Abstract
We modeled two different mechanisms, a shunting conductance and a slow sodium inactivation, to test whether they could modulate the active propagation of a train of action potentials in a dendritic tree. Computer simulations, using a compartmental model of a pyramidal neuron, suggest that each of these two mechanisms could account for the activity-dependent attenuation and failure of the action potentials in the dendrites during the train. Each mechanism is shown to be in good qualitative agreement with experimental findings on somatic or dendritic stimulation and on the effects of hyperpolarization. The conditions under which branch point failures can be observed, and a few experimentally testable predictions, are presented and discussed.
Full text
PDF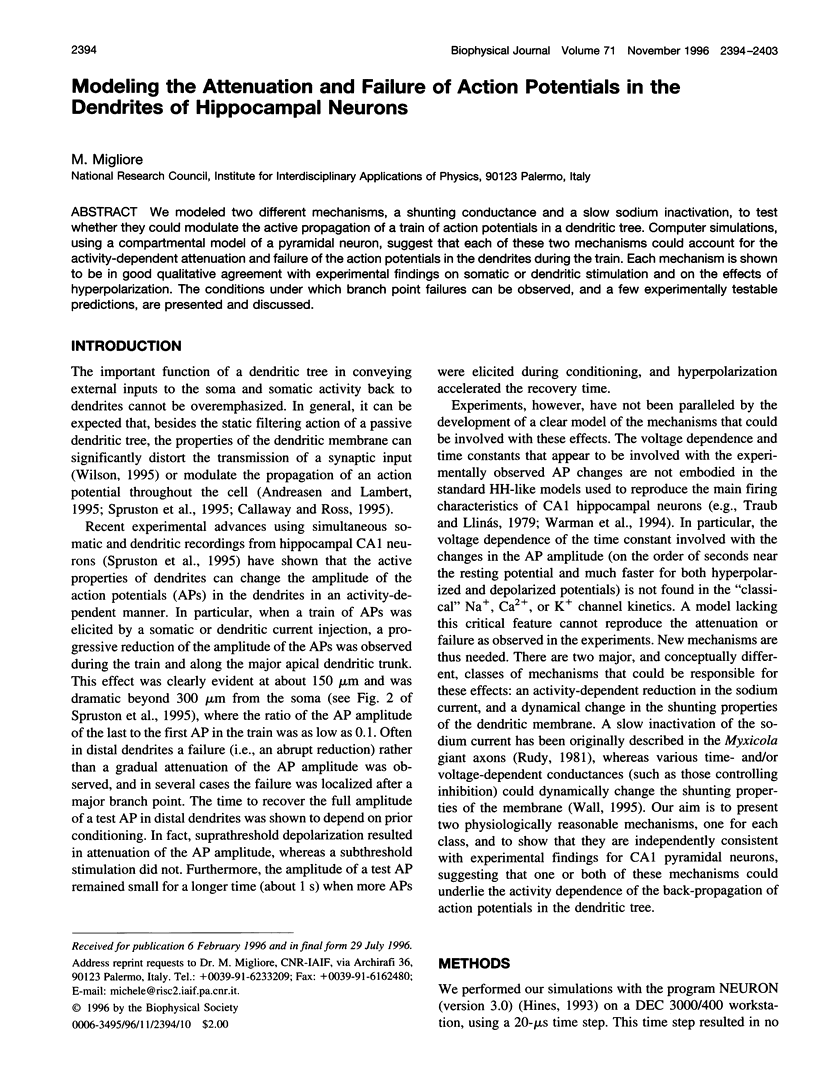
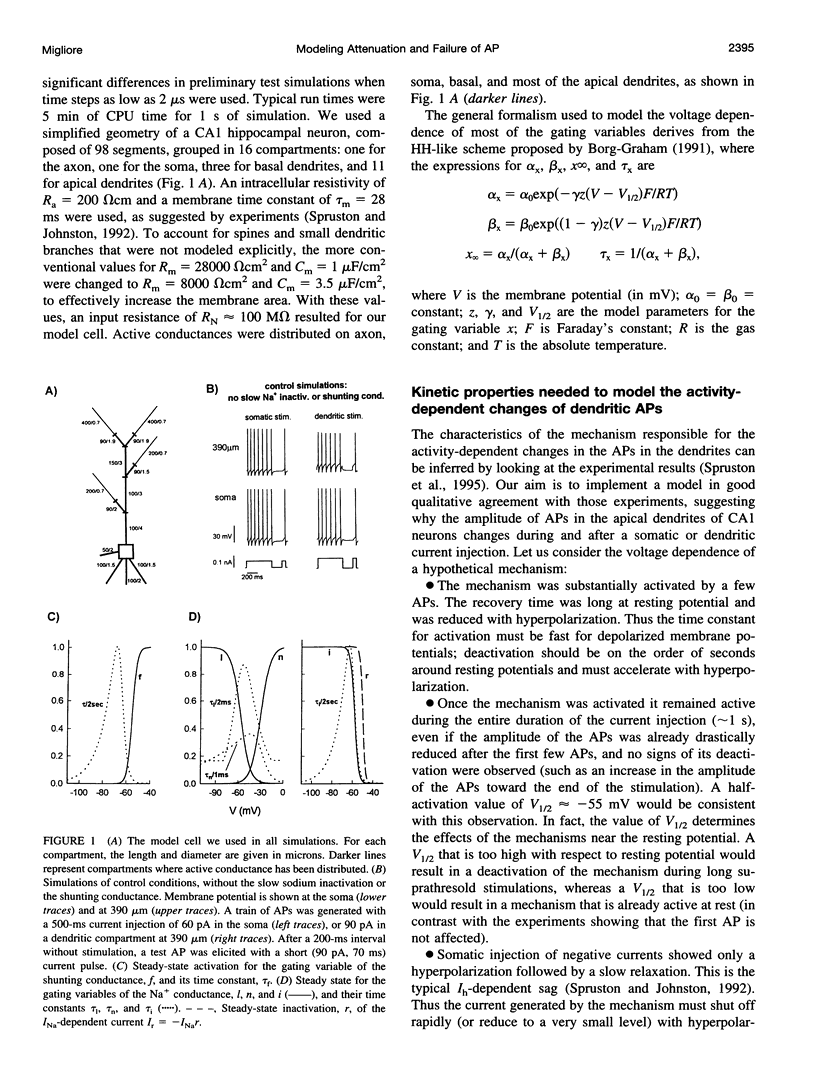
