Abstract
A novel microperfusion chamber was developed to measure kinetic cell volume changes under various extracellular conditions and to quantitatively determine cell membrane transport properties. This device eliminates modeling ambiguities and limitations inherent in the use of the microdiffusion chamber and the micropipette perfusion technique, both of which have been previously validated and are closely related optical technologies using light microscopy and image analysis. The resultant simplicity should prove to be especially valuable for study of the coupled transport of water and permeating solutes through cell membranes. Using the microperfusion chamber, water and dimethylsulfoxide (DMSO) permeability coefficients of mouse oocytes as well as the water permeability coefficient of golden hamster pancreatic islet cells were determined. In these experiments, the individual cells were held in the chamber and perfused at 22 degrees C with hyperosmotic media, with or without DMSO (1.5 M). The cell volume change was videotaped and quantified by image analysis. Based on the experimental data and irreversible thermodynamics theory for the coupled mass transfer across the cell membrane, the water permeability coefficient of the oocytes was determined to be 0.47 micron. min-1. atm-1 in the absence of DMSO and 0.65 microns. min-1. atm-1 in the presence of DMSO. The DMSO permeability coefficient of the oocyte membrane and associated membrane reflection coefficient to DMSO were determined to be 0.23 and 0.85 micron/s, respectively. These values are consistent with those determined using the micropipette perfusion and microdiffusion chamber techniques. The water permeability coefficient of the golden hamster pancreatic islet cells was determined to be 0.27 microns. min-1. atm-1, which agrees well with a value previously determined using an electronic sizing (Coulter counter) technique. The use of the microperfusion chamber has the following major advantages: 1) This method allows the extracellular condition(s) to