Abstract
To understand the mechanism of membrane fusion, we have to infer the sequence of structural transformations that occurs during the process. Here, it is shown how one can estimate the lipid composition-dependent free energies of intermediate structures of different geometries. One can then infer which fusion mechanism is the best explanation of observed behavior in different systems by selecting the mechanism that requires the least energy. The treatment involves no adjustable parameters. It includes contributions to the intermediate energy resulting from the presence of hydrophobic interstices within structures formed between apposed bilayers. Results of these calculations show that a modified form of the stalk mechanism proposed by others is a likely fusion mechanism in a wide range of lipid compositions, but a mechanism based on inverted micellar intermediates (IMIs) is not. This should be true even in the vicinity of the lamellar/inverted hexagonal phase transition, where IMI formation would be most facile. Another prediction of the calculations is that traces of apolar lipids (e.g., long-chain alkanes) in membranes should have a substantial influence on fusion rates in general. The same theoretical methods can be used to generate and refine mechanisms for protein-mediated fusion.
Full text
PDF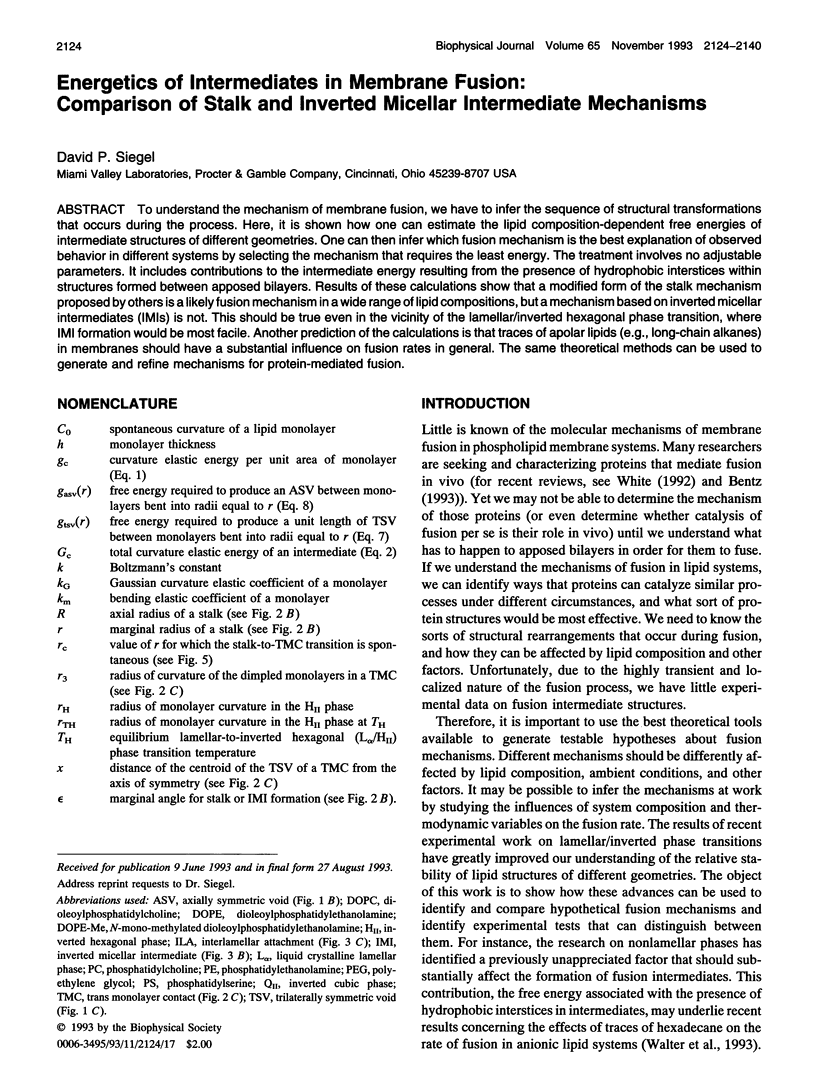
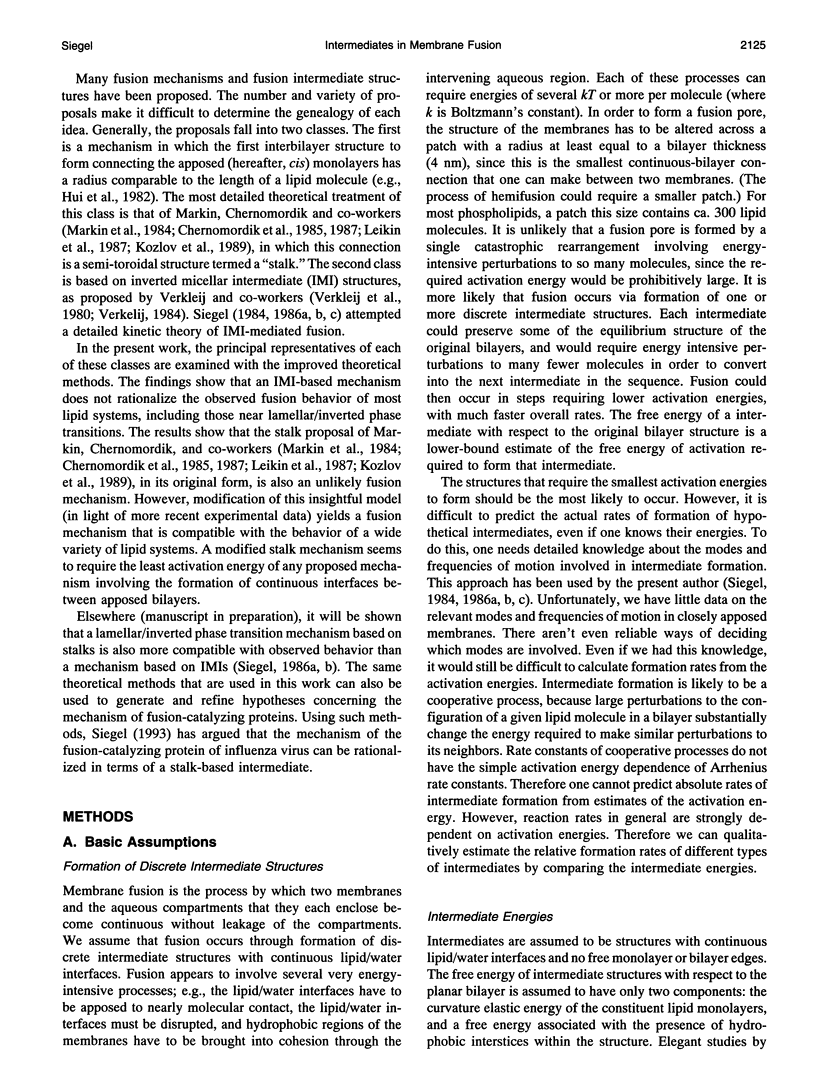
