Abstract
The antifungal polyene antibiotic amphotericin B forms channels in lipid membranes that are permeable to ions, water, and nonelectrolytes. Anion, cation, and ion pair coordination in the water-filled pore of the "barrel" unit of the channels was studied by molecular dynamics simulations. Unlike the case of the gramicidin A channel, the water molecules do not create a single-file configuration in the pore, and some cross sections of the channel contain three or four water molecules. Both the anion and cation are strongly bound to ligand groups and water molecules located in the channel. The coordination number of the ions is about six. The chloride has two binding sites in the pore. The binding with water is dominant; more than four water molecules are localized in the anion coordination sphere. Three motifs of the ion coordination were monitored. The dominant motif occurs when the anion is bound to one ligand group. The ion is bound to two or three ligand groups in the less favorable configurations. The strong affinity of cations to the channel is determined by the negatively charged ligand oxygens, whose electrostatic field dominates over the field of the hydrogens. The ligand contribution to the coordination number of the sodium ion is noticeably higher than in the case of the anion. As in the case of the anion, there are three motifs of the cation coordination. The favorable one occurs when the cation is bound to two ligand oxygens. In the less favorable cases, the cation is bound to three or four oxygens. In the contact ion pair, the cation and anion are bound to two ligand oxygens and one ligand hydrogen, respectively. There exist intermediate solvent-shared states of the ion pair. The average distances between ions in these states are twice as large as that of the contact ion pair. The stability of the solvent-shared state is defined by the water molecule oriented along the electrostatic field of both ions.
Full text
PDF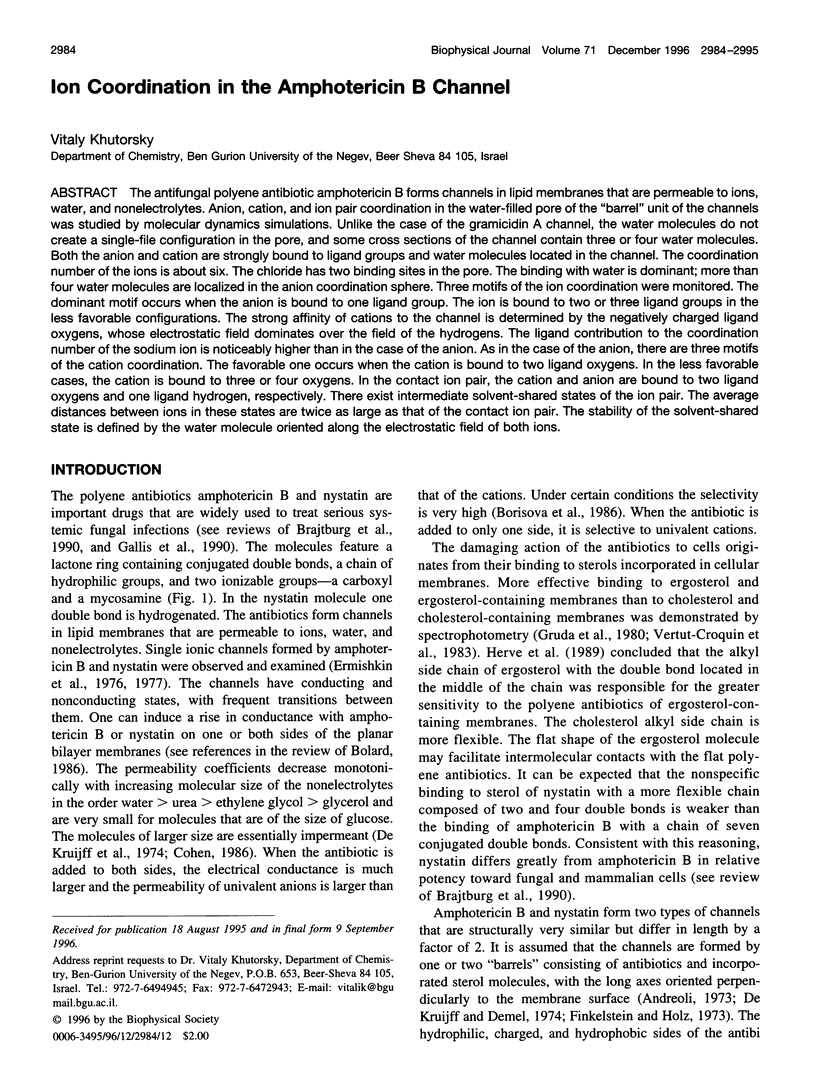
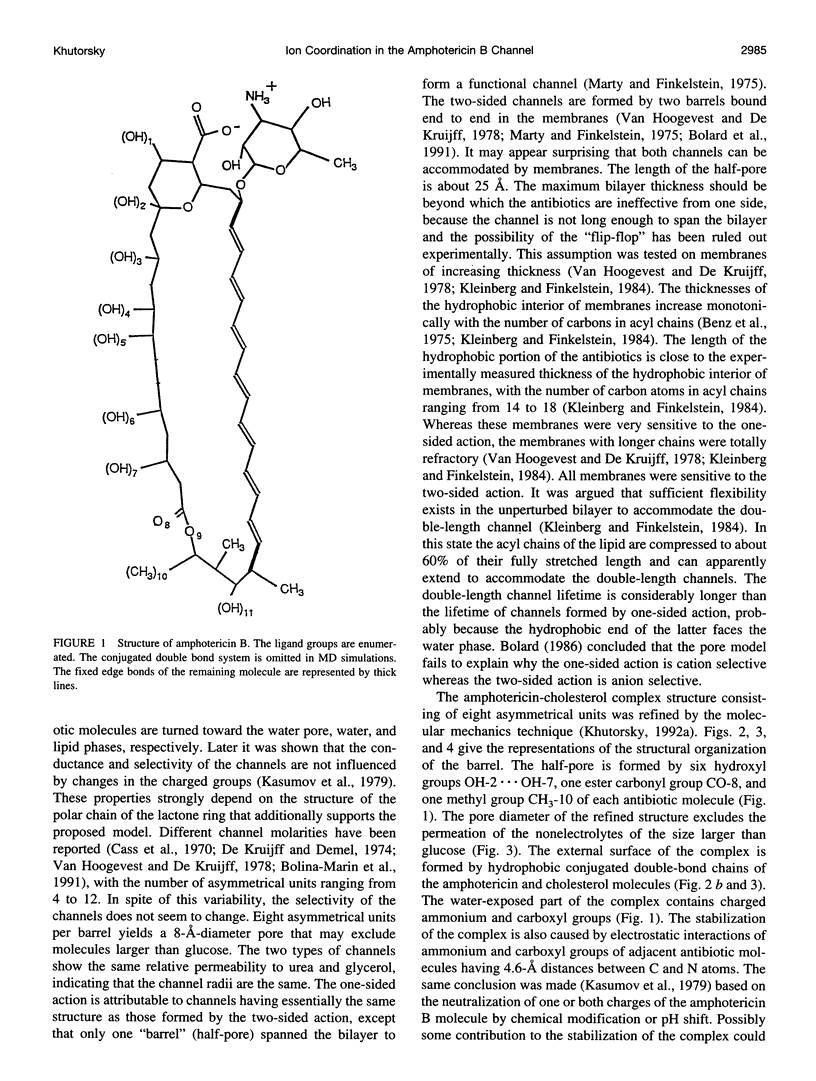
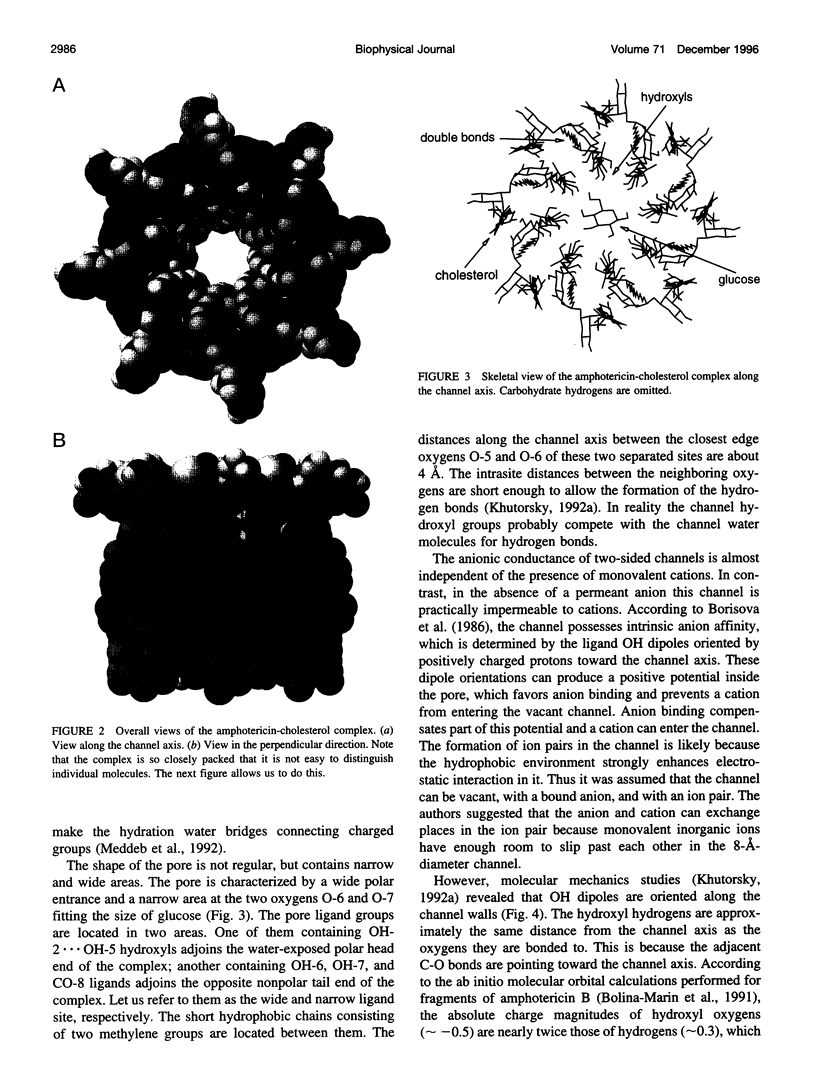
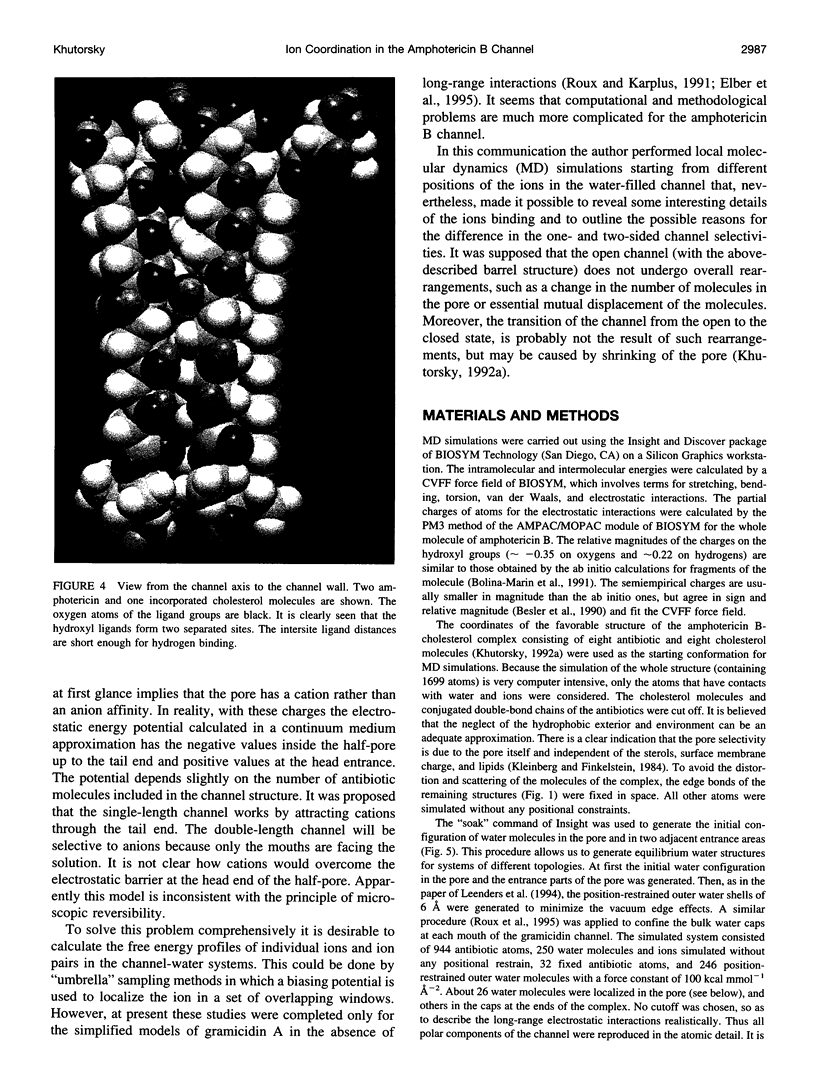
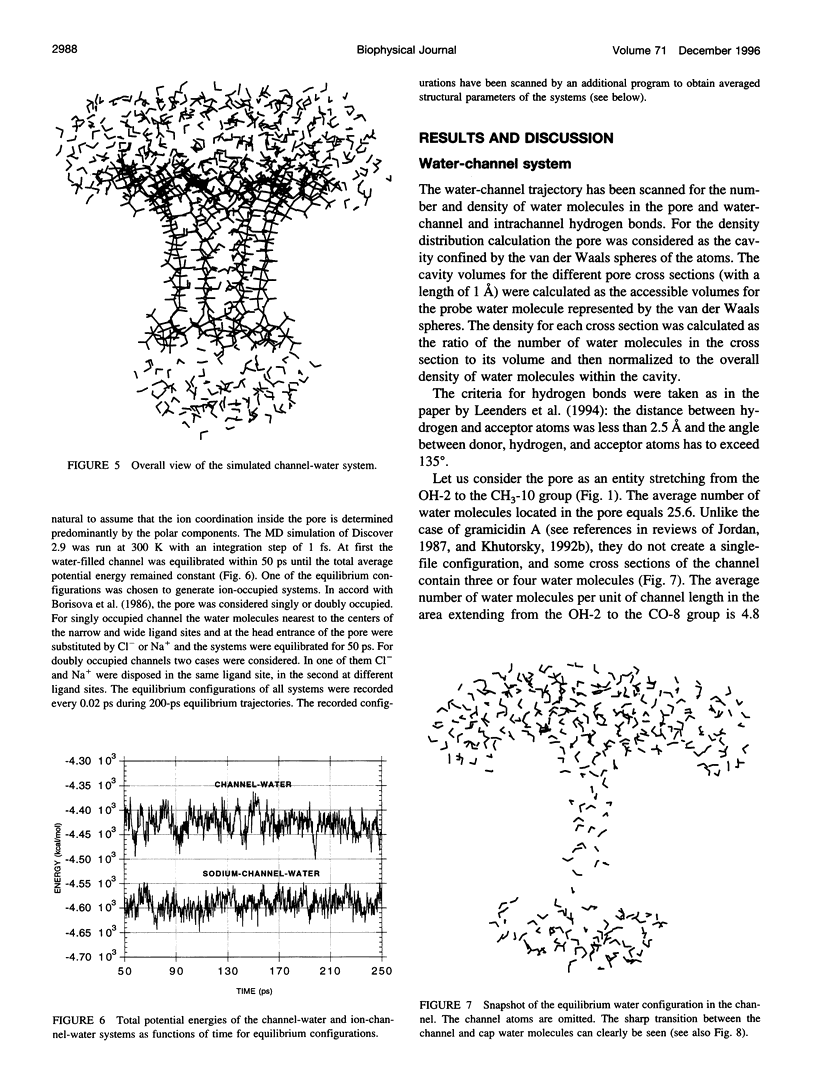
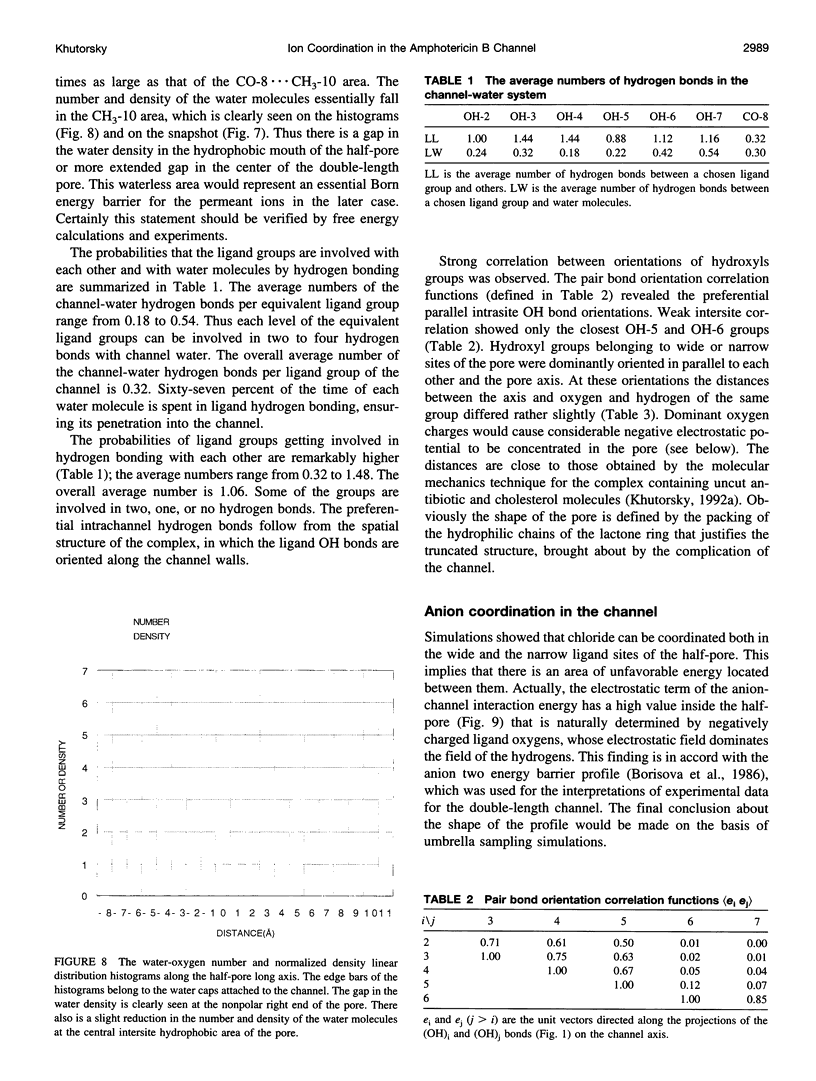
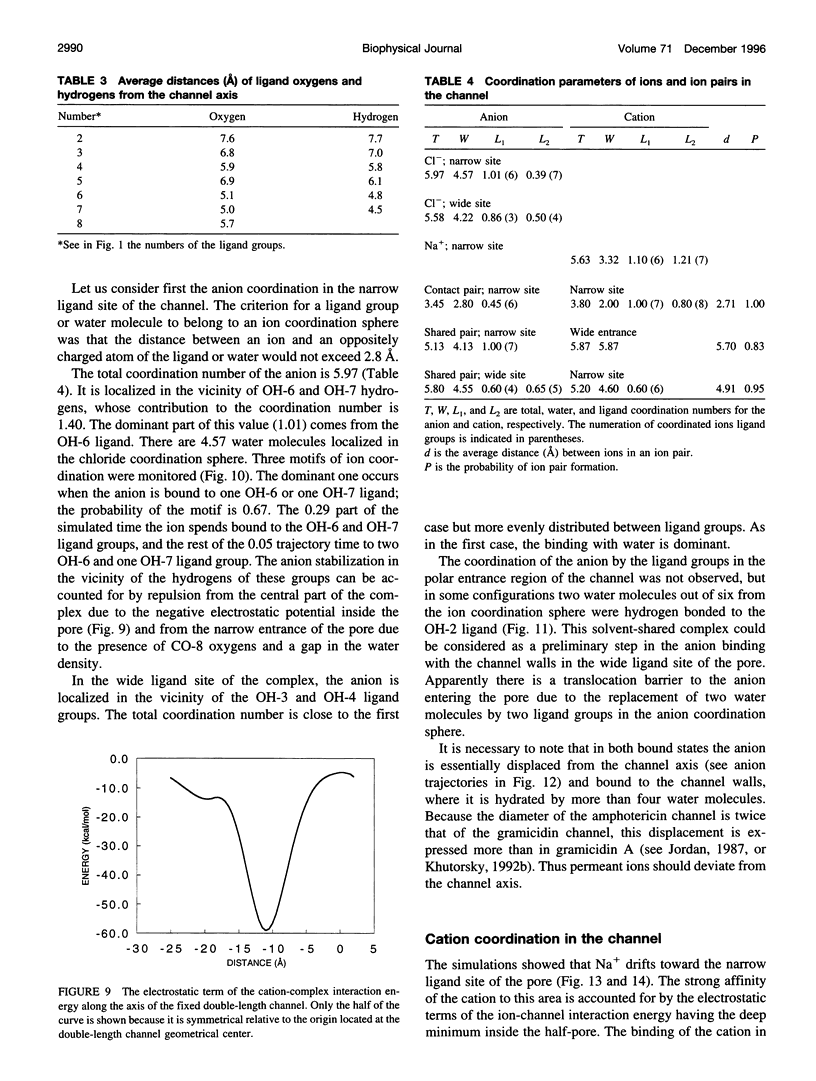
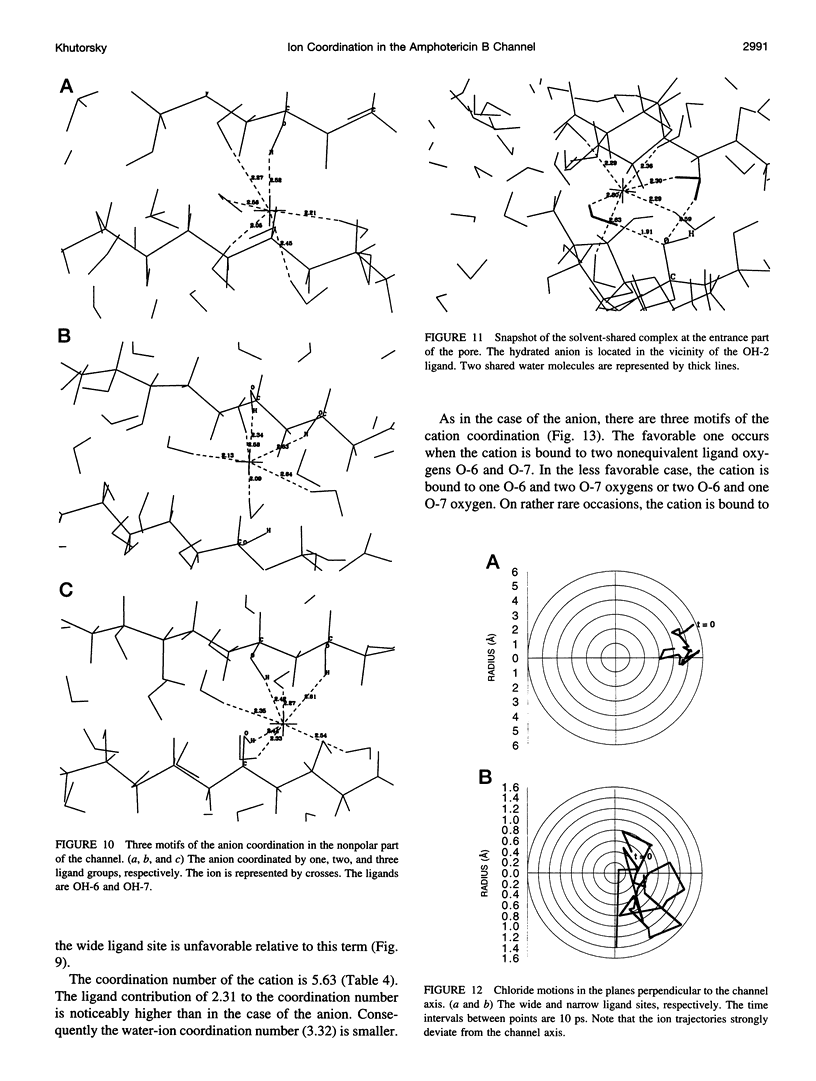
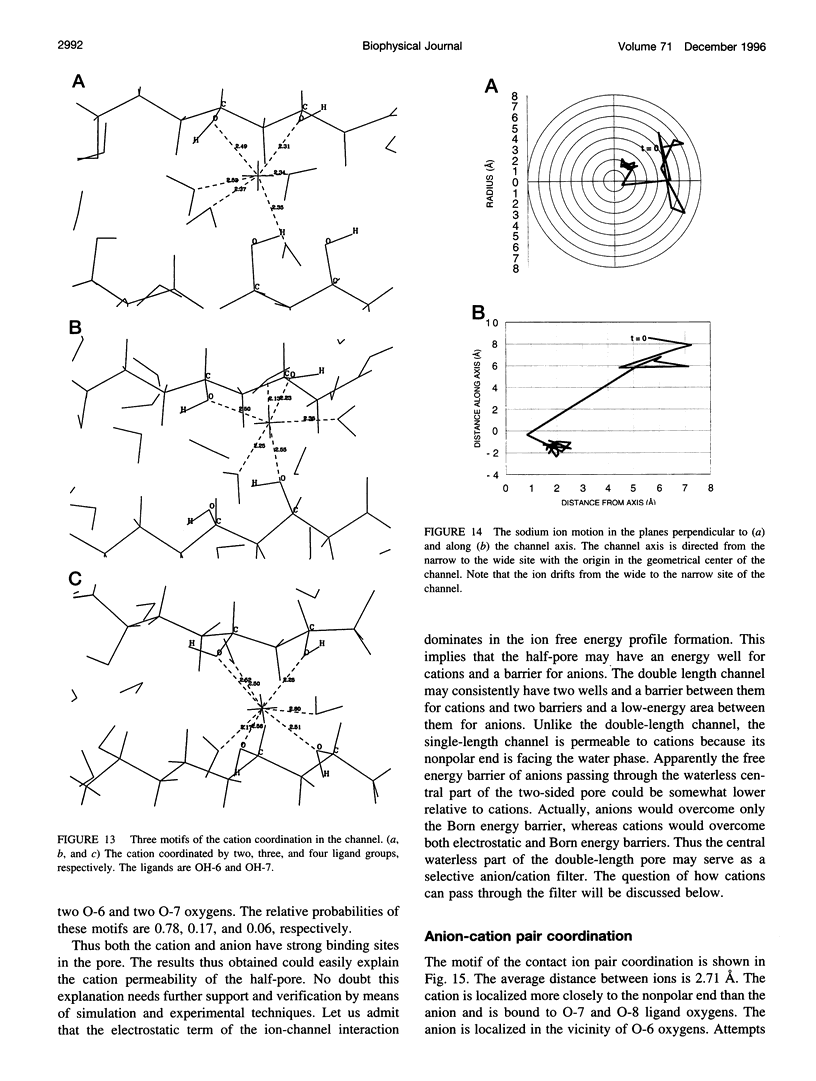
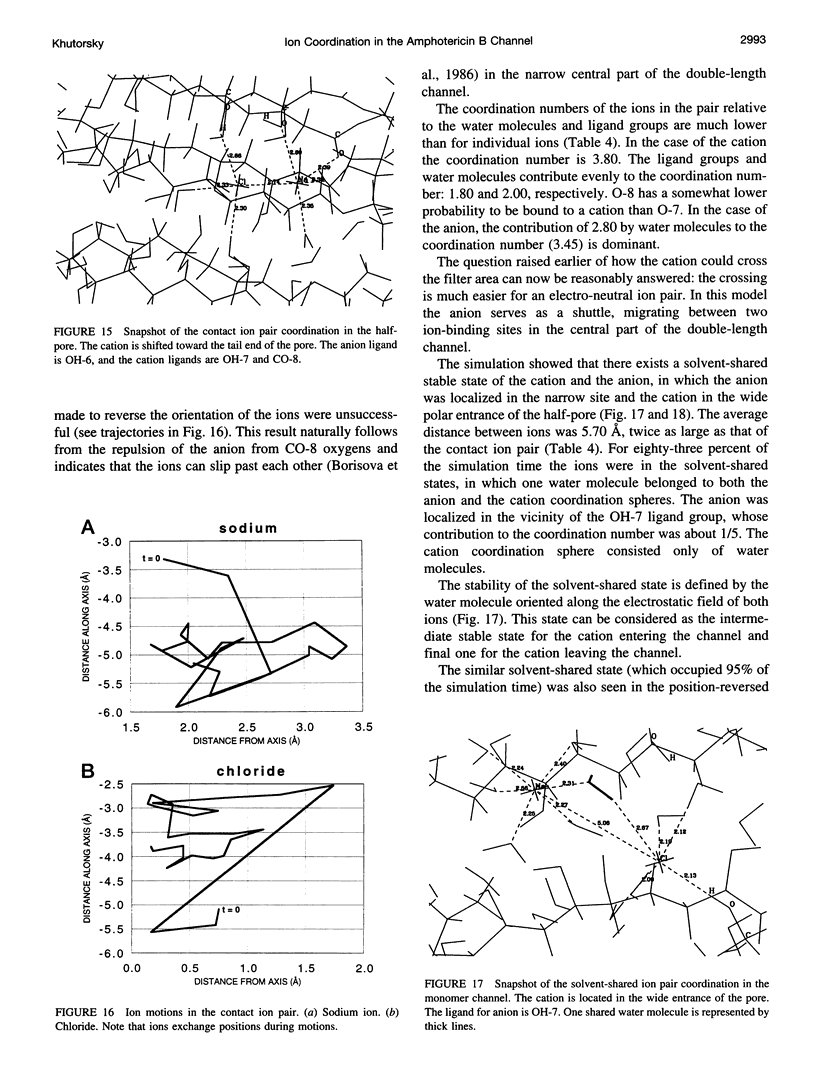
