Abstract
Using experimentally observed processes of linear growth, heterogeneous nucleation, and polymer bending, with no additional assumptions, we have been able to model the two-dimensional formation of polymer domains by sickle hemoglobin. The domains begin with twofold symmetry and proceed toward closure into spherulites at a constant rate. Relationships derived from the simulations presented and the requirements of scaling result in simple expressions for the sensitivity of the closure times to the model input parameters and allow the results to be extended to regions not actually simulated. For concentrations above approximately 25 g/dl, closure times are longer than the time required for the conclusion of the polymerization reaction, and thus incomplete spherulites will be the dominant geometry at high concentrations. Moreover, spherulites are not predicted to form in times less than a few seconds, implying that spherulites will not form during the transit of erythrocytes through the capillaries. Polymer-polymer exclusion, surface nucleation, and monomer exhaustion were also explored and found to have only weak effects on the results.
Full text
PDF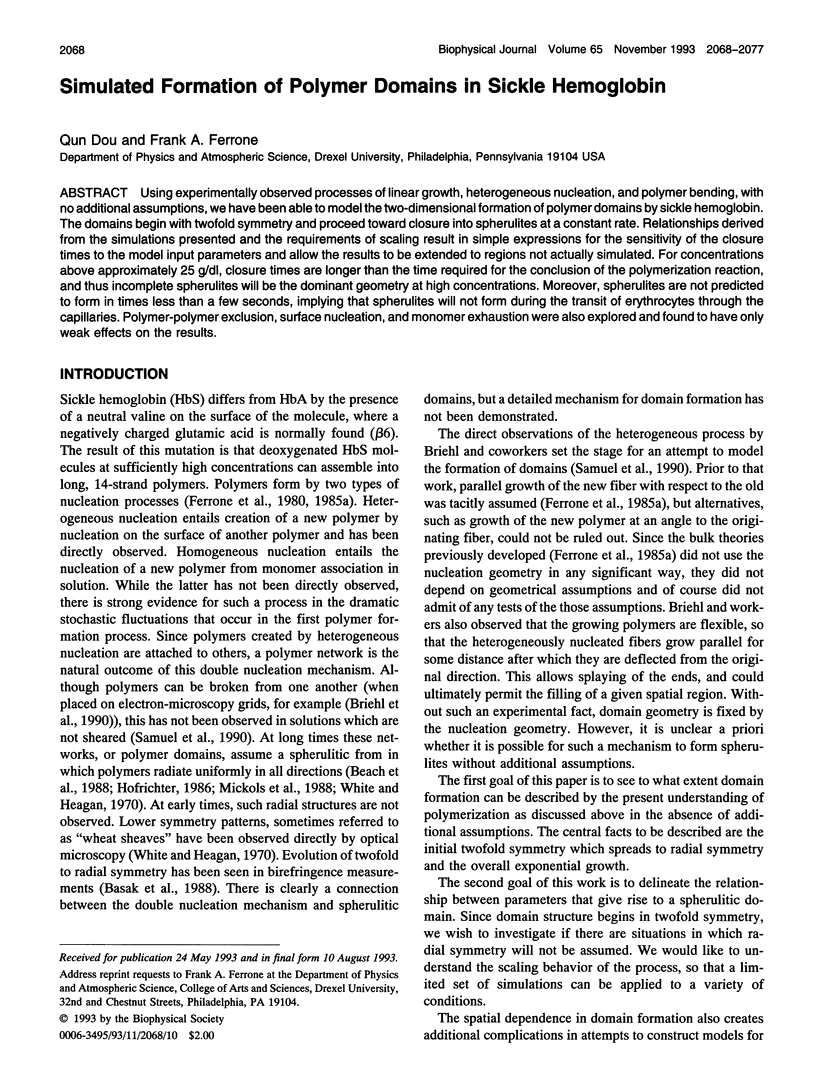
