Abstract
Cells of the bacterium Escherichia coli were tethered and spun in a high-frequency rotating electric field at a series of discrete field strengths. This was done first at low field strengths, then at field strengths generating speeds high enough to disrupt motor function, and finally at low field strengths. Comparison of the initial and final speed versus applied-torque plots yielded relative motor torque. For backward rotation, motor torque rose steeply at speeds close to zero, peaking, on average, at about 2.2 times the stall torque. For forward rotation, motor torque remained approximately constant up to speeds of about 60% of the zero-torque speed. Then the torque dropped linearly with speed, crossed zero, and reached a minimum, on average, at about -1.7 times the stall torque. The zero-torque speed increased with temperature (about 90 Hz at 11 degrees C, 140 Hz at 16 degrees C, and 290 Hz at 23 degrees C), while other parameters remained approximately constant. Sometimes the motor slipped at either extreme (delivered constant torque over a range of speeds), but eventually it broke. Similar results were obtained whether motors broke catastrophically (suddenly and completely) or progressively or were de-energized by brief treatment with an uncoupler. These results are consistent with a tightly coupled ratchet mechanism, provided that elastic deformation of force-generating elements is limited by a stop and that mechanical components yield at high applied torques.
Full text
PDF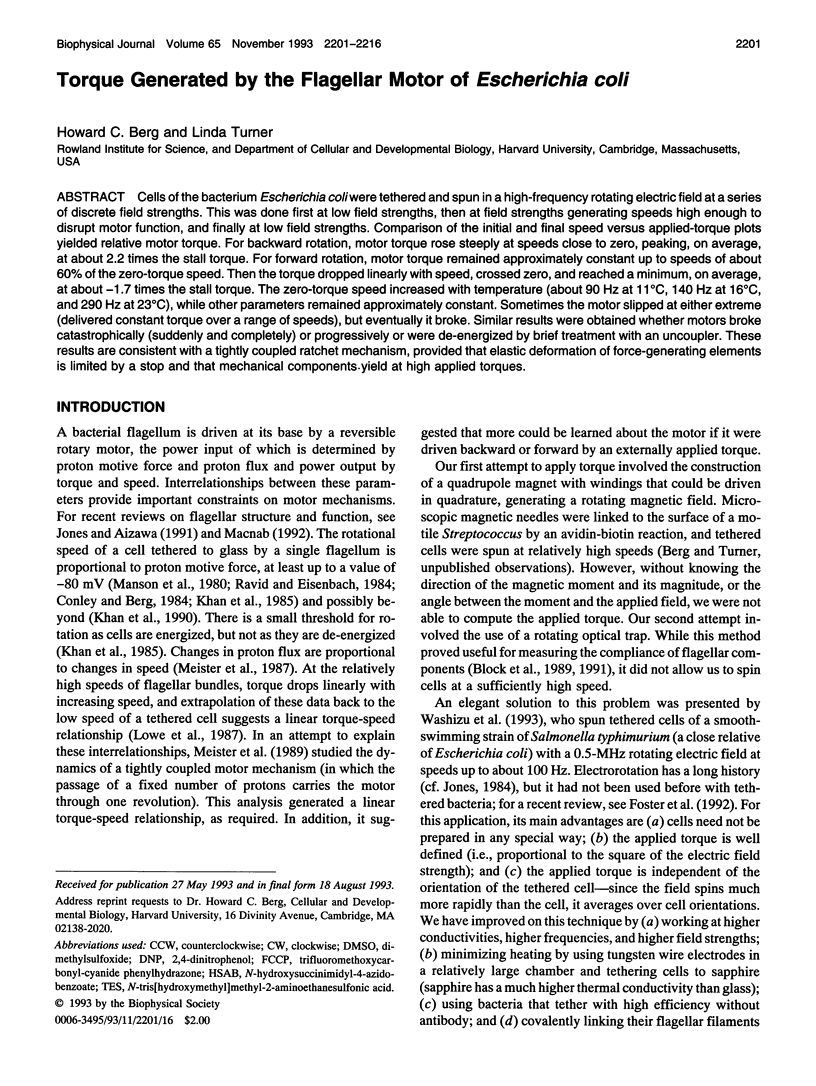
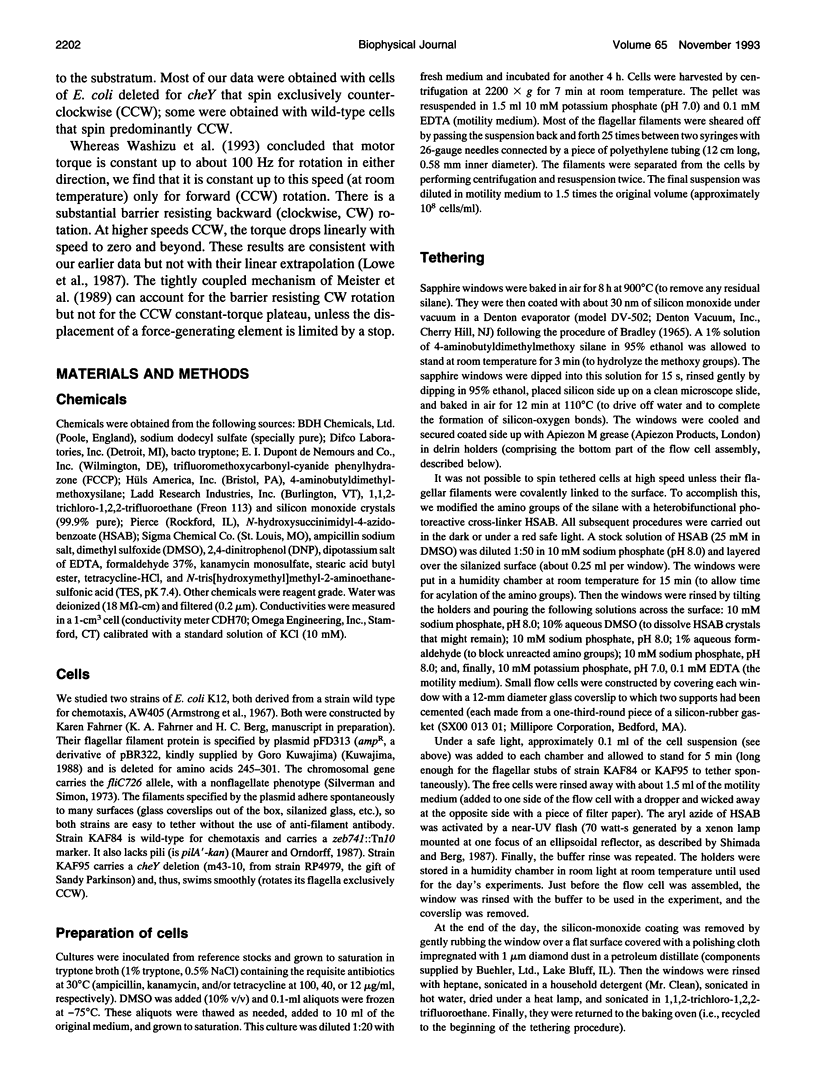
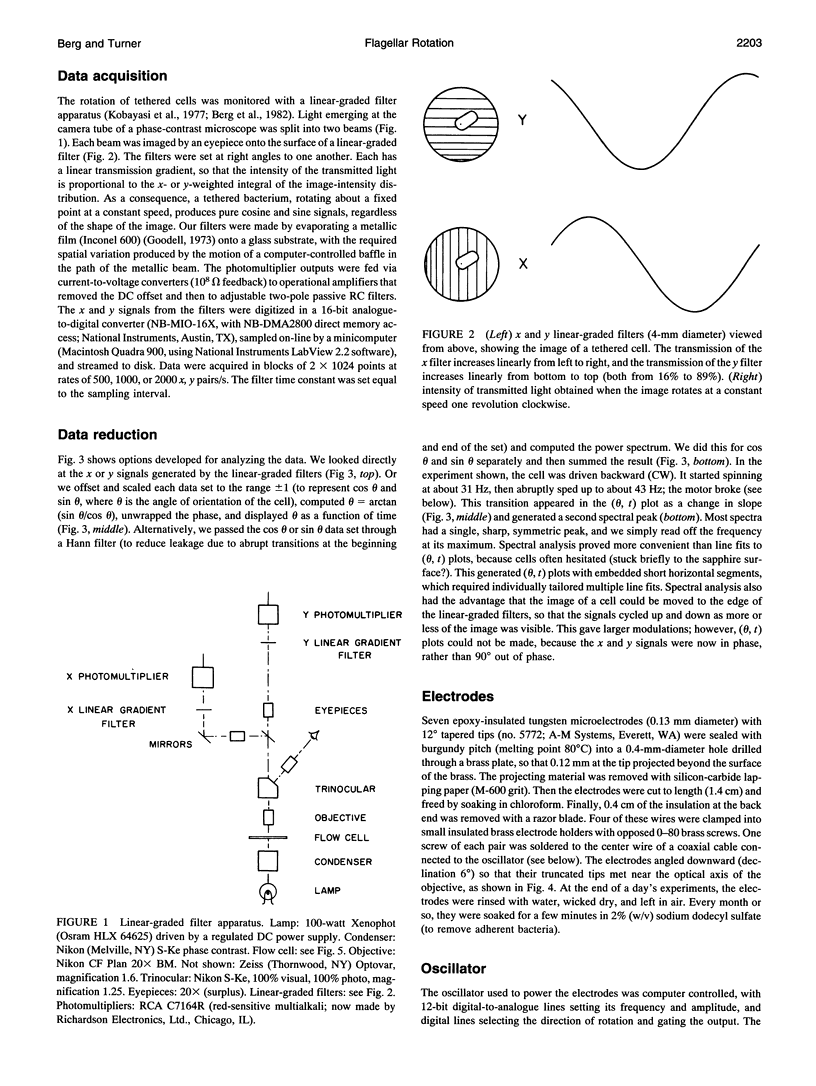
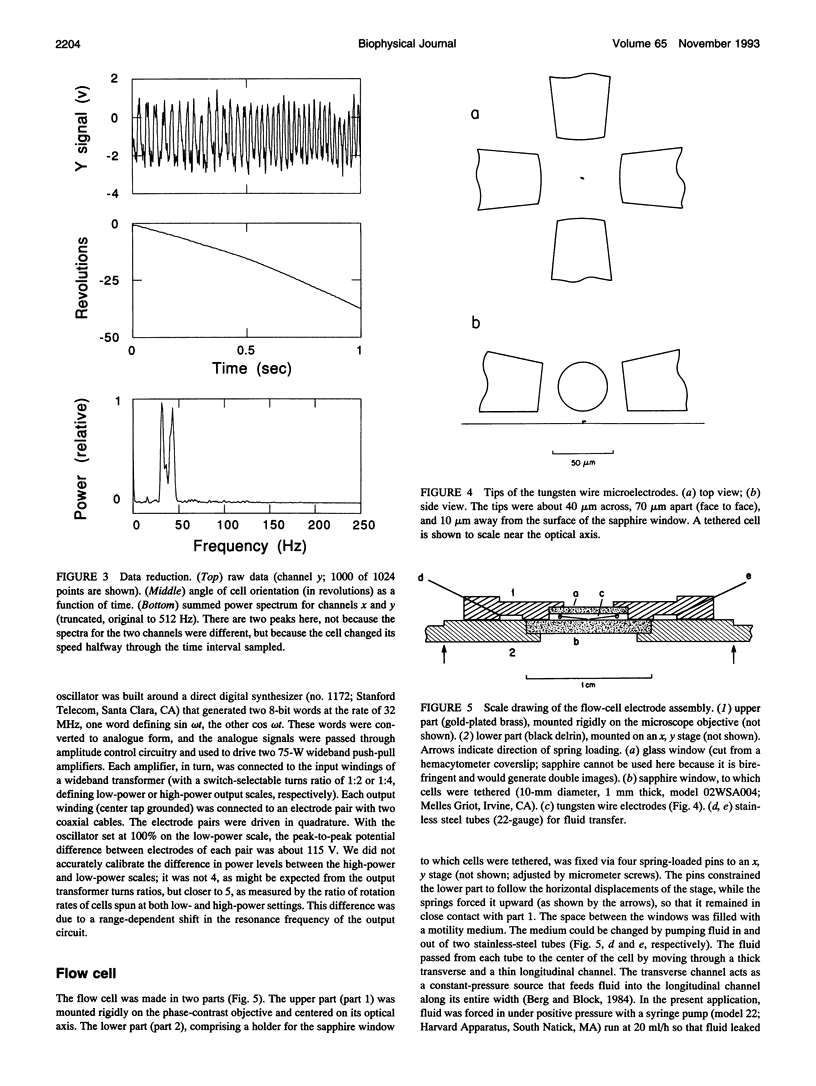
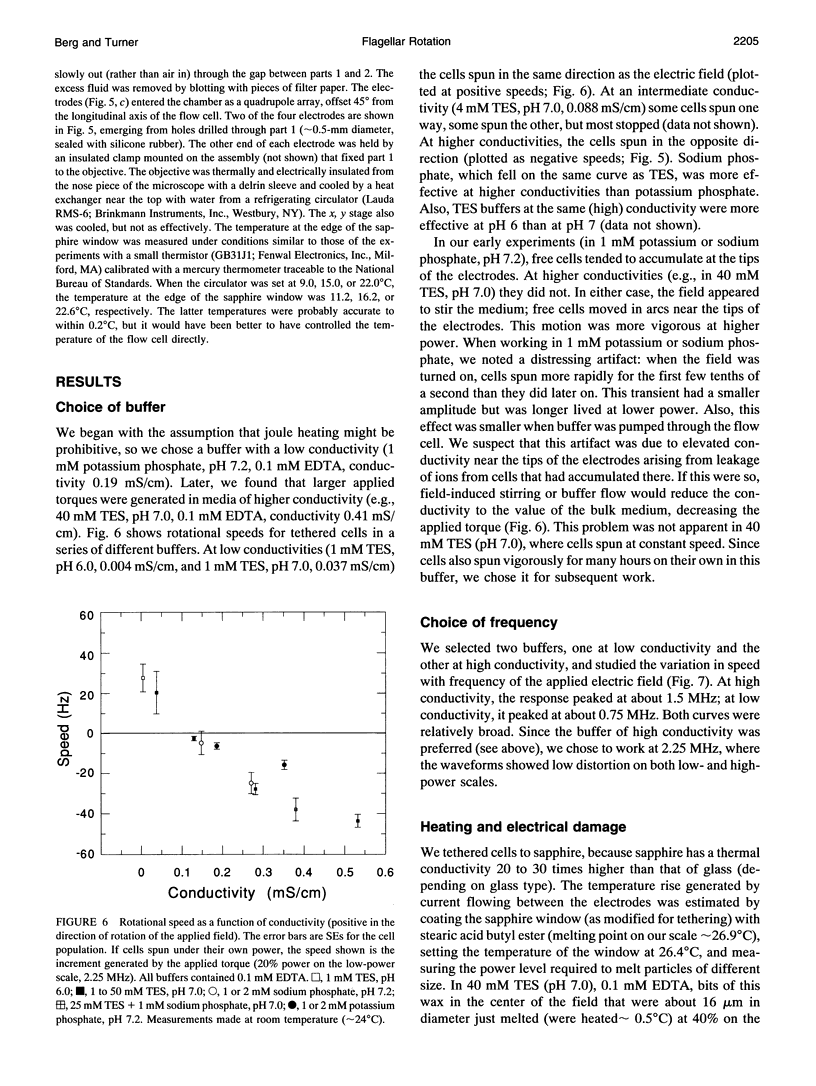
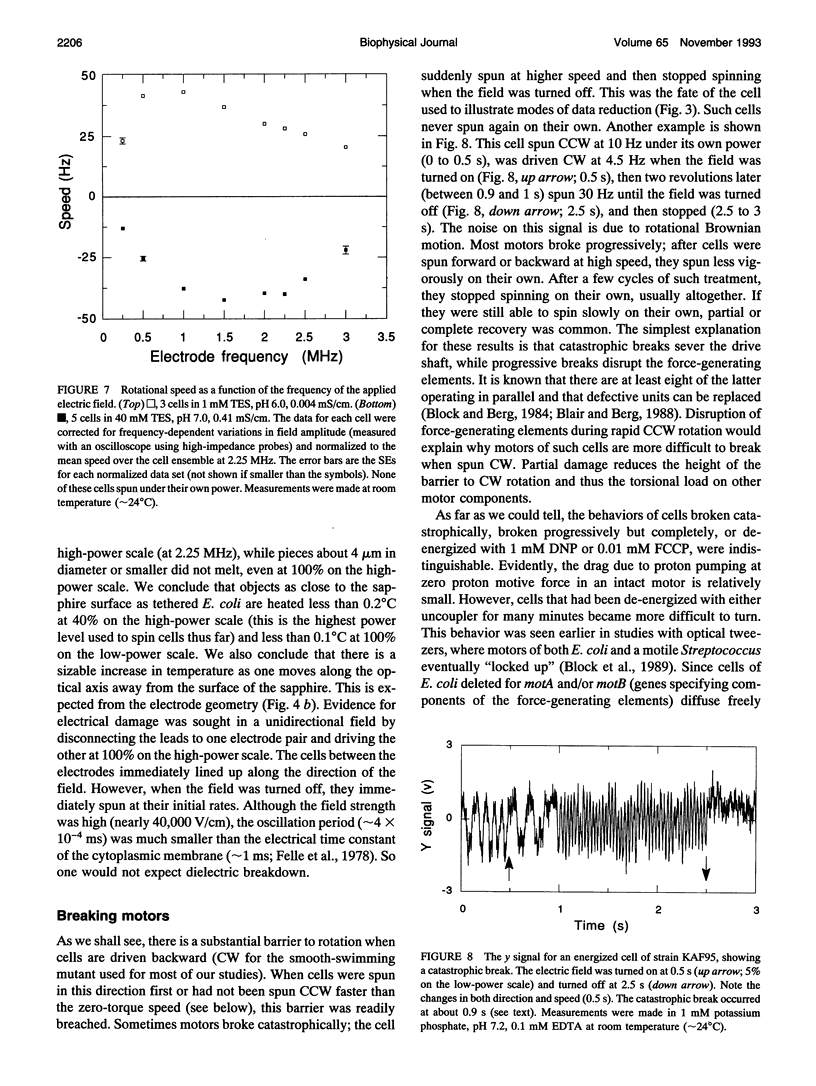
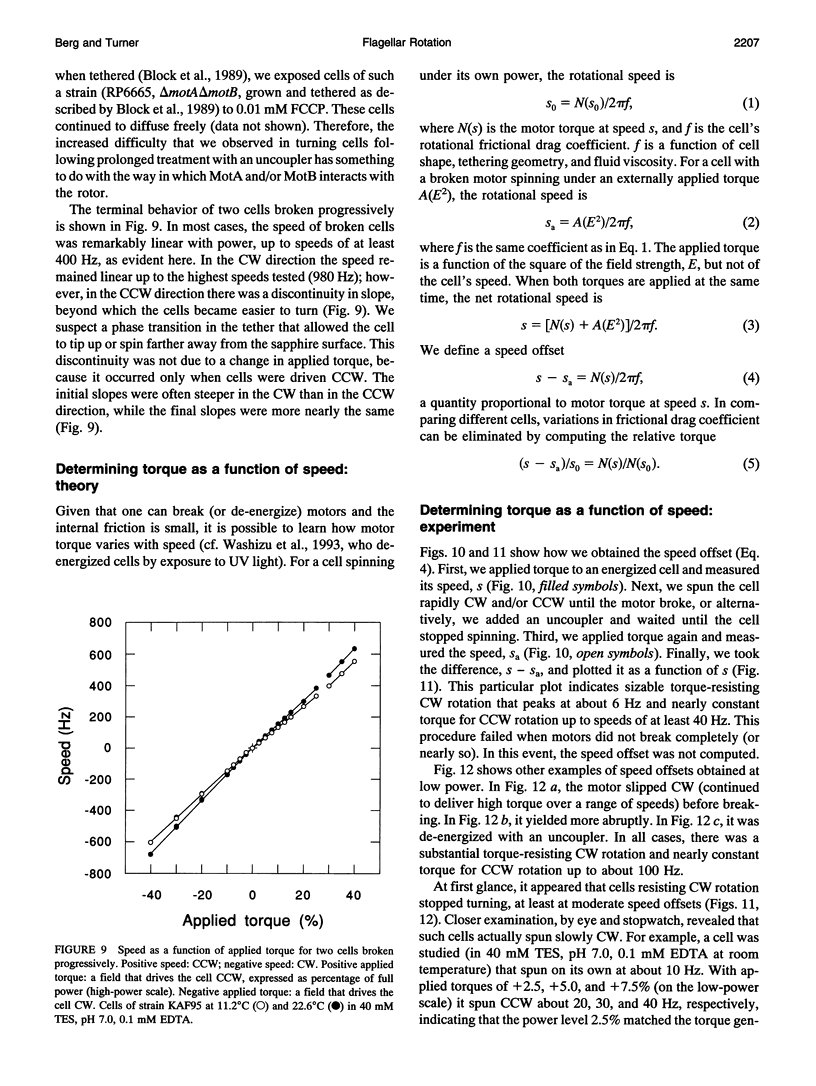
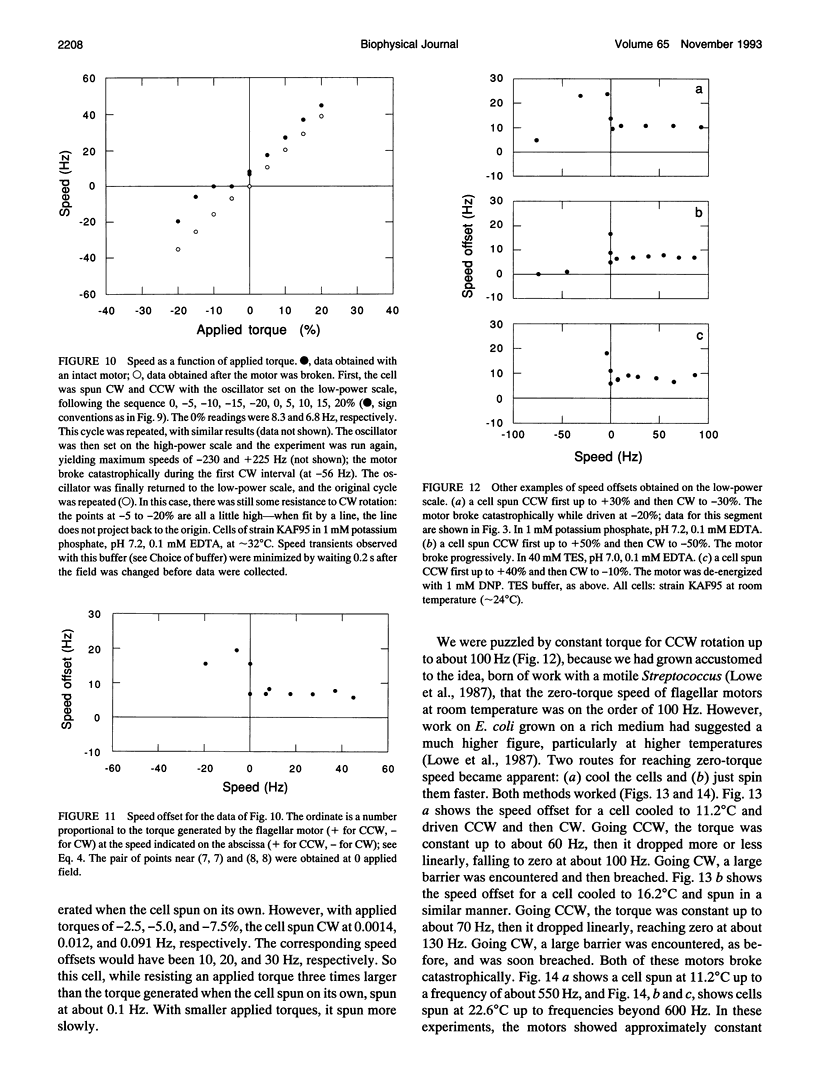
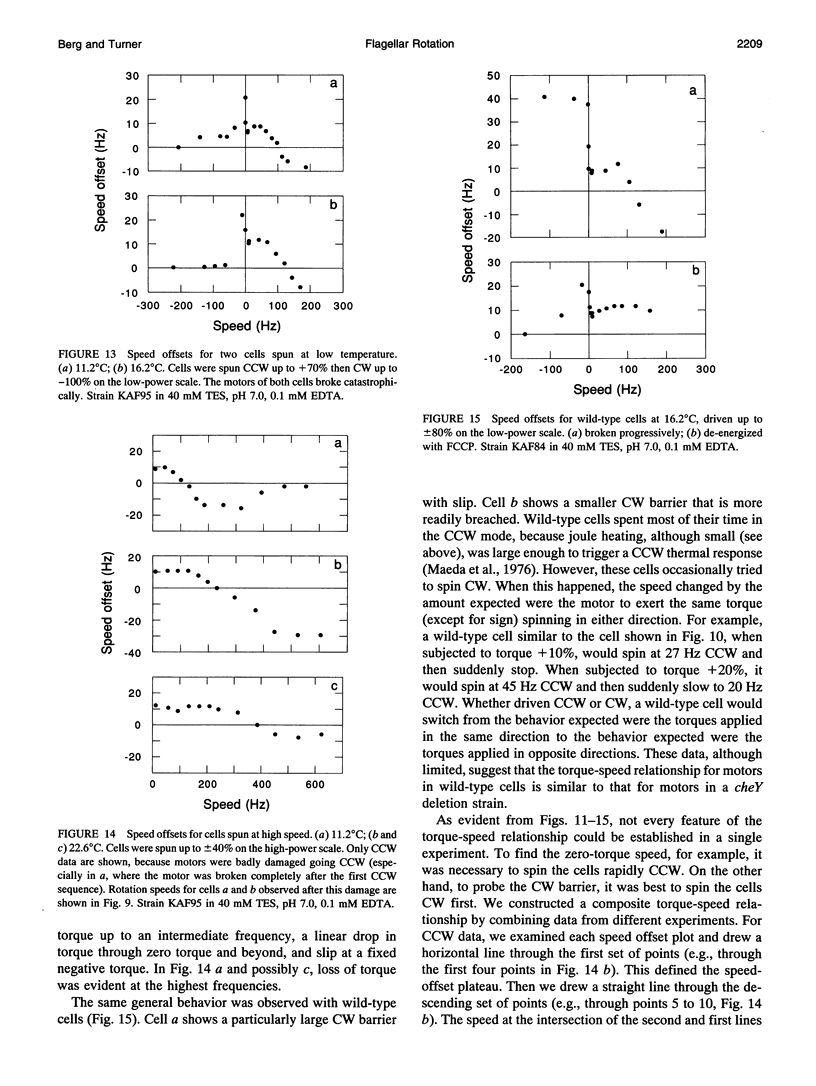
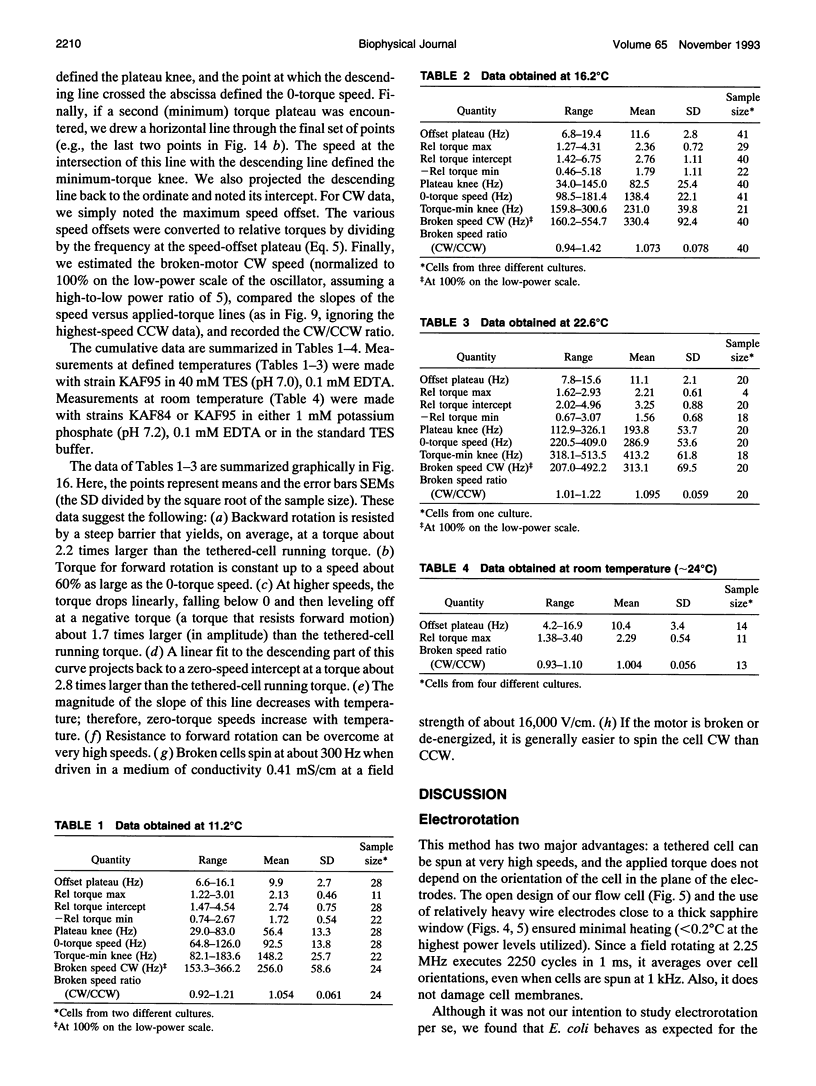
