Abstract
The structure of cytochrome c bound to anionic lipid membranes composed of dimyristoyl, dipalmitoyl, or dioleoyl phosphatidylglycerols, or of bovine heart cardiolipin, has been investigated by Fourier transform infrared spectroscopy. Only small changes in secondary structure, as registered by the amide I band of cytochrome c, were observed upon binding at temperatures below that of denaturation of the protein, and these were not coupled to the thermotropic phase transitions of the lipid. The denaturation temperature of the protein decreased by approximately 25-30 degrees upon binding, in a progression which correlated with that of the lipid phase transition temperatures, being approximately 7 degrees lower for complexes with dioleoyl than with dipalmitoyl phosphatidylglycerol. Large changes in the amide proton exchange characteristics, as monitored by the spectral shifts in the amide I band of the protein in D2O, were observed on binding cytochrome c to the lipid membranes. For the slowly exchanging population, the amide deuteration rates of the free protein were nearly independent of temperature, whereas those of the bound protein increased by up to two orders of magnitude over the temperature range from 10 to 40 degrees C. In addition, the extent of exchange differed between the bound and unbound protein. A structural transition in the bound protein was detected as a discontinuous step in Arrhenius plots of the deuterium exchange rates which occurred at a temperature in the region of 22 to 29 degrees C, depending on the lipid, far below that of denaturation. The temperature of this transition was determined by the physical state of the lipid, being 7 degrees lower for the lipids in the fluid state than for those in the gel state, and, for complexes with dimyristoyl phosphatidylglycerol, occurred at an intermediate temperature, being controlled