Abstract
Actin, one of the most abundant intracellular proteins, forms long linear polyelectrolytic polymers in solution. A novel technique to handle single actin filaments in solution was developed that allows the study of ionic currents elicited along the surface of electrically stimulated actin filaments. Electrical currents were observed about the polymer's surface under both high (100 mM KCl) and low (1 mM KCl) ionic strength conditions. The data are consistent with a dynamic behavior of the counterionic cloud surrounding the actin filaments that support ionic movements along their longitudinal axis upon electrical stimulation. Counterionic waves were highly nonlinear in nature and remained long after the electrical stimulation of the actin filaments ceased. In this report therefore, we demonstrate that actin filaments can function as biological "electrical wires" and can thus be conceptualized as nonlinear inhomogeneous transmission lines. This ability of actin filaments to conduct electrical signals may have important implications in the coupling of intracellular signals.
Full text
PDF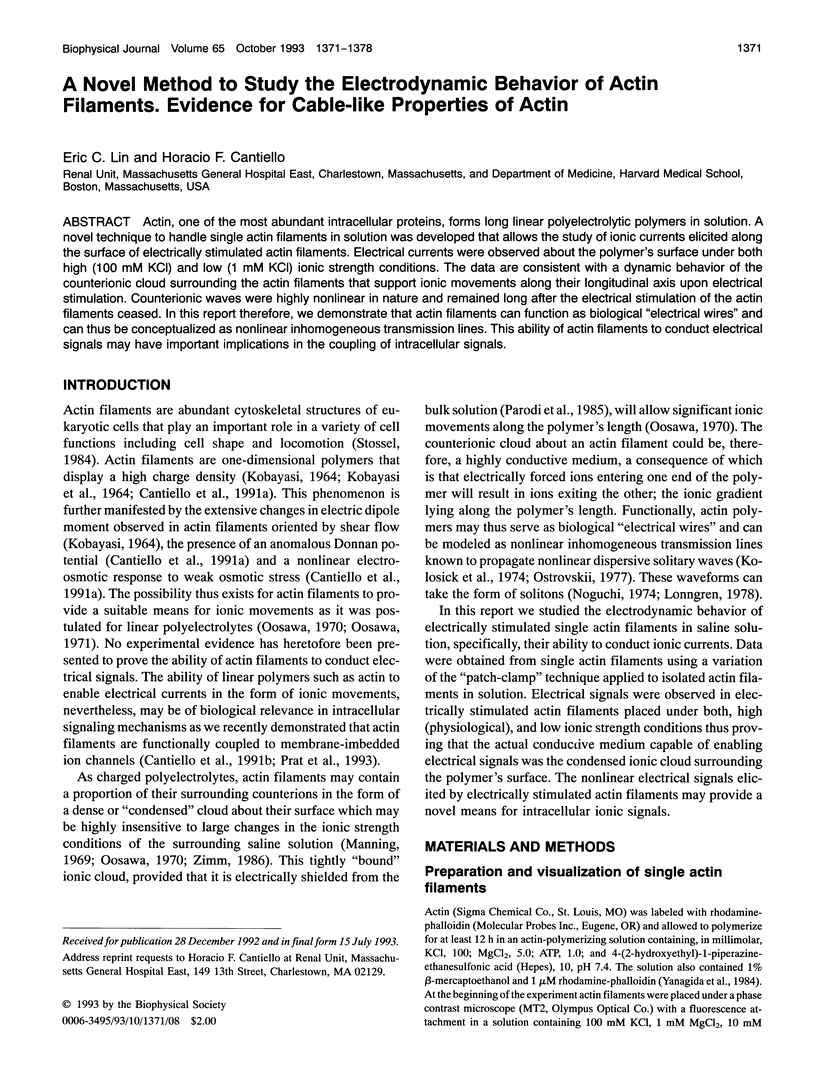
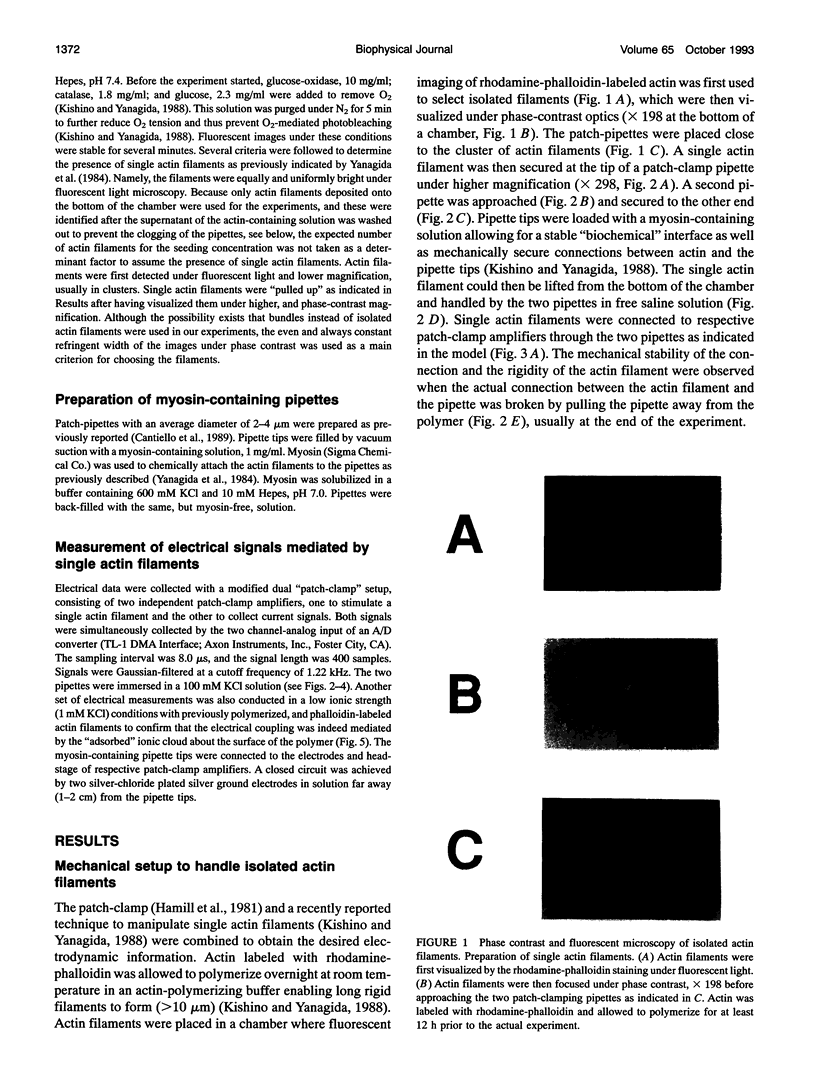
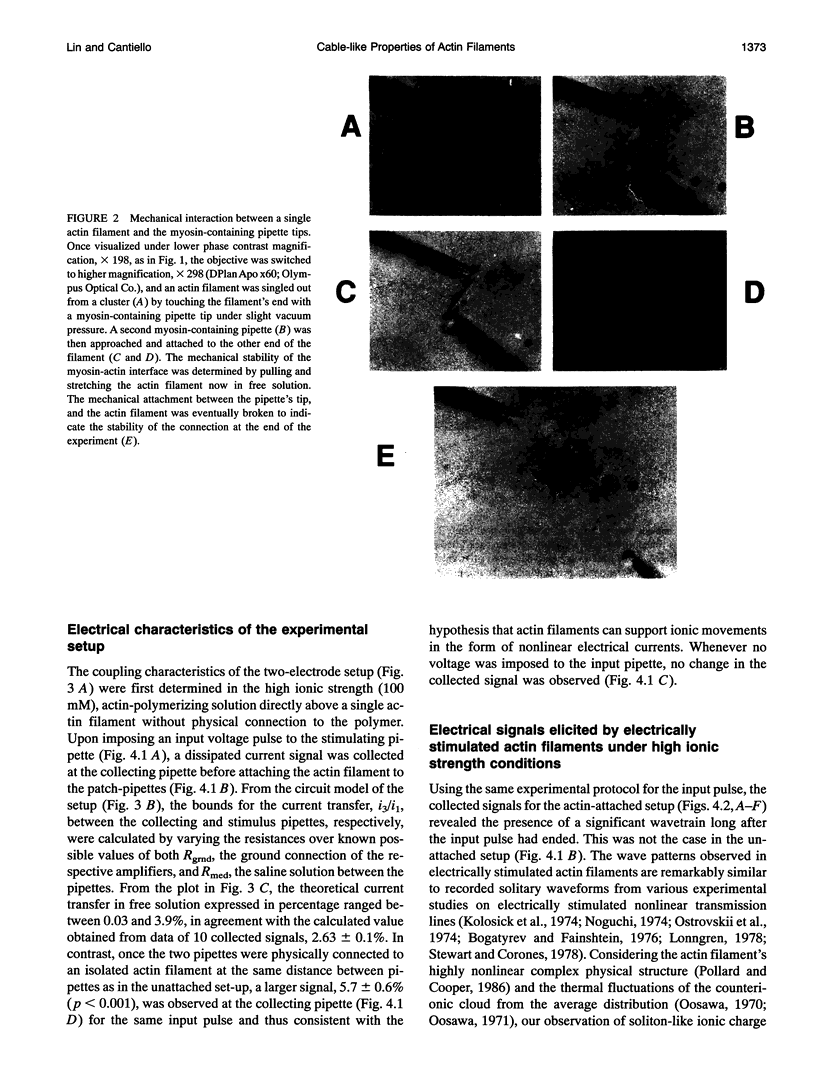
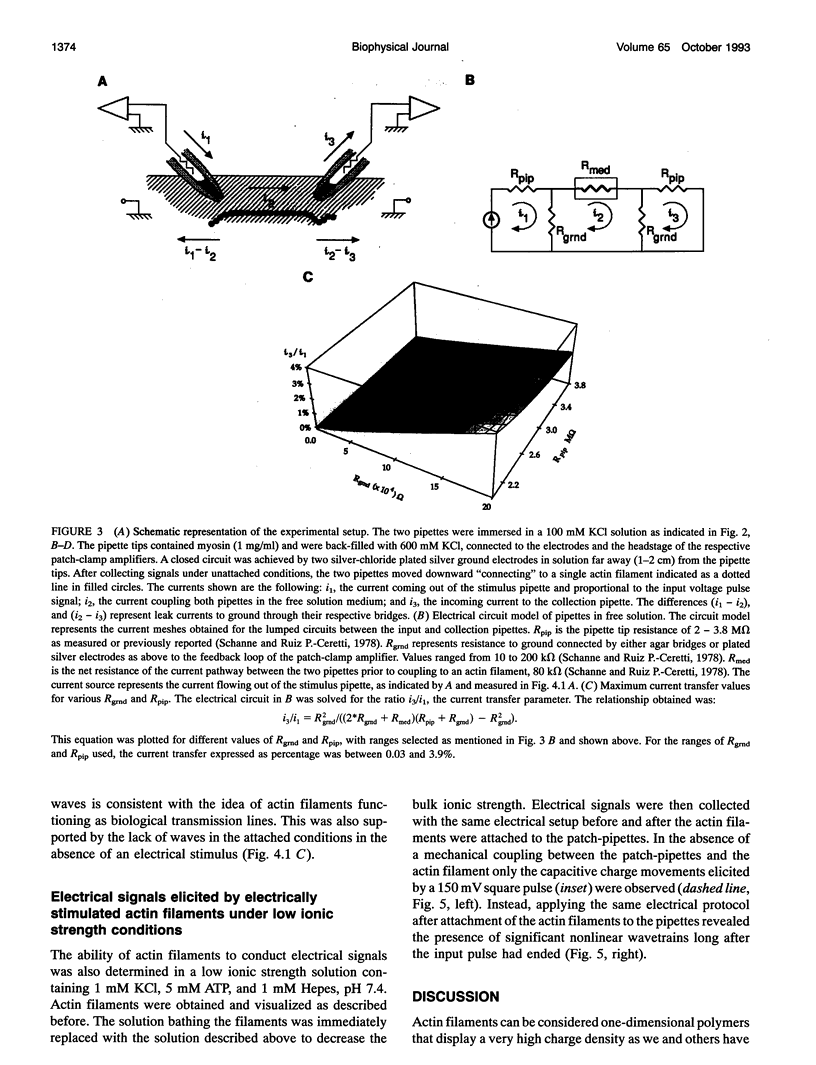
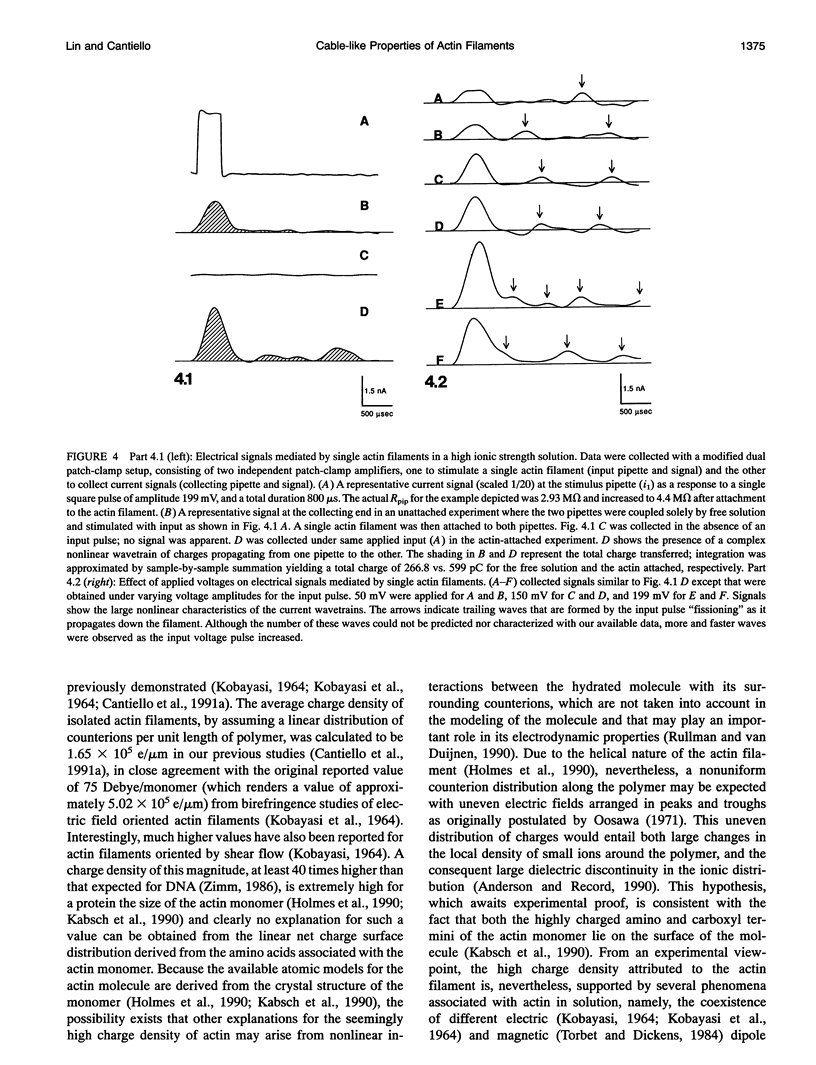
