Abstract
We previously described the use of a counter-rotating cone and plate rheoscope to measure the time and force dependence of break-up of doublets of sphered, swollen, and fixed red cells (SSRC) cross-linked by monoclonal IgM antibody. It has been shown that doublet break-up can occur by extraction of receptors from the membrane, rather than by antibody-antigen bond break-up, and is a stochastic process. We therefore prepared 4.62-microns carboxyl modified latex spheres with a covalently coupled synthetic blood group B antigen trisaccharide. Using a two-step carbodiimide process, ethylene diamine was covalently linked to the carboxyl modified latex spheres, and the trisaccharide, having an eight carbon spacer modified to bear a terminal carboxyl group, was linked to the ethylene diamine. Using these antigen spheres we carried out studies in Couette flow, in a transparent cone and plate rheoscope, of the shear-induced break-up of doublets cross-linked by monoclonal IgM anti-B antibody in 19% and 15% Dextran 40. As previously found with SSRC, over a range of normal force from 55 to 175 pN, there was a distribution in times to break-up. However, the fraction of antigen sphere doublets broken up, which increased from 0.08 to 0.43 at 75 pM IgM, and from 0.06 to 0.20 at 150 pM IgM, was significantly lower than that for the SSRC, where the fraction broken up at 150 pM IgM increased from 0.10 to 0.47. Thus, significantly higher forces were required to achieve the same degree of break-up for doublets of antigen-linked spheres than for SSRC. Computer simulation using a stochastic model of break-up showed that the