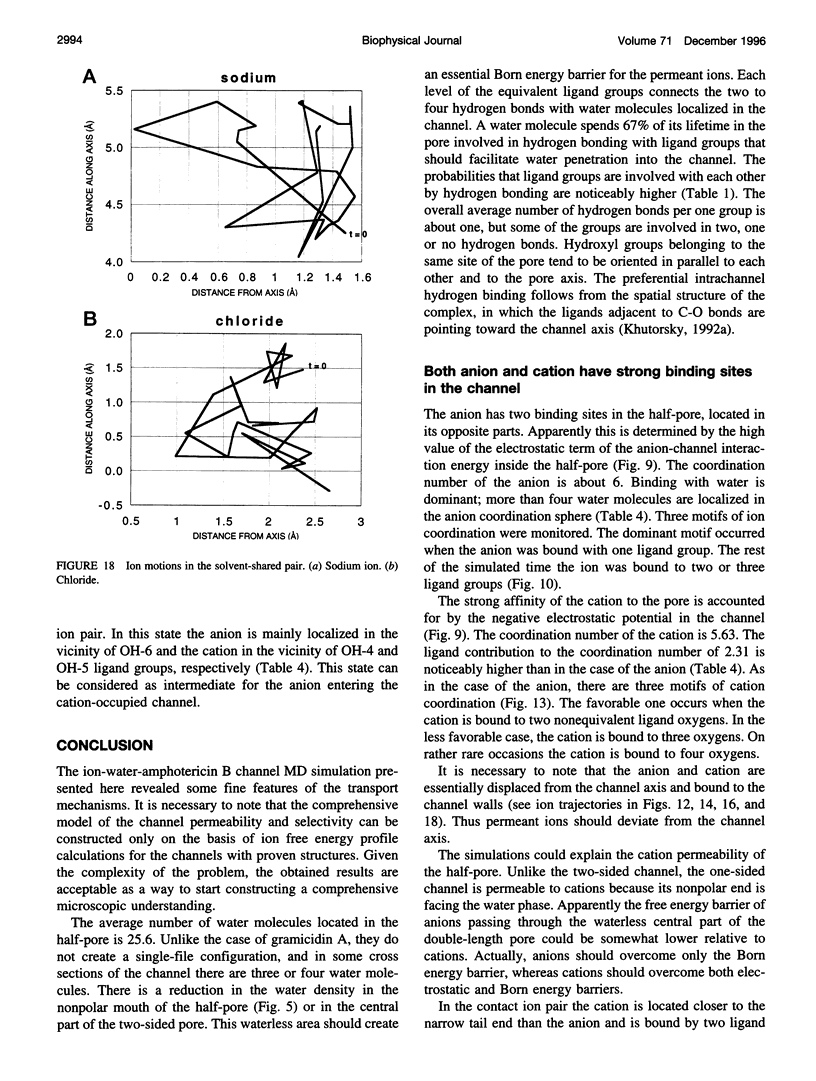
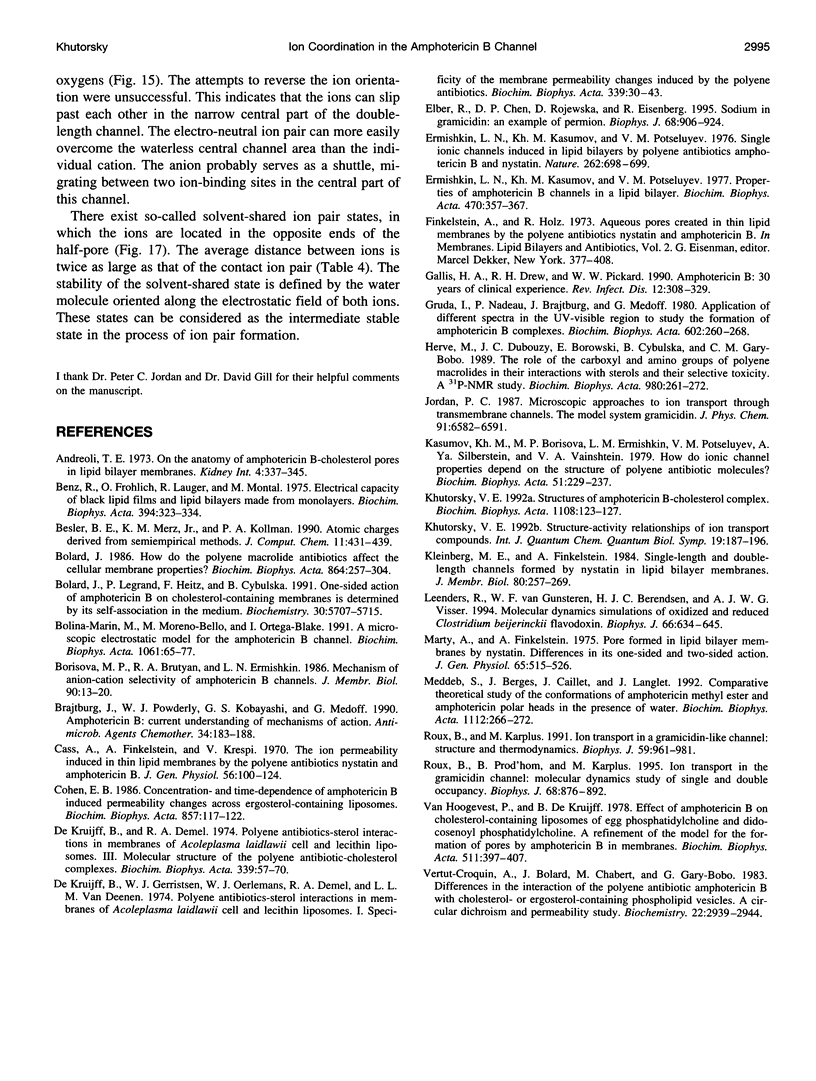
Images in this article
Selected References
These references are in PubMed. This may not be the complete list of references from this article.
- Andreoli T. E. On the anatomy of amphotericin B-cholesterol pores in lipid bilayer membranes. Kidney Int. 1973 Nov;4(5):337–345. doi: 10.1038/ki.1973.126. [DOI] [PubMed] [Google Scholar]
- Benz R., Fröhlich O., Läuger P., Montal M. Electrical capacity of black lipid films and of lipid bilayers made from monolayers. Biochim Biophys Acta. 1975 Jul 3;394(3):323–334. doi: 10.1016/0005-2736(75)90287-4. [DOI] [PubMed] [Google Scholar]
- Bolard J. How do the polyene macrolide antibiotics affect the cellular membrane properties? Biochim Biophys Acta. 1986 Dec 22;864(3-4):257–304. doi: 10.1016/0304-4157(86)90002-x. [DOI] [PubMed] [Google Scholar]
- Bolard J., Legrand P., Heitz F., Cybulska B. One-sided action of amphotericin B on cholesterol-containing membranes is determined by its self-association in the medium. Biochemistry. 1991 Jun 11;30(23):5707–5715. doi: 10.1021/bi00237a011. [DOI] [PubMed] [Google Scholar]
