Abstract
OBJECTIVE: The authors determined the effects of growth inhibition on glutamine transport and metabolism in human hepatoma cells. SUMMARY BACKGROUND DATA: Hepatoma cells exhibit markedly higher (10- to 30-fold) glutamine uptake than normal human hepatocytes, via a disparate transporter protein with a higher affinity for glutamine. Currently, little is known about the effects of growth arrest on glutamine transport and metabolism in hepatoma cells. METHODS: The authors determined proliferation rates, glutamine transport, and glutaminase activities in the human hepatoma cell lines HepG2, Huh-7, and SK-Hep, both in the presence and absence of the chemotherapeutic agents novobiocin and sodium butyrate. The transport activities for alanine, arginine, and leucine also were determined in both treated and untreated cells. Glutaminase activity was determined in normal human liver tissue and compared with that present in hepatoma cells. RESULTS: Glutaminase activities were similar in all three cell lines studied, despite differences in proliferation rates, and were sixfold higher than the activity in normal human liver. In contrast to normal hepatocytes, which expressed the liver-specific glutaminase, hepatomas expressed the kidney-type isoform. Sodium butyrate (1 mmol/L) and novobiocin (0.1 mmol/L) inhibited cellular proliferation and reduced both glutamine transport and glutaminase activity by more than 50% after 48 hours in the faster-growing, less differentiated SK-Hep cells. In contrast, the agents required 72 hours to attenuate glutamine uptake by 30% and 50% in the slower-growing, more differentiated HepG2 and Huh-7 cell lines, respectively. Treatment of all three cell lines with novobiocin/butyrate also