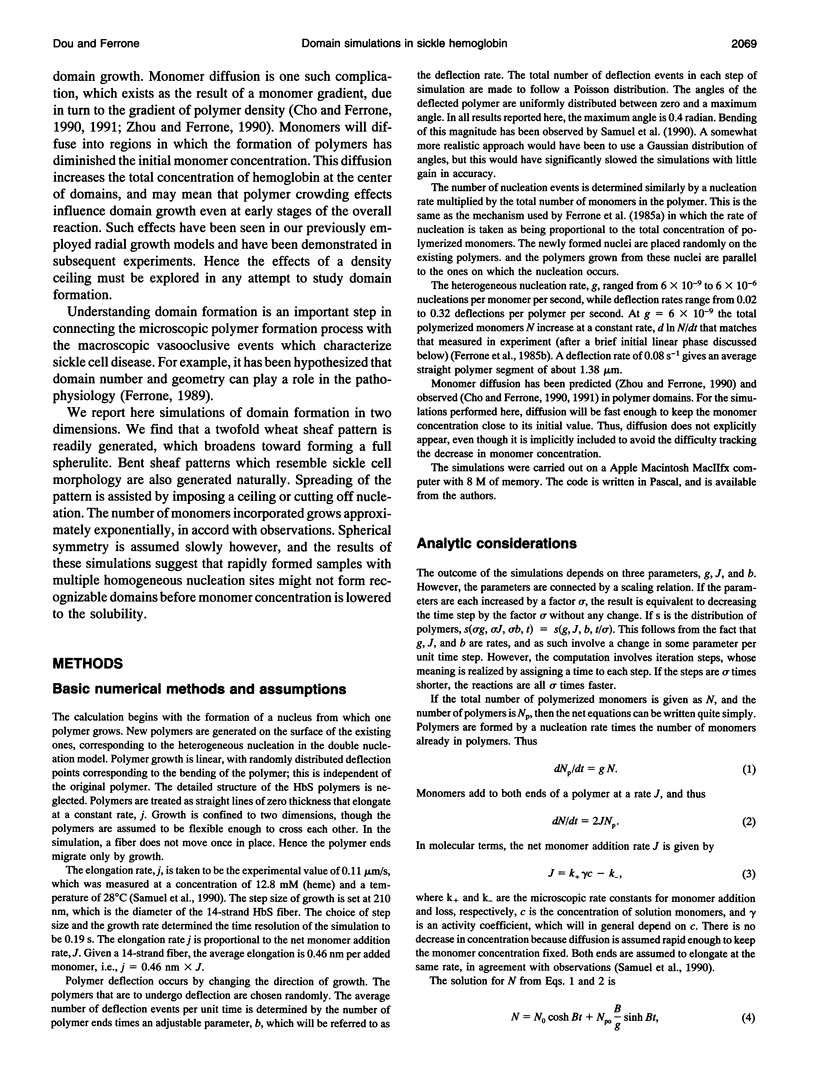
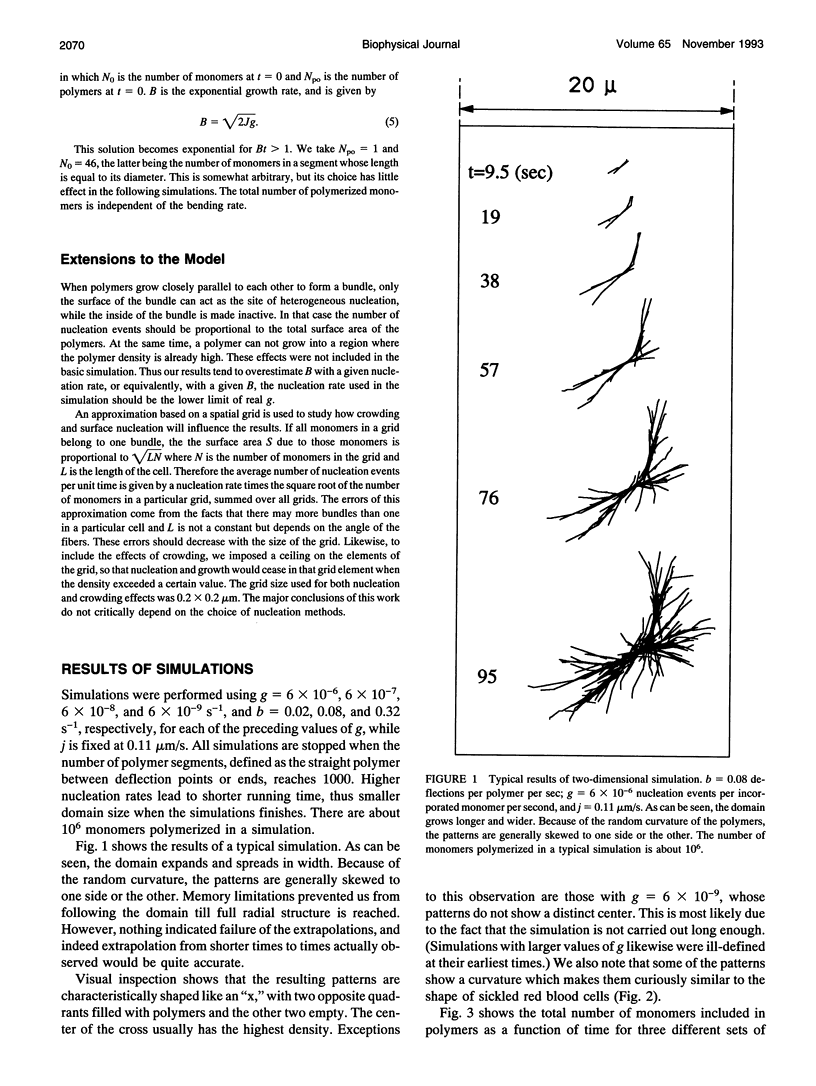
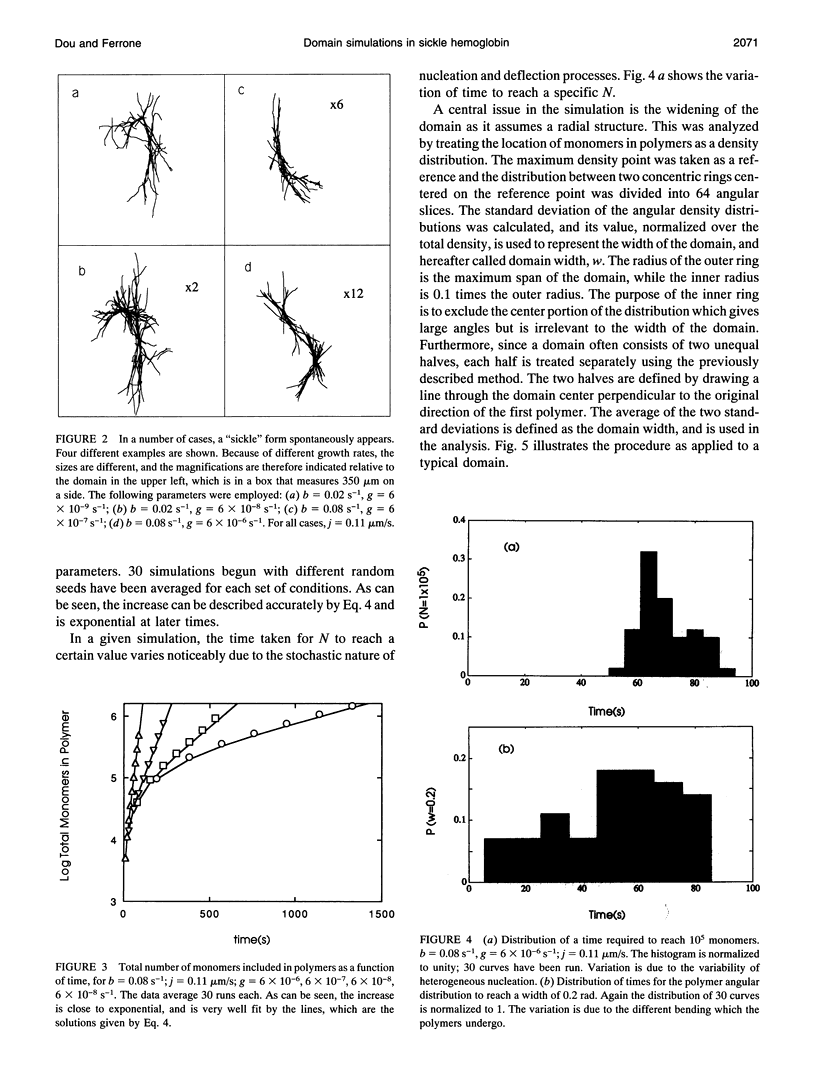
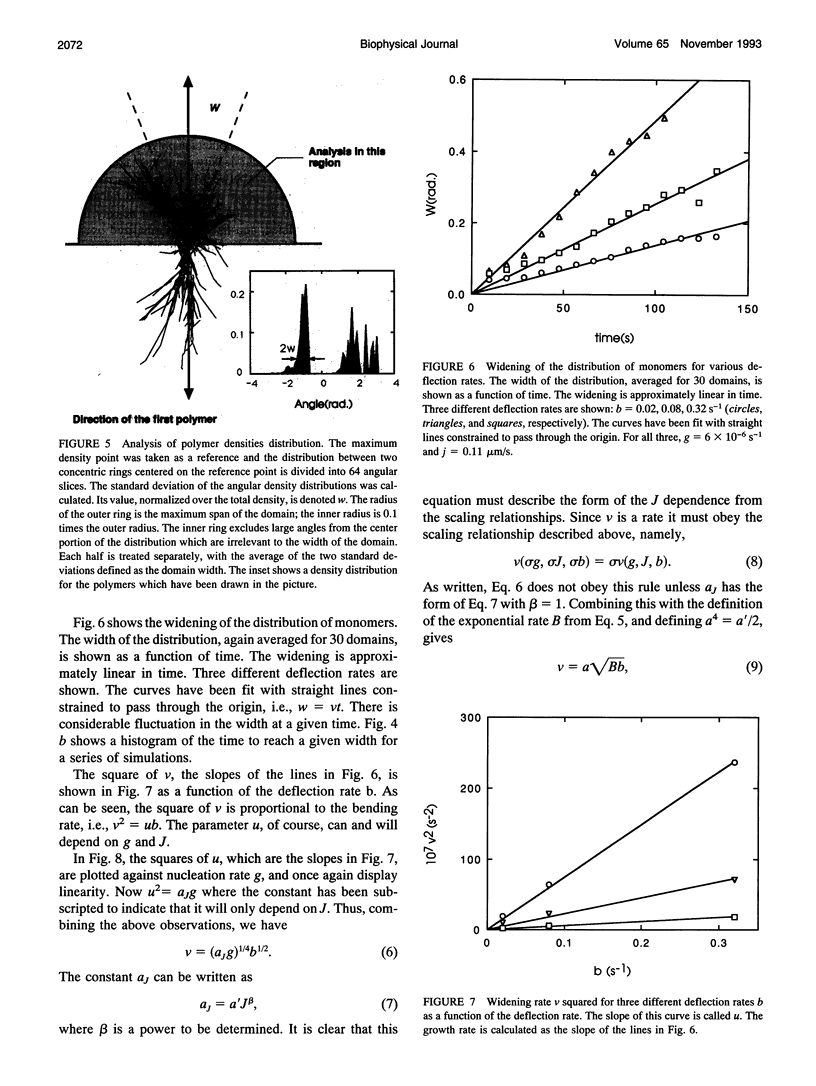
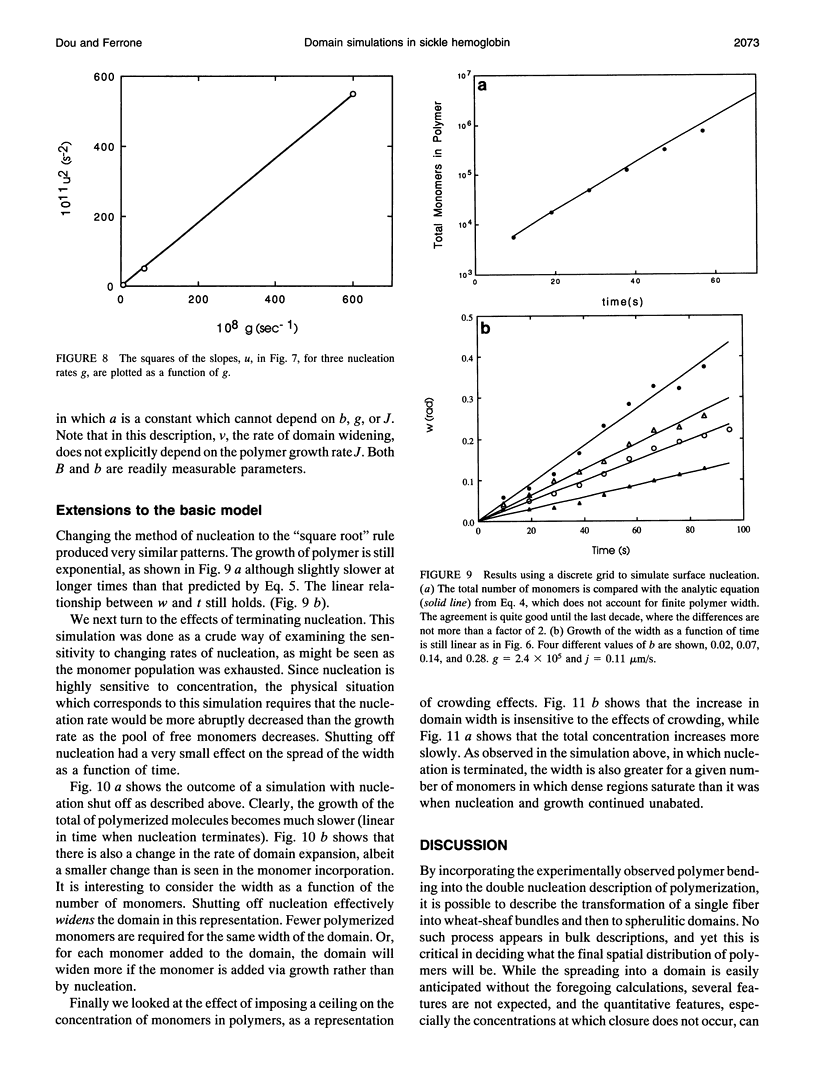
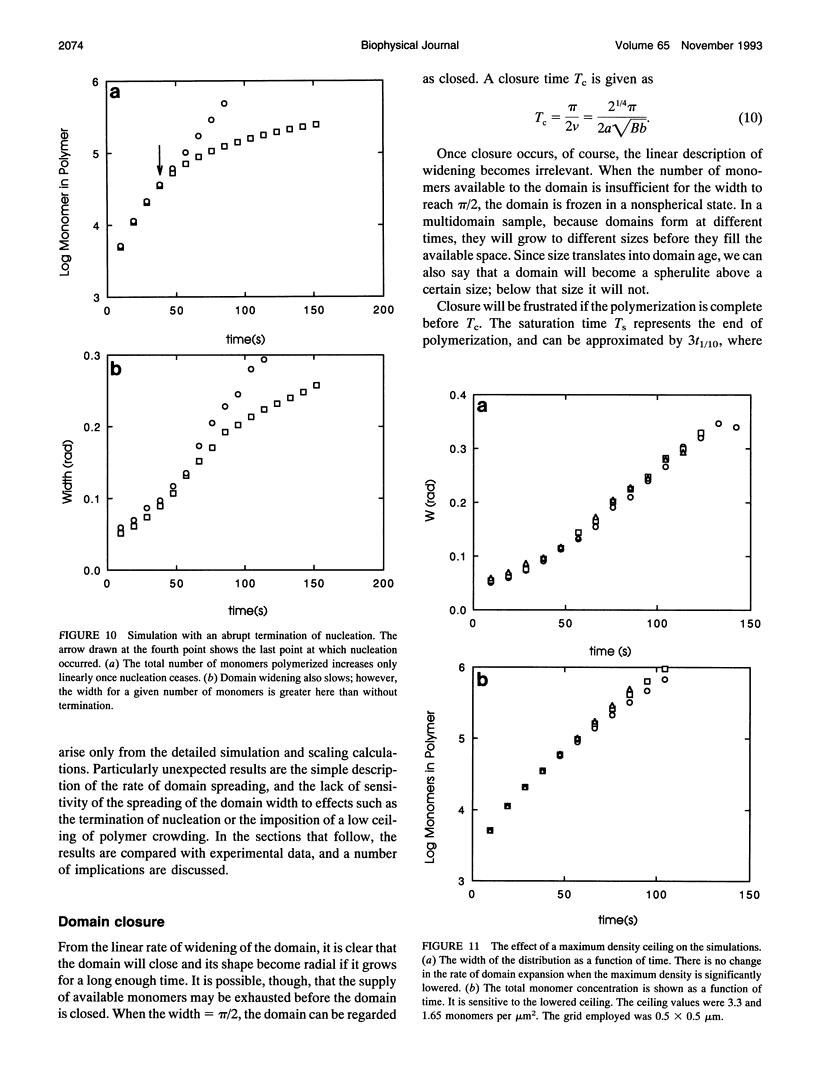
