Abstract
In earlier work in which electrorotation was used to apply external torque to tethered cells of the bacterium Escherichia coli, it was found that the torque required to force flagellar motors backward was considerably larger than the torque required to stop them. That is, there appeared to be substantial barrier to backward rotation. Here, we show that in most, possibly all, cases this barrier is an artifact due to angular variation of the torque applied by electrorotation, of the motor torque, or both; the motor torque appears to be independent to speed or to vary linearly with speed up to speeds of tens of Hertz, in either direction. However, motors often break catastrophically when driven backward, so backward rotation is not equivalent to forward rotation. Also, cells can rotate backward while stalled, either in randomly timed jumps of 180 degrees or very slowly and smoothly. When cells rotate slowly and smoothly backward, the motor takes several seconds to recover after electrorotation is stopped, suggesting that some form of reversible damage has occurred. These findings do not affect the interpretation of electrorotation experiments in which motors are driven rapidly forward.
Full text
PDF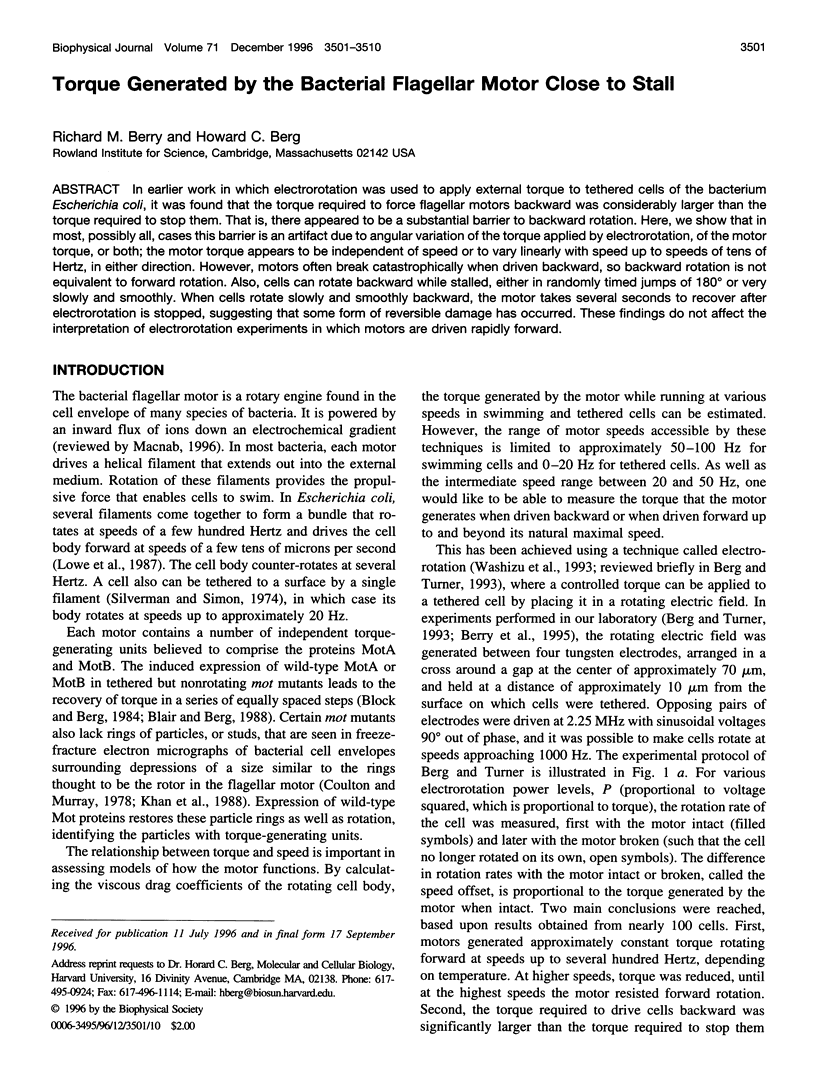
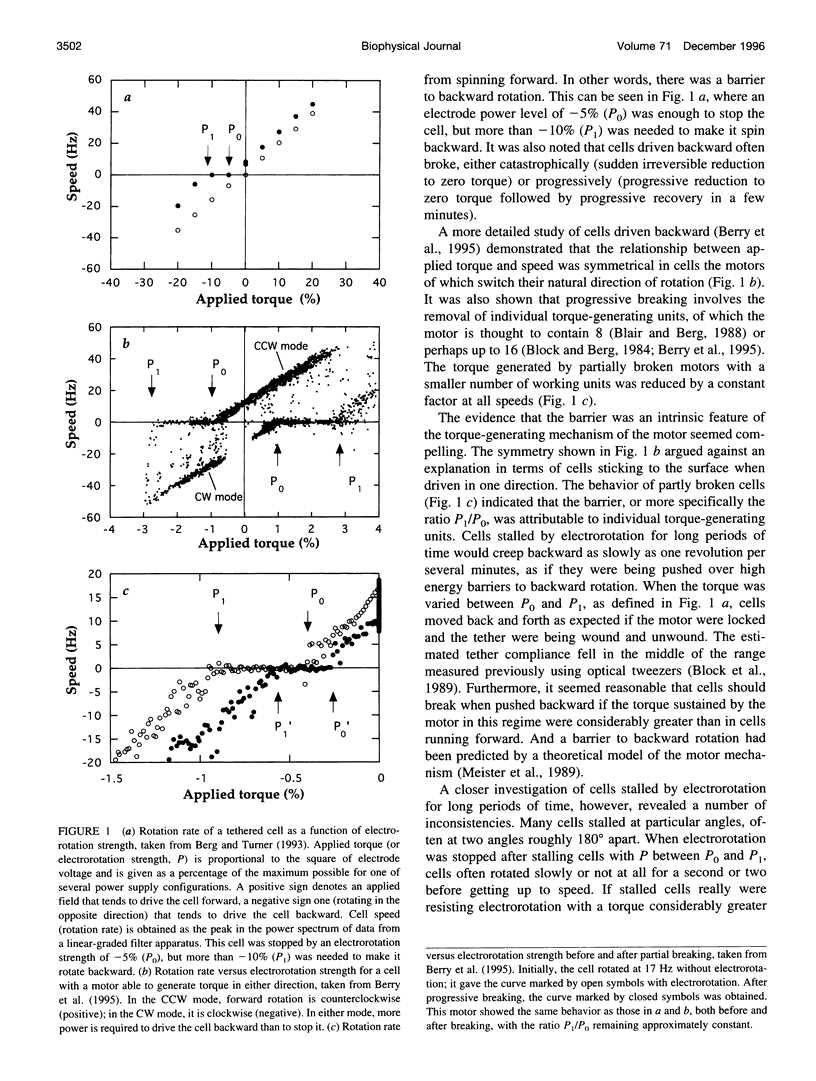
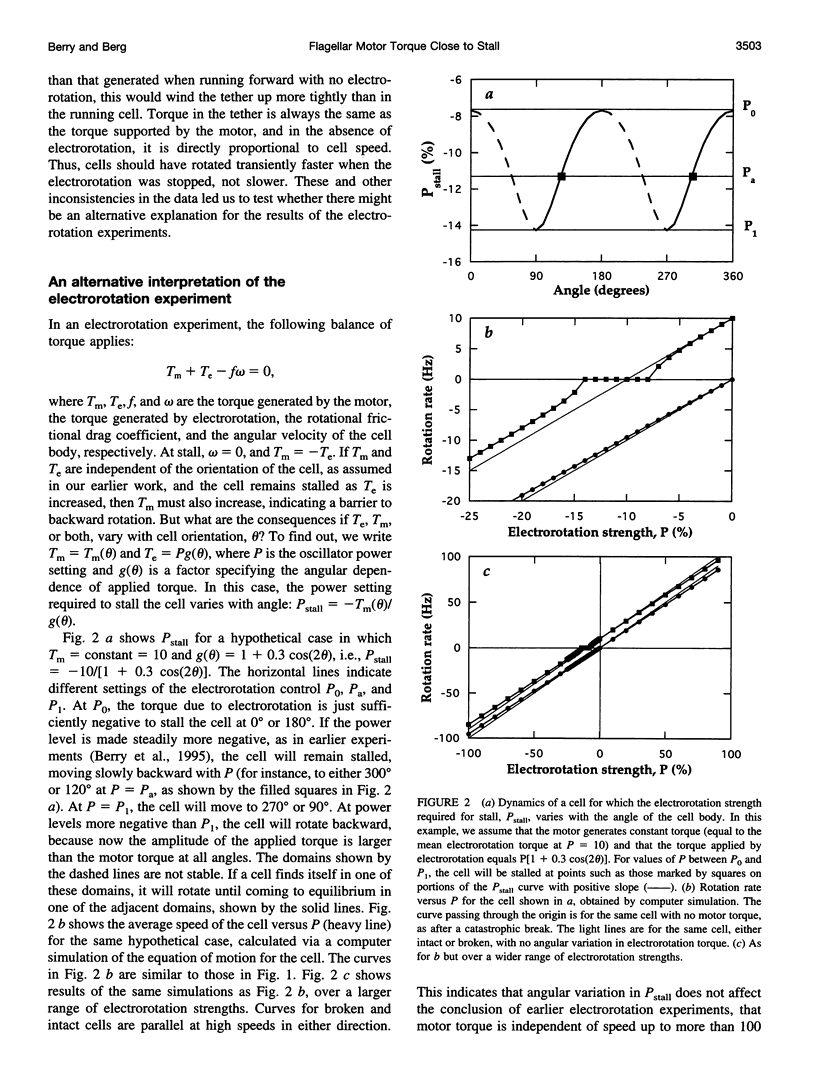
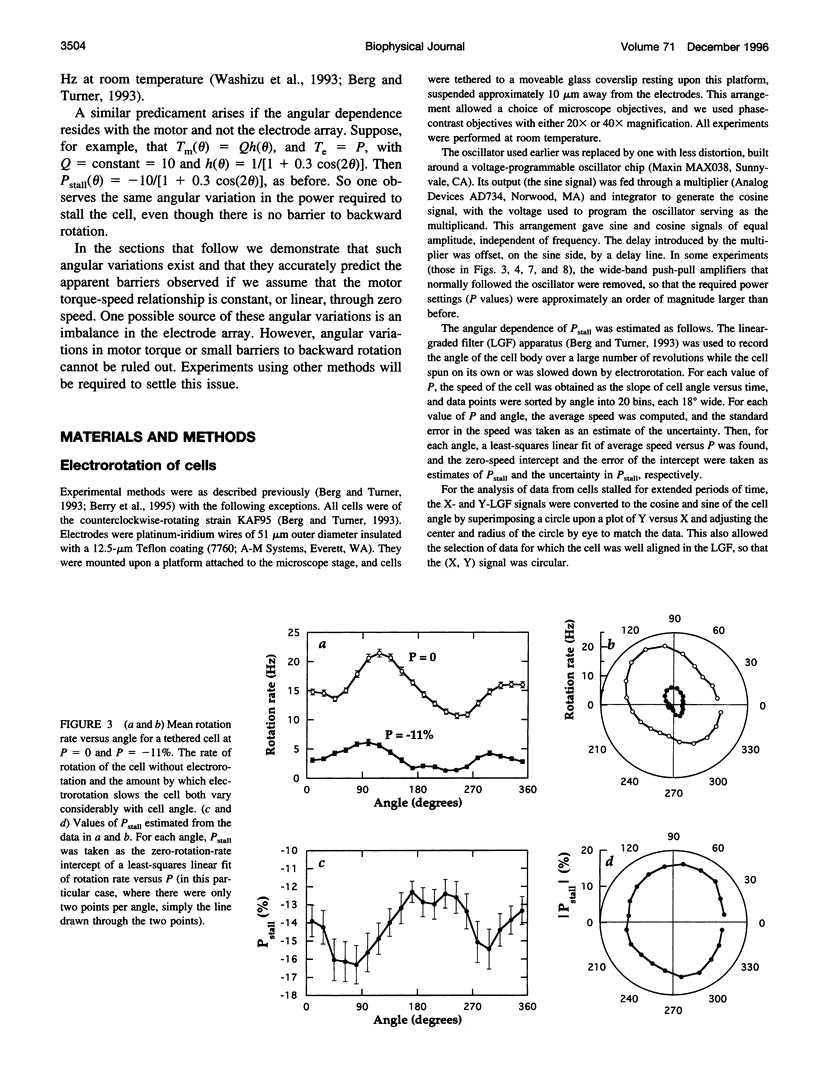
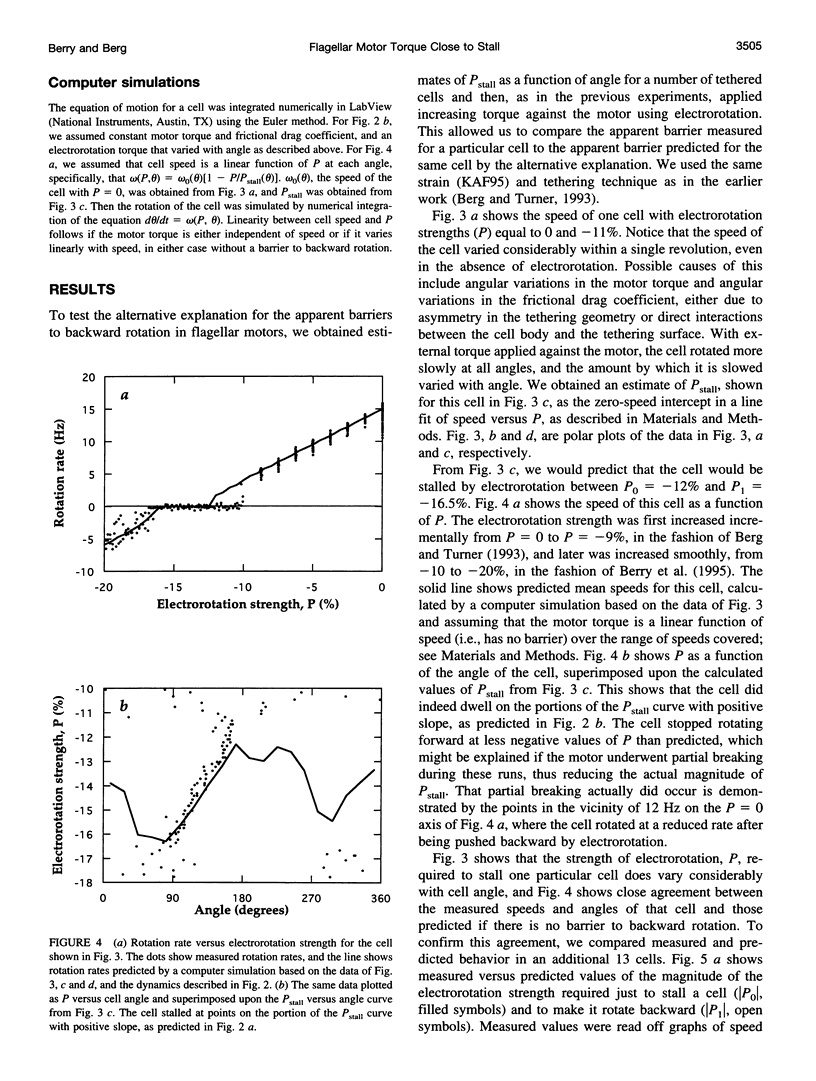
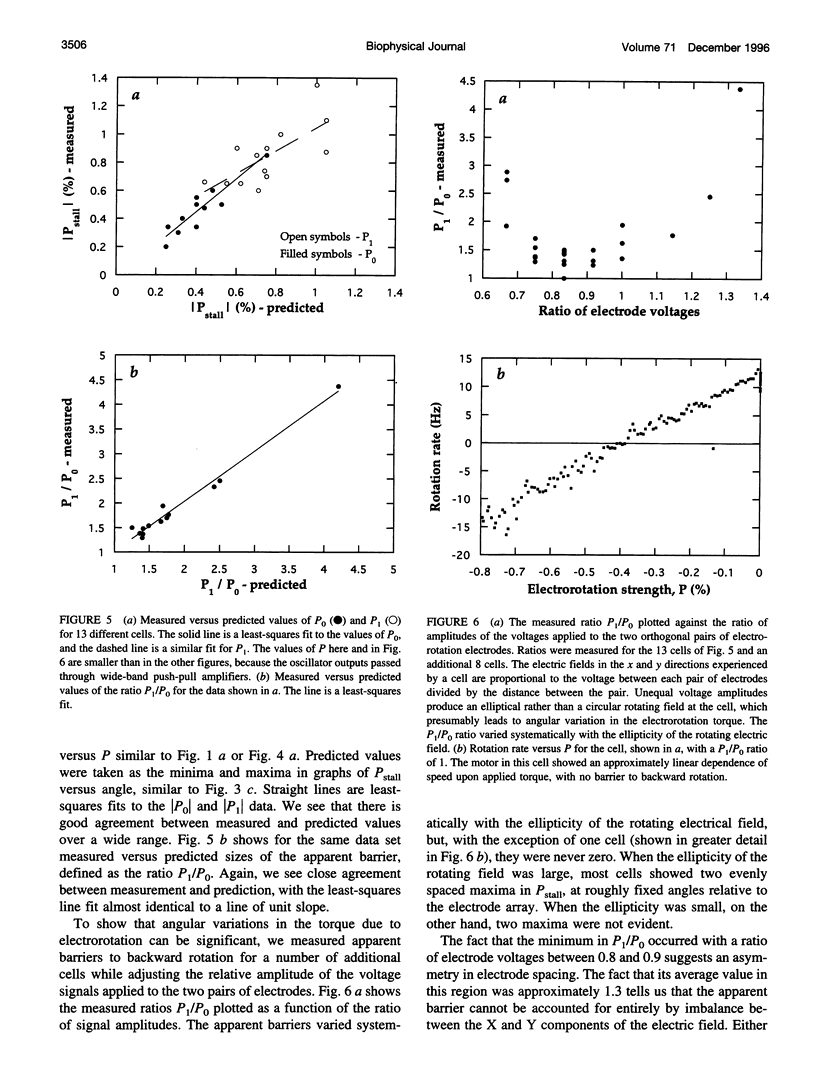