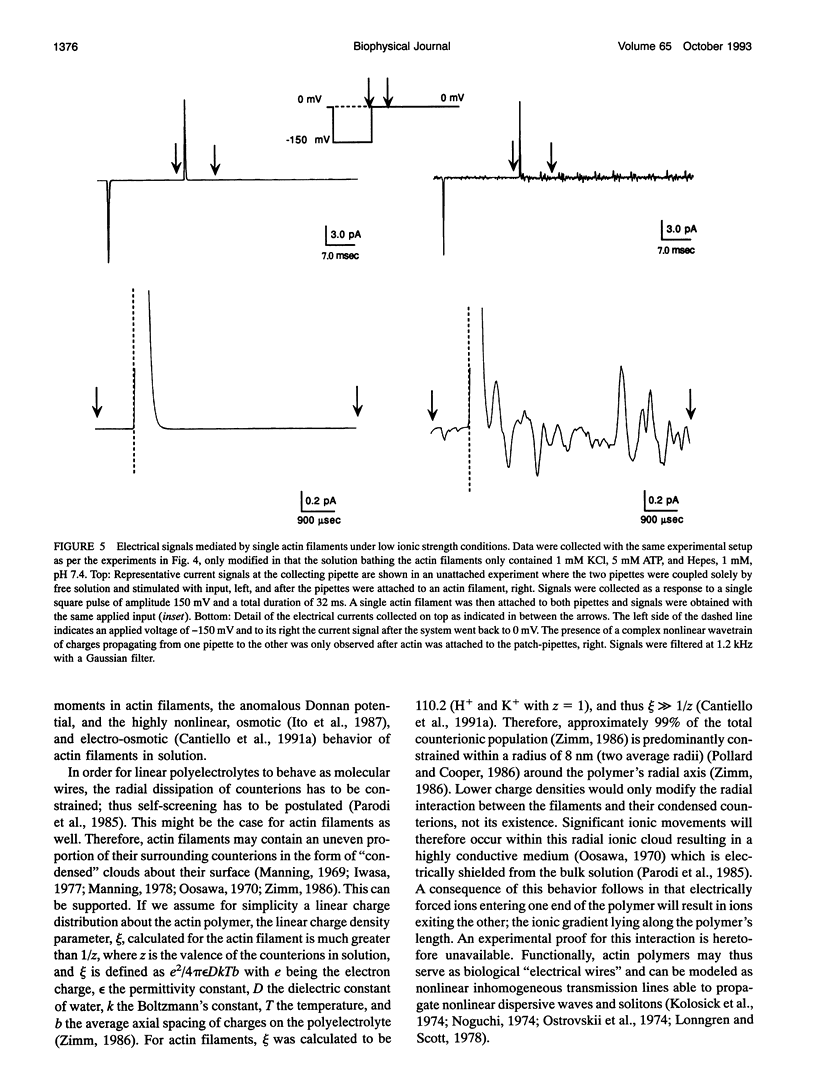
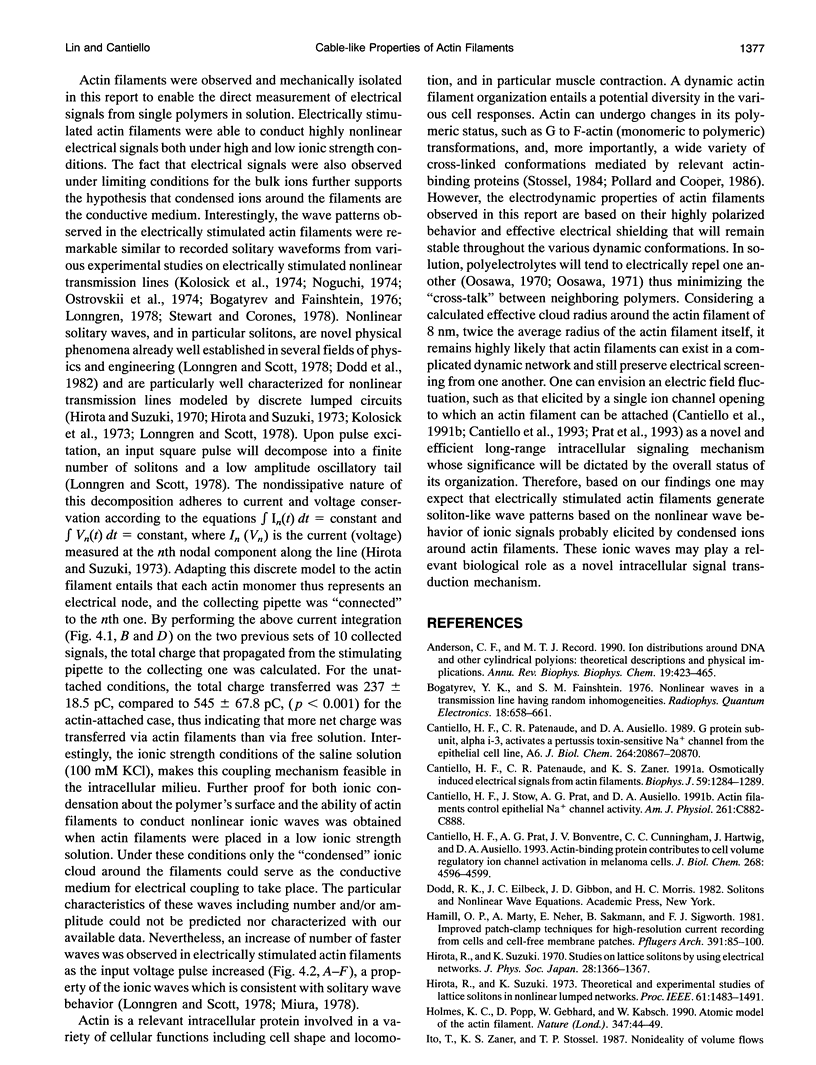
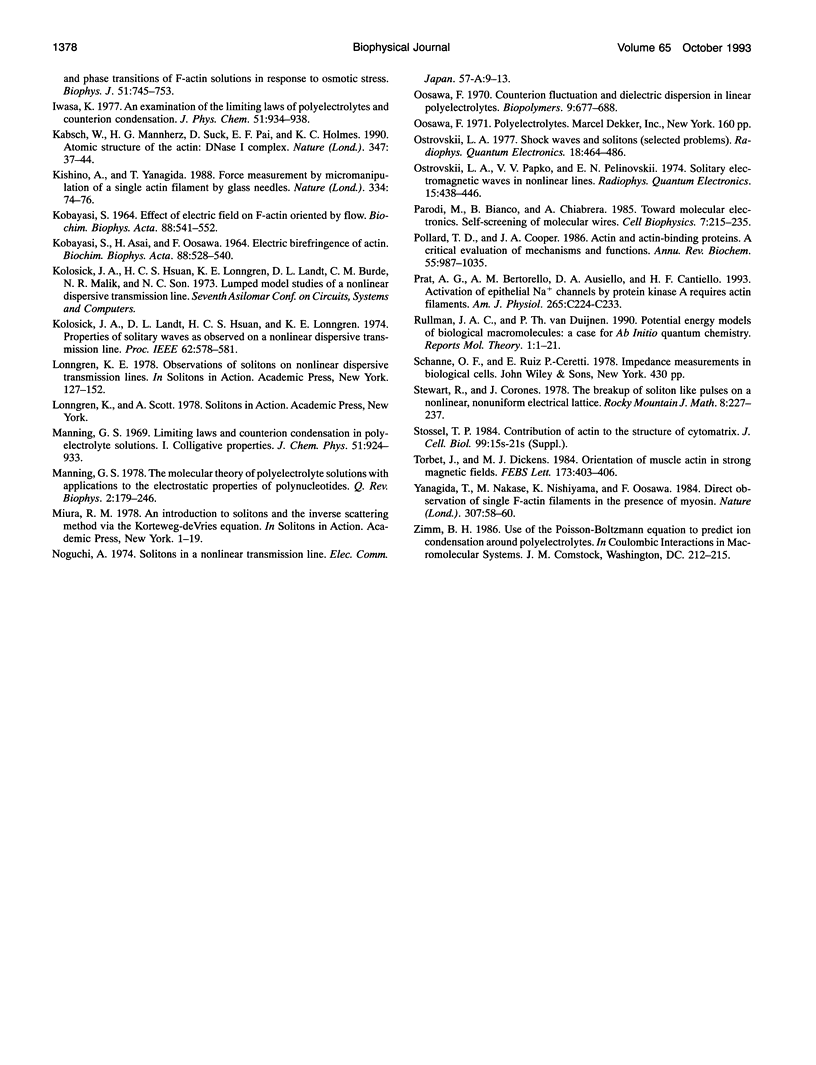
Images in this article
Selected References
These references are in PubMed. This may not be the complete list of references from this article.