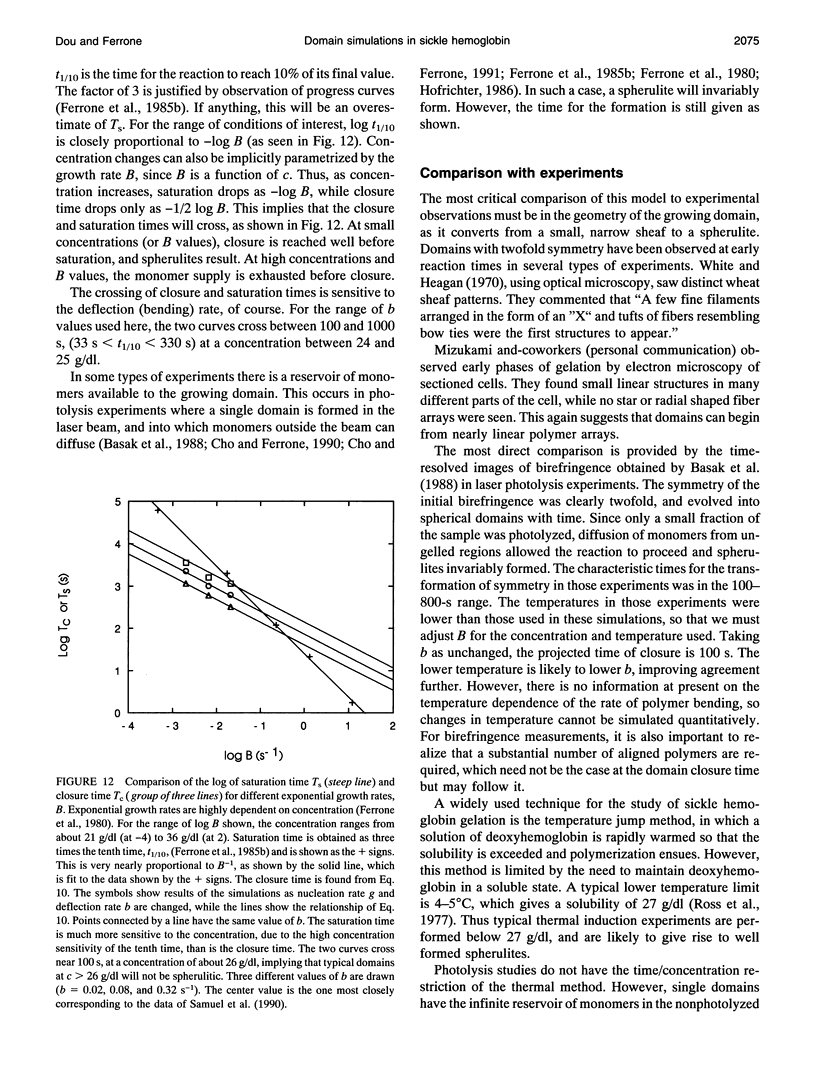
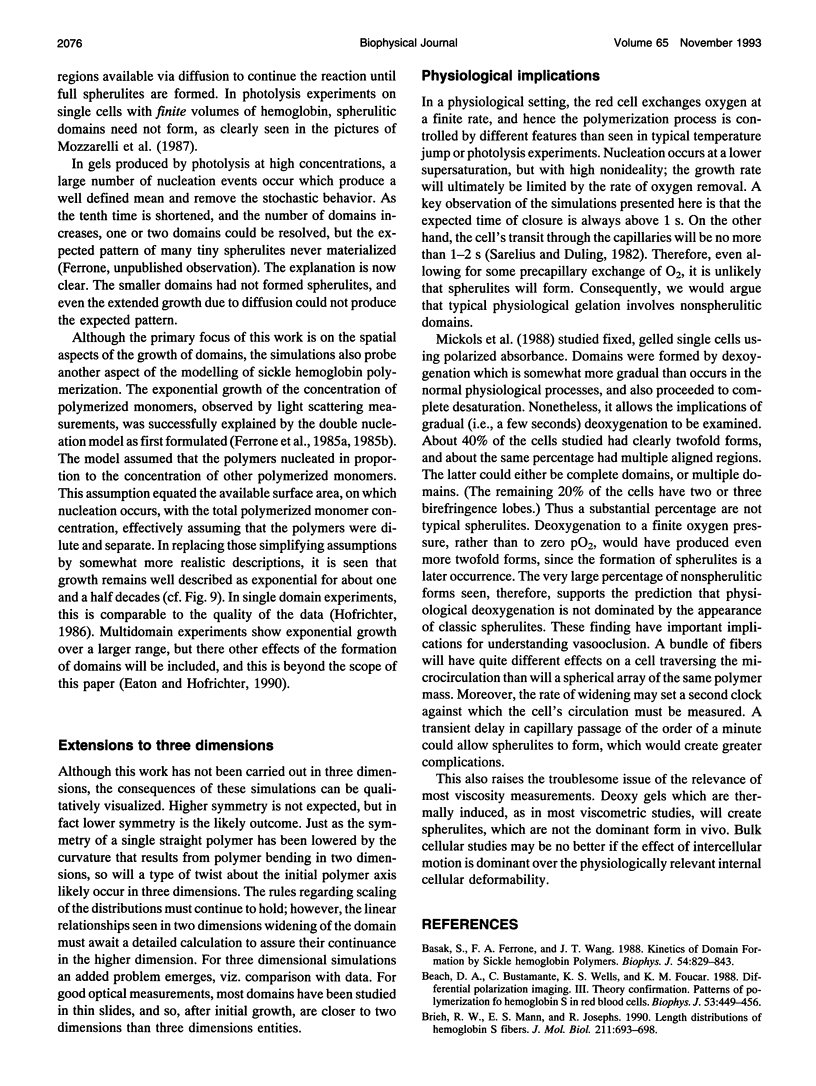
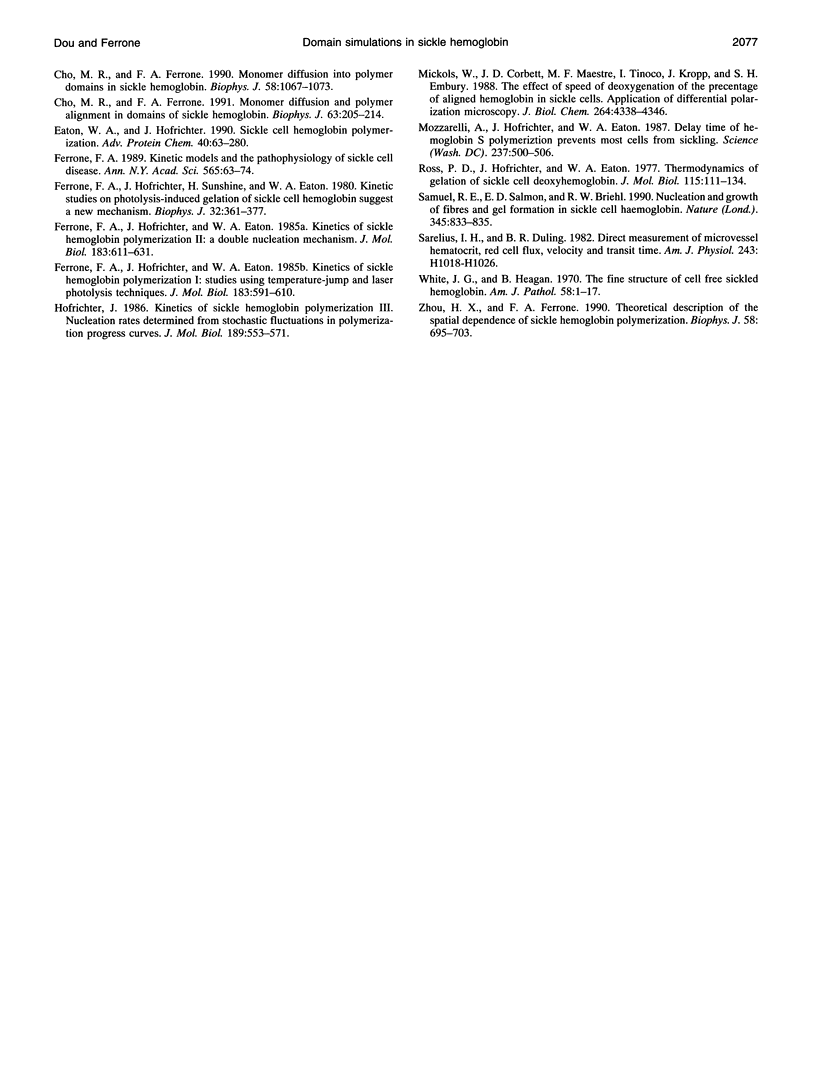
Images in this article
Selected References
These references are in PubMed. This may not be the complete list of references from this article.
