glass. Biorheology. 1989;26(2):359–375. doi: 10.3233/bir-1989-26219. [DOI] [PubMed] [Google Scholar]