be readily changed by perfusing a single cell or group of cells with a prepared medium (cells can be reperfused with a different medium to study the response of the same cell to different osmotic conditions). 2) The short mixing time of cells and perfusion medium allows for accurate control of the extracellular osmolality and ensures accuracy of the corresponding mathematical formulation (modeling). 3) This technique has wide applicability in studying the cell osmotic response and in determining cell membrane transport properties.
Full text
PDF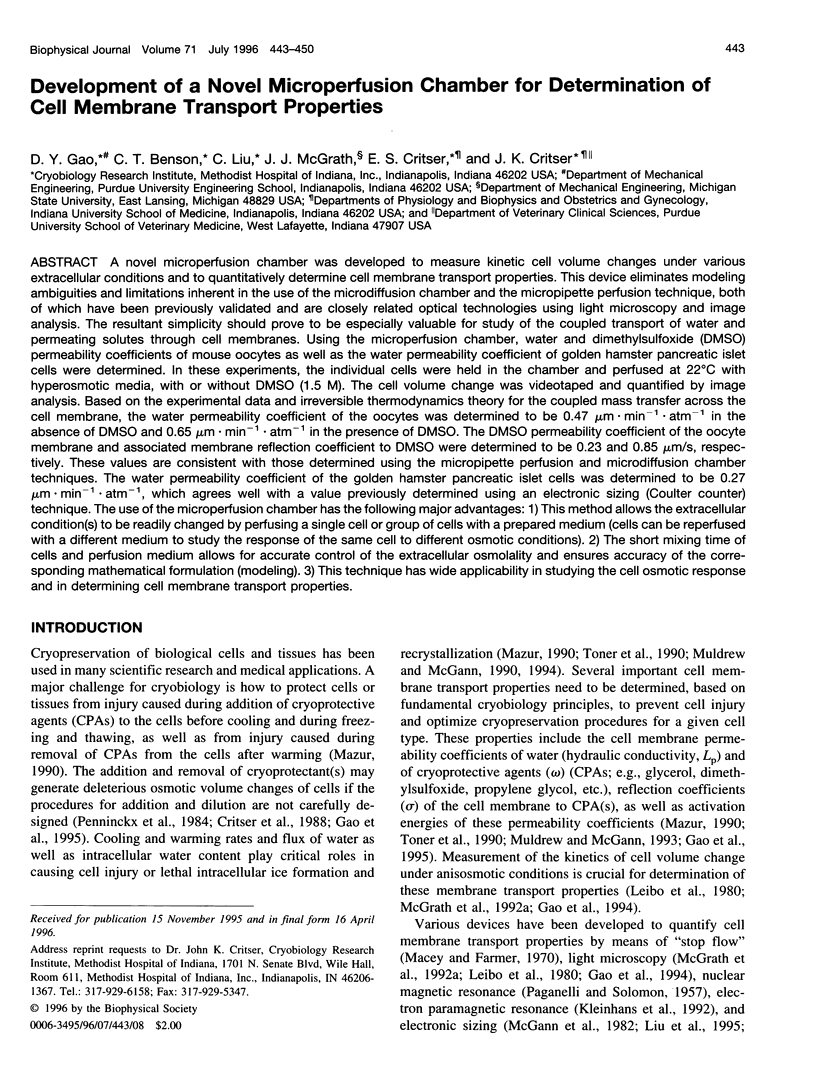
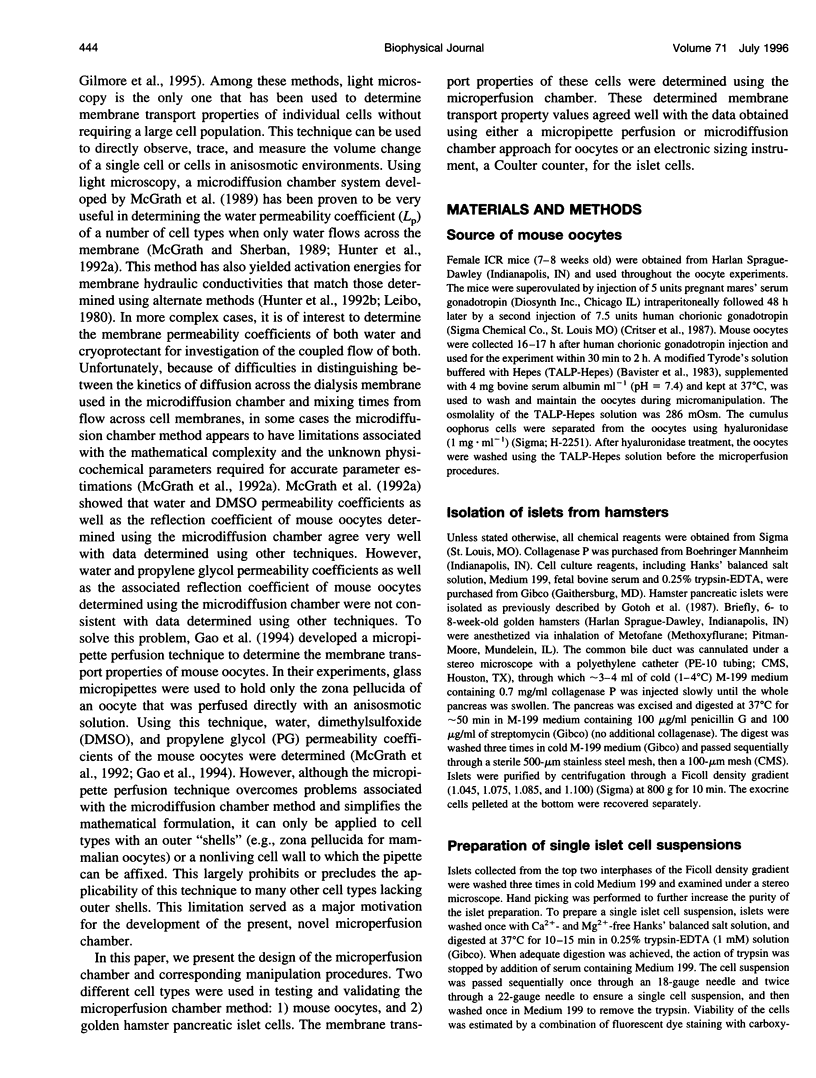
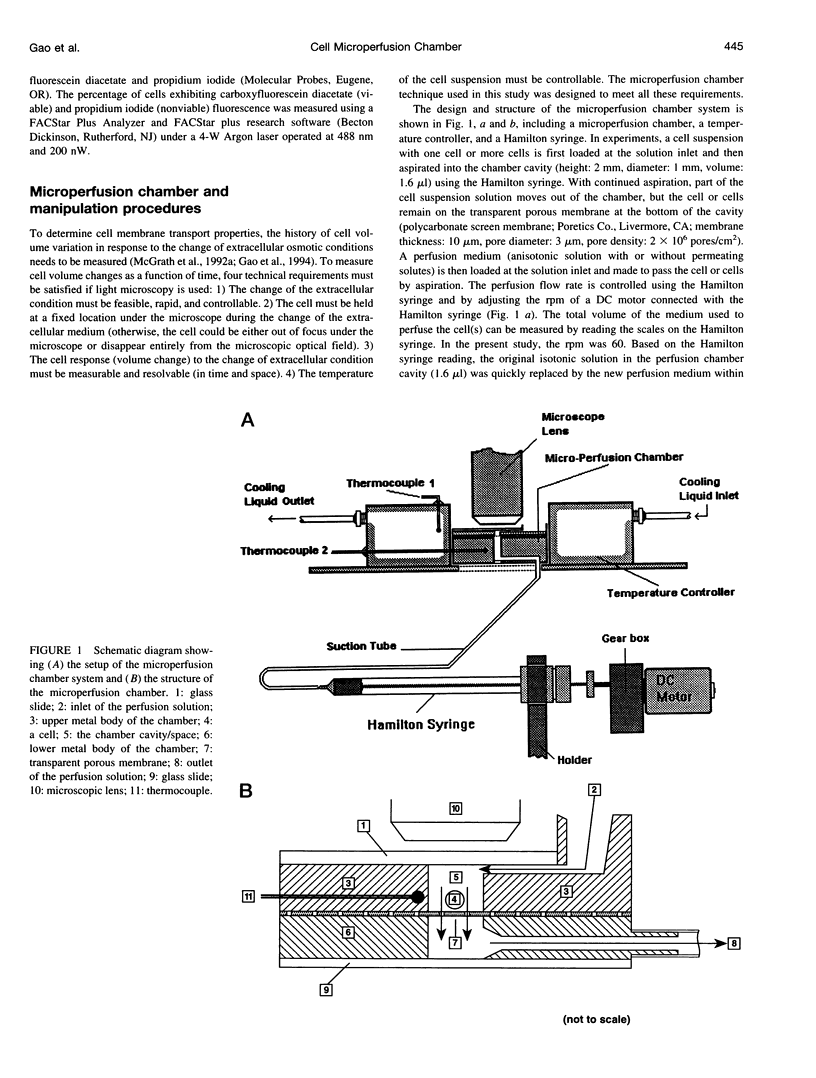
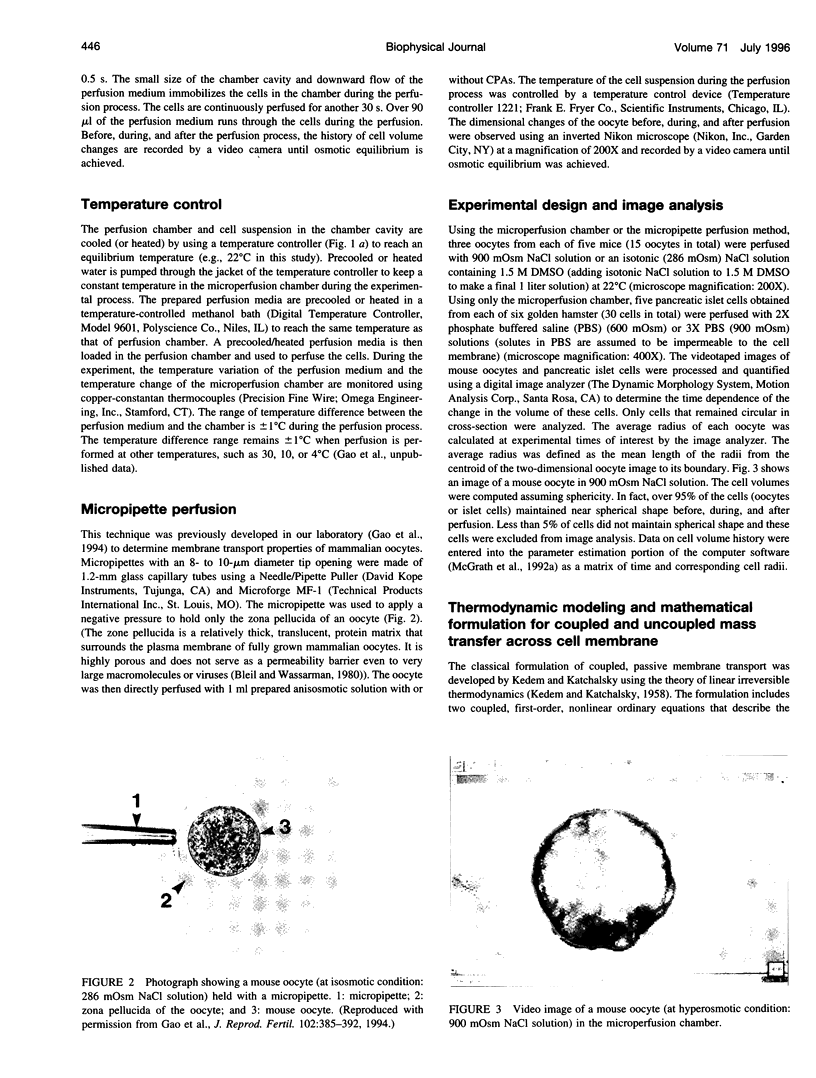
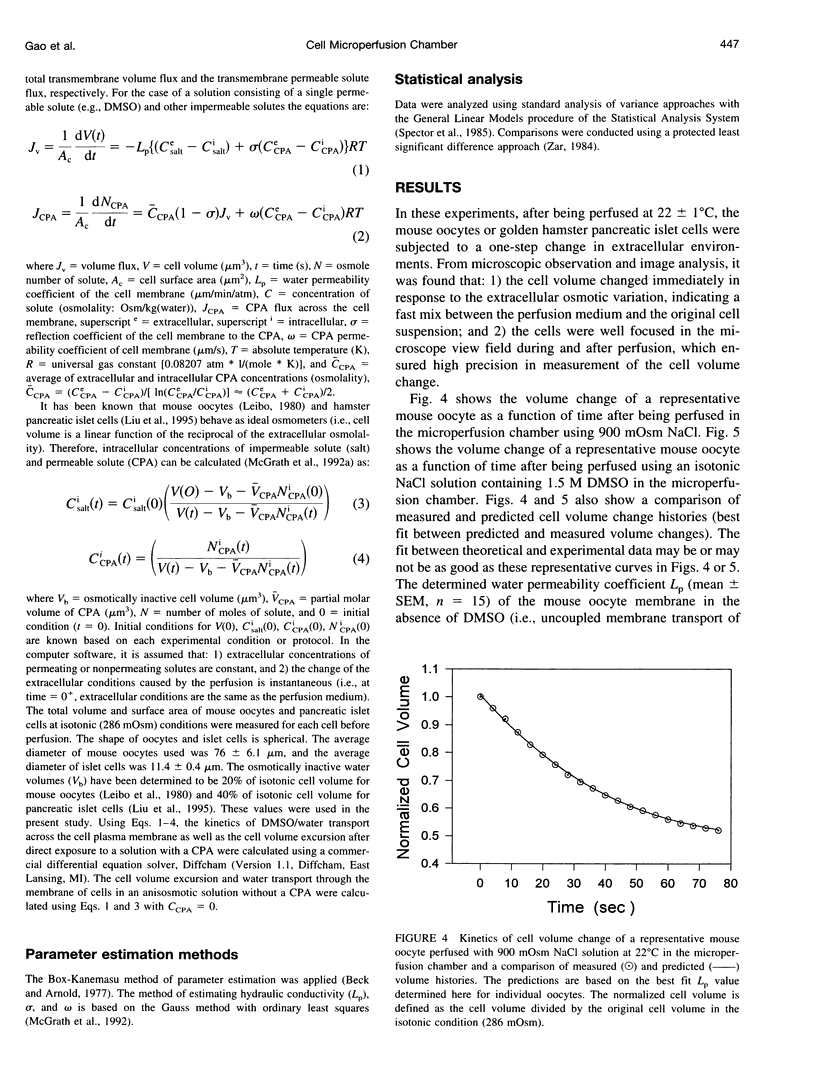
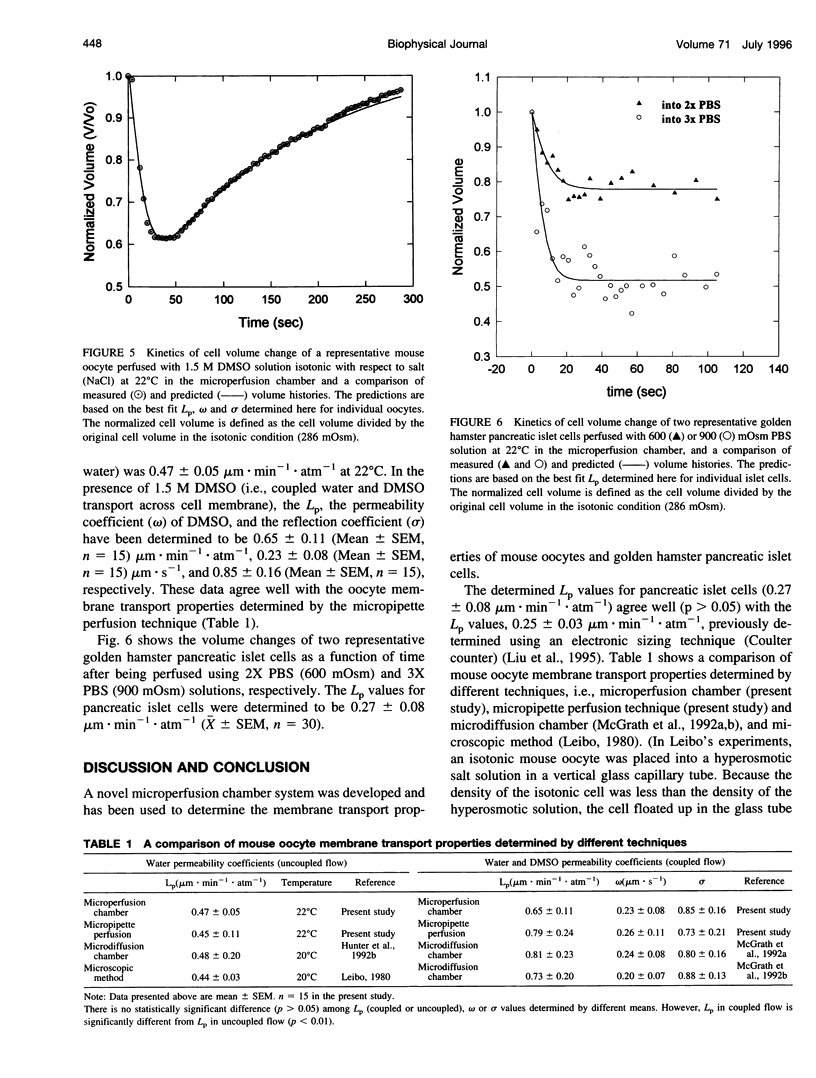
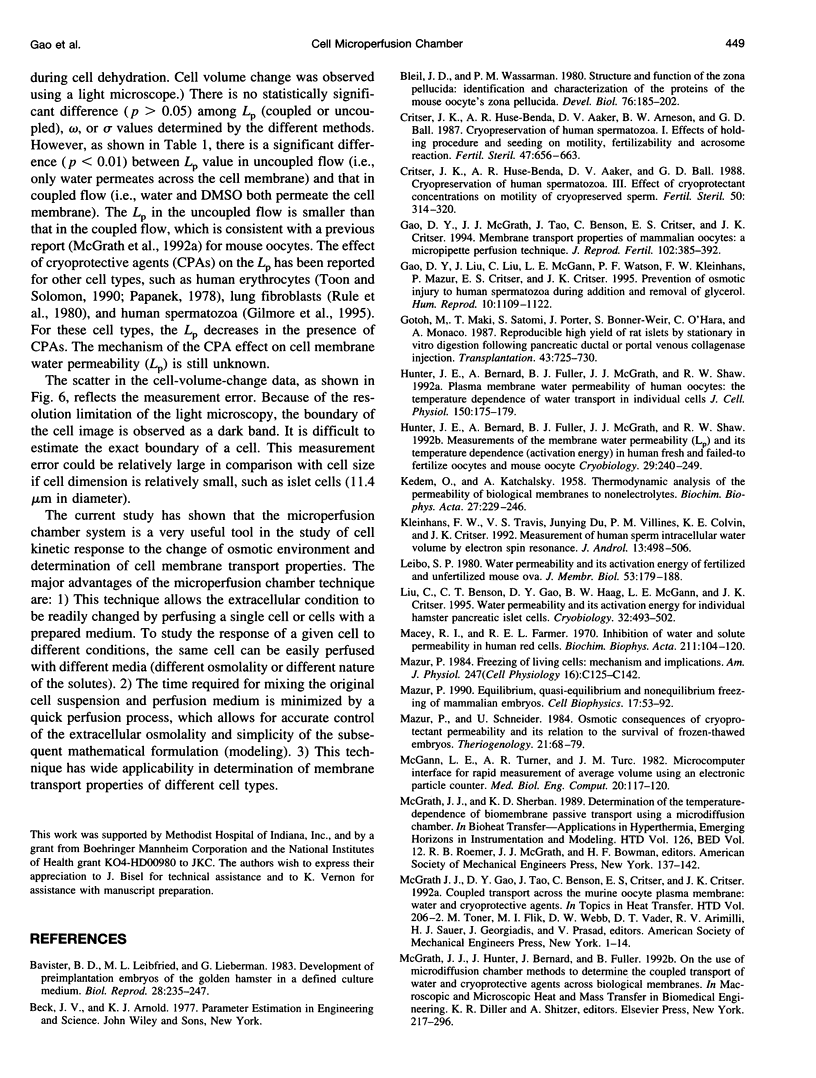
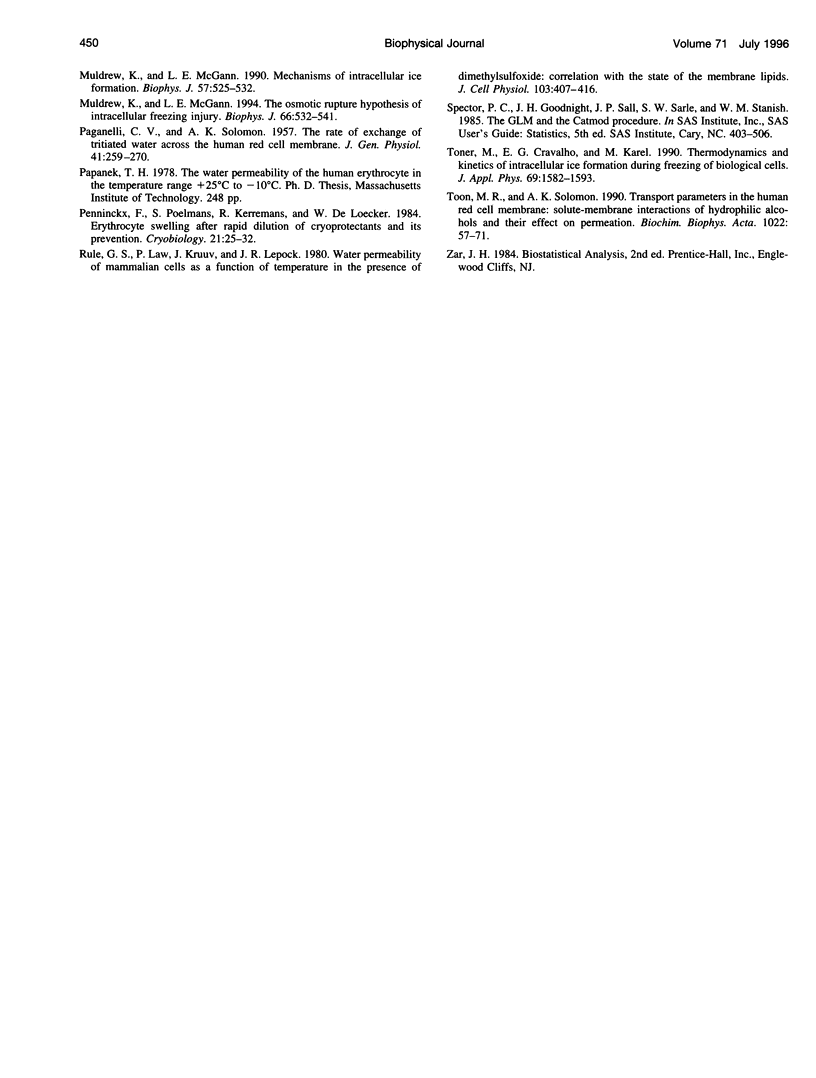
Images in this article
Selected References
These references are in PubMed. This may not be the complete list of references from this article.