differences between antigen sphere and SSRC doublet break-up were due to a change in bond character (the range and depth of the bond energy minimum) rather than to an increase in the number of bonds linking antigen-sphere doublets. This supports the notion that antibody-antigen bonds are ruptured in the case of antigen spheres, whereas antigen is able to be extracted from the membrane of SSRC, although changes of receptor substrate from cell to latex and the possibility of latex strand extraction from the microspheres are potential complicating factors.
Full text
PDF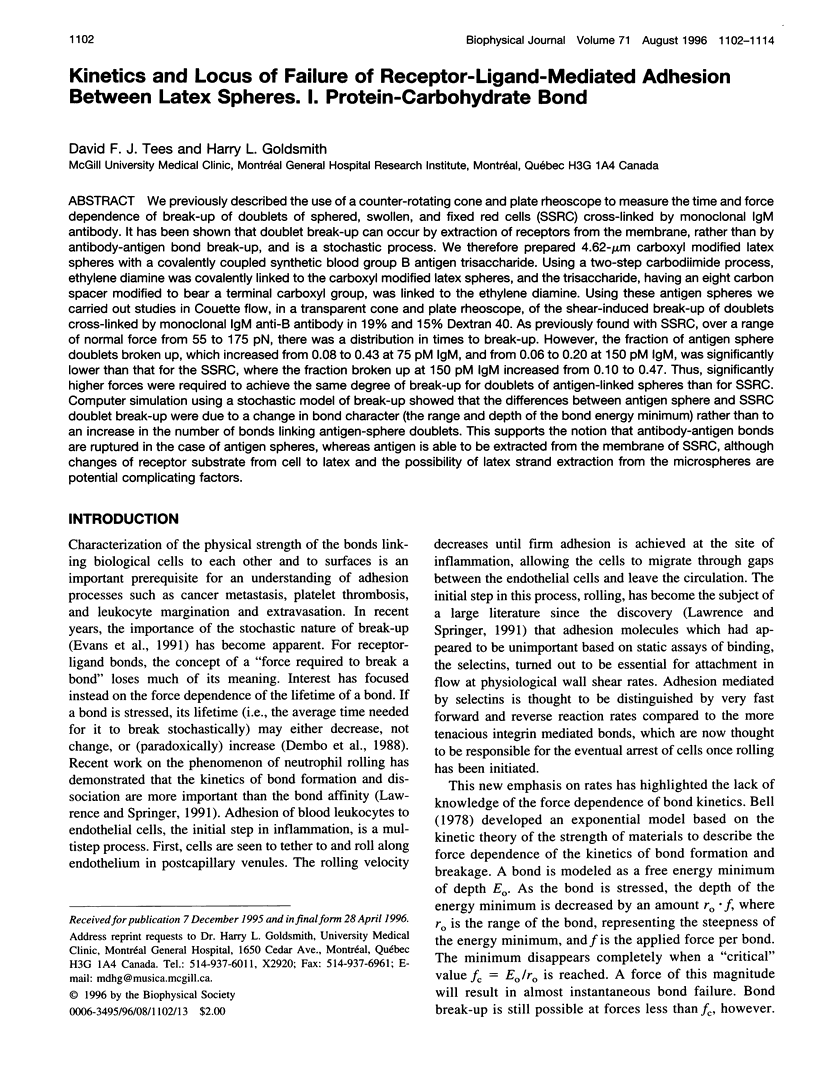
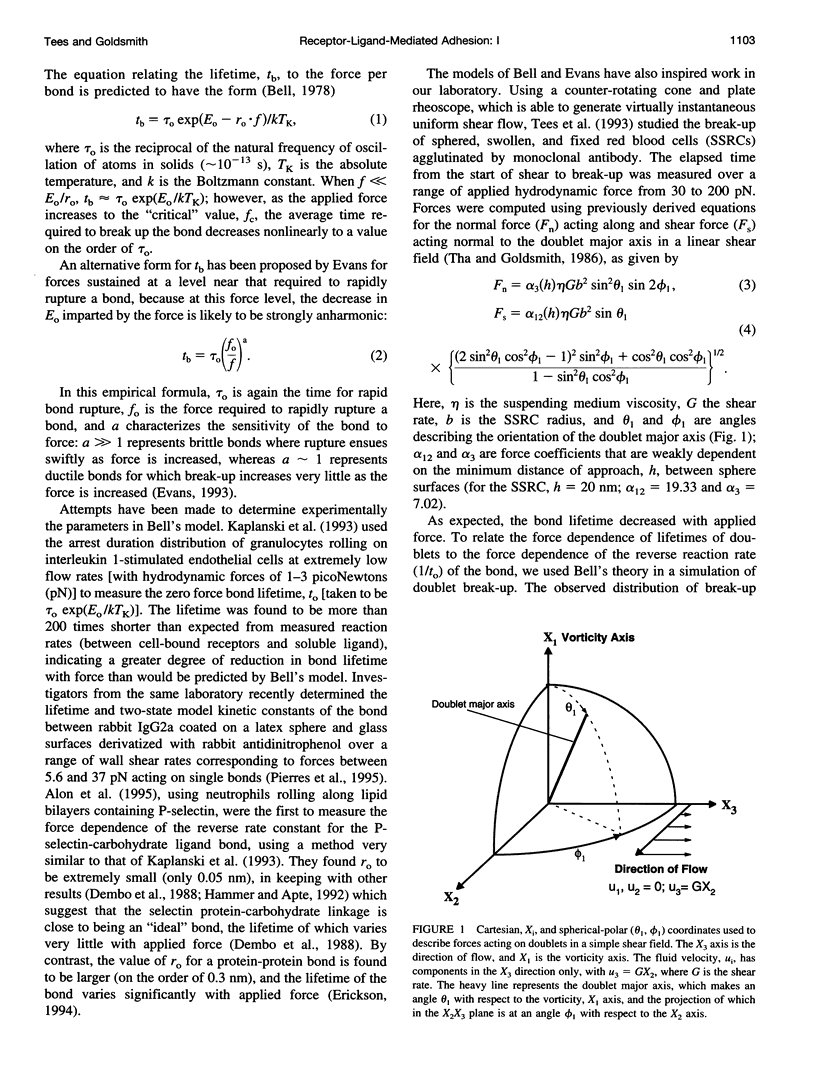
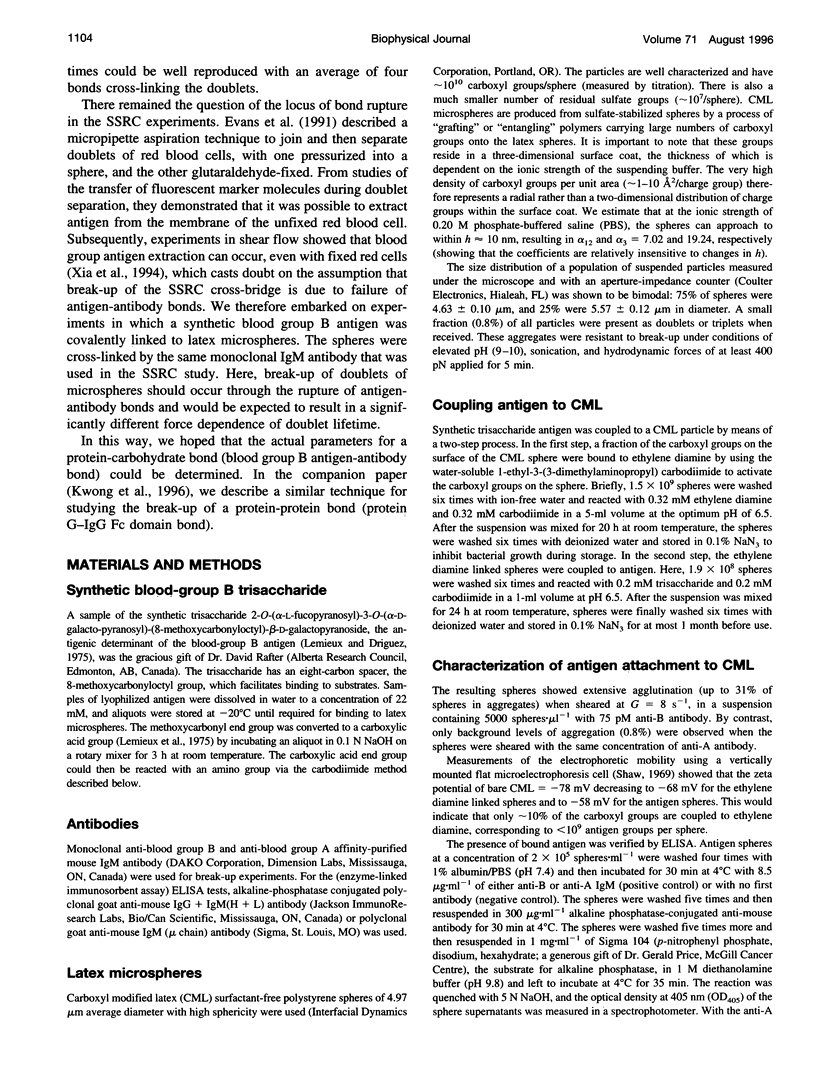
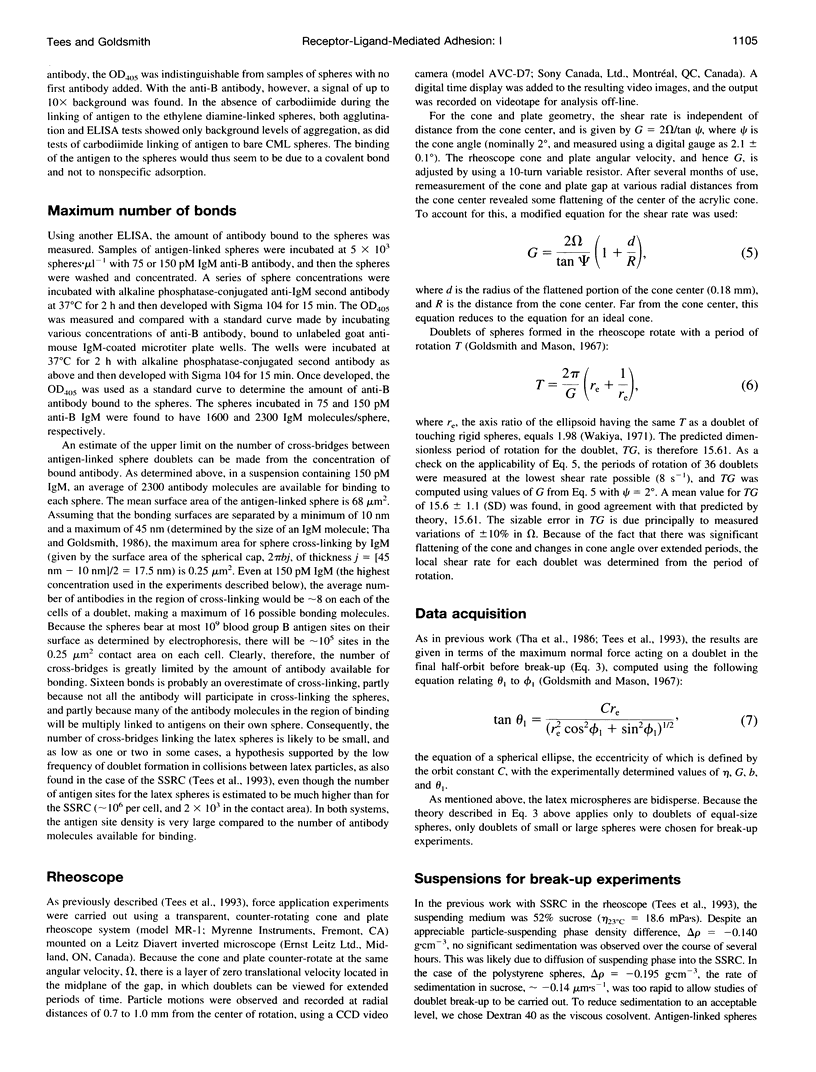
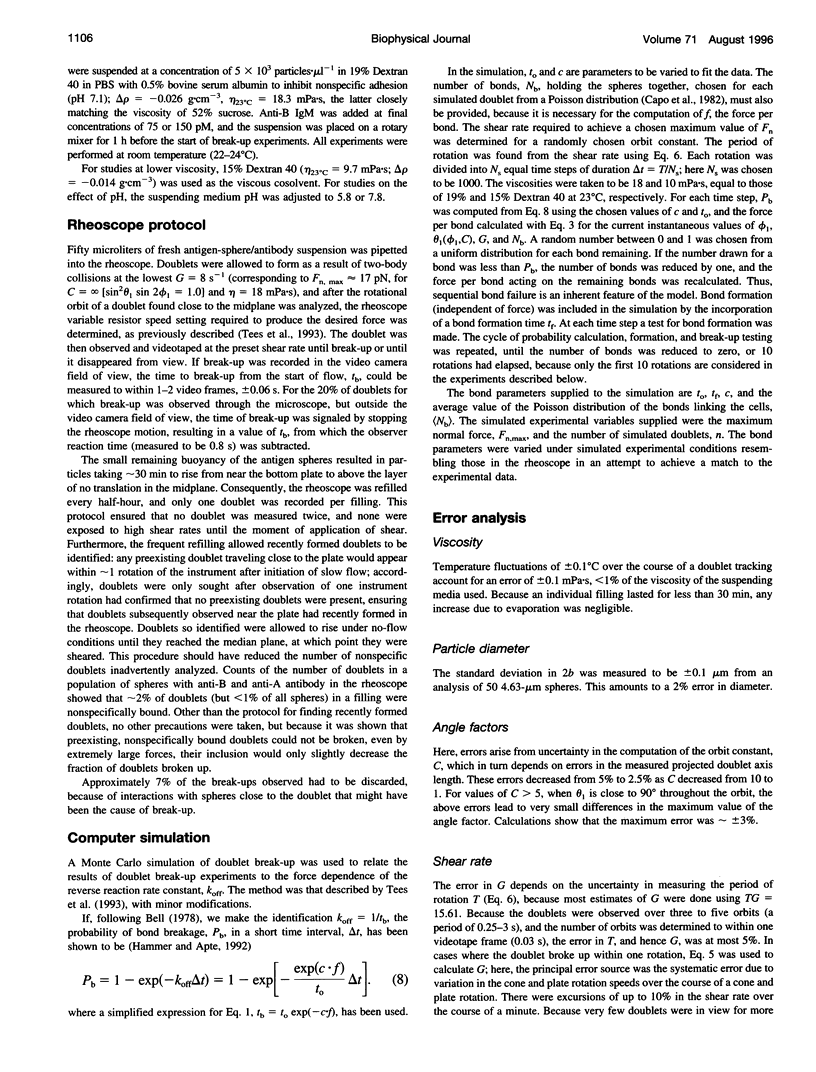
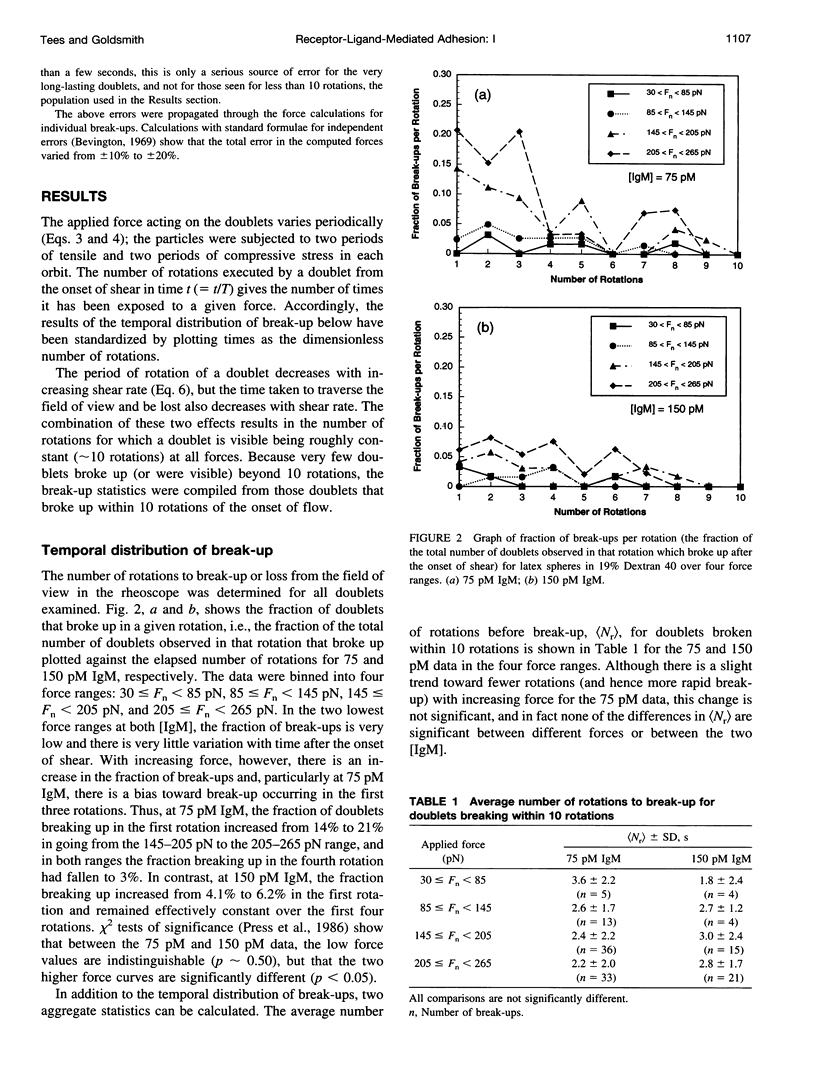