- Bonilla-Marín M., Moreno-Bello M., Ortega-Blake I. A microscopic electrostatic model for the amphotericin B channel. Biochim Biophys Acta. 1991 Jan 9;1061(1):65–77. doi: 10.1016/0005-2736(91)90269-e. [DOI] [PubMed] [Google Scholar]
- Borisova M. P., Brutyan R. A., Ermishkin L. N. Mechanism of anion-cation selectivity of amphotericin B channels. J Membr Biol. 1986;90(1):13–20. doi: 10.1007/BF01869681. [DOI] [PubMed] [Google Scholar]
- Brajtburg J., Powderly W. G., Kobayashi G. S., Medoff G. Amphotericin B: current understanding of mechanisms of action. Antimicrob Agents Chemother. 1990 Feb;34(2):183–188. doi: 10.1128/aac.34.2.183. [DOI] [PMC free article] [PubMed] [Google Scholar]
- Cass A., Finkelstein A., Krespi V. The ion permeability induced in thin lipid membranes by the polyene antibiotics nystatin and amphotericin B. J Gen Physiol. 1970 Jul;56(1):100–124. doi: 10.1085/jgp.56.1.100. [DOI] [PMC free article] [PubMed] [Google Scholar]
- Cohen B. E. Concentration- and time-dependence of amphotericin-B induced permeability changes across ergosterol-containing liposomes. Biochim Biophys Acta. 1986 May 9;857(1):117–122. doi: 10.1016/0005-2736(86)90104-5. [DOI] [PubMed] [Google Scholar]