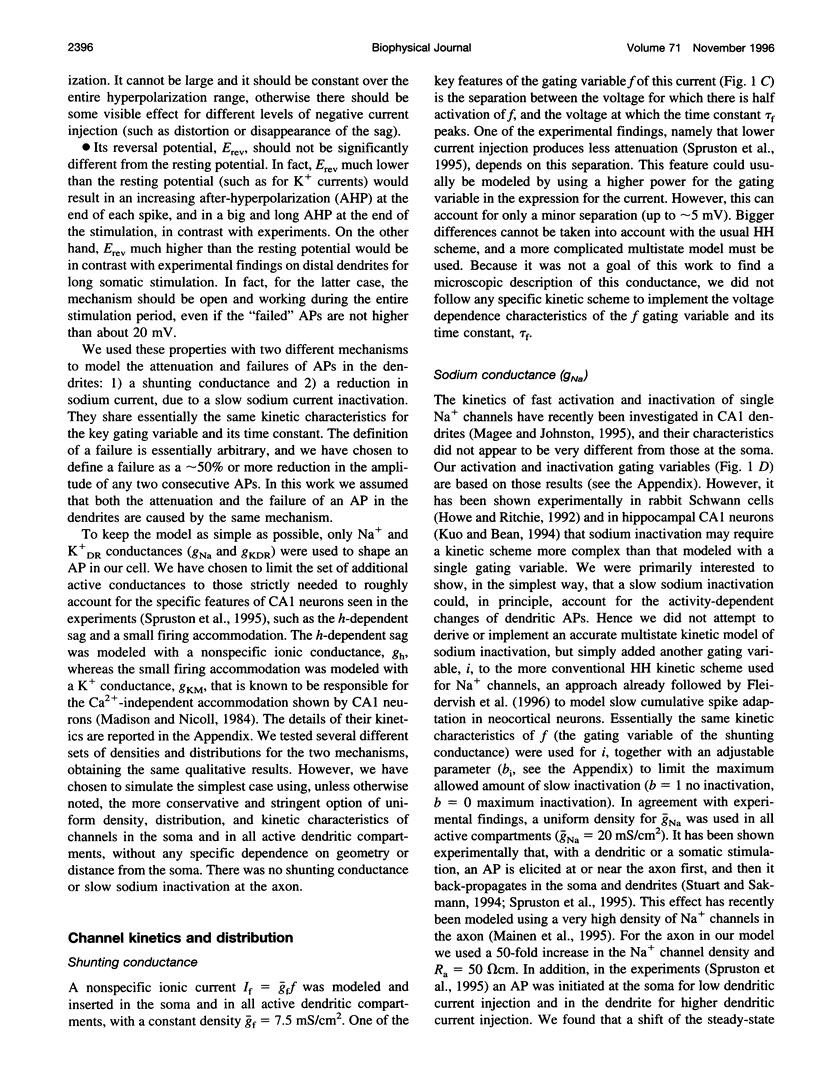
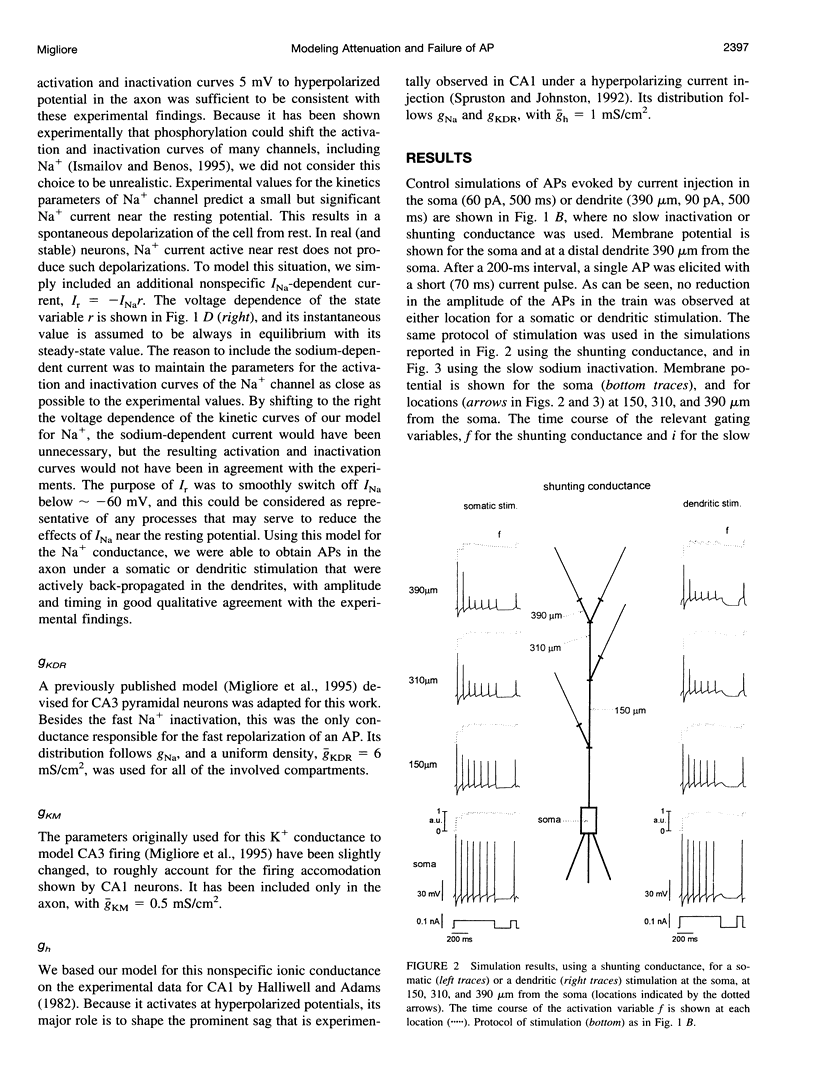
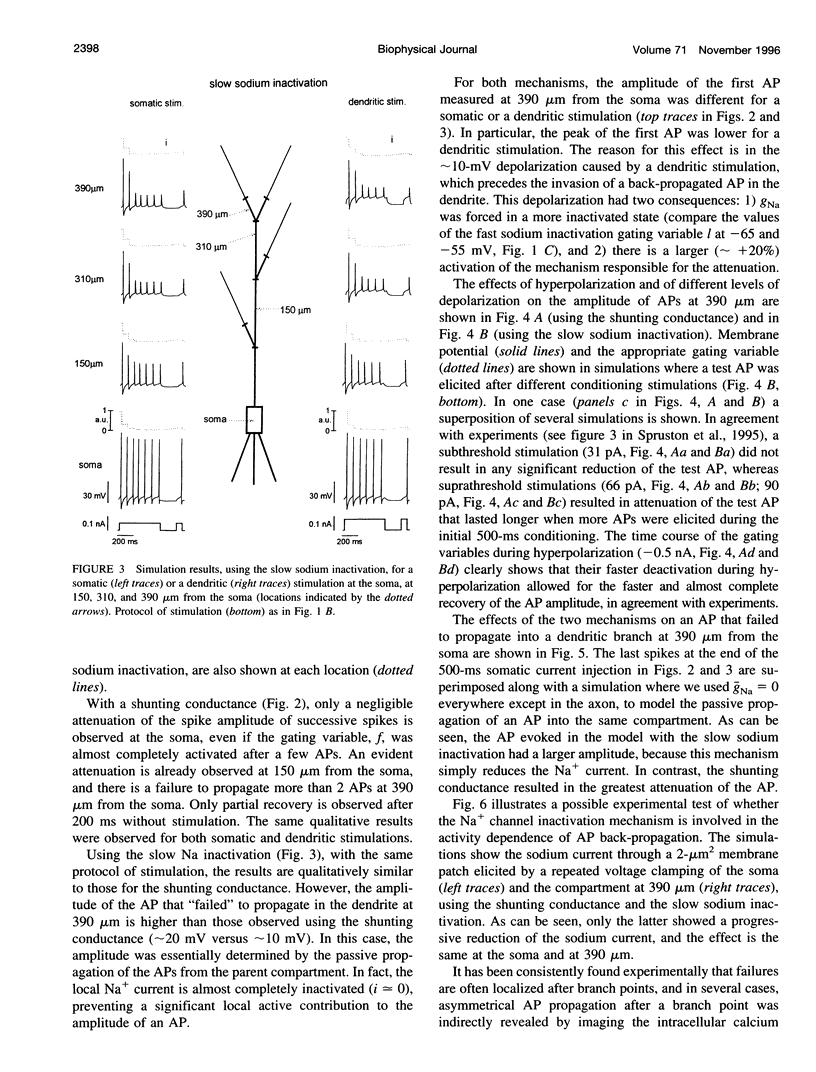
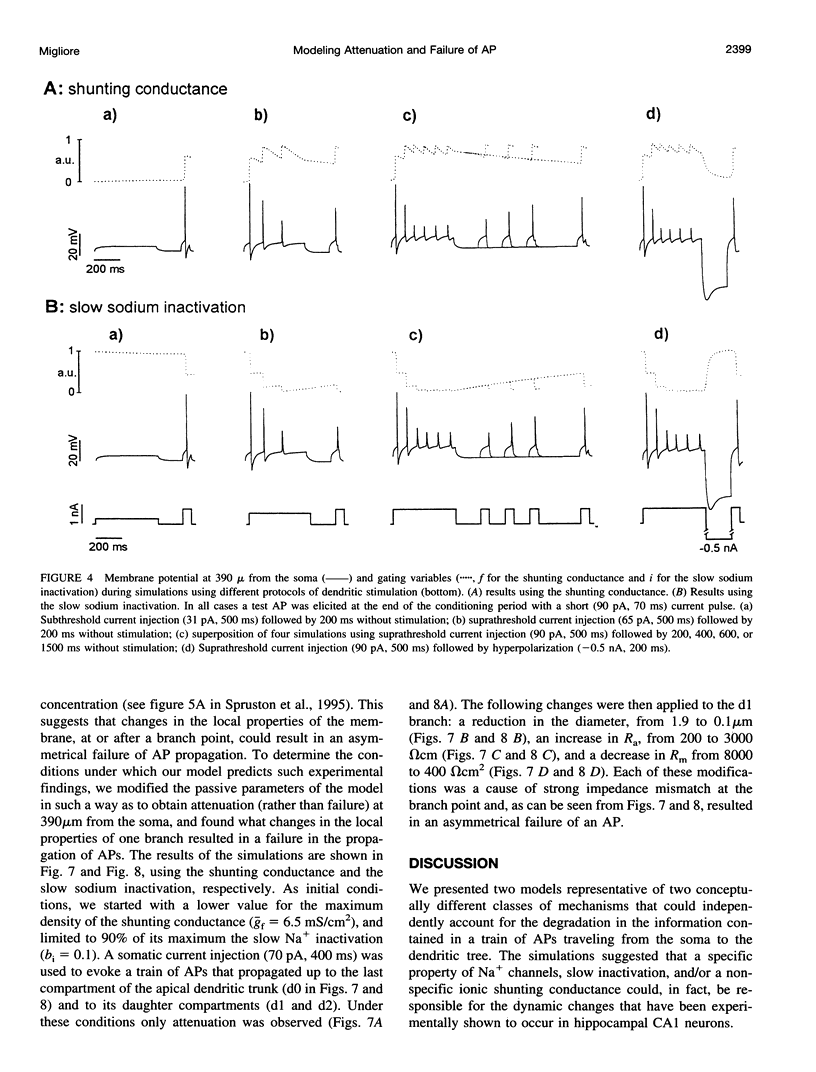
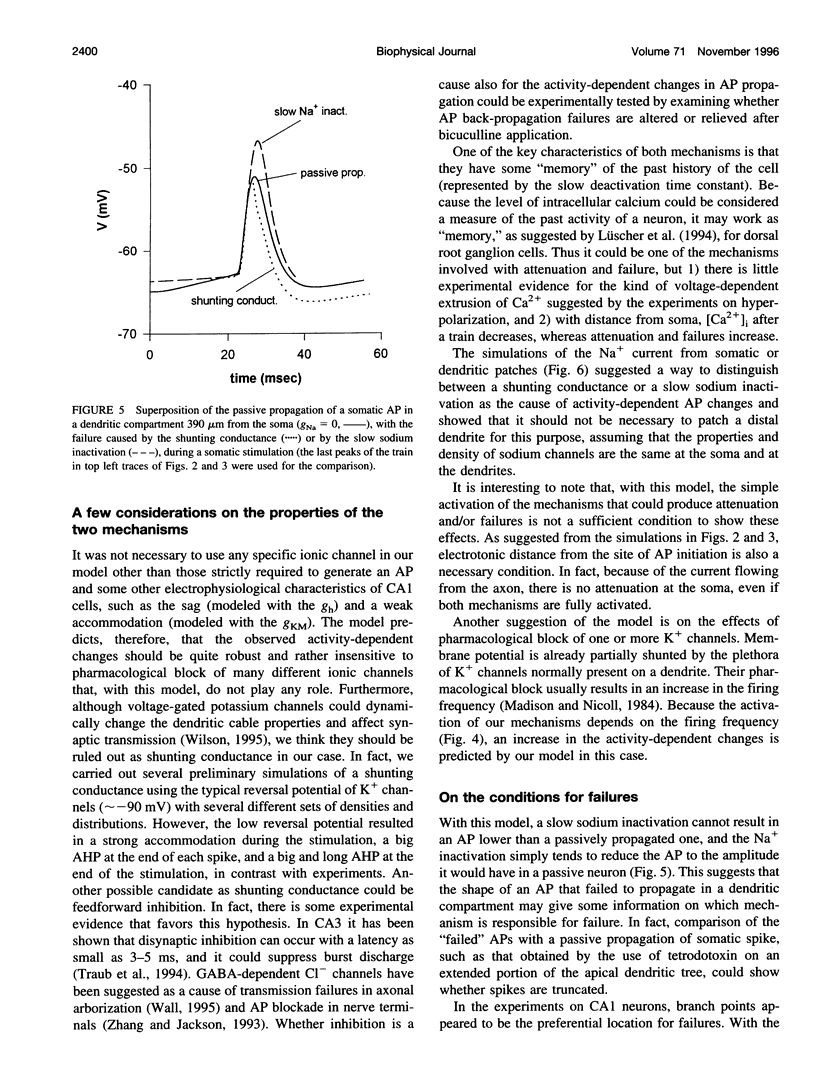
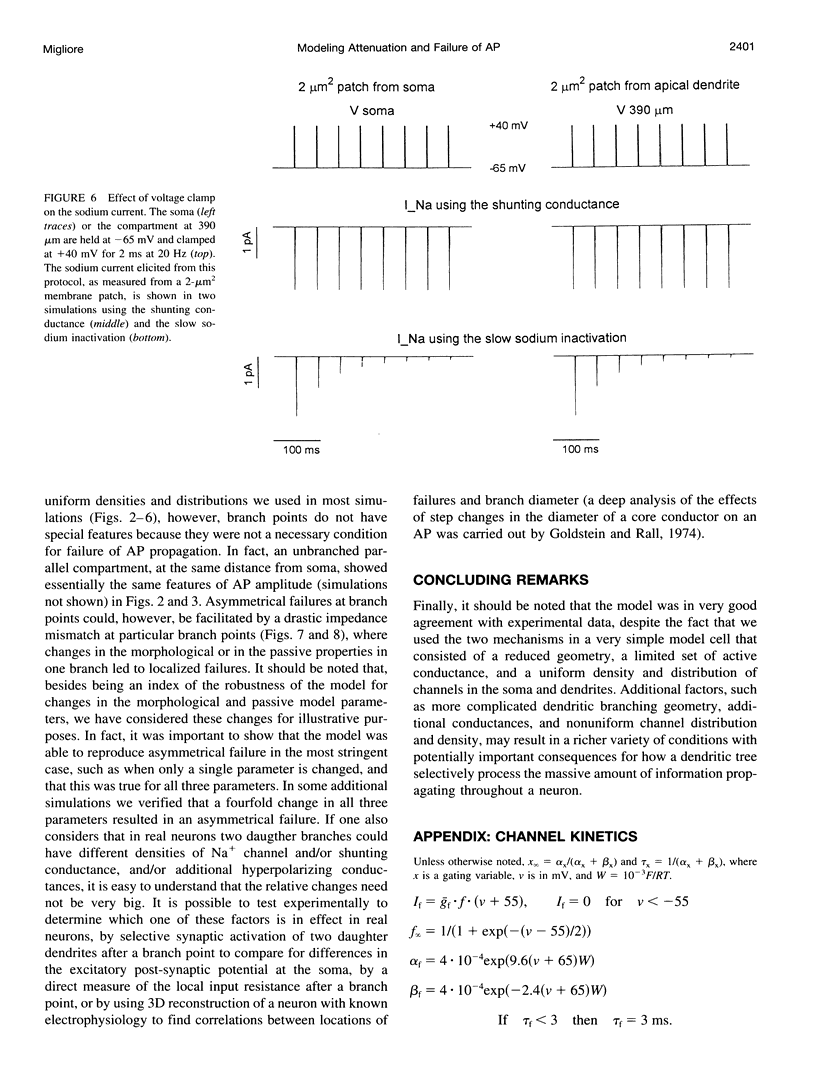
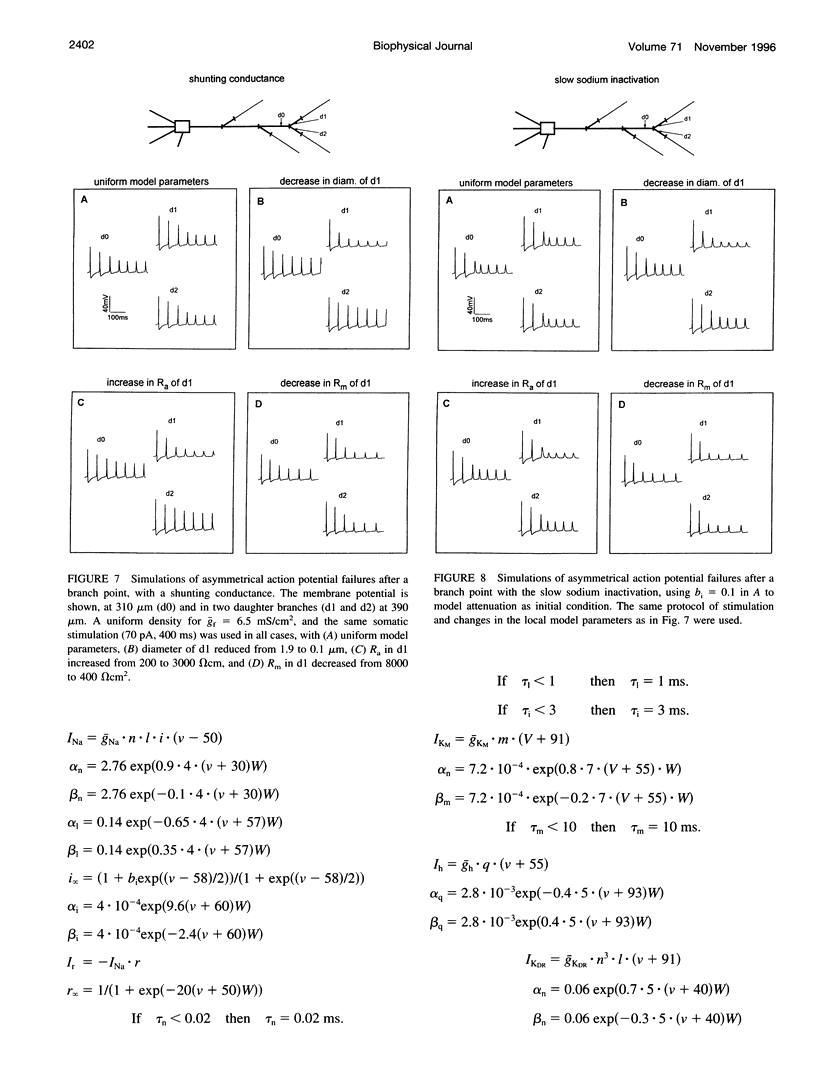
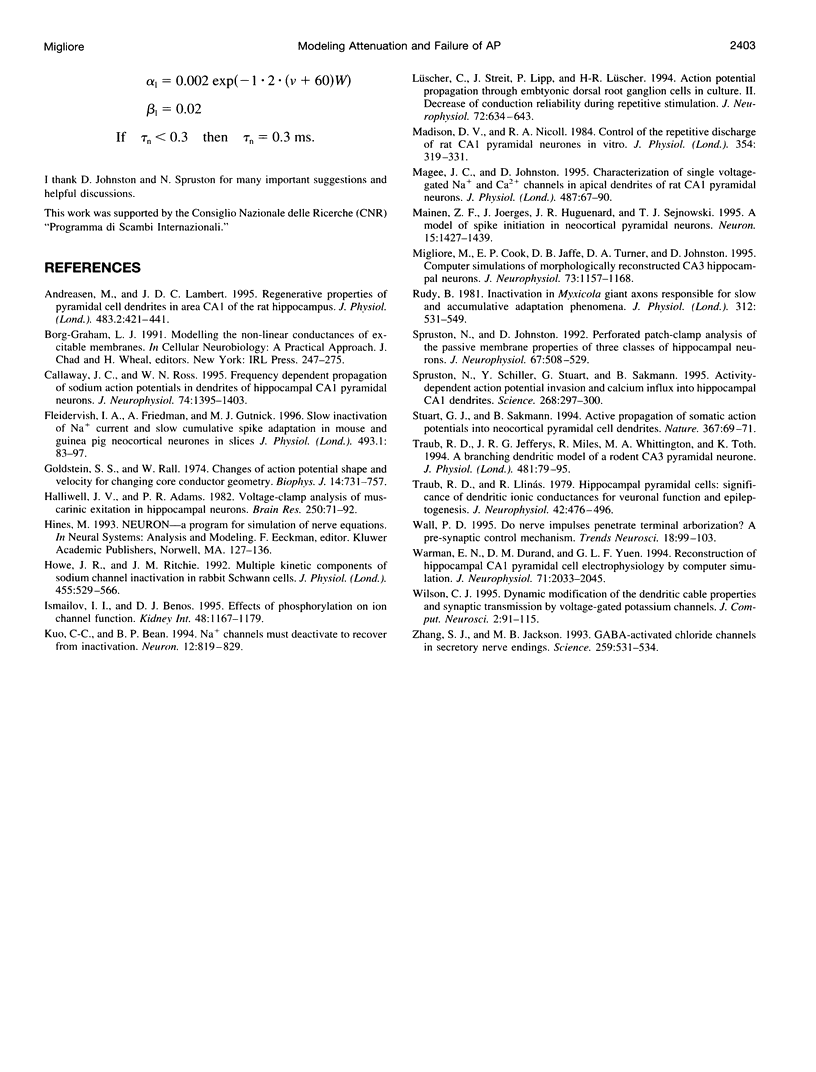
Selected References
These references are in PubMed. This may not be the complete list of references from this article.
- Andreasen M., Lambert J. D. Regenerative properties of pyramidal cell dendrites in area CA1 of the rat hippocampus. J Physiol. 1995 Mar 1;483(Pt 2):421–441. doi: 10.1113/jphysiol.1995.sp020595. [DOI] [PMC free article] [PubMed] [Google Scholar]
- Callaway J. C., Ross W. N. Frequency-dependent propagation of sodium action potentials in dendrites of hippocampal CA1 pyramidal neurons. J Neurophysiol. 1995 Oct;74(4):1395–1403. doi: 10.1152/jn.1995.74.4.1395. [DOI] [PubMed] [Google Scholar]