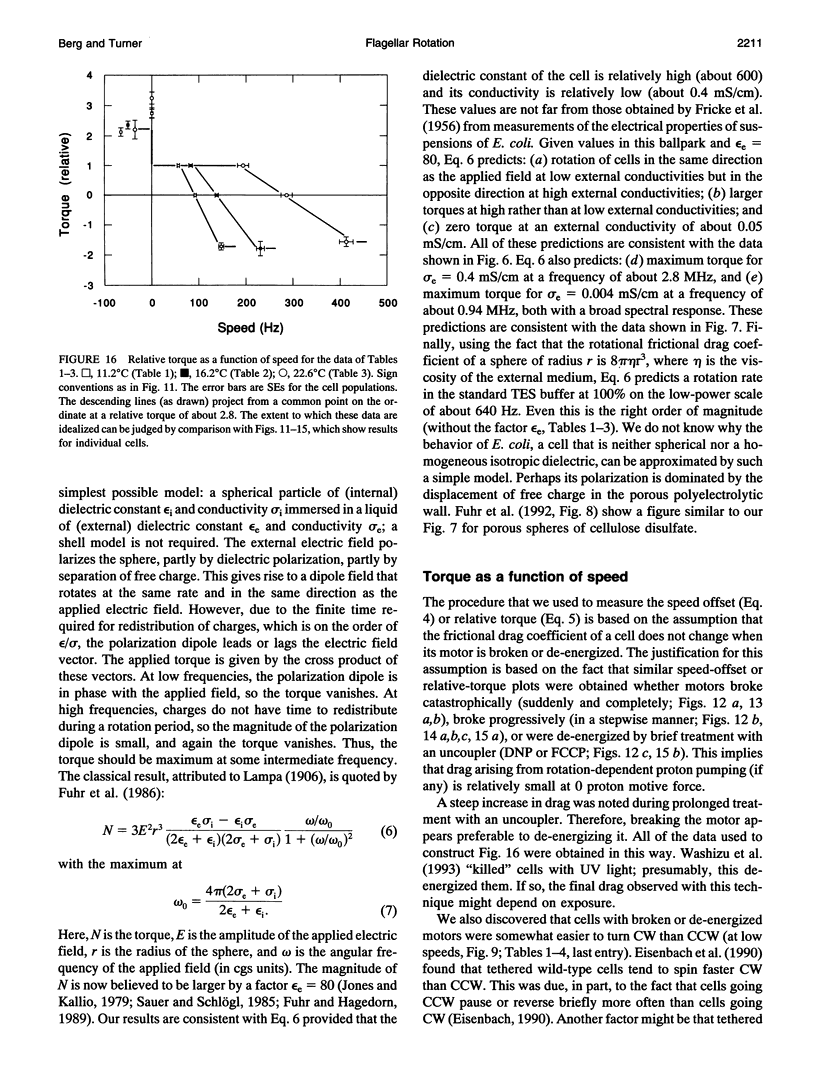
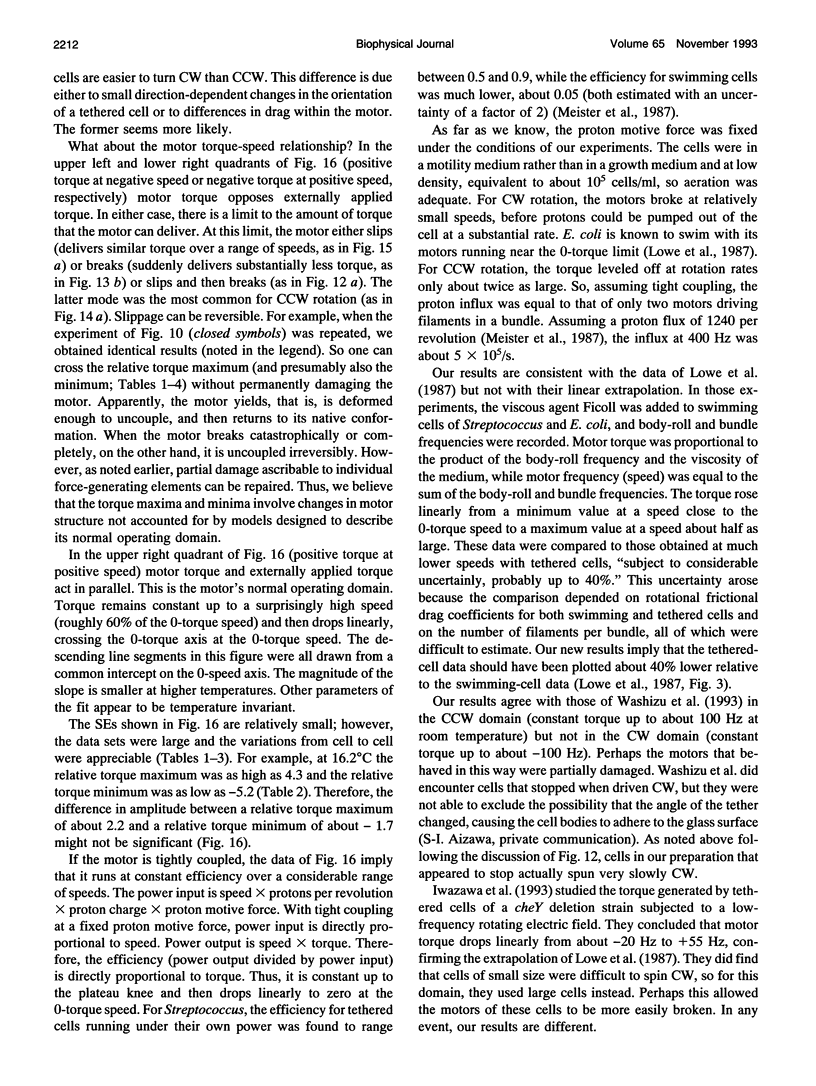
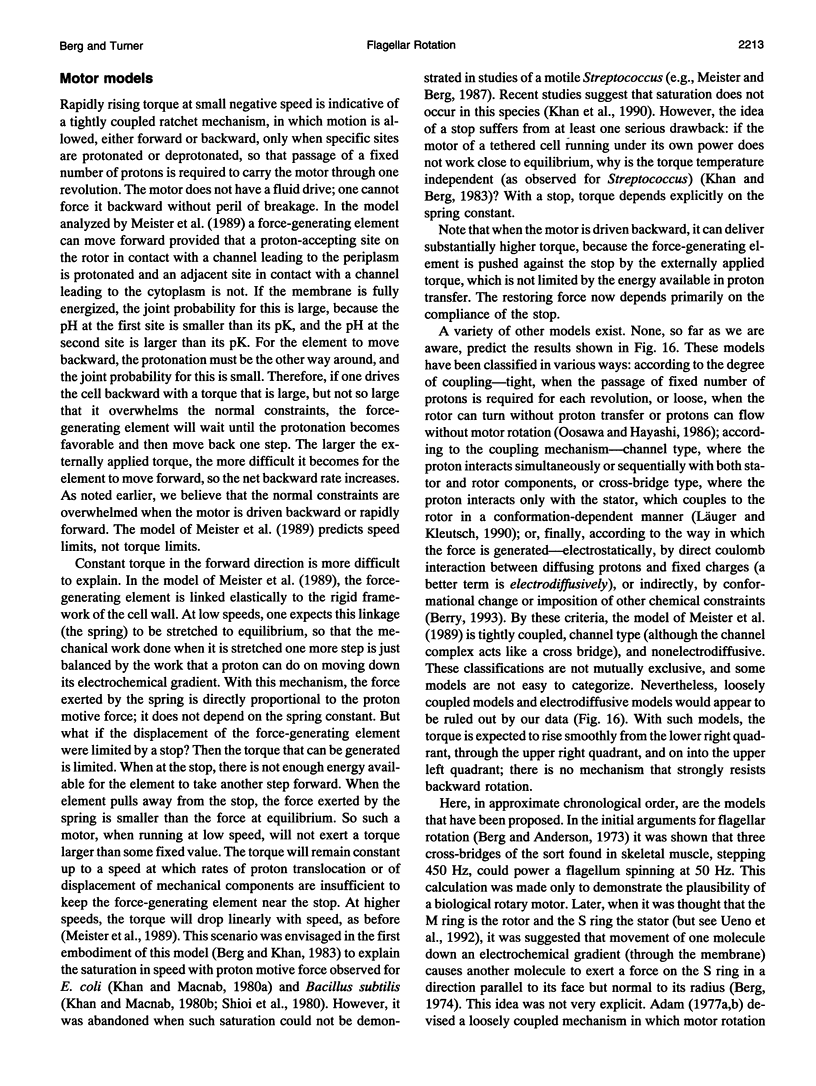
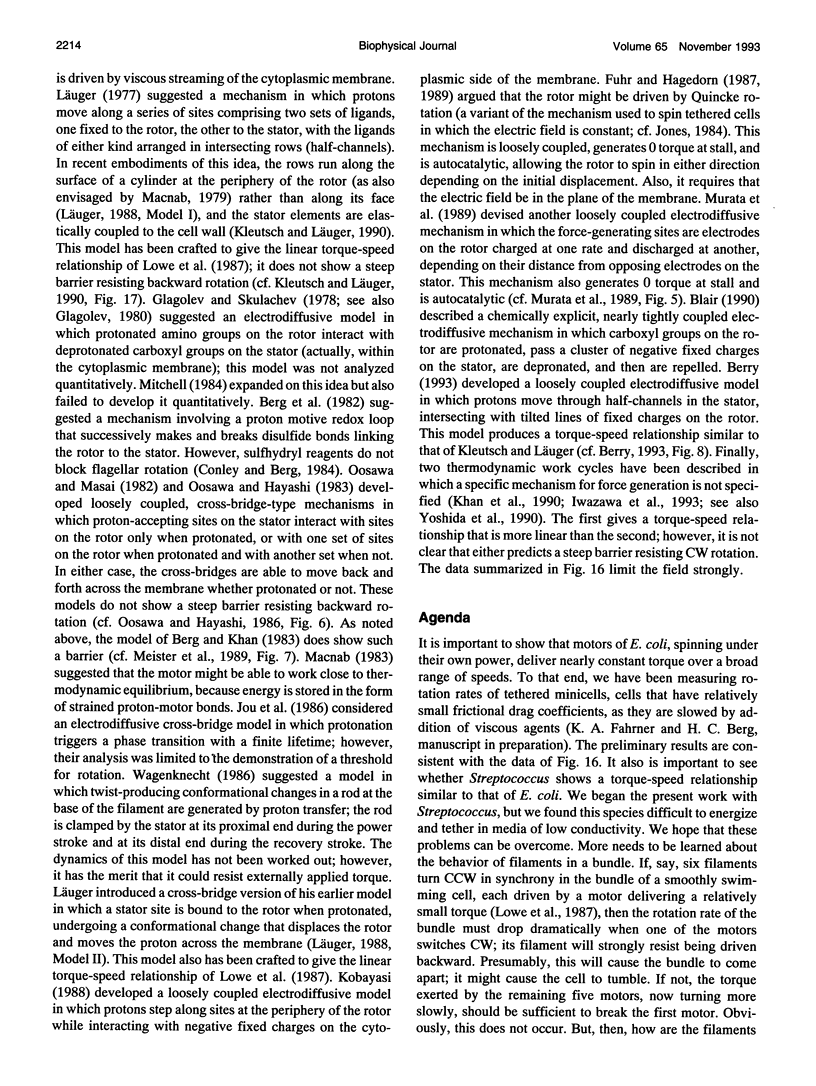
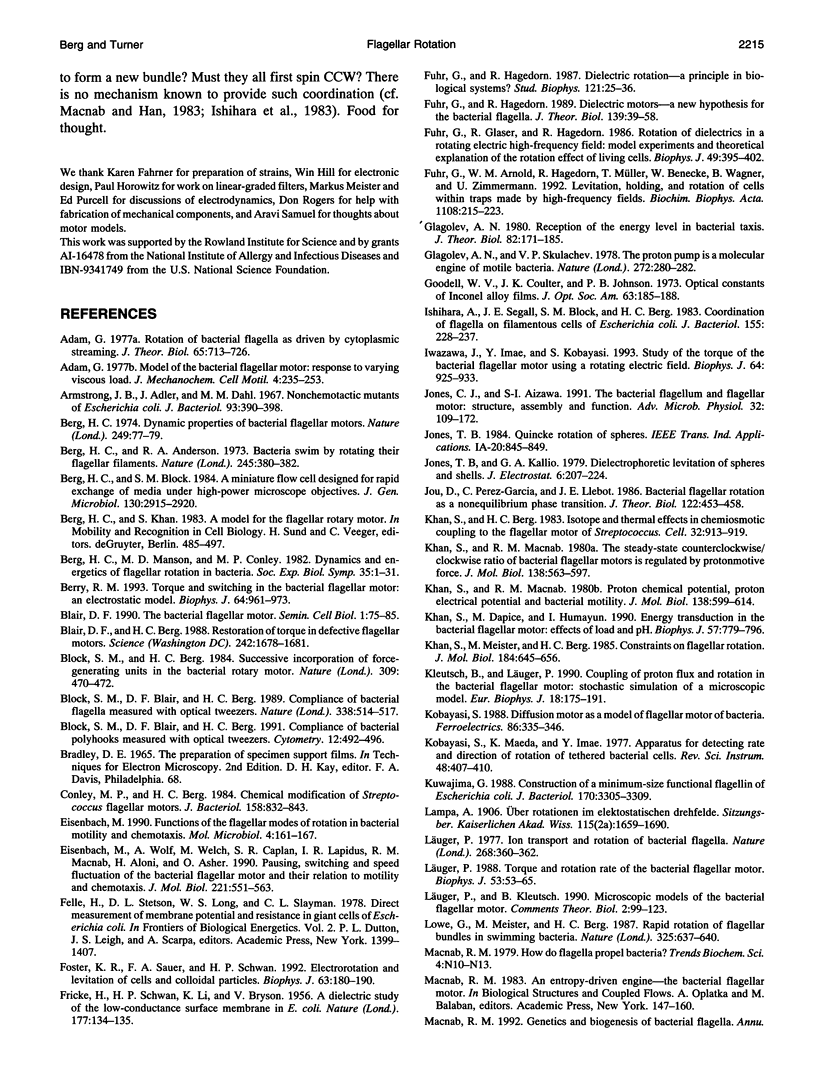
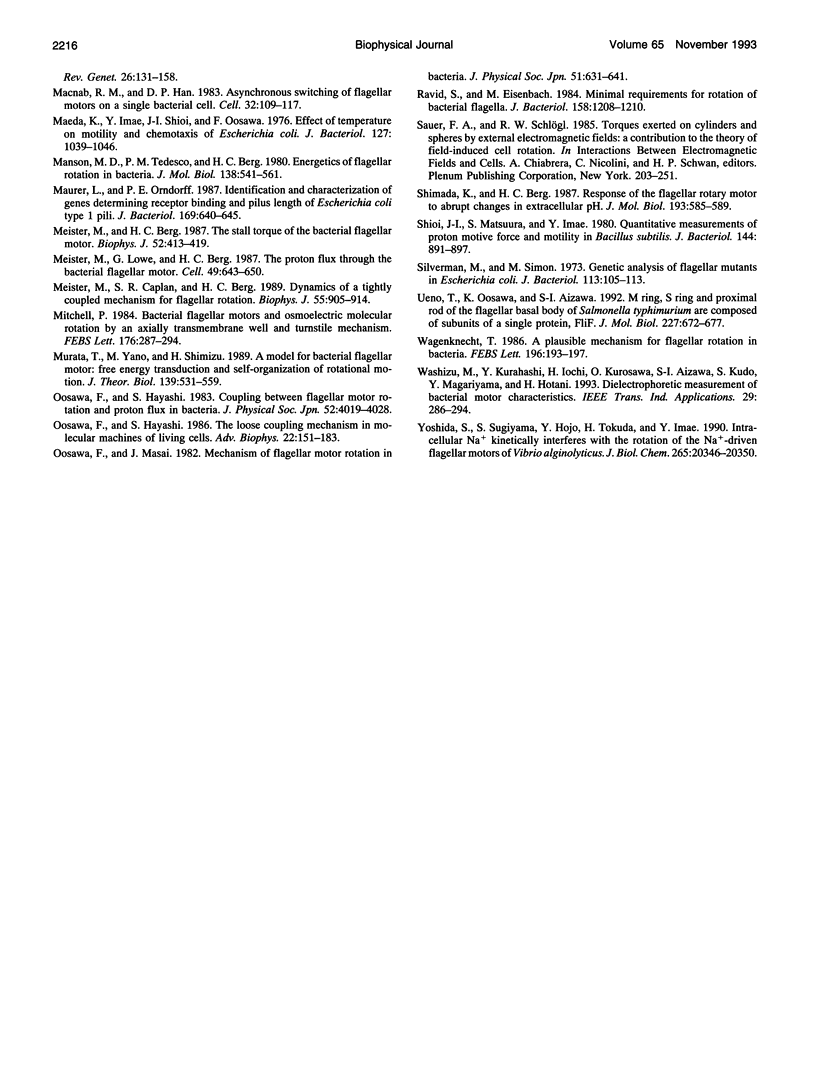
Selected References
These references are in PubMed. This may not be the complete list of references from this article.