- Elber R., Chen D. P., Rojewska D., Eisenberg R. Sodium in gramicidin: an example of a permion. Biophys J. 1995 Mar;68(3):906–924. doi: 10.1016/S0006-3495(95)80267-5. [DOI] [PMC free article] [PubMed] [Google Scholar]
- Ermishkin L. N., Kasumov K. M., Potseluyev V. M. Properties of amphotericin B channels in a lipid bilayer. Biochim Biophys Acta. 1977 Nov 1;470(3):357–367. doi: 10.1016/0005-2736(77)90127-4. [DOI] [PubMed] [Google Scholar]
- Ermishkin L. N., Kasumov K. M., Potzeluyev V. M. Single ionic channels induced in lipid bilayers by polyene antibiotics amphotericin B and nystatine. Nature. 1976 Aug 19;262(5570):698–699. doi: 10.1038/262698a0. [DOI] [PubMed] [Google Scholar]
- Finkelstein A., Holz R. Aqueous pores created in thin lipid membranes by the polyene antibiotics nystatin and amphotericin B. Membranes. 1973;2:377–408. [PubMed] [Google Scholar]
- Gallis H. A., Drew R. H., Pickard W. W. Amphotericin B: 30 years of clinical experience. Rev Infect Dis. 1990 Mar-Apr;12(2):308–329. doi: 10.1093/clinids/12.2.308. [DOI] [PubMed] [Google Scholar]
- Gruda I., Nadeau P., Brajtburg J., Medoff G. Application of differential spectra in the ultraviolet-visible region to study the formation of amphotericin B-sterol complexes. Biochim Biophys Acta. 1980 Nov 4;602(2):260–268. doi: 10.1016/0005-2736(80)90309-0. [DOI] [PubMed] [Google Scholar]