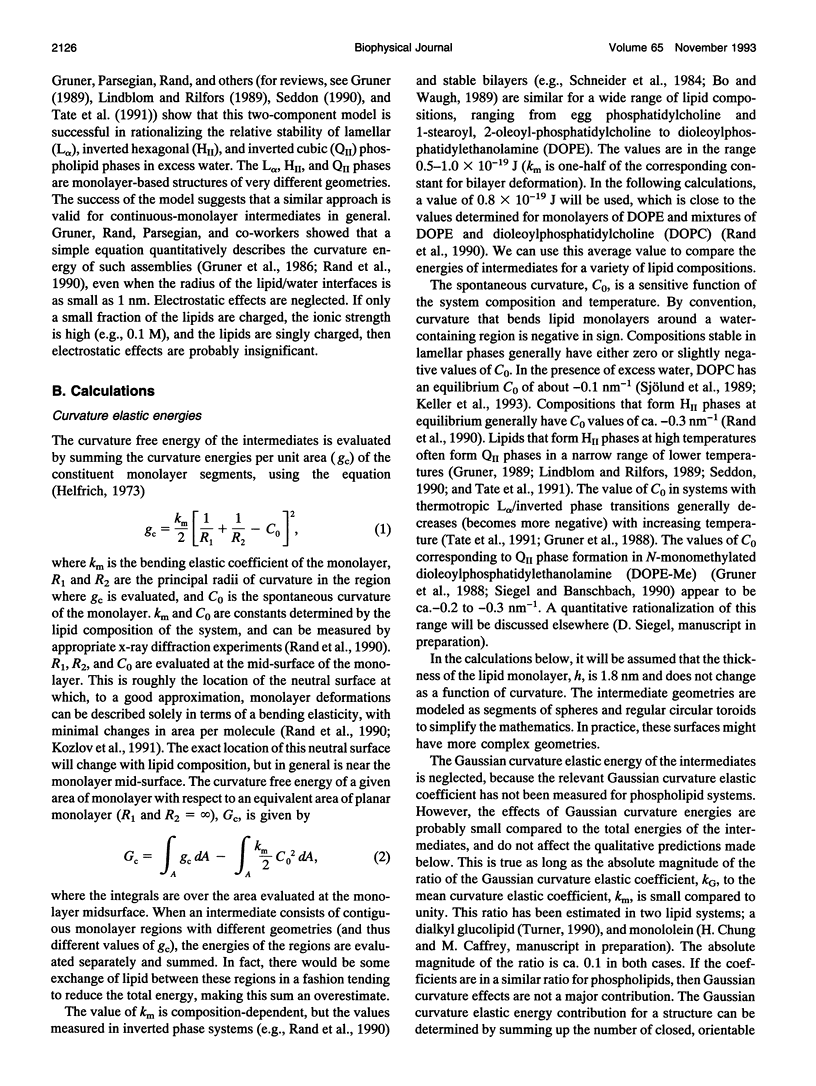
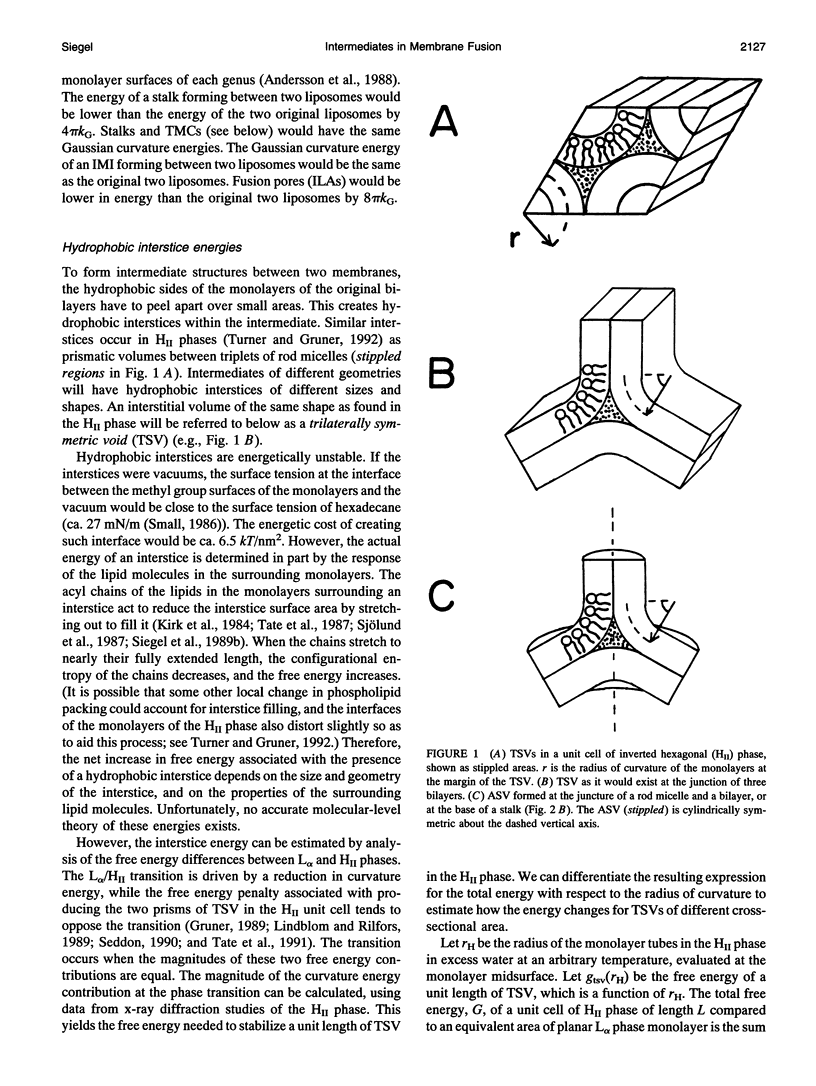

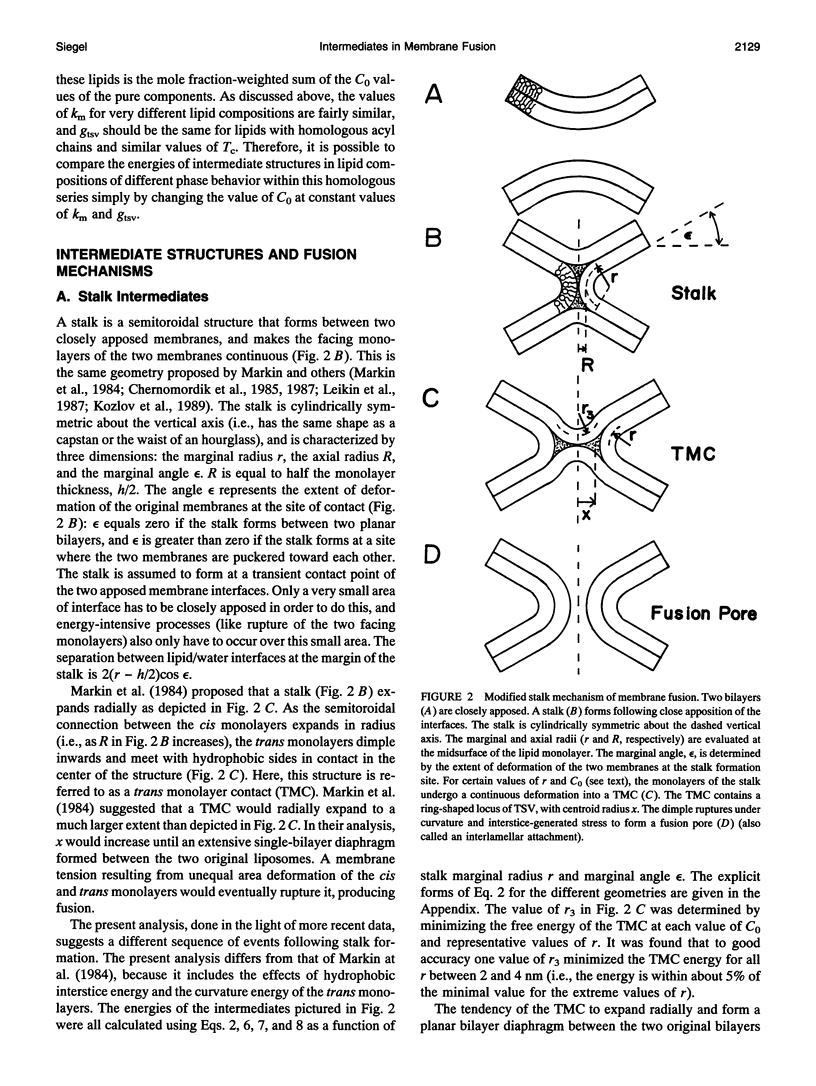
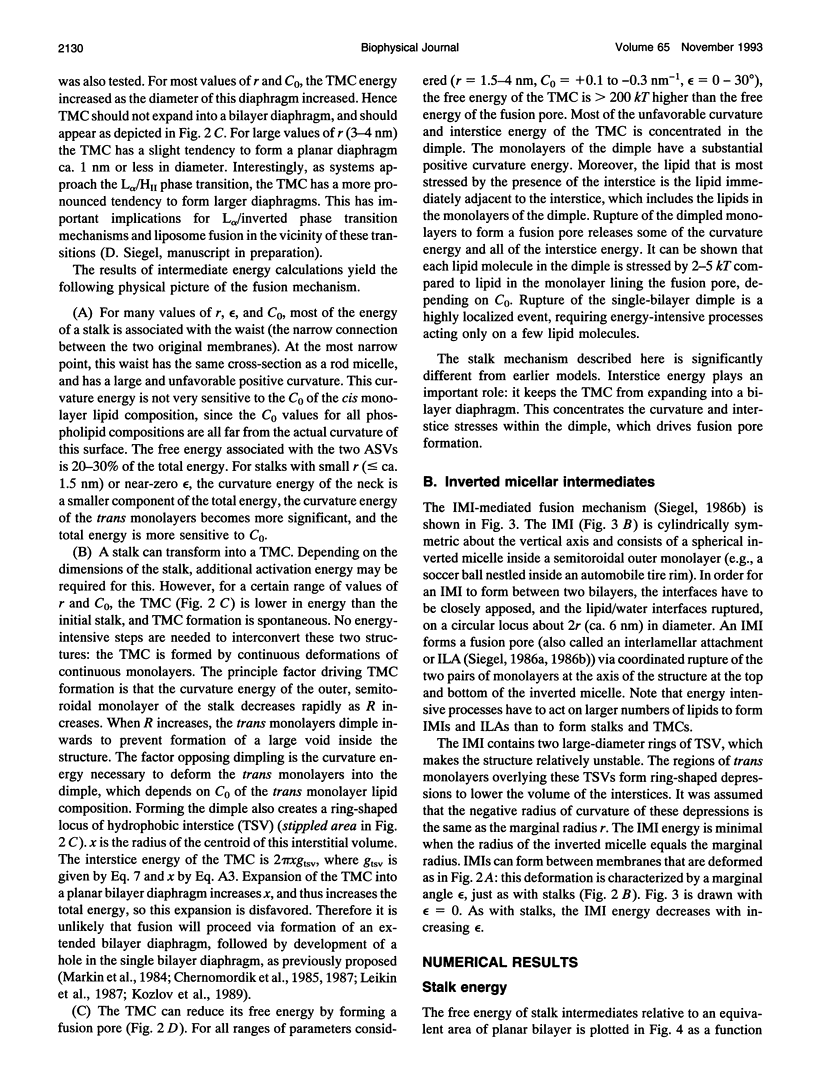
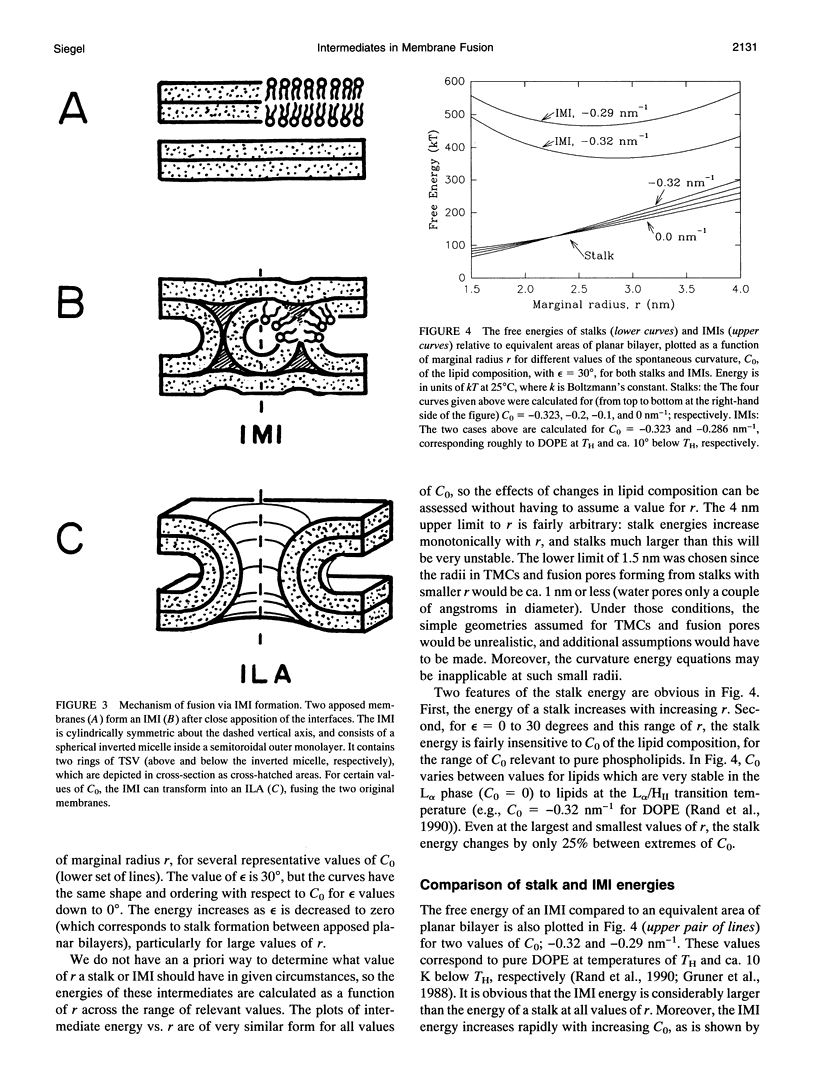
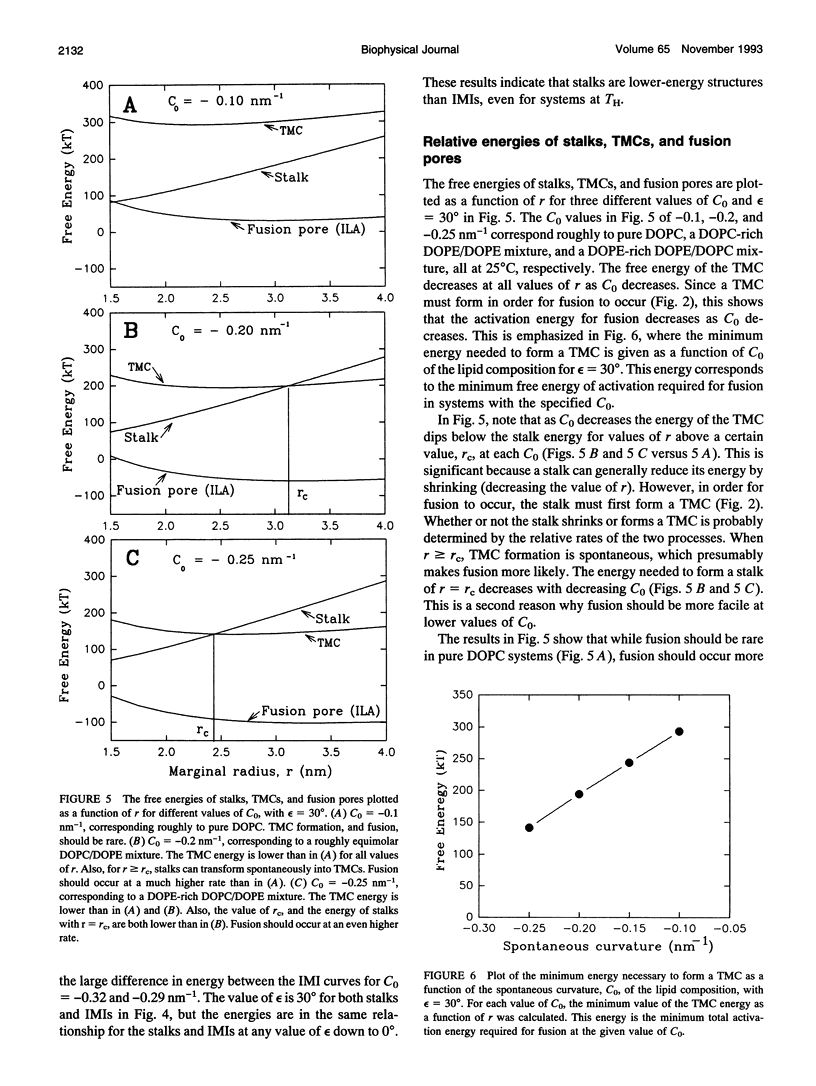
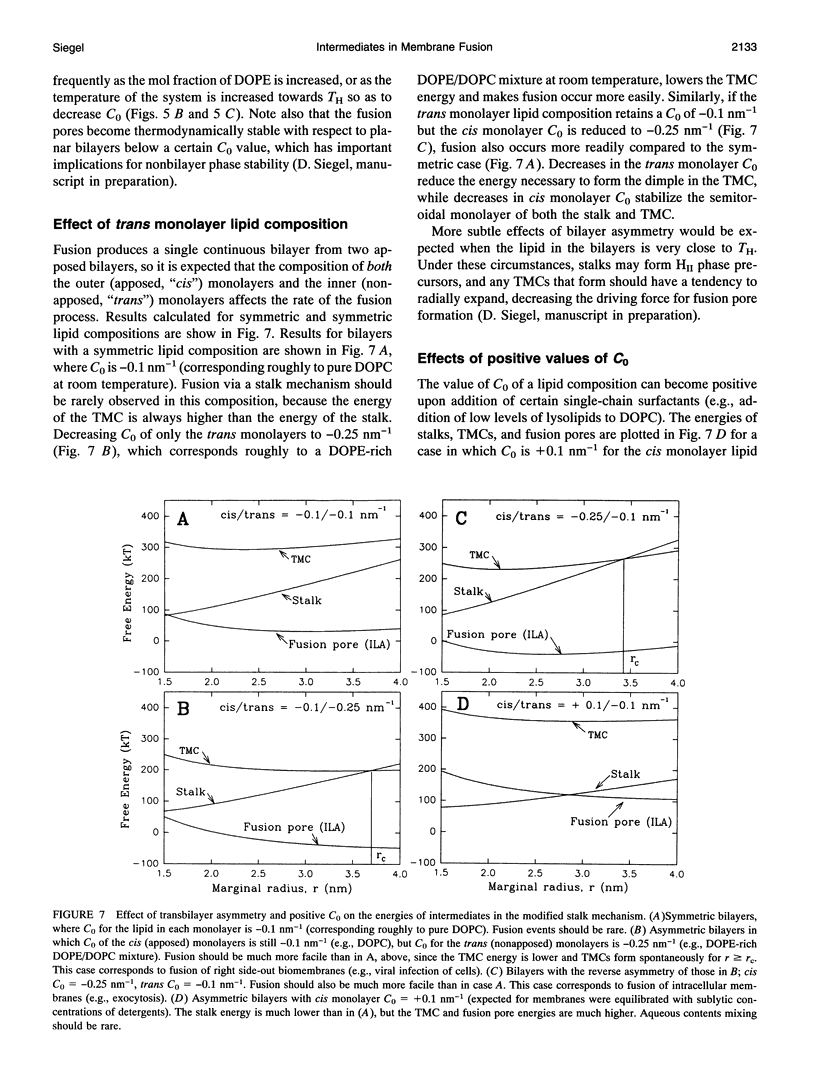
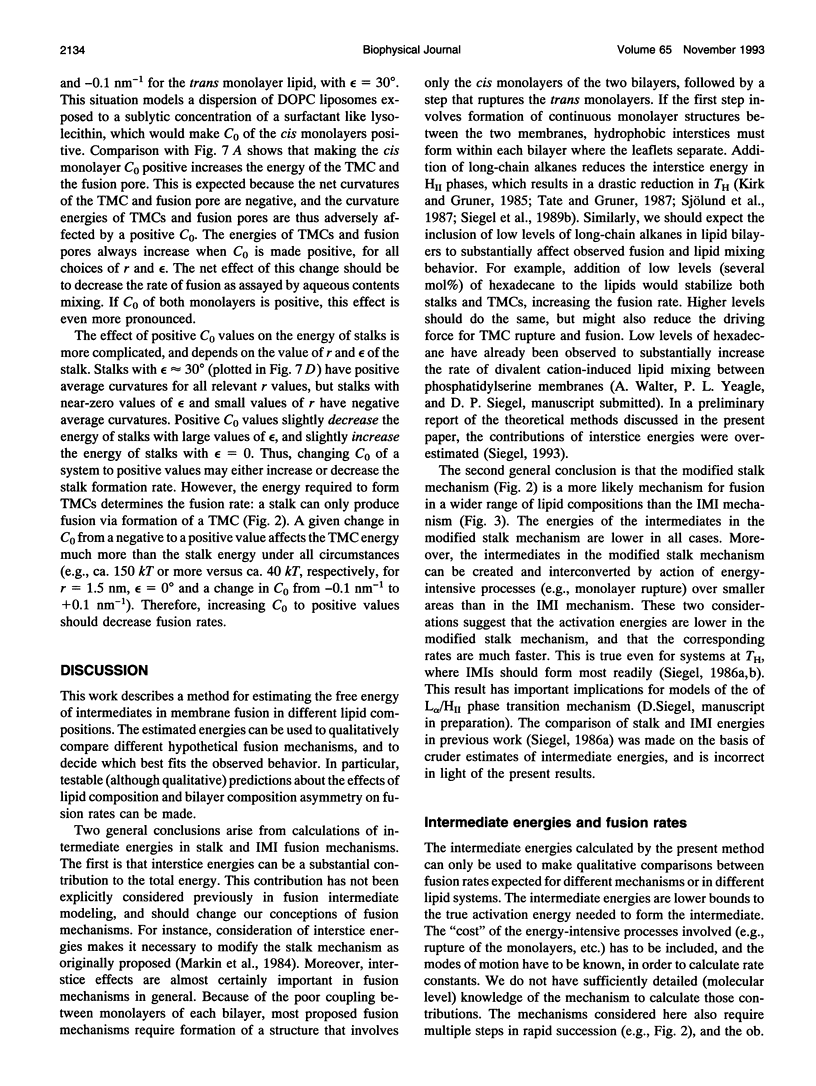