- Adam G. Model of the bacterial flagellar motor: response to varying viscous load. J Mechanochem Cell Motil. 1977 Dec;4(4):235–253. [PubMed] [Google Scholar]
- Adam G. Rotation of bacterial flagella as driven by cytomembrane streaming. J Theor Biol. 1977 Apr 21;65(4):713–726. doi: 10.1016/0022-5193(77)90017-0. [DOI] [PubMed] [Google Scholar]
- Armstrong J. B., Adler J., Dahl M. M. Nonchemotactic mutants of Escherichia coli. J Bacteriol. 1967 Jan;93(1):390–398. doi: 10.1128/jb.93.1.390-398.1967. [DOI] [PMC free article] [PubMed] [Google Scholar]
- Berg H. C., Anderson R. A. Bacteria swim by rotating their flagellar filaments. Nature. 1973 Oct 19;245(5425):380–382. doi: 10.1038/245380a0. [DOI] [PubMed] [Google Scholar]
- Berg H. C., Block S. M. A miniature flow cell designed for rapid exchange of media under high-power microscope objectives. J Gen Microbiol. 1984 Nov;130(11):2915–2920. doi: 10.1099/00221287-130-11-2915. [DOI] [PubMed] [Google Scholar]
- Berg H. C. Dynamic properties of bacterial flagellar motors. Nature. 1974 May 3;249(452):77–79. doi: 10.1038/249077a0. [DOI] [PubMed] [Google Scholar]
- Berg H. C., Manson M. D., Conley M. P. Dynamics and energetics of flagellar rotation in bacteria. Symp Soc Exp Biol. 1982;35:1–31. [PubMed] [Google Scholar]
- Berry R. M. Torque and switching in the bacterial flagellar motor. An electrostatic model. Biophys J. 1993 Apr;64(4):961–973. doi: 10.1016/S0006-3495(93)81462-0. [DOI] [PMC free article] [PubMed] [Google Scholar]
- Blair D. F., Berg H. C. Restoration of torque in defective flagellar motors. Science. 1988 Dec 23;242(4886):1678–1681. doi: 10.1126/science.2849208. [DOI] [PubMed] [Google Scholar]
- Blair D. F. The bacterial flagellar motor. Semin Cell Biol. 1990 Apr;1(2):75–85. [PubMed] [Google Scholar]
- Block S. M., Berg H. C. Successive incorporation of force-generating units in the bacterial rotary motor. 1984 May 31-Jun 6Nature. 309(5967):470–472. doi: 10.1038/309470a0. [DOI] [PubMed] [Google Scholar]
- Block S. M., Blair D. F., Berg H. C. Compliance of bacterial flagella measured with optical tweezers. Nature. 1989 Apr 6;338(6215):514–518. doi: 10.1038/338514a0. [DOI] [PubMed] [Google Scholar]
- Block S. M., Blair D. F., Berg H. C. Compliance of bacterial polyhooks measured with optical tweezers. Cytometry. 1991;12(6):492–496. doi: 10.1002/cyto.990120605. [DOI] [PubMed] [Google Scholar]
- Conley M. P., Berg H. C. Chemical modification of Streptococcus flagellar motors. J Bacteriol. 1984 Jun;158(3):832–843. doi: 10.1128/jb.158.3.832-843.1984. [DOI] [PMC free article] [PubMed] [Google Scholar]
- Eisenbach M. Functions of the flagellar modes of rotation in bacterial motility and chemotaxis. Mol Microbiol. 1990 Feb;4(2):161–167. doi: 10.1111/j.1365-2958.1990.tb00584.x. [DOI] [PubMed] [Google Scholar]
- Eisenbach M., Wolf A., Welch M., Caplan S. R., Lapidus I. R., Macnab R. M., Aloni H., Asher O. Pausing, switching and speed fluctuation of the bacterial flagellar motor and their relation to motility and chemotaxis. J Mol Biol. 1990 Feb 5;211(3):551–563. doi: 10.1016/0022-2836(90)90265-N. [DOI] [PubMed] [Google Scholar]
- FRICKE H., SCHWAN H. P., LI K., BRYSON V. A dielectric study of the low-conductance surface membrane in E. coli. Nature. 1956 Jan 21;177(4499):134–135. doi: 10.1038/177134a0. [DOI] [PubMed] [Google Scholar]
- Foster K. R., Sauer F. A., Schwan H. P. Electrorotation and levitation of cells and colloidal particles. Biophys J. 1992 Jul;63(1):180–190. doi: 10.1016/S0006-3495(92)81588-6. [DOI] [PMC free article] [PubMed] [Google Scholar]
- Fuhr G., Arnold W. M., Hagedorn R., Müller T., Benecke W., Wagner B., Zimmermann U. Levitation, holding, and rotation of cells within traps made by high-frequency fields. Biochim Biophys Acta. 1992 Jul 27;1108(2):215–223. doi: 10.1016/0005-2736(92)90028-k. [DOI] [PubMed] [Google Scholar]
- Fuhr G., Glaser R., Hagedorn R. Rotation of dielectrics in a rotating electric high-frequency field. Model experiments and theoretical explanation of the rotation effect of living cells. Biophys J. 1986 Feb;49(2):395–402. doi: 10.1016/S0006-3495(86)83649-9. [DOI] [PMC free article] [PubMed] [Google Scholar]
- Glagolev A. N. Reception of the energy level in bacterial taxis. J Theor Biol. 1980 Jan 21;82(2):171–185. doi: 10.1016/0022-5193(80)90097-1. [DOI] [PubMed] [Google Scholar]
- Glagolev A. N., Skulachev V. P. The proton pump is a molecular engine of motile bacteria. Nature. 1978 Mar 16;272(5650):280–282. doi: 10.1038/272280a0. [DOI] [PubMed] [Google Scholar]
- Ishihara A., Segall J. E., Block S. M., Berg H. C. Coordination of flagella on filamentous cells of Escherichia coli. J Bacteriol. 1983 Jul;155(1):228–237. doi: 10.1128/jb.155.1.228-237.1983. [DOI] [PMC free article] [PubMed] [Google Scholar]
- Iwazawa J., Imae Y., Kobayasi S. Study of the torque of the bacterial flagellar motor using a rotating electric field. Biophys J. 1993 Mar;64(3):925–933. doi: 10.1016/S0006-3495(93)81454-1. [DOI] [PMC free article] [PubMed] [Google Scholar]
- Jones C. J., Aizawa S. The bacterial flagellum and flagellar motor: structure, assembly and function. Adv Microb Physiol. 1991;32:109–172. doi: 10.1016/s0065-2911(08)60007-7. [DOI] [PubMed] [Google Scholar]