- Kasumov K. M., Borisova M. P., Ermishkin L. N., Potseluyev V. M., Silberstein A. Y., Vainshtein V. A. How do ionic channel properties depend on the structure of polyene antibiotic molecules? Biochim Biophys Acta. 1979 Mar 8;551(2):229–237. doi: 10.1016/0005-2736(89)90001-1. [DOI] [PubMed] [Google Scholar]
- Khutorsky V. E. Structures of amphotericin B-cholesterol complex. Biochim Biophys Acta. 1992 Jul 27;1108(2):123–127. doi: 10.1016/0005-2736(92)90015-e. [DOI] [PubMed] [Google Scholar]
- Kleinberg M. E., Finkelstein A. Single-length and double-length channels formed by nystatin in lipid bilayer membranes. J Membr Biol. 1984;80(3):257–269. doi: 10.1007/BF01868444. [DOI] [PubMed] [Google Scholar]
- Leenders R., van Gunsteren W. F., Berendsen H. J., Visser A. J. Molecular dynamics simulations of oxidized and reduced Clostridium beijerinckii flavodoxin. Biophys J. 1994 Mar;66(3 Pt 1):634–645. doi: 10.1016/s0006-3495(94)80837-9. [DOI] [PMC free article] [PubMed] [Google Scholar]