- Bavister B. D., Leibfried M. L., Lieberman G. Development of preimplantation embryos of the golden hamster in a defined culture medium. Biol Reprod. 1983 Feb;28(1):235–247. doi: 10.1095/biolreprod28.1.235. [DOI] [PubMed] [Google Scholar]
- Bleil J. D., Wassarman P. M. Structure and function of the zona pellucida: identification and characterization of the proteins of the mouse oocyte's zona pellucida. Dev Biol. 1980 Apr;76(1):185–202. doi: 10.1016/0012-1606(80)90371-1. [DOI] [PubMed] [Google Scholar]
- Critser J. K., Huse-Benda A. R., Aaker D. V., Arneson B. W., Ball G. D. Cryopreservation of human spermatozoa. I. Effects of holding procedure and seeding on motility, fertilizability, and acrosome reaction. Fertil Steril. 1987 Apr;47(4):656–663. doi: 10.1016/s0015-0282(16)59118-3. [DOI] [PubMed] [Google Scholar]
- Critser J. K., Huse-Benda A. R., Aaker D. V., Arneson B. W., Ball G. D. Cryopreservation of human spermatozoa. III. The effect of cryoprotectants on motility. Fertil Steril. 1988 Aug;50(2):314–320. [PubMed] [Google Scholar]
- Gao D. Y., Liu J., Liu C., McGann L. E., Watson P. F., Kleinhans F. W., Mazur P., Critser E. S., Critser J. K. Prevention of osmotic injury to human spermatozoa during addition and removal of glycerol. Hum Reprod. 1995 May;10(5):1109–1122. doi: 10.1093/oxfordjournals.humrep.a136103. [DOI] [PubMed] [Google Scholar]
- Gao D. Y., McGrath J. J., Tao J., Benson C. T., Critser E. S., Critser J. K. Membrane transport properties of mammalian oocytes: a micropipette perfusion technique. J Reprod Fertil. 1994 Nov;102(2):385–392. doi: 10.1530/jrf.0.1020385. [DOI] [PubMed] [Google Scholar]
- Gotoh M., Maki T., Satomi S., Porter J., Bonner-Weir S., O'Hara C. J., Monaco A. P. Reproducible high yield of rat islets by stationary in vitro digestion following pancreatic ductal or portal venous collagenase injection. Transplantation. 1987 May;43(5):725–730. doi: 10.1097/00007890-198705000-00024. [DOI] [PubMed] [Google Scholar]
- Hunter J. E., Bernard A., Fuller B. J., McGrath J. J., Shaw R. W. Measurements of the membrane water permeability (Lp) and its temperature dependence (activation energy) in human fresh and failed-to-fertilize oocytes and mouse oocyte. Cryobiology. 1992 Apr;29(2):240–249. doi: 10.1016/0011-2240(92)90022-t. [DOI] [PubMed] [Google Scholar]
- Hunter J., Bernard A., Fuller B., McGrath J., Shaw R. W. Plasma membrane water permeabilities of human oocytes: the temperature dependence of water movement in individual cells. J Cell Physiol. 1992 Jan;150(1):175–179. doi: 10.1002/jcp.1041500123. [DOI] [PubMed] [Google Scholar]
- KEDEM O., KATCHALSKY A. Thermodynamic analysis of the permeability of biological membranes to non-electrolytes. Biochim Biophys Acta. 1958 Feb;27(2):229–246. doi: 10.1016/0006-3002(58)90330-5. [DOI] [PubMed] [Google Scholar]