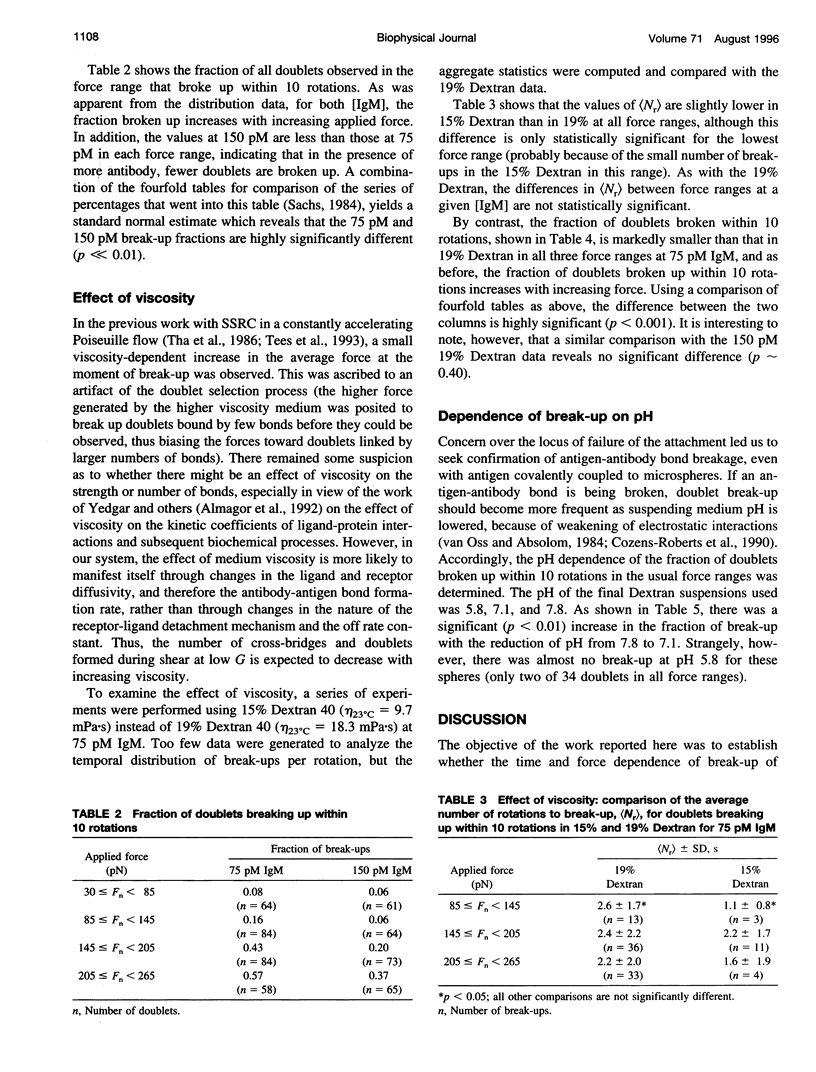
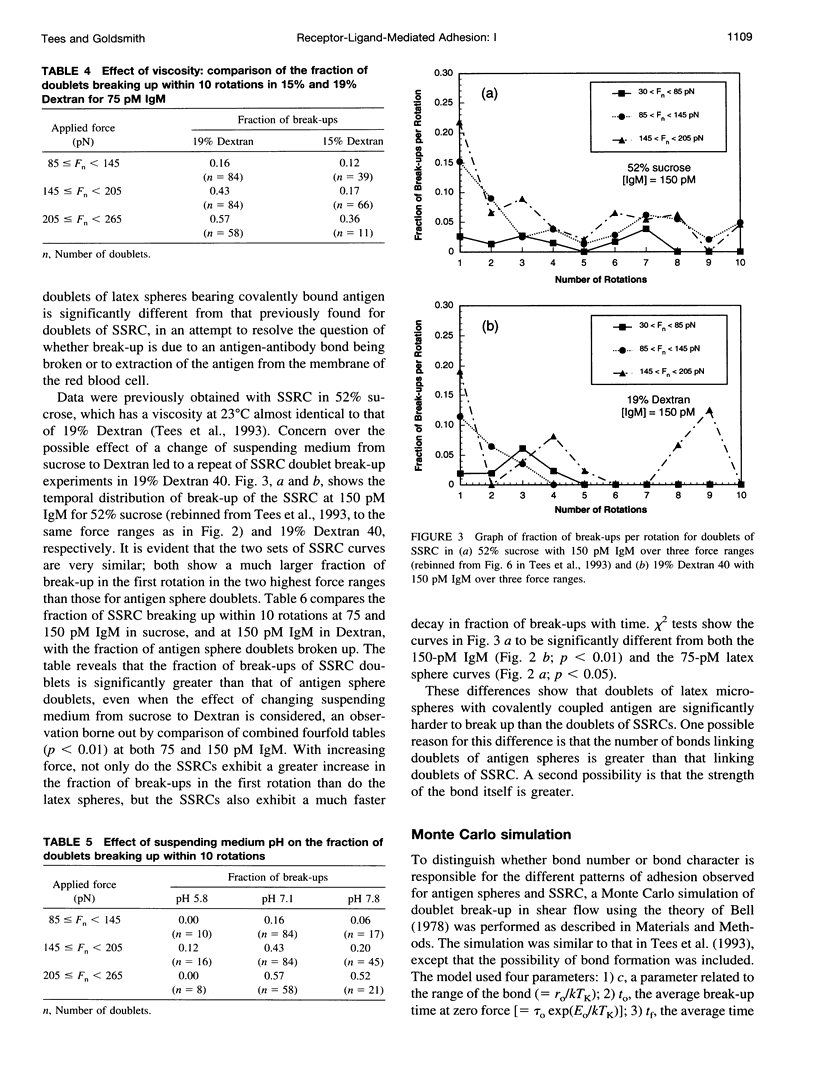
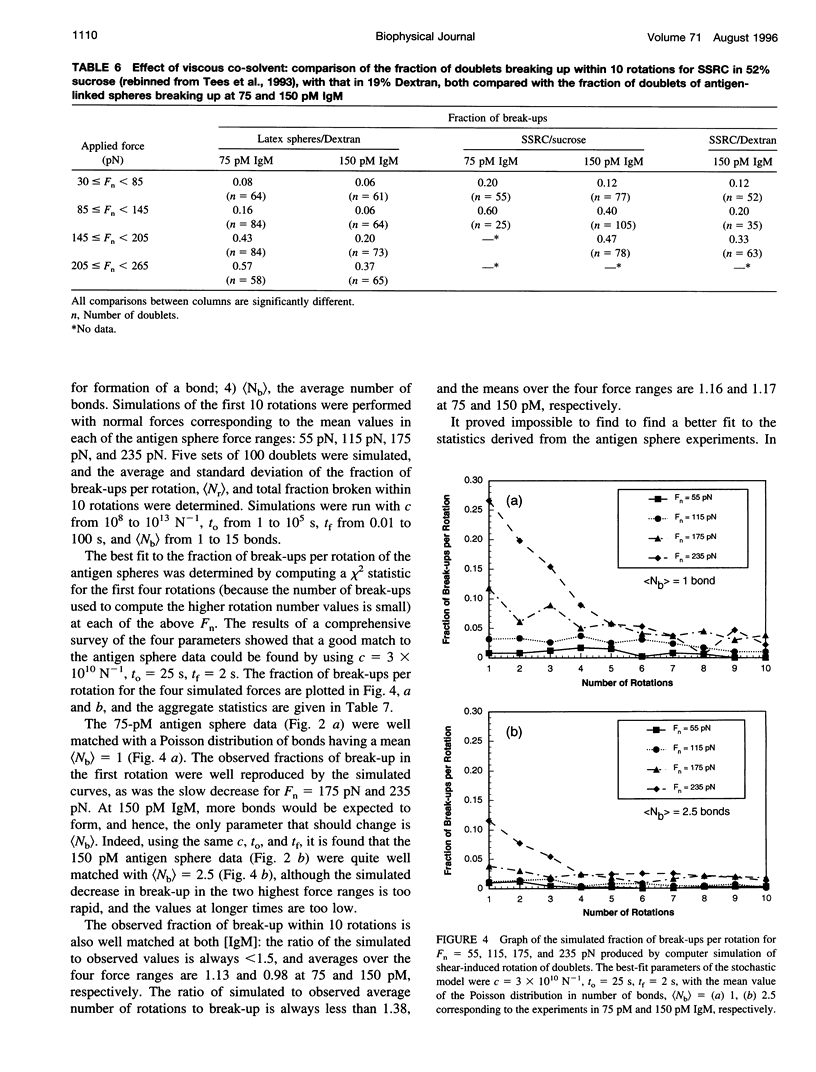
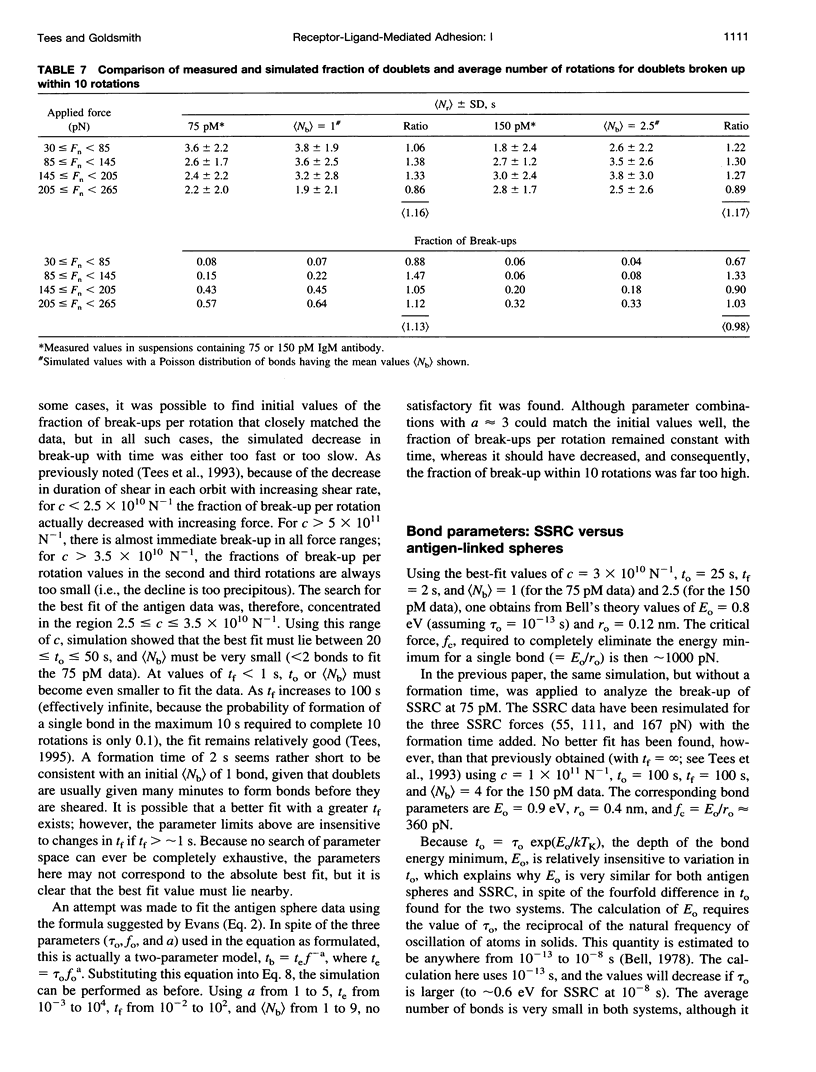
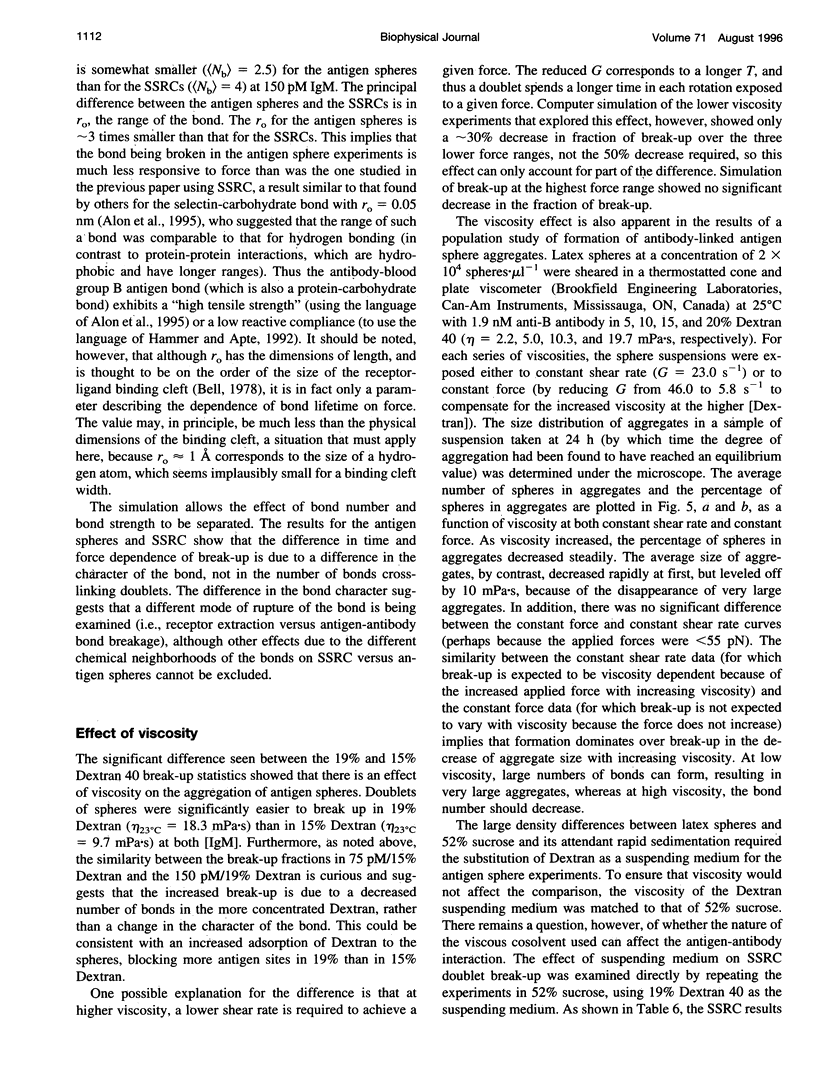
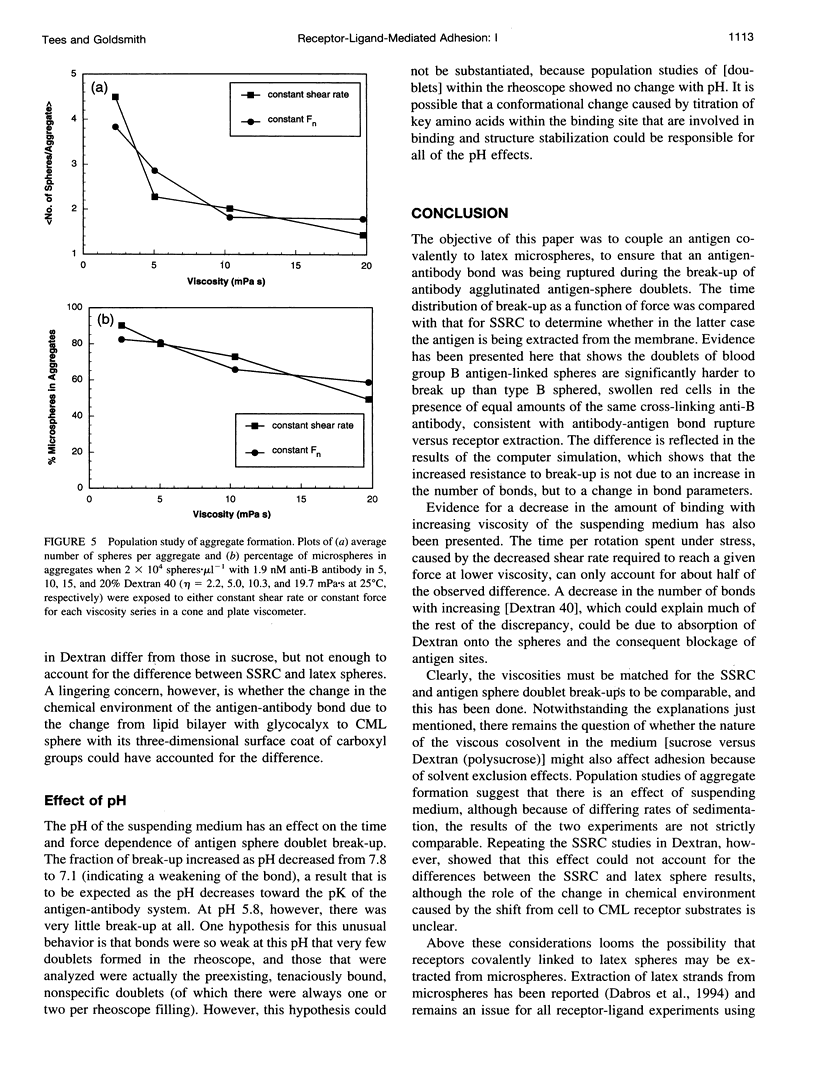
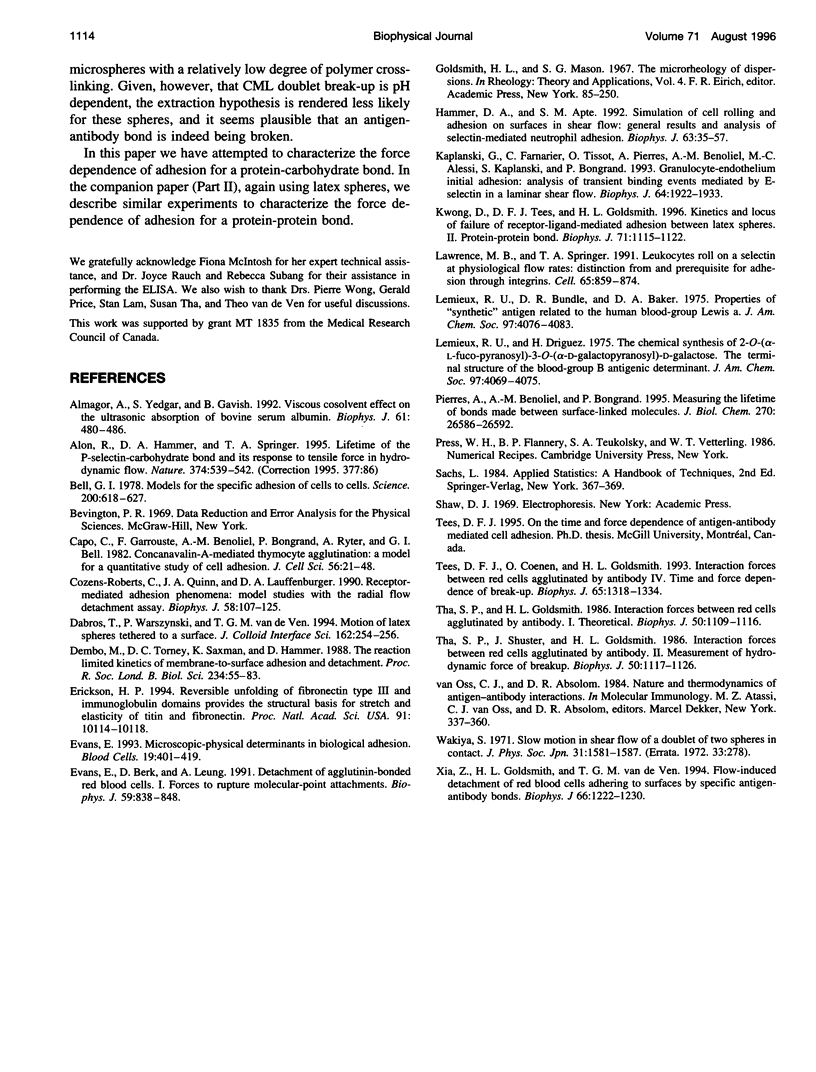
Selected References
These references are in PubMed. This may not be the complete list of references from this article.