- Marty A., Finkelstein A. Pores formed in lipid bilayer membranes by nystatin, Differences in its one-sided and two-sided action. J Gen Physiol. 1975 Apr;65(4):515–526. doi: 10.1085/jgp.65.4.515. [DOI] [PMC free article] [PubMed] [Google Scholar]
- Meddeb S., Bergès J., Caillet J., Langlet J. Comparative theoretical study of the conformations of amphotericin methyl ester and amphotericin B polar heads in the presence of water. Biochim Biophys Acta. 1992 Dec 9;1112(2):266–272. doi: 10.1016/0005-2736(92)90401-7. [DOI] [PubMed] [Google Scholar]
- Roux B., Karplus M. Ion transport in a model gramicidin channel. Structure and thermodynamics. Biophys J. 1991 May;59(5):961–981. doi: 10.1016/S0006-3495(91)82311-6. [DOI] [PMC free article] [PubMed] [Google Scholar]
- Roux B., Prod'hom B., Karplus M. Ion transport in the gramicidin channel: molecular dynamics study of single and double occupancy. Biophys J. 1995 Mar;68(3):876–892. doi: 10.1016/S0006-3495(95)80264-X. [DOI] [PMC free article] [PubMed] [Google Scholar]
- Vertut-Croquin A., Bolard J., Chabbert M., Gary-Bobo C. Differences in the interaction of the polyene antibiotic amphotericin B with cholesterol- or ergosterol-containing phospholipid vesicles. A circular dichroism and permeability study. Biochemistry. 1983 Jun 7;22(12):2939–2944. doi: 10.1021/bi00281a024. [DOI] [PubMed] [Google Scholar]