by the lipid chain-melting transition at 27-28 degrees C. These results provide evidence for a coupling of the tertiary structure of the membrane-bound protein with the physical state of the membrane lipids.
Full text
PDF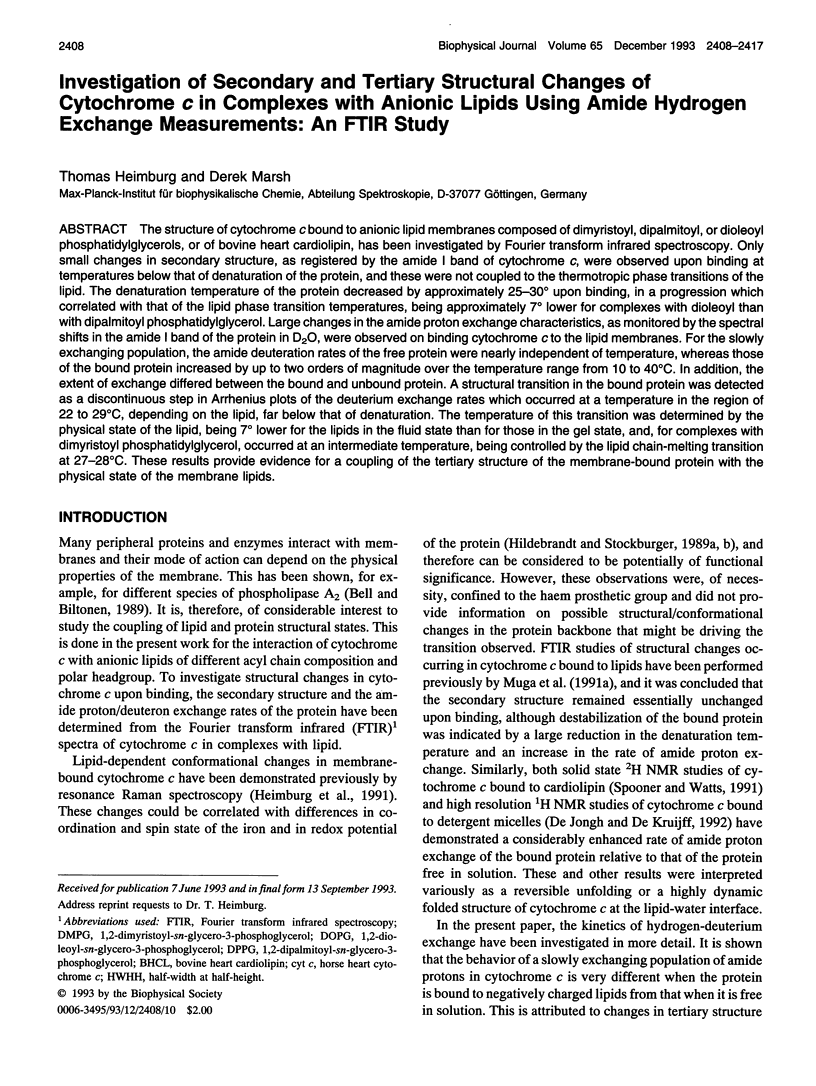
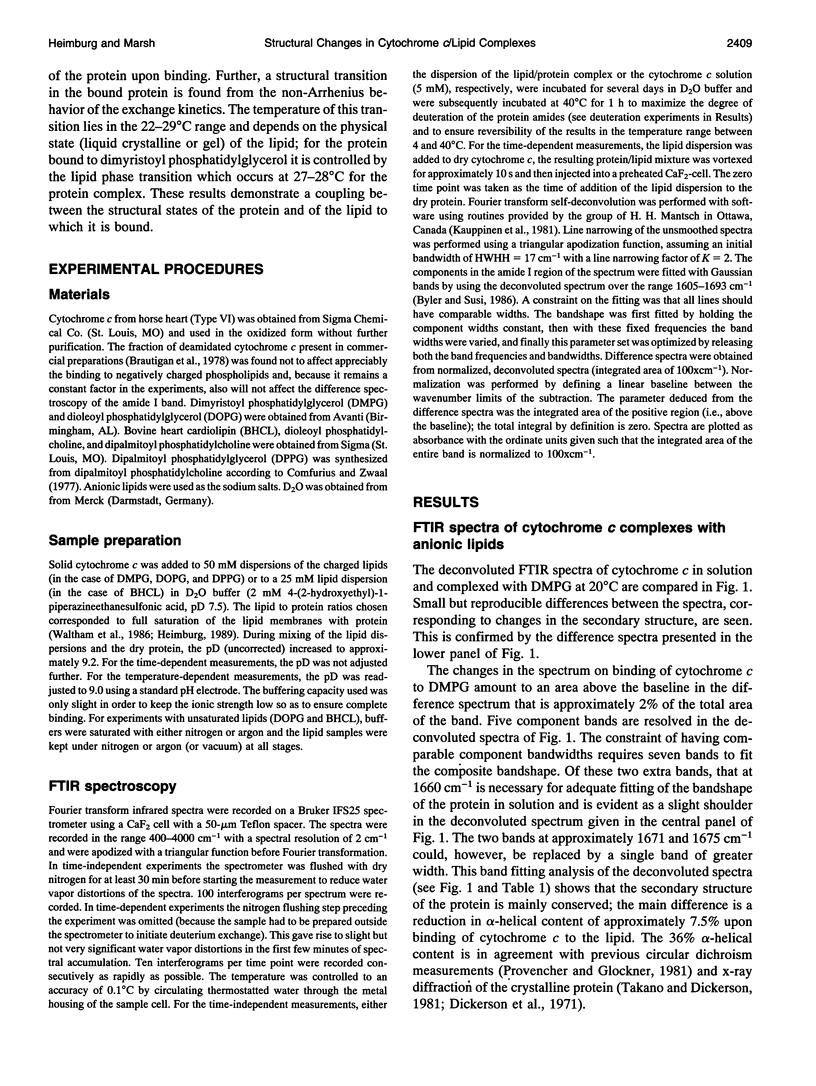
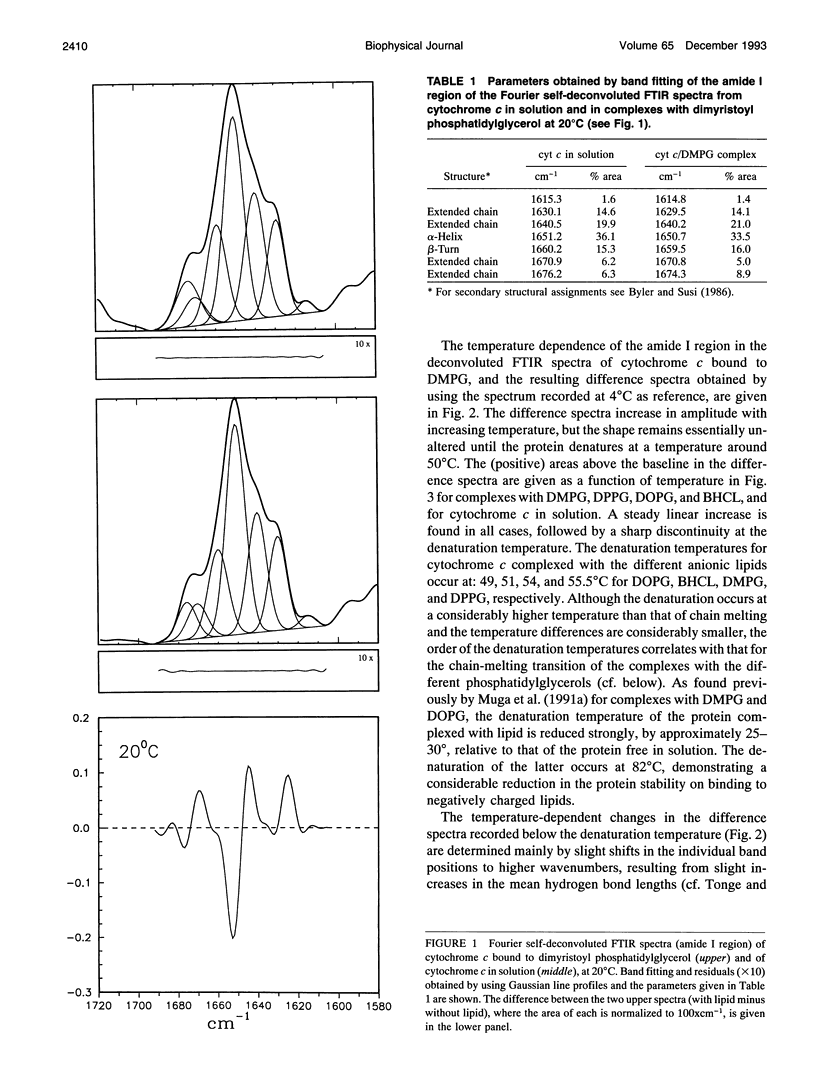
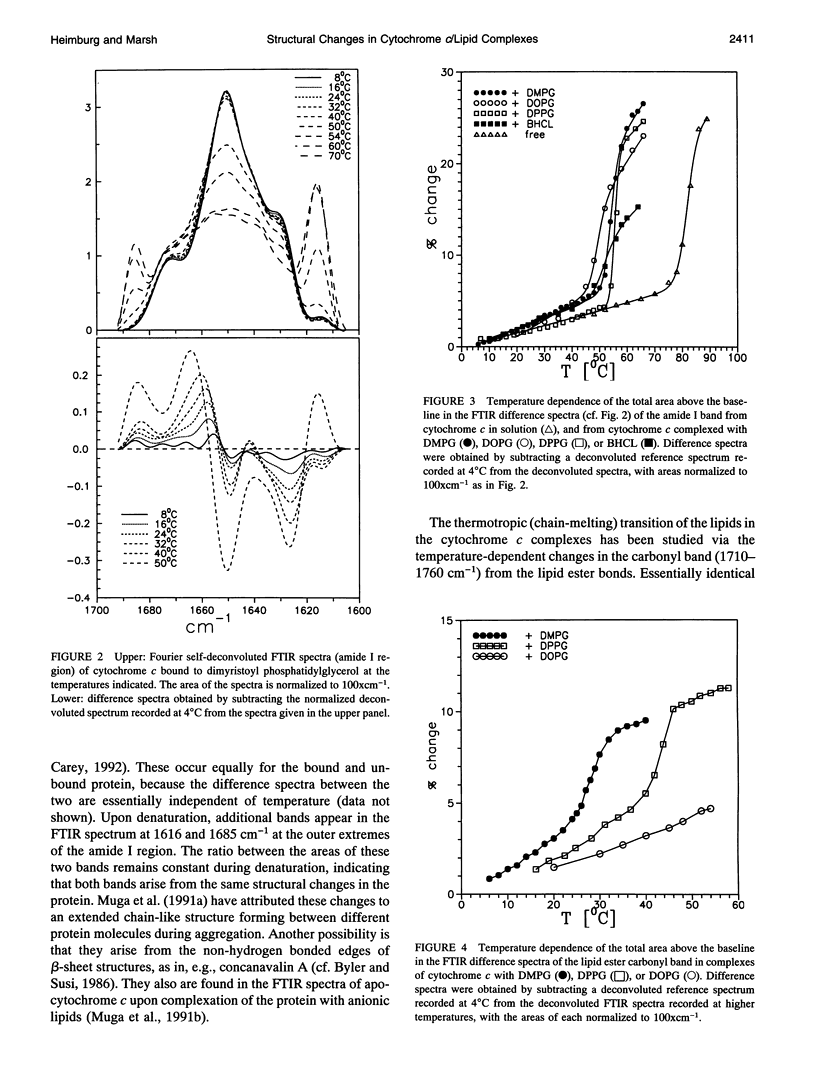