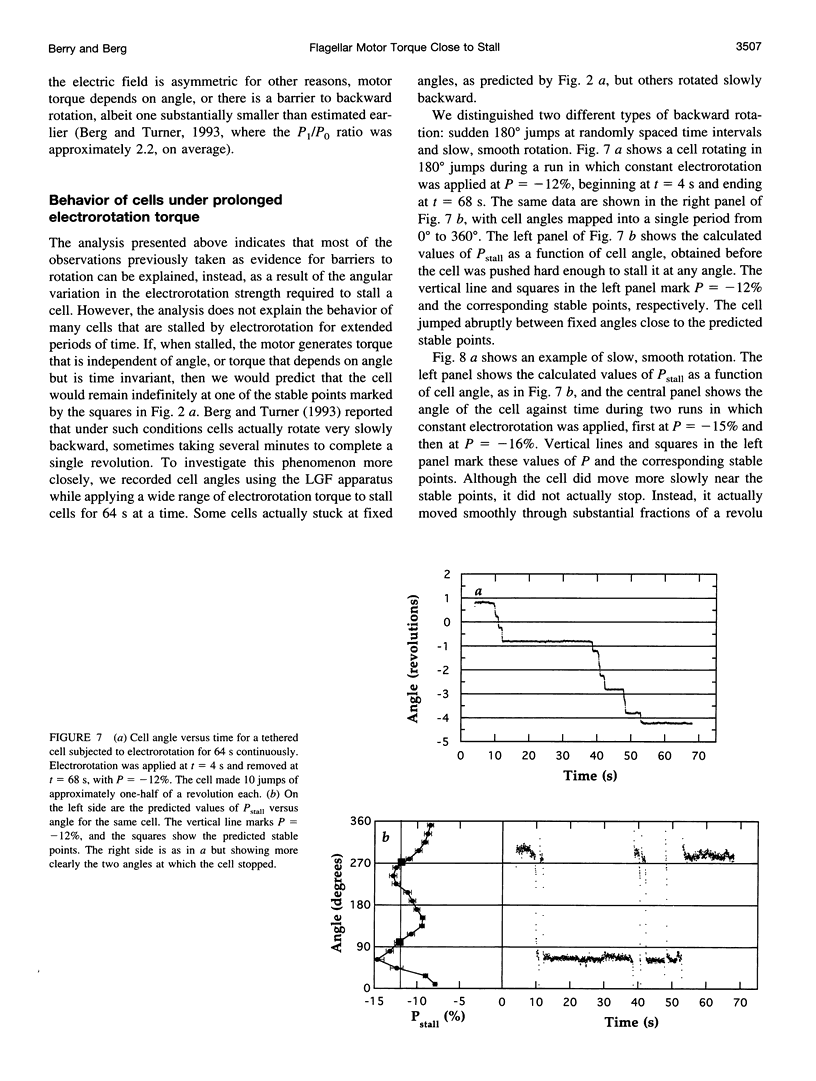
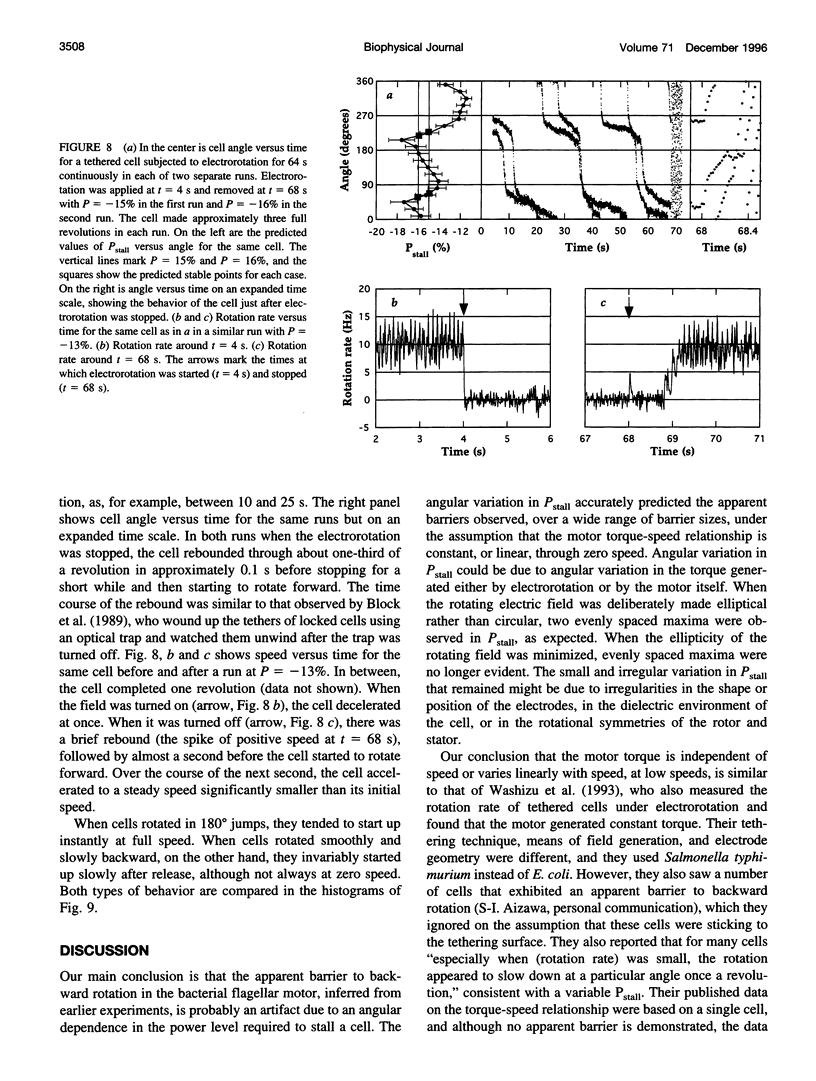
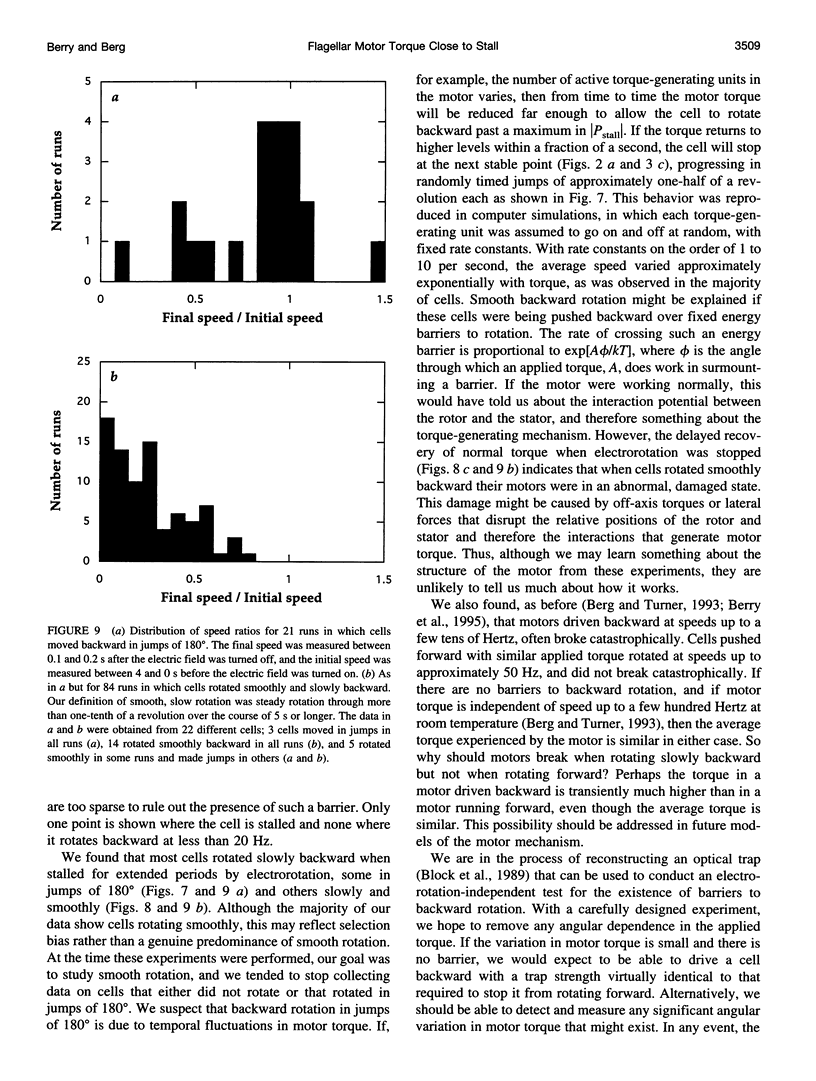
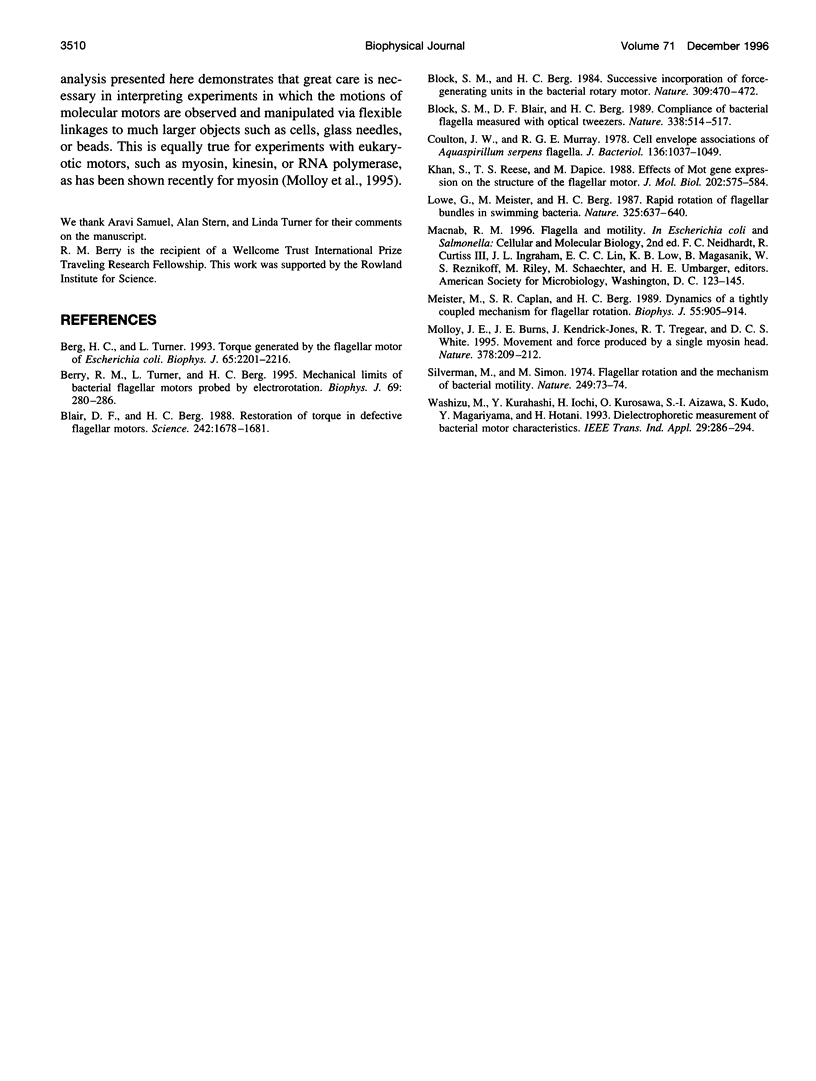
Selected References
These references are in PubMed. This may not be the complete list of references from this article.
- Berg H. C., Turner L. Torque generated by the flagellar motor of Escherichia coli. Biophys J. 1993 Nov;65(5):2201–2216. doi: 10.1016/S0006-3495(93)81278-5. [DOI] [PMC free article] [PubMed] [Google Scholar]