- de Kruijff B., Demel R. A. Polyene antibiotic-sterol interactions in membranes of Acholeplasma laidlawii cells and lecithin liposomes. 3. Molecular structure of the polyene antibiotic-cholesterol complexes. Biochim Biophys Acta. 1974 Feb 26;339(1):57–70. doi: 10.1016/0005-2736(74)90332-0. [DOI] [PubMed] [Google Scholar]
- de Kruijff B., Gerritsen W. J., Oerlemans A., Demel R. A., van Deenen L. L. Polyene antibiotic-sterol interactions in membranes of Acholeplasma laidlawii cells and lecithin liposomes. I. Specificity of the membrane permeability changes induced by the polyene antibiotics. Biochim Biophys Acta. 1974 Feb 26;339(1):30–43. doi: 10.1016/0005-2736(74)90330-7. [DOI] [PubMed] [Google Scholar]
- van Hoogevest P., de Kruijff B. Effect of amphotericin B on cholesterol-containing liposomes of egg phosphatidylcholine and didocosenoyl phosphatidylcholine. A refinement of the model for the formation of pores by amphotericin B in membranes. Biochim Biophys Acta. 1978 Aug 17;511(3):397–407. doi: 10.1016/0005-2736(78)90276-6. [DOI] [PubMed] [Google Scholar]