- Almagor A., Yedgar S., Gavish B. Viscous cosolvent effect on the ultrasonic absorption of bovine serum albumin. Biophys J. 1992 Feb;61(2):480–486. doi: 10.1016/S0006-3495(92)81852-0. [DOI] [PMC free article] [PubMed] [Google Scholar]
- Alon R., Hammer D. A., Springer T. A. Lifetime of the P-selectin-carbohydrate bond and its response to tensile force in hydrodynamic flow. Nature. 1995 Apr 6;374(6522):539–542. doi: 10.1038/374539a0. [DOI] [PubMed] [Google Scholar]
- Bell G. I. Models for the specific adhesion of cells to cells. Science. 1978 May 12;200(4342):618–627. doi: 10.1126/science.347575. [DOI] [PubMed] [Google Scholar]
- Capo C., Garrouste F., Benoliel A. M., Bongrand P., Ryter A., Bell G. I. Concanavalin-A-mediated thymocyte agglutination: a model for a quantitative study of cell adhesion. J Cell Sci. 1982 Aug;56:21–48. doi: 10.1242/jcs.56.1.21. [DOI] [PubMed] [Google Scholar]
- Cozens-Roberts C., Quinn J. A., Lauffenberger D. A. Receptor-mediated adhesion phenomena. Model studies with the Radical-Flow Detachment Assay. Biophys J. 1990 Jul;58(1):107–125. doi: 10.1016/S0006-3495(90)82357-2. [DOI] [PMC free article] [PubMed] [Google Scholar]
- Dembo M., Torney D. C., Saxman K., Hammer D. The reaction-limited kinetics of membrane-to-surface adhesion and detachment. Proc R Soc Lond B Biol Sci. 1988 Jun 22;234(1274):55–83. doi: 10.1098/rspb.1988.0038. [DOI] [PubMed] [Google Scholar]
- Erickson H. P. Reversible unfolding of fibronectin type III and immunoglobulin domains provides the structural basis for stretch and elasticity of titin and fibronectin. Proc Natl Acad Sci U S A. 1994 Oct 11;91(21):10114–10118. doi: 10.1073/pnas.91.21.10114. [DOI] [PMC free article] [PubMed] [Google Scholar]
- Evans E., Berk D., Leung A. Detachment of agglutinin-bonded red blood cells. I. Forces to rupture molecular-point attachments. Biophys J. 1991 Apr;59(4):838–848. doi: 10.1016/S0006-3495(91)82296-2. [DOI] [PMC free article] [PubMed] [Google Scholar]
- Evans E. Microscopic-physical determinants in biological adhesion. Blood Cells. 1993;19(2):401–419. [PubMed] [Google Scholar]
- Hammer D. A., Apte S. M. Simulation of cell rolling and adhesion on surfaces in shear flow: general results and analysis of selectin-mediated neutrophil adhesion. Biophys J. 1992 Jul;63(1):35–57. doi: 10.1016/S0006-3495(92)81577-1. [DOI] [PMC free article] [PubMed] [Google Scholar]
- Kaplanski G., Farnarier C., Tissot O., Pierres A., Benoliel A. M., Alessi M. C., Kaplanski S., Bongrand P. Granulocyte-endothelium initial adhesion. Analysis of transient binding events mediated by E-selectin in a laminar shear flow. Biophys J. 1993 Jun;64(6):1922–1933. doi: 10.1016/S0006-3495(93)81563-7. [DOI] [PMC free article] [PubMed] [Google Scholar]
- Kwong D., Tees D. F., Goldsmith H. L. Kinetics and locus of failure of receptor-ligand-mediated adhesion between latex spheres. II. Protein-protein bond. Biophys J. 1996 Aug;71(2):1115–1122. doi: 10.1016/S0006-3495(96)79313-X. [DOI] [PMC free article] [PubMed] [Google Scholar]
- Lawrence M. B., Springer T. A. Leukocytes roll on a selectin at physiologic flow rates: distinction from and prerequisite for adhesion through integrins. Cell. 1991 May 31;65(5):859–873. doi: 10.1016/0092-8674(91)90393-d. [DOI] [PubMed] [Google Scholar]
- Lemieux R. U., Bundle D. R., Baker D. A. The properties of a "synthetic" antigen related to the human blood-group Lewis a. J Am Chem Soc. 1975 Jul 9;97(14):4076–4083. doi: 10.1021/ja00847a035. [DOI] [PubMed] [Google Scholar]
- Lemieux R. U., Driguez H. The chemical synthesis of 2-O-(alpha-L-fucopyranosyl)-3-O-(alpha-D-galactopyranosyl)-D-galactose. The terminal structure of the blood-group B antigenic determinant. J Am Chem Soc. 1975 Jul 9;97(14):4069–4075. doi: 10.1021/ja00847a034. [DOI] [PubMed] [Google Scholar]
- Pierres A., Benoliel A. M., Bongrand P. Measuring the lifetime of bonds made between surface-linked molecules. J Biol Chem. 1995 Nov 3;270(44):26586–26592. doi: 10.1074/jbc.270.44.26586. [DOI] [PubMed] [Google Scholar]
- Tees D. F., Coenen O., Goldsmith H. L. Interaction forces between red cells agglutinated by antibody. IV. Time and force dependence of break-up. Biophys J. 1993 Sep;65(3):1318–1334. doi: 10.1016/S0006-3495(93)81180-9. [DOI] [PMC free article] [PubMed] [Google Scholar]
- Tha S. P., Goldsmith H. L. Interaction forces between red cells agglutinated by antibody. I. Theoretical. Biophys J. 1986 Dec;50(6):1109–1116. doi: 10.1016/S0006-3495(86)83555-X. [DOI] [PMC free article] [PubMed] [Google Scholar]
- Tha S. P., Shuster J., Goldsmith H. L. Interaction forces between red cells agglutinated by antibody. II. Measurement of hydrodynamic force of breakup. Biophys J. 1986 Dec;50(6):1117–1126. doi: 10.1016/S0006-3495(86)83556-1. [DOI] [PMC free article] [PubMed] [Google Scholar]
- Xia Z., Goldsmith H. L., van de Ven T. G. Flow-induced detachment of red blood cells adhering to surfaces by specific antigen-antibody bonds. Biophys J. 1994 Apr;66(4):1222–1230. doi: 10.1016/S0006-3495(94)80906-3. [DOI] [PMC free article] [PubMed] [Google Scholar]