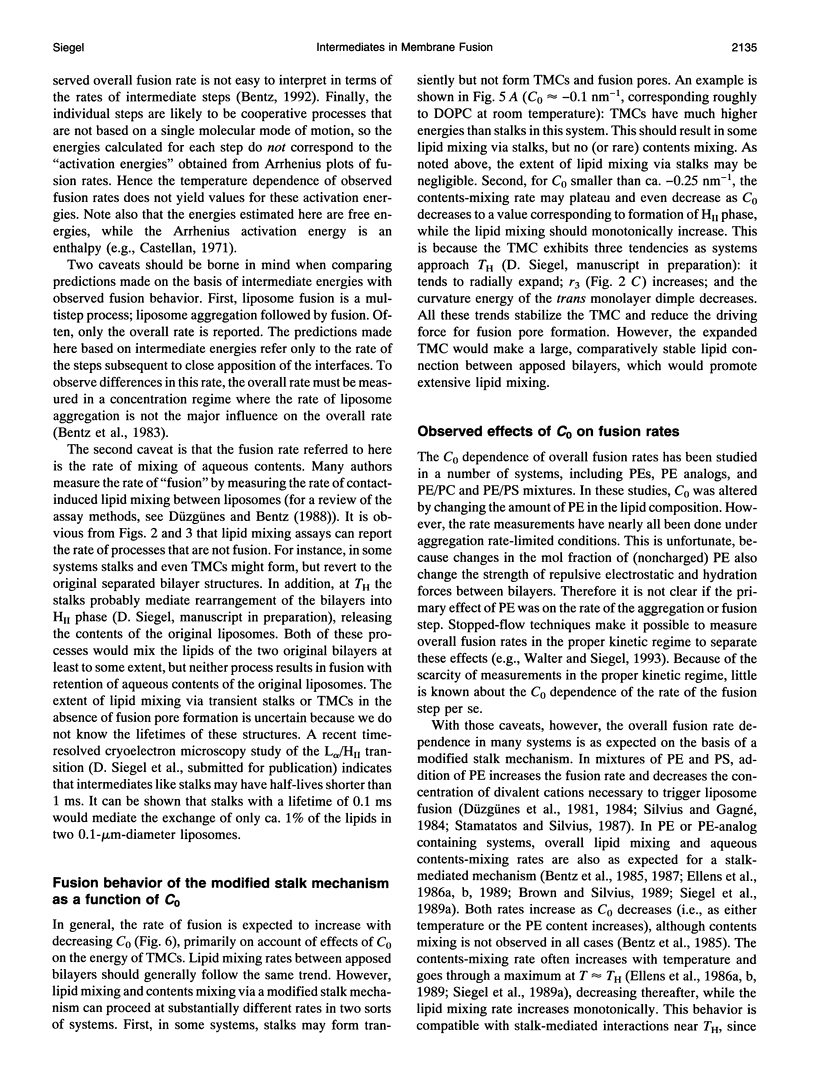
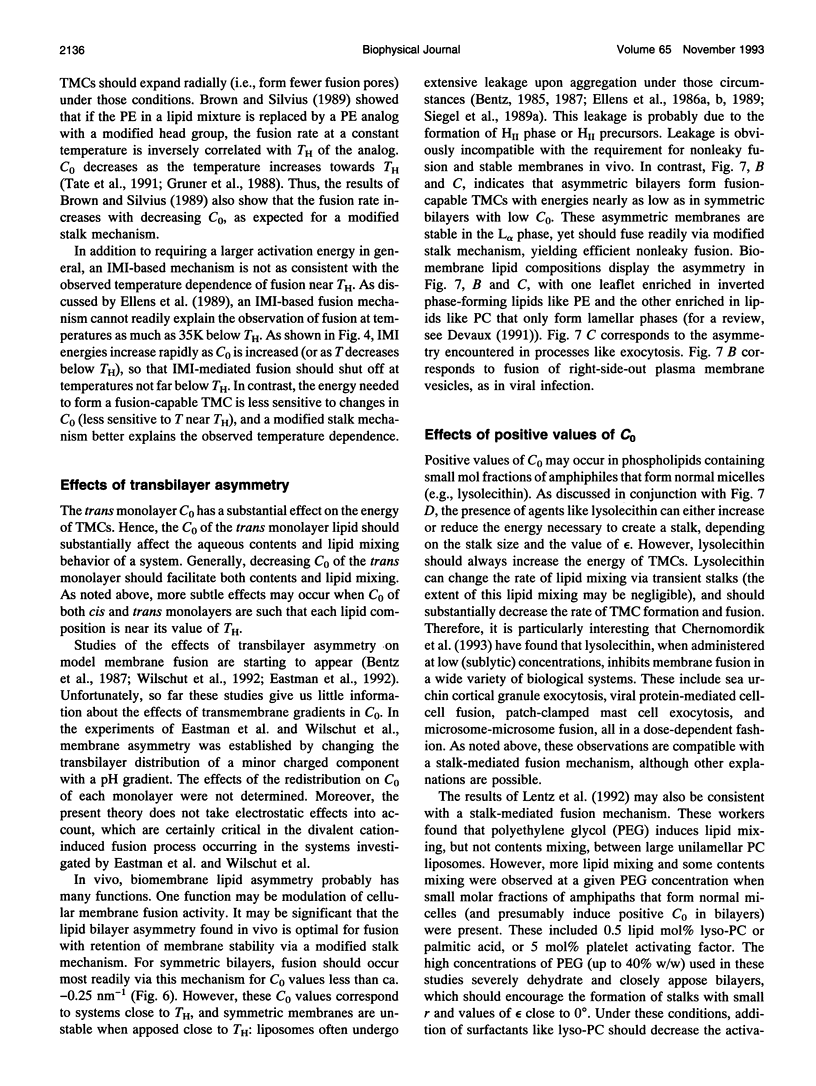
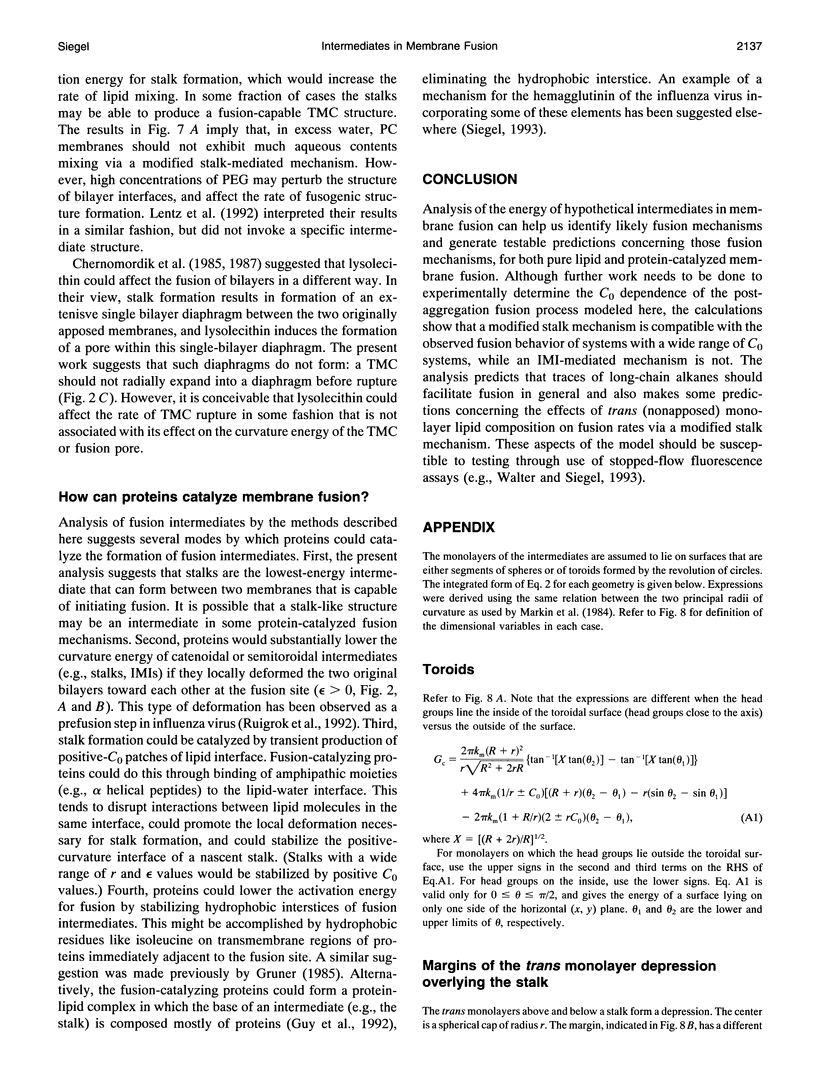
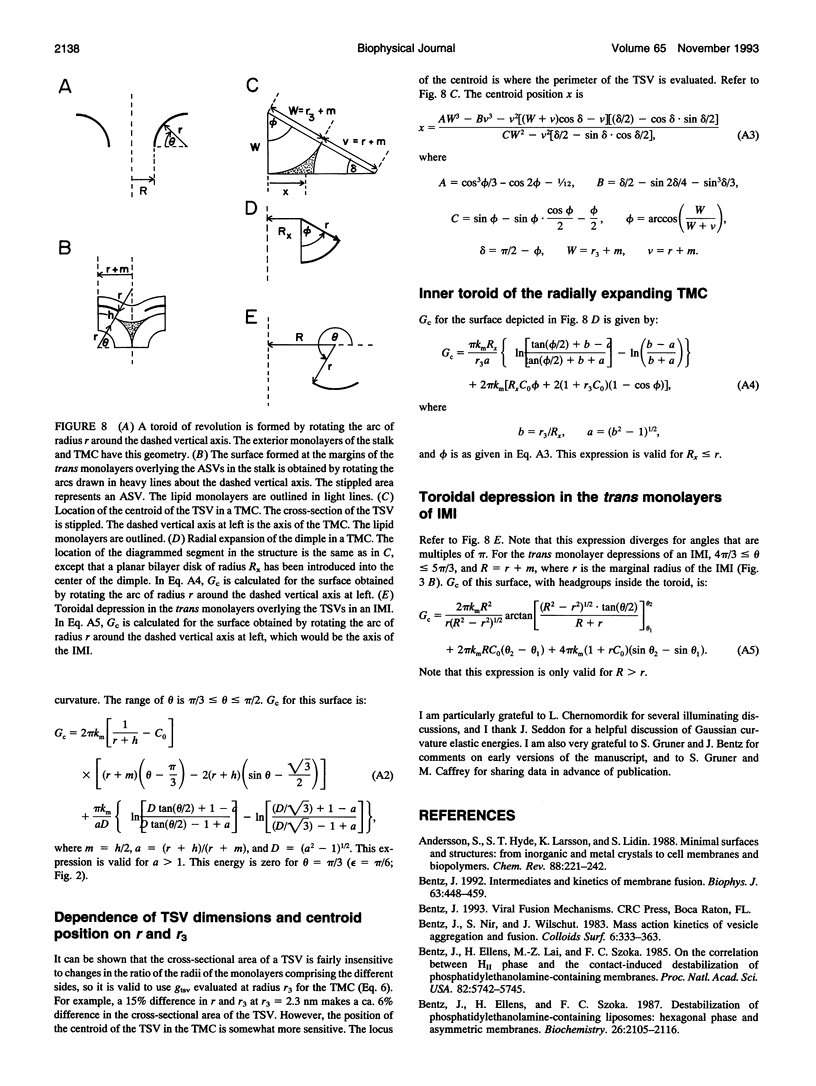
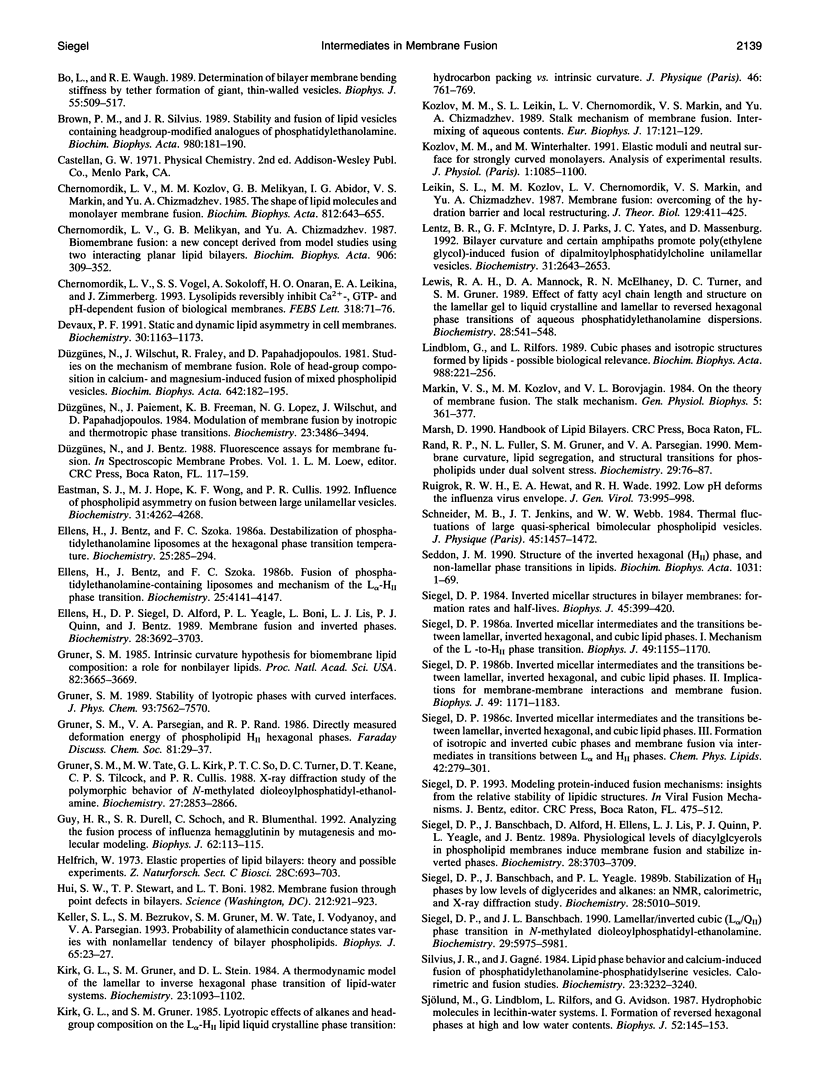
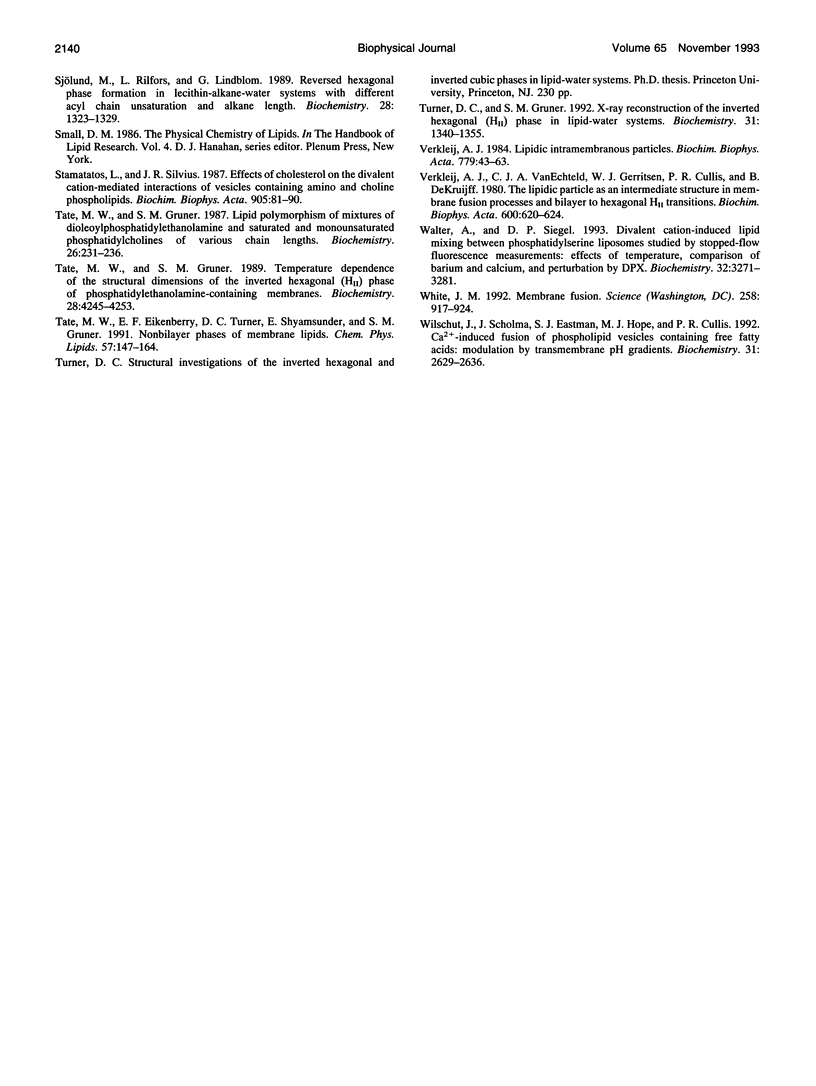
Selected References
These references are in PubMed. This may not be the complete list of references from this article.