- Anderson C. F., Record M. T., Jr Ion distributions around DNA and other cylindrical polyions: theoretical descriptions and physical implications. Annu Rev Biophys Biophys Chem. 1990;19:423–465. doi: 10.1146/annurev.bb.19.060190.002231. [DOI] [PubMed] [Google Scholar]
- Cantiello H. F., Patenaude C. R., Ausiello D. A. G protein subunit, alpha i-3, activates a pertussis toxin-sensitive Na+ channel from the epithelial cell line, A6. J Biol Chem. 1989 Dec 15;264(35):20867–20870. [PubMed] [Google Scholar]
- Cantiello H. F., Patenaude C., Zaner K. Osmotically induced electrical signals from actin filaments. Biophys J. 1991 Jun;59(6):1284–1289. doi: 10.1016/S0006-3495(91)82343-8. [DOI] [PMC free article] [PubMed] [Google Scholar]
- Cantiello H. F., Prat A. G., Bonventre J. V., Cunningham C. C., Hartwig J. H., Ausiello D. A. Actin-binding protein contributes to cell volume regulatory ion channel activation in melanoma cells. J Biol Chem. 1993 Mar 5;268(7):4596–4599. [PubMed] [Google Scholar]
- Cantiello H. F., Stow J. L., Prat A. G., Ausiello D. A. Actin filaments regulate epithelial Na+ channel activity. Am J Physiol. 1991 Nov;261(5 Pt 1):C882–C888. doi: 10.1152/ajpcell.1991.261.5.C882. [DOI] [PubMed] [Google Scholar]
- Hamill O. P., Marty A., Neher E., Sakmann B., Sigworth F. J. Improved patch-clamp techniques for high-resolution current recording from cells and cell-free membrane patches. Pflugers Arch. 1981 Aug;391(2):85–100. doi: 10.1007/BF00656997. [DOI] [PubMed] [Google Scholar]
- Holmes K. C., Popp D., Gebhard W., Kabsch W. Atomic model of the actin filament. Nature. 1990 Sep 6;347(6288):44–49. doi: 10.1038/347044a0. [DOI] [PubMed] [Google Scholar]
- Ito T., Zaner K. S., Stossel T. P. Nonideality of volume flows and phase transitions of F-actin solutions in response to osmotic stress. Biophys J. 1987 May;51(5):745–753. doi: 10.1016/S0006-3495(87)83401-X. [DOI] [PMC free article] [PubMed] [Google Scholar]
- KOBAYASI S., ASAI H., OOSAWA F. ELECTRIC BIREFRINGENCE OF ACTIN. Biochim Biophys Acta. 1964 Nov 29;88:528–540. doi: 10.1016/0926-6577(64)90096-8. [DOI] [PubMed] [Google Scholar]
- KOBAYASI S. EFFECT OF ELECTRIC FIELD ON F-ACTIN ORIENTED BY FLOW. Biochim Biophys Acta. 1964 Nov 29;88:541–552. [PubMed] [Google Scholar]
- Kabsch W., Mannherz H. G., Suck D., Pai E. F., Holmes K. C. Atomic structure of the actin:DNase I complex. Nature. 1990 Sep 6;347(6288):37–44. doi: 10.1038/347037a0. [DOI] [PubMed] [Google Scholar]
- Kishino A., Yanagida T. Force measurements by micromanipulation of a single actin filament by glass needles. Nature. 1988 Jul 7;334(6177):74–76. doi: 10.1038/334074a0. [DOI] [PubMed] [Google Scholar]
- Manning G. S. The molecular theory of polyelectrolyte solutions with applications to the electrostatic properties of polynucleotides. Q Rev Biophys. 1978 May;11(2):179–246. doi: 10.1017/s0033583500002031. [DOI] [PubMed] [Google Scholar]
- Parodi M., Bianco B., Chiabrera A. Toward molecular electronics. Self-screening of molecular wires. Cell Biophys. 1985 Sep;7(3):215–235. doi: 10.1007/BF02790467. [DOI] [PubMed] [Google Scholar]
- Pollard T. D., Cooper J. A. Actin and actin-binding proteins. A critical evaluation of mechanisms and functions. Annu Rev Biochem. 1986;55:987–1035. doi: 10.1146/annurev.bi.55.070186.005011. [DOI] [PubMed] [Google Scholar]
- Prat A. G., Bertorello A. M., Ausiello D. A., Cantiello H. F. Activation of epithelial Na+ channels by protein kinase A requires actin filaments. Am J Physiol. 1993 Jul;265(1 Pt 1):C224–C233. doi: 10.1152/ajpcell.1993.265.1.C224. [DOI] [PubMed] [Google Scholar]
- Stossel T. P. Contribution of actin to the structure of the cytoplasmic matrix. J Cell Biol. 1984 Jul;99(1 Pt 2):15s–21s. doi: 10.1083/jcb.99.1.15s. [DOI] [PMC free article] [PubMed] [Google Scholar]
- Torbet J., Dickens M. J. Orientation of skeletal muscle actin in strong magnetic fields. FEBS Lett. 1984 Aug 6;173(2):403–406. doi: 10.1016/0014-5793(84)80814-5. [DOI] [PubMed] [Google Scholar]
- Yanagida T., Nakase M., Nishiyama K., Oosawa F. Direct observation of motion of single F-actin filaments in the presence of myosin. Nature. 1984 Jan 5;307(5946):58–60. doi: 10.1038/307058a0. [DOI] [PubMed] [Google Scholar]