- Fleidervish I. A., Friedman A., Gutnick M. J. Slow inactivation of Na+ current and slow cumulative spike adaptation in mouse and guinea-pig neocortical neurones in slices. J Physiol. 1996 May 15;493(Pt 1):83–97. doi: 10.1113/jphysiol.1996.sp021366. [DOI] [PMC free article] [PubMed] [Google Scholar]
- Goldstein S. S., Rall W. Changes of action potential shape and velocity for changing core conductor geometry. Biophys J. 1974 Oct;14(10):731–757. doi: 10.1016/S0006-3495(74)85947-3. [DOI] [PMC free article] [PubMed] [Google Scholar]
- Halliwell J. V., Adams P. R. Voltage-clamp analysis of muscarinic excitation in hippocampal neurons. Brain Res. 1982 Oct 28;250(1):71–92. doi: 10.1016/0006-8993(82)90954-4. [DOI] [PubMed] [Google Scholar]
- Howe J. R., Ritchie J. M. Multiple kinetic components of sodium channel inactivation in rabbit Schwann cells. J Physiol. 1992 Sep;455:529–566. doi: 10.1113/jphysiol.1992.sp019315. [DOI] [PMC free article] [PubMed] [Google Scholar]
- Ismailov I. I., Benos D. J. Effects of phosphorylation on ion channel function. Kidney Int. 1995 Oct;48(4):1167–1179. doi: 10.1038/ki.1995.400. [DOI] [PubMed] [Google Scholar]
- Kuo C. C., Bean B. P. Na+ channels must deactivate to recover from inactivation. Neuron. 1994 Apr;12(4):819–829. doi: 10.1016/0896-6273(94)90335-2. [DOI] [PubMed] [Google Scholar]
- Lüscher C., Streit J., Lipp P., Lüscher H. R. Action potential propagation through embryonic dorsal root ganglion cells in culture. II. Decrease of conduction reliability during repetitive stimulation. J Neurophysiol. 1994 Aug;72(2):634–643. doi: 10.1152/jn.1994.72.2.634. [DOI] [PubMed] [Google Scholar]