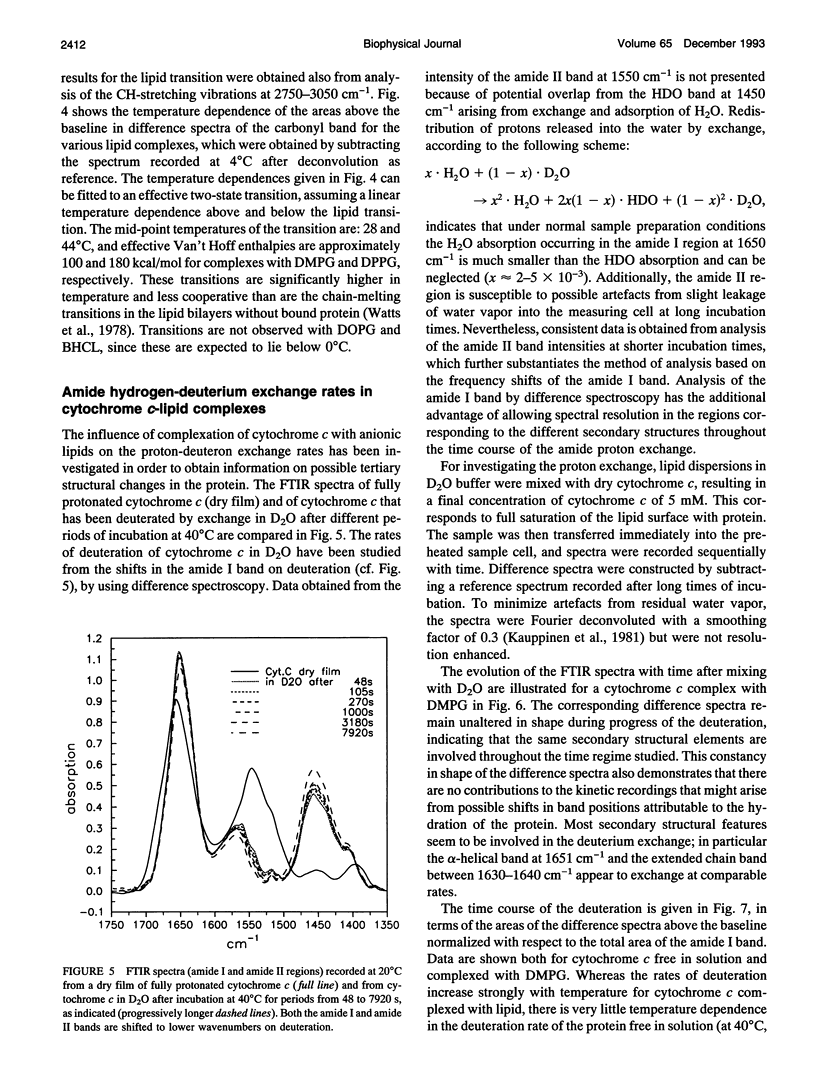
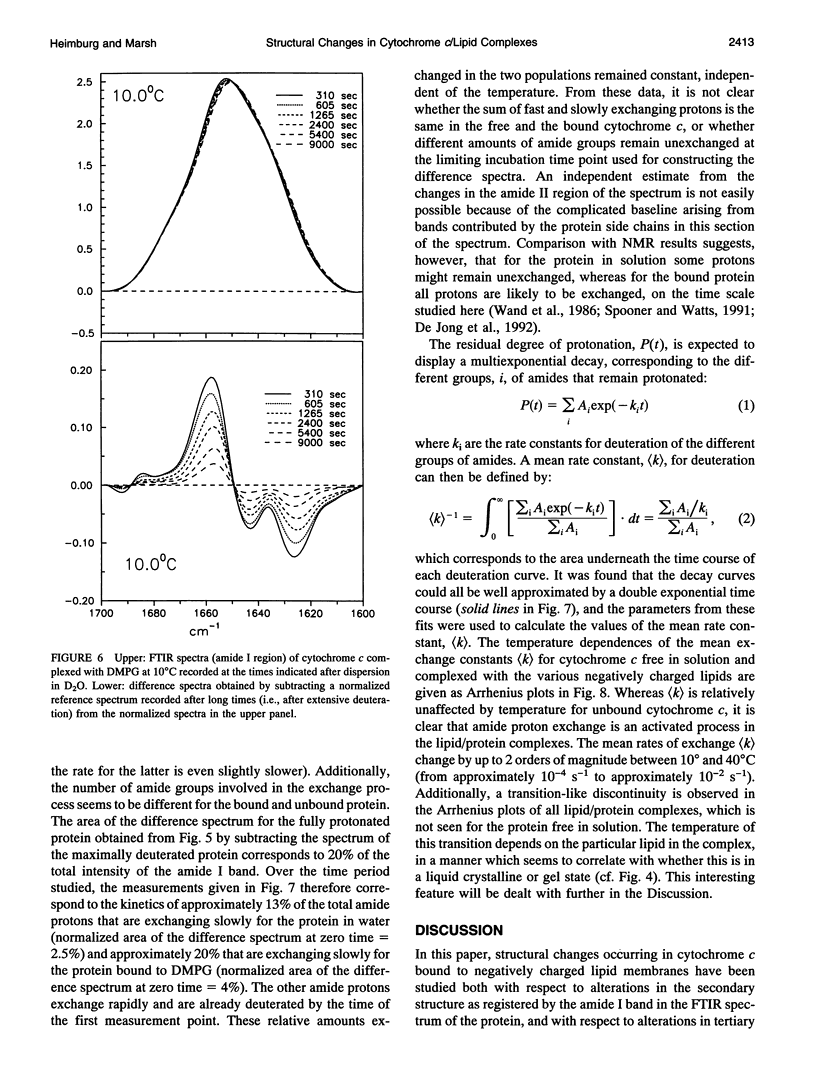
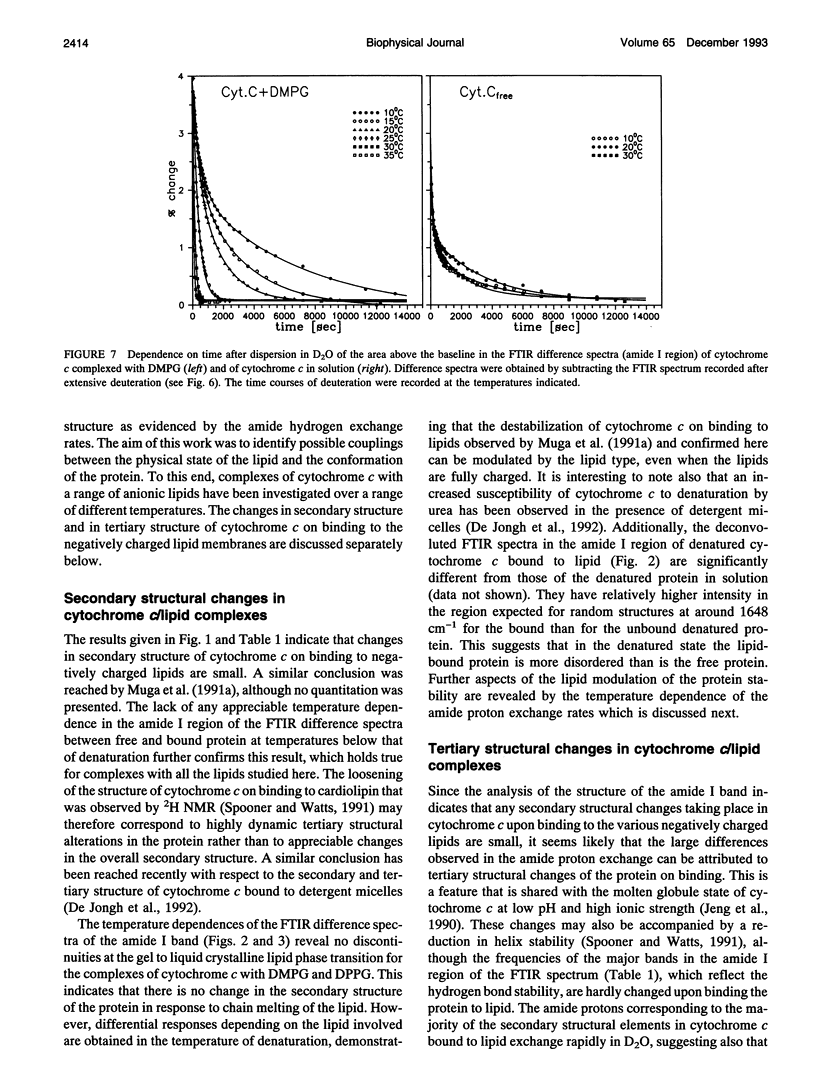
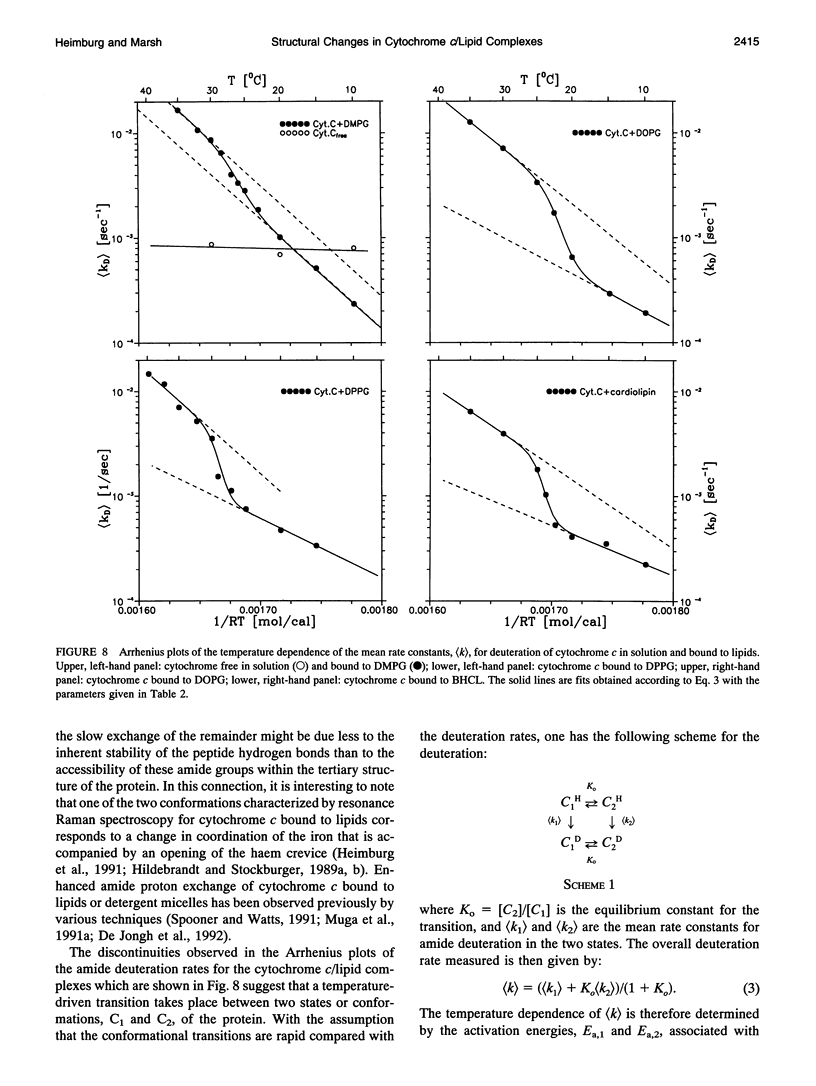
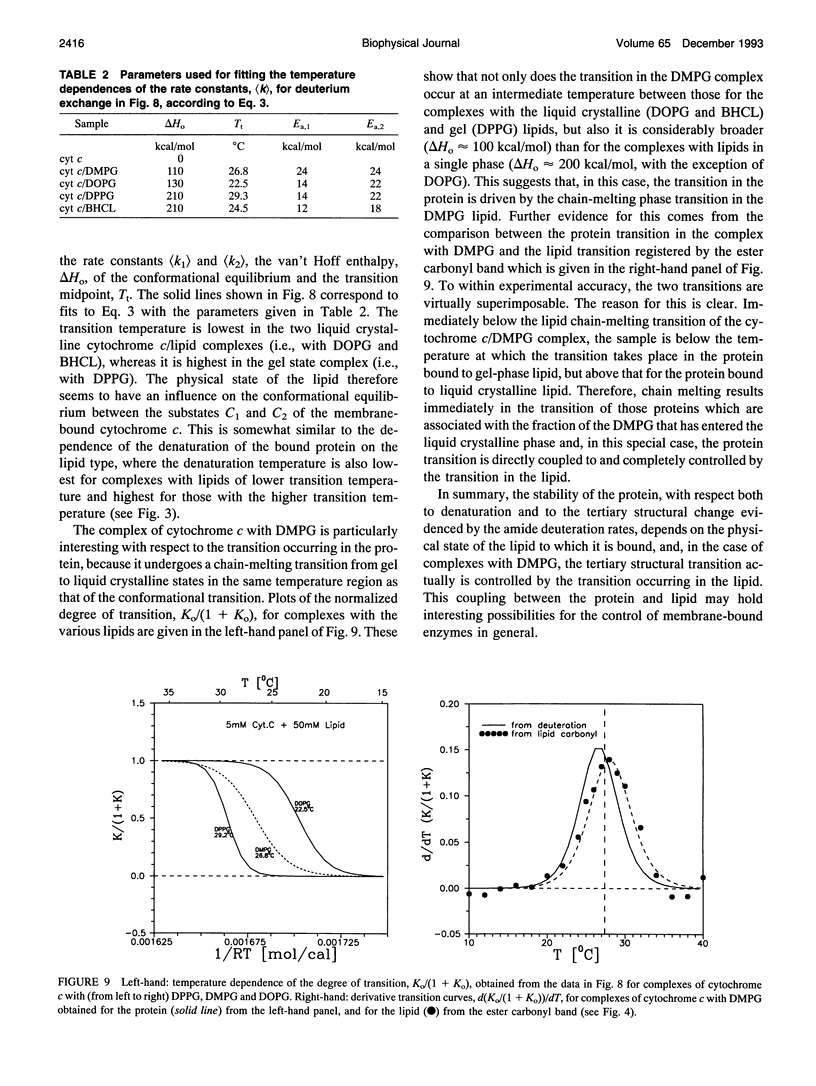
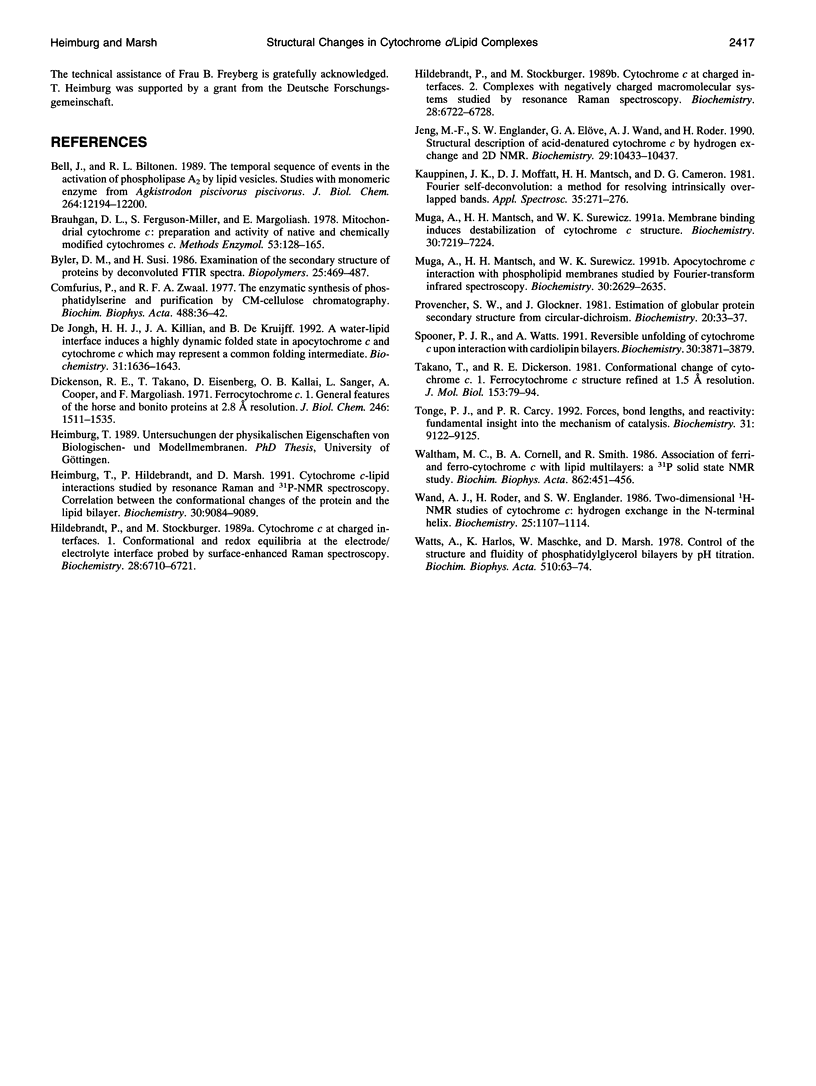
Selected References
These references are in PubMed. This may not be the complete list of references from this article.