- Berry R. M., Turner L., Berg H. C. Mechanical limits of bacterial flagellar motors probed by electrorotation. Biophys J. 1995 Jul;69(1):280–286. doi: 10.1016/S0006-3495(95)79900-3. [DOI] [PMC free article] [PubMed] [Google Scholar]
- Blair D. F., Berg H. C. Restoration of torque in defective flagellar motors. Science. 1988 Dec 23;242(4886):1678–1681. doi: 10.1126/science.2849208. [DOI] [PubMed] [Google Scholar]
- Block S. M., Berg H. C. Successive incorporation of force-generating units in the bacterial rotary motor. 1984 May 31-Jun 6Nature. 309(5967):470–472. doi: 10.1038/309470a0. [DOI] [PubMed] [Google Scholar]
- Block S. M., Blair D. F., Berg H. C. Compliance of bacterial flagella measured with optical tweezers. Nature. 1989 Apr 6;338(6215):514–518. doi: 10.1038/338514a0. [DOI] [PubMed] [Google Scholar]
- Coulton J. W., Murray R. G. Cell envelope associations of Aquaspirillum serpens flagella. J Bacteriol. 1978 Dec;136(3):1037–1049. doi: 10.1128/jb.136.3.1037-1049.1978. [DOI] [PMC free article] [PubMed] [Google Scholar]
- Khan S., Dapice M., Reese T. S. Effects of mot gene expression on the structure of the flagellar motor. J Mol Biol. 1988 Aug 5;202(3):575–584. doi: 10.1016/0022-2836(88)90287-2. [DOI] [PubMed] [Google Scholar]
- Meister M., Caplan S. R., Berg H. C. Dynamics of a tightly coupled mechanism for flagellar rotation. Bacterial motility, chemiosmotic coupling, protonmotive force. Biophys J. 1989 May;55(5):905–914. doi: 10.1016/S0006-3495(89)82889-9. [DOI] [PMC free article] [PubMed] [Google Scholar]
- Molloy J. E., Burns J. E., Kendrick-Jones J., Tregear R. T., White D. C. Movement and force produced by a single myosin head. Nature. 1995 Nov 9;378(6553):209–212. doi: 10.1038/378209a0. [DOI] [PubMed] [Google Scholar]
- Silverman M., Simon M. Flagellar rotation and the mechanism of bacterial motility. Nature. 1974 May 3;249(452):73–74. doi: 10.1038/249073a0. [DOI] [PubMed] [Google Scholar]