- Madison D. V., Nicoll R. A. Control of the repetitive discharge of rat CA 1 pyramidal neurones in vitro. J Physiol. 1984 Sep;354:319–331. doi: 10.1113/jphysiol.1984.sp015378. [DOI] [PMC free article] [PubMed] [Google Scholar]
- Magee J. C., Johnston D. Characterization of single voltage-gated Na+ and Ca2+ channels in apical dendrites of rat CA1 pyramidal neurons. J Physiol. 1995 Aug 15;487(1):67–90. doi: 10.1113/jphysiol.1995.sp020862. [DOI] [PMC free article] [PubMed] [Google Scholar]
- Mainen Z. F., Joerges J., Huguenard J. R., Sejnowski T. J. A model of spike initiation in neocortical pyramidal neurons. Neuron. 1995 Dec;15(6):1427–1439. doi: 10.1016/0896-6273(95)90020-9. [DOI] [PubMed] [Google Scholar]
- Migliore M., Cook E. P., Jaffe D. B., Turner D. A., Johnston D. Computer simulations of morphologically reconstructed CA3 hippocampal neurons. J Neurophysiol. 1995 Mar;73(3):1157–1168. doi: 10.1152/jn.1995.73.3.1157. [DOI] [PubMed] [Google Scholar]
- Rudy B. Inactivation in Myxicola giant axons responsible for slow and accumulative adaptation phenomena. J Physiol. 1981 Mar;312:531–549. doi: 10.1113/jphysiol.1981.sp013642. [DOI] [PMC free article] [PubMed] [Google Scholar]
- Spruston N., Johnston D. Perforated patch-clamp analysis of the passive membrane properties of three classes of hippocampal neurons. J Neurophysiol. 1992 Mar;67(3):508–529. doi: 10.1152/jn.1992.67.3.508. [DOI] [PubMed] [Google Scholar]
- Spruston N., Schiller Y., Stuart G., Sakmann B. Activity-dependent action potential invasion and calcium influx into hippocampal CA1 dendrites. Science. 1995 Apr 14;268(5208):297–300. doi: 10.1126/science.7716524. [DOI] [PubMed] [Google Scholar]