- Kleinhans F. W., Travis V. S., Du J., Villines P. M., Colvin K. E., Critser J. K. Measurement of human sperm intracellular water volume by electron spin resonance. J Androl. 1992 Nov-Dec;13(6):498–506. [PubMed] [Google Scholar]
- Leibo S. P. Water permeability and its activation energy of fertilized and unfertilized mouse ova. J Membr Biol. 1980;53(3):179–188. doi: 10.1007/BF01868823. [DOI] [PubMed] [Google Scholar]
- Liu C., Benson C. T., Gao D., Haag B. W., McGann L. E., Critser J. K. Water permeability and its activation energy for individual hamster pancreatic islet cells. Cryobiology. 1995 Oct;32(5):493–502. doi: 10.1006/cryo.1995.1049. [DOI] [PubMed] [Google Scholar]
- Macey R. I., Farmer R. E. Inhibition of water and solute permeability in human red cells. Biochim Biophys Acta. 1970 Jul 7;211(1):104–106. doi: 10.1016/0005-2736(70)90130-6. [DOI] [PubMed] [Google Scholar]
- Mazur P. Equilibrium, quasi-equilibrium, and nonequilibrium freezing of mammalian embryos. Cell Biophys. 1990 Aug;17(1):53–92. doi: 10.1007/BF02989804. [DOI] [PubMed] [Google Scholar]
- Mazur P. Freezing of living cells: mechanisms and implications. Am J Physiol. 1984 Sep;247(3 Pt 1):C125–C142. doi: 10.1152/ajpcell.1984.247.3.C125. [DOI] [PubMed] [Google Scholar]
- McGann L. E., Turner A. R., Turc J. M. Microcomputer interface for rapid measurements of average volume using an electronic particle counter. Med Biol Eng Comput. 1982 Jan;20(1):117–120. doi: 10.1007/BF02441862. [DOI] [PubMed] [Google Scholar]
- Muldrew K., McGann L. E. Mechanisms of intracellular ice formation. Biophys J. 1990 Mar;57(3):525–532. doi: 10.1016/S0006-3495(90)82568-6. [DOI] [PMC free article] [PubMed] [Google Scholar]
- Muldrew K., McGann L. E. The osmotic rupture hypothesis of intracellular freezing injury. Biophys J. 1994 Feb;66(2 Pt 1):532–541. doi: 10.1016/s0006-3495(94)80806-9. [DOI] [PMC free article] [PubMed] [Google Scholar]
- PAGANELLI C. V., SOLOMON A. K. The rate of exchange of tritiated water across the human red cell membrane. J Gen Physiol. 1957 Nov 20;41(2):259–277. doi: 10.1085/jgp.41.2.259. [DOI] [PMC free article] [PubMed] [Google Scholar]
- Penninckx F., Poelmans S., Kerremans R., De Loecker W. Erythrocyte swelling after rapid dilution of cryoprotectants and its prevention. Cryobiology. 1984 Feb;21(1):25–32. doi: 10.1016/0011-2240(84)90019-1. [DOI] [PubMed] [Google Scholar]
- Rule G. S., Law P., Kruuv J., Lepock J. R. Water permeability of mammalian cells as a function of temperature in the presence of dimethylsulfoxide: correlation with the state of the membrane lipids. J Cell Physiol. 1980 Jun;103(3):407–416. doi: 10.1002/jcp.1041030306. [DOI] [PubMed] [Google Scholar]
- Toon M. R., Solomon A. K. Transport parameters in the human red cell membrane: solute-membrane interactions of hydrophilic alcohols and their effect on permeation. Biochim Biophys Acta. 1990 Feb 16;1022(1):57–71. doi: 10.1016/0005-2736(90)90400-i. [DOI] [PubMed] [Google Scholar]