- Bentz J., Ellens H., Lai M. Z., Szoka F. C., Jr On the correlation between HII phase and the contact-induced destabilization of phosphatidylethanolamine-containing membranes. Proc Natl Acad Sci U S A. 1985 Sep;82(17):5742–5745. doi: 10.1073/pnas.82.17.5742. [DOI] [PMC free article] [PubMed] [Google Scholar]
- Bentz J., Ellens H., Szoka F. C. Destabilization of phosphatidylethanolamine-containing liposomes: hexagonal phase and asymmetric membranes. Biochemistry. 1987 Apr 21;26(8):2105–2116. doi: 10.1021/bi00382a008. [DOI] [PubMed] [Google Scholar]
- Bentz J. Intermediates and kinetics of membrane fusion. Biophys J. 1992 Aug;63(2):448–459. doi: 10.1016/S0006-3495(92)81622-3. [DOI] [PMC free article] [PubMed] [Google Scholar]
- Bo L., Waugh R. E. Determination of bilayer membrane bending stiffness by tether formation from giant, thin-walled vesicles. Biophys J. 1989 Mar;55(3):509–517. doi: 10.1016/S0006-3495(89)82844-9. [DOI] [PMC free article] [PubMed] [Google Scholar]
- Brown P. M., Silvius J. R. Stability and fusion of lipid vesicles containing headgroup-modified analogues of phosphatidylethanolamine. Biochim Biophys Acta. 1989 Apr 14;980(2):181–190. doi: 10.1016/0005-2736(89)90398-2. [DOI] [PubMed] [Google Scholar]
- Chernomordik L. V., Melikyan G. B., Chizmadzhev Y. A. Biomembrane fusion: a new concept derived from model studies using two interacting planar lipid bilayers. Biochim Biophys Acta. 1987 Oct 5;906(3):309–352. doi: 10.1016/0304-4157(87)90016-5. [DOI] [PubMed] [Google Scholar]
- Chernomordik L. V., Vogel S. S., Sokoloff A., Onaran H. O., Leikina E. A., Zimmerberg J. Lysolipids reversibly inhibit Ca(2+)-, GTP- and pH-dependent fusion of biological membranes. FEBS Lett. 1993 Feb 22;318(1):71–76. doi: 10.1016/0014-5793(93)81330-3. [DOI] [PubMed] [Google Scholar]
- Devaux P. F. Static and dynamic lipid asymmetry in cell membranes. Biochemistry. 1991 Feb 5;30(5):1163–1173. doi: 10.1021/bi00219a001. [DOI] [PubMed] [Google Scholar]
- Düzgünes N., Paiement J., Freeman K. B., Lopez N. G., Wilschut J., Papahadjopoulos D. Modulation of membrane fusion by ionotropic and thermotropic phase transitions. Biochemistry. 1984 Jul 17;23(15):3486–3494. doi: 10.1021/bi00310a016. [DOI] [PubMed] [Google Scholar]
- Düzgüneş N., Wilschut J., Fraley R., Papahadjopoulos D. Studies on the mechanism of membrane fusion. Role of head-group composition in calcium- and magnesium-induced fusion of mixed phospholipid vesicles. Biochim Biophys Acta. 1981 Mar 20;642(1):182–195. doi: 10.1016/0005-2736(81)90148-6. [DOI] [PubMed] [Google Scholar]
- Eastman S. J., Hope M. J., Wong K. F., Cullis P. R. Influence of phospholipid asymmetry on fusion between large unilamellar vesicles. Biochemistry. 1992 May 5;31(17):4262–4268. doi: 10.1021/bi00132a016. [DOI] [PubMed] [Google Scholar]
- Ellens H., Bentz J., Szoka F. C. Destabilization of phosphatidylethanolamine liposomes at the hexagonal phase transition temperature. Biochemistry. 1986 Jan 28;25(2):285–294. doi: 10.1021/bi00350a001. [DOI] [PubMed] [Google Scholar]
- Ellens H., Bentz J., Szoka F. C. Fusion of phosphatidylethanolamine-containing liposomes and mechanism of the L alpha-HII phase transition. Biochemistry. 1986 Jul 15;25(14):4141–4147. doi: 10.1021/bi00362a023. [DOI] [PubMed] [Google Scholar]
- Ellens H., Siegel D. P., Alford D., Yeagle P. L., Boni L., Lis L. J., Quinn P. J., Bentz J. Membrane fusion and inverted phases. Biochemistry. 1989 May 2;28(9):3692–3703. doi: 10.1021/bi00435a011. [DOI] [PubMed] [Google Scholar]
- Gruner S. M. Intrinsic curvature hypothesis for biomembrane lipid composition: a role for nonbilayer lipids. Proc Natl Acad Sci U S A. 1985 Jun;82(11):3665–3669. doi: 10.1073/pnas.82.11.3665. [DOI] [PMC free article] [PubMed] [Google Scholar]
- Gruner S. M., Parsegian V. A., Rand R. P. Directly measured deformation energy of phospholipid HII hexagonal phases. Faraday Discuss Chem Soc. 1986;(81):29–37. doi: 10.1039/dc9868100029. [DOI] [PubMed] [Google Scholar]
- Gruner S. M., Tate M. W., Kirk G. L., So P. T., Turner D. C., Keane D. T., Tilcock C. P., Cullis P. R. X-ray diffraction study of the polymorphic behavior of N-methylated dioleoylphosphatidylethanolamine. Biochemistry. 1988 Apr 19;27(8):2853–2866. doi: 10.1021/bi00408a029. [DOI] [PubMed] [Google Scholar]
- Helfrich W. Elastic properties of lipid bilayers: theory and possible experiments. Z Naturforsch C. 1973 Nov-Dec;28(11):693–703. doi: 10.1515/znc-1973-11-1209. [DOI] [PubMed] [Google Scholar]
- Hui S. W., Stewart T. P., Boni L. T., Yeagle P. L. Membrane fusion through point defects in bilayers. Science. 1981 May 22;212(4497):921–923. doi: 10.1126/science.7233185. [DOI] [PubMed] [Google Scholar]
- Keller S. L., Bezrukov S. M., Gruner S. M., Tate M. W., Vodyanoy I., Parsegian V. A. Probability of alamethicin conductance states varies with nonlamellar tendency of bilayer phospholipids. Biophys J. 1993 Jul;65(1):23–27. doi: 10.1016/S0006-3495(93)81040-3. [DOI] [PMC free article] [PubMed] [Google Scholar]
- Kozlov M. M., Leikin S. L., Chernomordik L. V., Markin V. S., Chizmadzhev Y. A. Stalk mechanism of vesicle fusion. Intermixing of aqueous contents. Eur Biophys J. 1989;17(3):121–129. doi: 10.1007/BF00254765. [DOI] [PubMed] [Google Scholar]
- Leikin S. L., Kozlov M. M., Chernomordik L. V., Markin V. S., Chizmadzhev Y. A. Membrane fusion: overcoming of the hydration barrier and local restructuring. J Theor Biol. 1987 Dec 21;129(4):411–425. doi: 10.1016/s0022-5193(87)80021-8. [DOI] [PubMed] [Google Scholar]
- Lentz B. R., McIntyre G. F., Parks D. J., Yates J. C., Massenburg D. Bilayer curvature and certain amphipaths promote poly(ethylene glycol)-induced fusion of dipalmitoylphosphatidylcholine unilamellar vesicles. Biochemistry. 1992 Mar 17;31(10):2643–2653. doi: 10.1021/bi00125a003. [DOI] [PubMed] [Google Scholar]