- Stuart G. J., Sakmann B. Active propagation of somatic action potentials into neocortical pyramidal cell dendrites. Nature. 1994 Jan 6;367(6458):69–72. doi: 10.1038/367069a0. [DOI] [PubMed] [Google Scholar]
- Traub R. D., Jefferys J. G., Miles R., Whittington M. A., Tóth K. A branching dendritic model of a rodent CA3 pyramidal neurone. J Physiol. 1994 Nov 15;481(Pt 1):79–95. doi: 10.1113/jphysiol.1994.sp020420. [DOI] [PMC free article] [PubMed] [Google Scholar]
- Traub R. D., Llinás R. Hippocampal pyramidal cells: significance of dendritic ionic conductances for neuronal function and epileptogenesis. J Neurophysiol. 1979 Mar;42(2):476–496. doi: 10.1152/jn.1979.42.2.476. [DOI] [PubMed] [Google Scholar]
- Wall P. D. Do nerve impulses penetrate terminal arborizations? A pre-presynaptic control mechanism. Trends Neurosci. 1995 Feb;18(2):99–103. doi: 10.1016/0166-2236(95)93883-y. [DOI] [PubMed] [Google Scholar]
- Warman E. N., Durand D. M., Yuen G. L. Reconstruction of hippocampal CA1 pyramidal cell electrophysiology by computer simulation. J Neurophysiol. 1994 Jun;71(6):2033–2045. doi: 10.1152/jn.1994.71.6.2033. [DOI] [PubMed] [Google Scholar]
- Wilson C. J. Dynamic modification of dendritic cable properties and synaptic transmission by voltage-gated potassium channels. J Comput Neurosci. 1995 Jun;2(2):91–115. doi: 10.1007/BF00961882. [DOI] [PubMed] [Google Scholar]
- Zhang S. J., Jackson M. B. GABA-activated chloride channels in secretory nerve endings. Science. 1993 Jan 22;259(5094):531–534. doi: 10.1126/science.8380942. [DOI] [PubMed] [Google Scholar]