- Khan S., Berg H. C. Isotope and thermal effects in chemiosmotic coupling to the flagellar motor of Streptococcus. Cell. 1983 Mar;32(3):913–919. doi: 10.1016/0092-8674(83)90076-4. [DOI] [PubMed] [Google Scholar]
- Khan S., Dapice M., Humayun I. Energy transduction in the bacterial flagellar motor. Effects of load and pH. Biophys J. 1990 Apr;57(4):779–796. doi: 10.1016/S0006-3495(90)82598-4. [DOI] [PMC free article] [PubMed] [Google Scholar]
- Khan S., Macnab R. M. Proton chemical potential, proton electrical potential and bacterial motility. J Mol Biol. 1980 Apr 15;138(3):599–614. doi: 10.1016/s0022-2836(80)80019-2. [DOI] [PubMed] [Google Scholar]
- Khan S., Macnab R. M. The steady-state counterclockwise/clockwise ratio of bacterial flagellar motors is regulated by protonmotive force. J Mol Biol. 1980 Apr 15;138(3):563–597. doi: 10.1016/s0022-2836(80)80018-0. [DOI] [PubMed] [Google Scholar]
- Khan S., Meister M., Berg H. C. Constraints on flagellar rotation. J Mol Biol. 1985 Aug 20;184(4):645–656. doi: 10.1016/0022-2836(85)90310-9. [DOI] [PubMed] [Google Scholar]
- Kobayasi S., Maeda K., Imae Y. Apparatus for detecting rate and direction of rotation of tethered bacterial cells. Rev Sci Instrum. 1977 Apr;48(4):407–410. doi: 10.1063/1.1135033. [DOI] [PubMed] [Google Scholar]
- Kuwajima G. Construction of a minimum-size functional flagellin of Escherichia coli. J Bacteriol. 1988 Jul;170(7):3305–3309. doi: 10.1128/jb.170.7.3305-3309.1988. [DOI] [PMC free article] [PubMed] [Google Scholar]
- Läuger P. Ion transport and rotation of bacterial flagella. Nature. 1977 Jul 28;268(5618):360–362. doi: 10.1038/268360a0. [DOI] [PubMed] [Google Scholar]
- Macnab R. M. Genetics and biogenesis of bacterial flagella. Annu Rev Genet. 1992;26:131–158. doi: 10.1146/annurev.ge.26.120192.001023. [DOI] [PubMed] [Google Scholar]
- Macnab R. M., Han D. P. Asynchronous switching of flagellar motors on a single bacterial cell. Cell. 1983 Jan;32(1):109–117. doi: 10.1016/0092-8674(83)90501-9. [DOI] [PubMed] [Google Scholar]
- Maeda K., Imae Y., Shioi J. I., Oosawa F. Effect of temperature on motility and chemotaxis of Escherichia coli. J Bacteriol. 1976 Sep;127(3):1039–1046. doi: 10.1128/jb.127.3.1039-1046.1976. [DOI] [PMC free article] [PubMed] [Google Scholar]
- Manson M. D., Tedesco P. M., Berg H. C. Energetics of flagellar rotation in bacteria. J Mol Biol. 1980 Apr 15;138(3):541–561. doi: 10.1016/s0022-2836(80)80017-9. [DOI] [PubMed] [Google Scholar]
- Maurer L., Orndorff P. E. Identification and characterization of genes determining receptor binding and pilus length of Escherichia coli type 1 pili. J Bacteriol. 1987 Feb;169(2):640–645. doi: 10.1128/jb.169.2.640-645.1987. [DOI] [PMC free article] [PubMed] [Google Scholar]
- Meister M., Berg H. C. The stall torque of the bacterial flagellar motor. Biophys J. 1987 Sep;52(3):413–419. doi: 10.1016/S0006-3495(87)83230-7. [DOI] [PMC free article] [PubMed] [Google Scholar]
- Meister M., Caplan S. R., Berg H. C. Dynamics of a tightly coupled mechanism for flagellar rotation. Bacterial motility, chemiosmotic coupling, protonmotive force. Biophys J. 1989 May;55(5):905–914. doi: 10.1016/S0006-3495(89)82889-9. [DOI] [PMC free article] [PubMed] [Google Scholar]
- Meister M., Lowe G., Berg H. C. The proton flux through the bacterial flagellar motor. Cell. 1987 Jun 5;49(5):643–650. doi: 10.1016/0092-8674(87)90540-x. [DOI] [PubMed] [Google Scholar]
- Murata T., Yano M., Shimizu H. A model for bacterial flagellar motor: free energy transduction and self-organization of rotational motion. J Theor Biol. 1989 Aug 22;139(4):531–559. doi: 10.1016/s0022-5193(89)80069-4. [DOI] [PubMed] [Google Scholar]
- Oosawa F., Hayashi S. The loose coupling mechanism in molecular machines of living cells. Adv Biophys. 1986;22:151–183. doi: 10.1016/0065-227x(86)90005-5. [DOI] [PubMed] [Google Scholar]
- Ravid S., Eisenbach M. Minimal requirements for rotation of bacterial flagella. J Bacteriol. 1984 Jun;158(3):1208–1210. doi: 10.1128/jb.158.3.1208-1210.1984. [DOI] [PMC free article] [PubMed] [Google Scholar]
- Shimada K., Berg H. C. Response of the flagellar rotary motor to abrupt changes in extracellular pH. J Mol Biol. 1987 Feb 5;193(3):585–589. doi: 10.1016/0022-2836(87)90269-5. [DOI] [PubMed] [Google Scholar]
- Shioi J. I., Matsuura S., Imae Y. Quantitative measurements of proton motive force and motility in Bacillus subtilis. J Bacteriol. 1980 Dec;144(3):891–897. doi: 10.1128/jb.144.3.891-897.1980. [DOI] [PMC free article] [PubMed] [Google Scholar]
- Silverman M., Simon M. Genetic analysis of flagellar mutants in Escherichia coli. J Bacteriol. 1973 Jan;113(1):105–113. doi: 10.1128/jb.113.1.105-113.1973. [DOI] [PMC free article] [PubMed] [Google Scholar]
- Ueno T., Oosawa K., Aizawa S. M ring, S ring and proximal rod of the flagellar basal body of Salmonella typhimurium are composed of subunits of a single protein, FliF. J Mol Biol. 1992 Oct 5;227(3):672–677. doi: 10.1016/0022-2836(92)90216-7. [DOI] [PubMed] [Google Scholar]
- Wagenknecht T. A plausible mechanism for flagellar rotation in bacteria. FEBS Lett. 1986 Feb 17;196(2):193–197. doi: 10.1016/0014-5793(86)80244-7. [DOI] [PubMed] [Google Scholar]
- Yoshida S., Sugiyama S., Hojo Y., Tokuda H., Imae Y. Intracellular Na+ kinetically interferes with the rotation of the Na(+)-driven flagellar motors of Vibrio alginolyticus. J Biol Chem. 1990 Nov 25;265(33):20346–20350. [PubMed] [Google Scholar]