- Basak S., Ferrone F. A., Wang J. T. Kinetics of domain formation by sickle hemoglobin polymers. Biophys J. 1988 Nov;54(5):829–843. doi: 10.1016/S0006-3495(88)83020-0. [DOI] [PMC free article] [PubMed] [Google Scholar]
- Beach D. A., Bustamante C., Wells K. S., Foucar K. M. Differential polarization imaging. III. Theory confirmation. Patterns of polymerization of hemoglobin S in red blood sickle cells. Biophys J. 1988 Mar;53(3):449–456. doi: 10.1016/S0006-3495(88)83121-7. [DOI] [PMC free article] [PubMed] [Google Scholar]
- Briehl R. W., Mann E. S., Josephs R. Length distributions of hemoglobin S fibers. J Mol Biol. 1990 Feb 20;211(4):693–698. doi: 10.1016/0022-2836(90)90070-3. [DOI] [PubMed] [Google Scholar]
- Cho M. R., Ferrone F. A. Monomer diffusion and polymer alignment in domains of sickle hemoglobin. Biophys J. 1992 Jul;63(1):205–214. doi: 10.1016/S0006-3495(92)81595-3. [DOI] [PMC free article] [PubMed] [Google Scholar]
- Cho M. R., Ferrone F. A. Monomer diffusion into polymer domains in sickle hemoglobin. Biophys J. 1990 Oct;58(4):1067–1073. doi: 10.1016/S0006-3495(90)82449-8. [DOI] [PMC free article] [PubMed] [Google Scholar]
- Eaton W. A., Hofrichter J. Sickle cell hemoglobin polymerization. Adv Protein Chem. 1990;40:63–279. doi: 10.1016/s0065-3233(08)60287-9. [DOI] [PubMed] [Google Scholar]
- Ferrone F. A., Hofrichter J., Eaton W. A. Kinetics of sickle hemoglobin polymerization. I. Studies using temperature-jump and laser photolysis techniques. J Mol Biol. 1985 Jun 25;183(4):591–610. doi: 10.1016/0022-2836(85)90174-3. [DOI] [PubMed] [Google Scholar]
- Ferrone F. A., Hofrichter J., Eaton W. A. Kinetics of sickle hemoglobin polymerization. II. A double nucleation mechanism. J Mol Biol. 1985 Jun 25;183(4):611–631. doi: 10.1016/0022-2836(85)90175-5. [DOI] [PubMed] [Google Scholar]
- Ferrone F. A., Hofrichter J., Sunshine H. R., Eaton W. A. Kinetic studies on photolysis-induced gelation of sickle cell hemoglobin suggest a new mechanism. Biophys J. 1980 Oct;32(1):361–380. doi: 10.1016/S0006-3495(80)84962-9. [DOI] [PMC free article] [PubMed] [Google Scholar]
- Ferrone F. A. Kinetic models and the pathophysiology of sickle cell disease. Ann N Y Acad Sci. 1989;565:63–74. doi: 10.1111/j.1749-6632.1989.tb24151.x. [DOI] [PubMed] [Google Scholar]
- Hofrichter J. Kinetics of sickle hemoglobin polymerization. III. Nucleation rates determined from stochastic fluctuations in polymerization progress curves. J Mol Biol. 1986 Jun 5;189(3):553–571. doi: 10.1016/0022-2836(86)90324-4. [DOI] [PubMed] [Google Scholar]
- Mickols W. E., Corbett J. D., Maestre M. F., Tinoco I., Jr, Kropp J., Embury S. H. The effect of speed of deoxygenation on the percentage of aligned hemoglobin in sickle cells. Application of differential polarization microscopy. J Biol Chem. 1988 Mar 25;263(9):4338–4346. [PubMed] [Google Scholar]
- Mozzarelli A., Hofrichter J., Eaton W. A. Delay time of hemoglobin S polymerization prevents most cells from sickling in vivo. Science. 1987 Jul 31;237(4814):500–506. doi: 10.1126/science.3603036. [DOI] [PubMed] [Google Scholar]
- Ross P. D., Hofrichter J., Eaton W. A. Thermodynamics of gelation of sickle cell deoxyhemoglobin. J Mol Biol. 1977 Sep 15;115(2):111–134. doi: 10.1016/0022-2836(77)90093-6. [DOI] [PubMed] [Google Scholar]
- Samuel R. E., Salmon E. D., Briehl R. W. Nucleation and growth of fibres and gel formation in sickle cell haemoglobin. Nature. 1990 Jun 28;345(6278):833–835. doi: 10.1038/345833a0. [DOI] [PubMed] [Google Scholar]
- Sarelius I. H., Duling B. R. Direct measurement of microvessel hematocrit, red cell flux, velocity, and transit time. Am J Physiol. 1982 Dec;243(6):H1018–H1026. doi: 10.1152/ajpheart.1982.243.6.H1018. [DOI] [PubMed] [Google Scholar]
- White J. G., Heagan B. The fine structure of cell-free sickled hemoglobin. Am J Pathol. 1970 Jan;58(1):1–17. [PMC free article] [PubMed] [Google Scholar]
- Zhou H. X., Ferrone F. A. Theoretical description of the spatial dependence of sickle hemoglobin polymerization. Biophys J. 1990 Sep;58(3):695–703. doi: 10.1016/S0006-3495(90)82412-7. [DOI] [PMC free article] [PubMed] [Google Scholar]