- Bell J. D., Biltonen R. L. The temporal sequence of events in the activation of phospholipase A2 by lipid vesicles. Studies with the monomeric enzyme from Agkistrodon piscivorus piscivorus. J Biol Chem. 1989 Jul 25;264(21):12194–12200. [PubMed] [Google Scholar]
- Brautigan D. L., Ferguson-Miller S., Margoliash E. Mitochondrial cytochrome c: preparation and activity of native and chemically modified cytochromes c. Methods Enzymol. 1978;53:128–164. doi: 10.1016/s0076-6879(78)53021-8. [DOI] [PubMed] [Google Scholar]
- Byler D. M., Susi H. Examination of the secondary structure of proteins by deconvolved FTIR spectra. Biopolymers. 1986 Mar;25(3):469–487. doi: 10.1002/bip.360250307. [DOI] [PubMed] [Google Scholar]
- Comfurius P., Zwaal R. F. The enzymatic synthesis of phosphatidylserine and purification by CM-cellulose column chromatography. Biochim Biophys Acta. 1977 Jul 20;488(1):36–42. doi: 10.1016/0005-2760(77)90120-5. [DOI] [PubMed] [Google Scholar]
- Dickerson R. E., Takano T., Eisenberg D., Kallai O. B., Samson L., Cooper A., Margoliash E. Ferricytochrome c. I. General features of the horse and bonito proteins at 2.8 A resolution. J Biol Chem. 1971 Mar 10;246(5):1511–1535. [PubMed] [Google Scholar]
- Heimburg T., Hildebrandt P., Marsh D. Cytochrome c-lipid interactions studied by resonance Raman and 31P NMR spectroscopy. Correlation between the conformational changes of the protein and the lipid bilayer. Biochemistry. 1991 Sep 17;30(37):9084–9089. doi: 10.1021/bi00101a025. [DOI] [PubMed] [Google Scholar]
- Hildebrandt P., Stockburger M. Cytochrome c at charged interfaces. 1. Conformational and redox equilibria at the electrode/electrolyte interface probed by surface-enhanced resonance Raman spectroscopy. Biochemistry. 1989 Aug 8;28(16):6710–6721. doi: 10.1021/bi00442a026. [DOI] [PubMed] [Google Scholar]
- Hildebrandt P., Stockburger M. Cytochrome c at charged interfaces. 2. Complexes with negatively charged macromolecular systems studied by resonance Raman spectroscopy. Biochemistry. 1989 Aug 8;28(16):6722–6728. doi: 10.1021/bi00442a027. [DOI] [PubMed] [Google Scholar]
- Jeng M. F., Englander S. W., Elöve G. A., Wand A. J., Roder H. Structural description of acid-denatured cytochrome c by hydrogen exchange and 2D NMR. Biochemistry. 1990 Nov 20;29(46):10433–10437. doi: 10.1021/bi00498a001. [DOI] [PubMed] [Google Scholar]
- Muga A., Mantsch H. H., Surewicz W. K. Apocytochrome c interaction with phospholipid membranes studied by Fourier-transform infrared spectroscopy. Biochemistry. 1991 Mar 12;30(10):2629–2635. doi: 10.1021/bi00224a010. [DOI] [PubMed] [Google Scholar]
- Muga A., Mantsch H. H., Surewicz W. K. Membrane binding induces destabilization of cytochrome c structure. Biochemistry. 1991 Jul 23;30(29):7219–7224. doi: 10.1021/bi00243a025. [DOI] [PubMed] [Google Scholar]
- Provencher S. W., Glöckner J. Estimation of globular protein secondary structure from circular dichroism. Biochemistry. 1981 Jan 6;20(1):33–37. doi: 10.1021/bi00504a006. [DOI] [PubMed] [Google Scholar]
- Spooner P. J., Watts A. Reversible unfolding of cytochrome c upon interaction with cardiolipin bilayers. 1. Evidence from deuterium NMR measurements. Biochemistry. 1991 Apr 23;30(16):3871–3879. doi: 10.1021/bi00230a010. [DOI] [PubMed] [Google Scholar]
- Takano T., Dickerson R. E. Conformation change of cytochrome c. I. Ferrocytochrome c structure refined at 1.5 A resolution. J Mol Biol. 1981 Nov 25;153(1):79–94. doi: 10.1016/0022-2836(81)90528-3. [DOI] [PubMed] [Google Scholar]
- Tonge P. J., Carey P. R. Forces, bond lengths, and reactivity: fundamental insight into the mechanism of enzyme catalysis. Biochemistry. 1992 Sep 29;31(38):9122–9125. doi: 10.1021/bi00153a002. [DOI] [PubMed] [Google Scholar]
- Waltham M. C., Cornell B. A., Smith R. Association of ferri- and ferro-cytochrome c with lipid multilayers: a 31P solid-state NMR study. Biochim Biophys Acta. 1986 Nov 17;862(2):451–456. doi: 10.1016/0005-2736(86)90250-6. [DOI] [PubMed] [Google Scholar]
- Wand A. J., Roder H., Englander S. W. Two-dimensional 1H NMR studies of cytochrome c: hydrogen exchange in the N-terminal helix. Biochemistry. 1986 Mar 11;25(5):1107–1114. doi: 10.1021/bi00353a025. [DOI] [PubMed] [Google Scholar]
- Watts A., Harlos K., Maschke W., Marsh D. Control of the structure and fluidity of phosphatidylglycerol bilayers by pH titration. Biochim Biophys Acta. 1978 Jun 16;510(1):63–74. doi: 10.1016/0005-2736(78)90130-x. [DOI] [PubMed] [Google Scholar]
- de Jongh H. H., Killian J. A., de Kruijff B. A water-lipid interface induces a highly dynamic folded state in apocytochrome c and cytochrome c, which may represent a common folding intermediate. Biochemistry. 1992 Feb 18;31(6):1636–1643. doi: 10.1021/bi00121a008. [DOI] [PubMed] [Google Scholar]