- Lewis R. N., Mannock D. A., McElhaney R. N., Turner D. C., Gruner S. M. Effect of fatty acyl chain length and structure on the lamellar gel to liquid-crystalline and lamellar to reversed hexagonal phase transitions of aqueous phosphatidylethanolamine dispersions. Biochemistry. 1989 Jan 24;28(2):541–548. doi: 10.1021/bi00428a020. [DOI] [PubMed] [Google Scholar]
- Markin V. S., Kozlov M. M., Borovjagin V. L. On the theory of membrane fusion. The stalk mechanism. Gen Physiol Biophys. 1984 Oct;3(5):361–377. [PubMed] [Google Scholar]
- Rand R. P., Fuller N. L., Gruner S. M., Parsegian V. A. Membrane curvature, lipid segregation, and structural transitions for phospholipids under dual-solvent stress. Biochemistry. 1990 Jan 9;29(1):76–87. doi: 10.1021/bi00453a010. [DOI] [PubMed] [Google Scholar]
- Ruigrok R. W., Hewat E. A., Wade R. H. Low pH deforms the influenza virus envelope. J Gen Virol. 1992 Apr;73(Pt 4):995–998. doi: 10.1099/0022-1317-73-4-995. [DOI] [PubMed] [Google Scholar]
- Seddon J. M. Structure of the inverted hexagonal (HII) phase, and non-lamellar phase transitions of lipids. Biochim Biophys Acta. 1990 Feb 28;1031(1):1–69. doi: 10.1016/0304-4157(90)90002-t. [DOI] [PubMed] [Google Scholar]
- Siegel D. P., Banschbach J. L. Lamellar/inverted cubic (L alpha/QII) phase transition in N-methylated dioleoylphosphatidylethanolamine. Biochemistry. 1990 Jun 26;29(25):5975–5981. doi: 10.1021/bi00477a014. [DOI] [PubMed] [Google Scholar]
- Siegel D. P., Banschbach J., Alford D., Ellens H., Lis L. J., Quinn P. J., Yeagle P. L., Bentz J. Physiological levels of diacylglycerols in phospholipid membranes induce membrane fusion and stabilize inverted phases. Biochemistry. 1989 May 2;28(9):3703–3709. doi: 10.1021/bi00435a012. [DOI] [PubMed] [Google Scholar]
- Siegel D. P. Inverted micellar intermediates and the transitions between lamellar, cubic, and inverted hexagonal amphiphile phases. III. Isotropic and inverted cubic state formation via intermediates in transitions between L alpha and HII phases. Chem Phys Lipids. 1986 Dec 31;42(4):279–301. doi: 10.1016/0009-3084(86)90087-3. [DOI] [PubMed] [Google Scholar]
- Siegel D. P. Inverted micellar intermediates and the transitions between lamellar, cubic, and inverted hexagonal lipid phases. I. Mechanism of the L alpha----HII phase transitions. Biophys J. 1986 Jun;49(6):1155–1170. doi: 10.1016/S0006-3495(86)83744-4. [DOI] [PMC free article] [PubMed] [Google Scholar]
- Siegel D. P. Inverted micellar intermediates and the transitions between lamellar, cubic, and inverted hexagonal lipid phases. II. Implications for membrane-membrane interactions and membrane fusion. Biophys J. 1986 Jun;49(6):1171–1183. doi: 10.1016/S0006-3495(86)83745-6. [DOI] [PMC free article] [PubMed] [Google Scholar]
- Siegel D. P. Inverted micellar structures in bilayer membranes. Formation rates and half-lives. Biophys J. 1984 Feb;45(2):399–420. doi: 10.1016/S0006-3495(84)84164-8. [DOI] [PMC free article] [PubMed] [Google Scholar]
- Sjölund M., Lindblom G., Rilfors L., Arvidson G. Hydrophobic molecules in lecithin-water systems. I. Formation of reversed hexagonal phases at high and low water contents. Biophys J. 1987 Aug;52(2):145–153. doi: 10.1016/S0006-3495(87)83202-2. [DOI] [PMC free article] [PubMed] [Google Scholar]
- Sjölund M., Rilfors L., Lindblom G. Reversed hexagonal phase formation in lecithin-alkane-water systems with different acyl chain unsaturation and alkane length. Biochemistry. 1989 Feb 7;28(3):1323–1329. doi: 10.1021/bi00429a057. [DOI] [PubMed] [Google Scholar]
- Stamatotos L., Silvius J. R. Effects of cholesterol on the divalent cation-mediated interactions of vesicles containing amino and choline phospholipids. Biochim Biophys Acta. 1987 Nov 27;905(1):81–90. doi: 10.1016/0005-2736(87)90011-3. [DOI] [PubMed] [Google Scholar]
- Tate M. W., Eikenberry E. F., Turner D. C., Shyamsunder E., Gruner S. M. Nonbilayer phases of membrane lipids. Chem Phys Lipids. 1991 Mar;57(2-3):147–164. doi: 10.1016/0009-3084(91)90073-k. [DOI] [PubMed] [Google Scholar]
- Tate M. W., Gruner S. M. Lipid polymorphism of mixtures of dioleoylphosphatidylethanolamine and saturated and monounsaturated phosphatidylcholines of various chain lengths. Biochemistry. 1987 Jan 13;26(1):231–236. doi: 10.1021/bi00375a031. [DOI] [PubMed] [Google Scholar]
- Tate M. W., Gruner S. M. Temperature dependence of the structural dimensions of the inverted hexagonal (HII) phase of phosphatidylethanolamine-containing membranes. Biochemistry. 1989 May 16;28(10):4245–4253. doi: 10.1021/bi00436a019. [DOI] [PubMed] [Google Scholar]
- Turner D. C., Gruner S. M. X-ray diffraction reconstruction of the inverted hexagonal (HII) phase in lipid-water systems. Biochemistry. 1992 Feb 11;31(5):1340–1355. doi: 10.1021/bi00120a009. [DOI] [PubMed] [Google Scholar]
- Verkleij A. J. Lipidic intramembranous particles. Biochim Biophys Acta. 1984 Jan 27;779(1):43–63. doi: 10.1016/0304-4157(84)90003-0. [DOI] [PubMed] [Google Scholar]
- Verkleij A. J., van Echteld C. J., Gerritsen W. J., Cullis P. R., de Kruijff B. The lipidic particle as an intermediate structure in membrane fusion processes and bilayer to hexagonal HII transitions. Biochim Biophys Acta. 1980 Aug 14;600(3):620–624. doi: 10.1016/0005-2736(80)90465-4. [DOI] [PubMed] [Google Scholar]
- Walter A., Siegel D. P. Divalent cation-induced lipid mixing between phosphatidylserine liposomes studied by stopped-flow fluorescence measurements: effects of temperature, comparison of barium and calcium, and perturbation by DPX. Biochemistry. 1993 Apr 6;32(13):3271–3281. doi: 10.1021/bi00064a009. [DOI] [PubMed] [Google Scholar]
- White J. M. Membrane fusion. Science. 1992 Nov 6;258(5084):917–924. doi: 10.1126/science.1439803. [DOI] [PubMed] [Google Scholar]
- Wilschut J., Scholma J., Eastman S. J., Hope M. J., Cullis P. R. Ca(2+)-induced fusion of phospholipid vesicles containing free fatty acids: modulation by transmembrane pH gradients. Biochemistry. 1992 Mar 17;31(10):2629–2636. doi: 10.1021/bi00125a001. [DOI] [PubMed] [Google Scholar]