resulted in a 30% to 60% attenuation of the transport of alanine, arginine, and leucine, and glutamine, indicating that inhibition of cellular proliferation similarly affects disparate amino acid transporters. CONCLUSIONS: Hepatocellular transformation is characterized by a marked increase in glutamine transport and metabolism. Inhibition of cellular proliferation attenuates glutamine transport and metabolism, especially in fast-growing, relatively undifferentiated hepatoma cells. Because the uptake of other amino acids is similarly reduced under cytostatic conditions, plasma membrane amino acid transport activity in hepatoma cells is regulated by the proliferation state of the cells.
Full text
PDF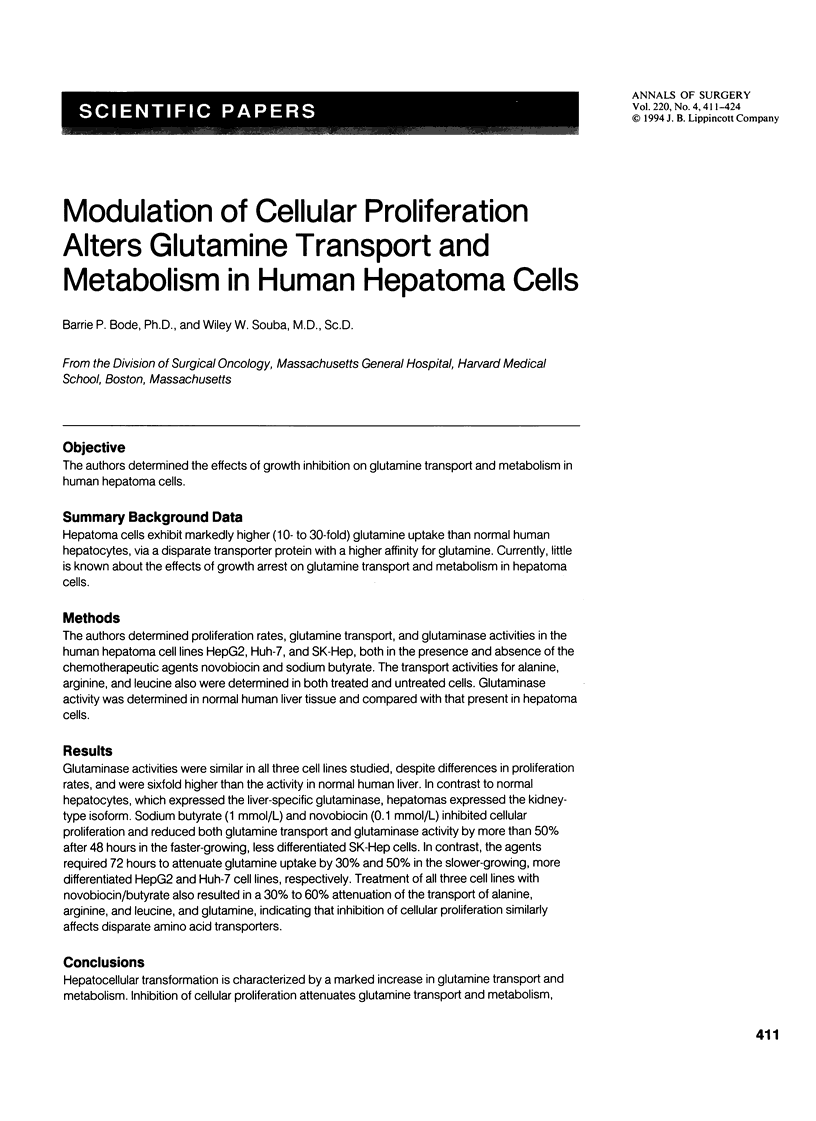
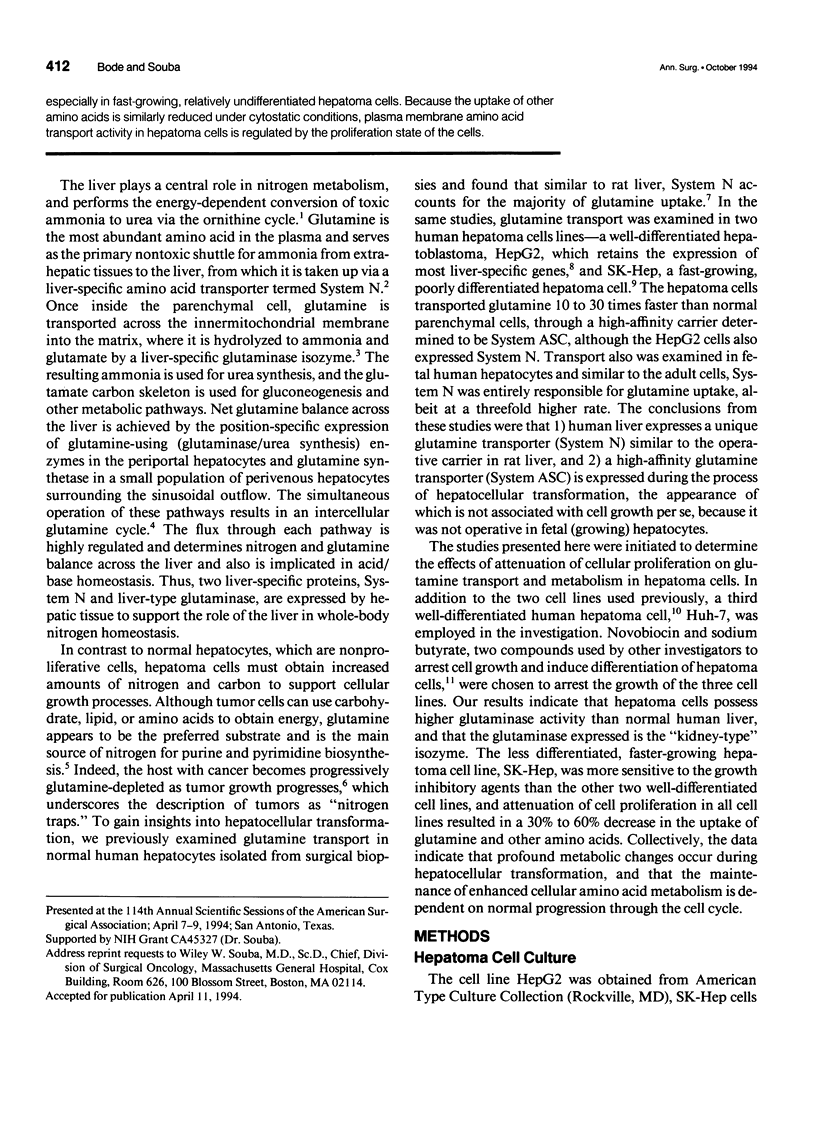
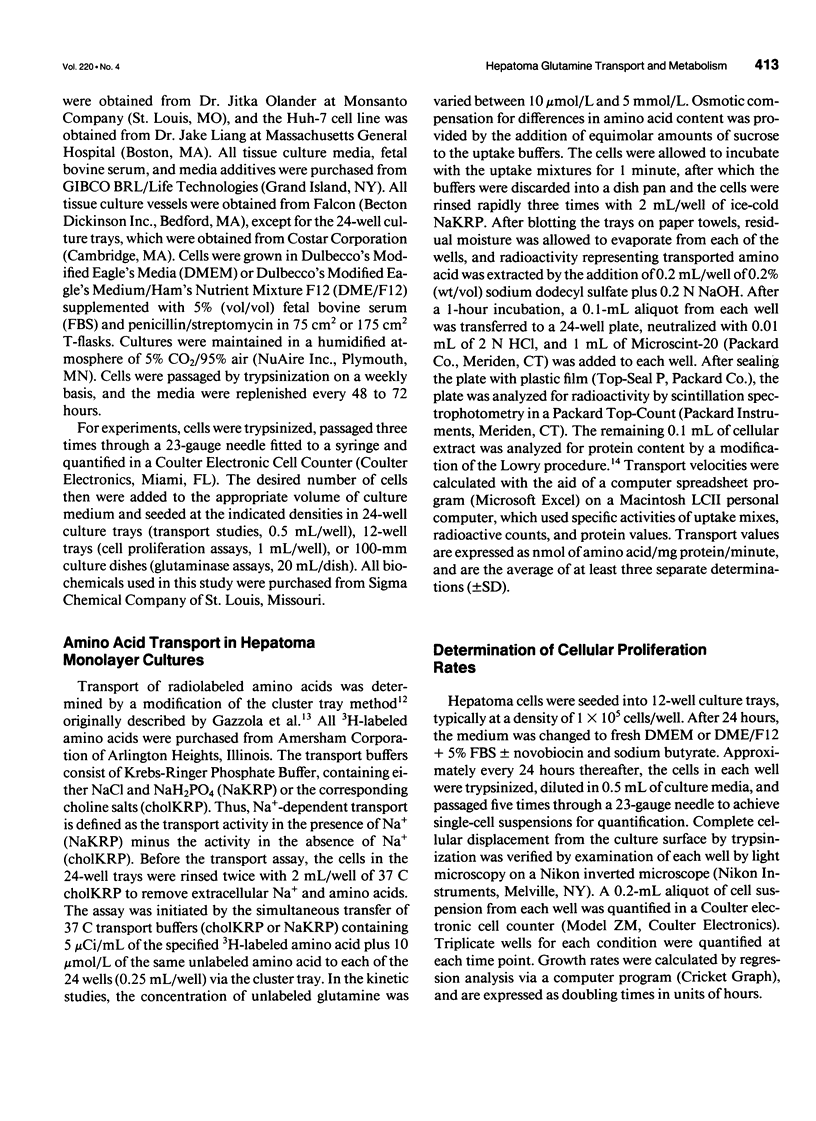
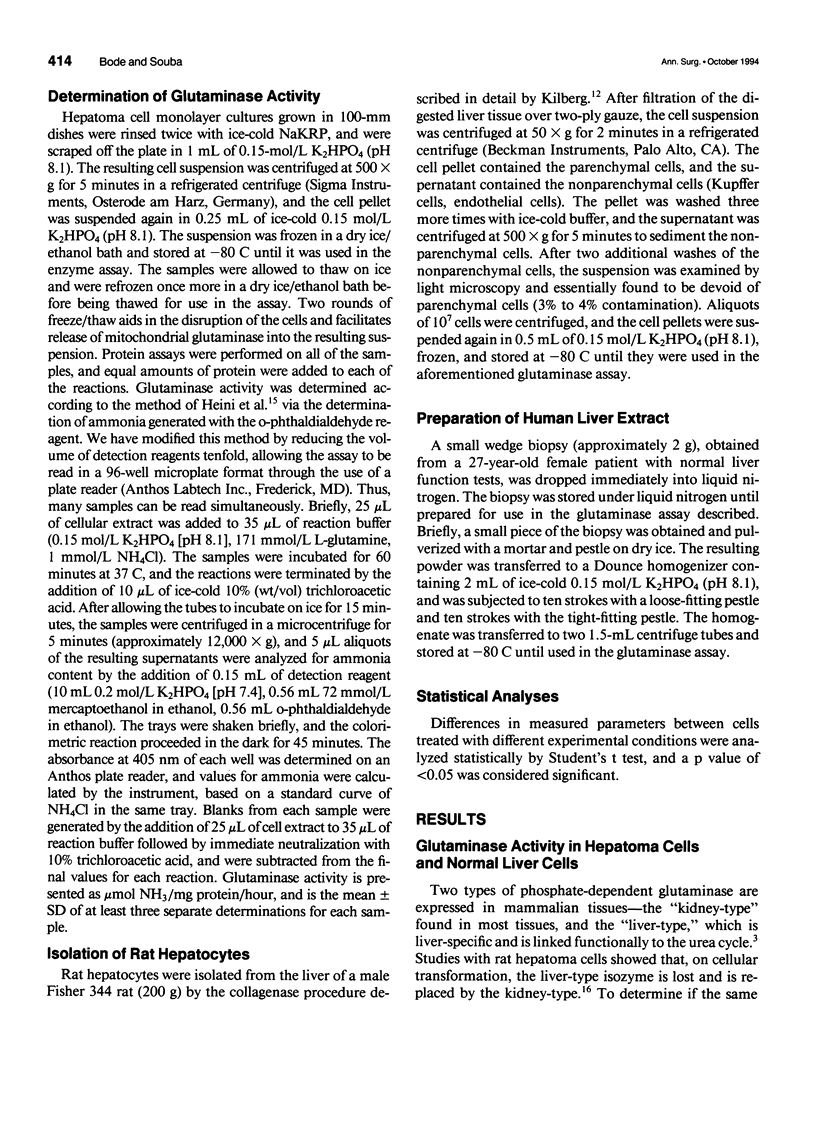
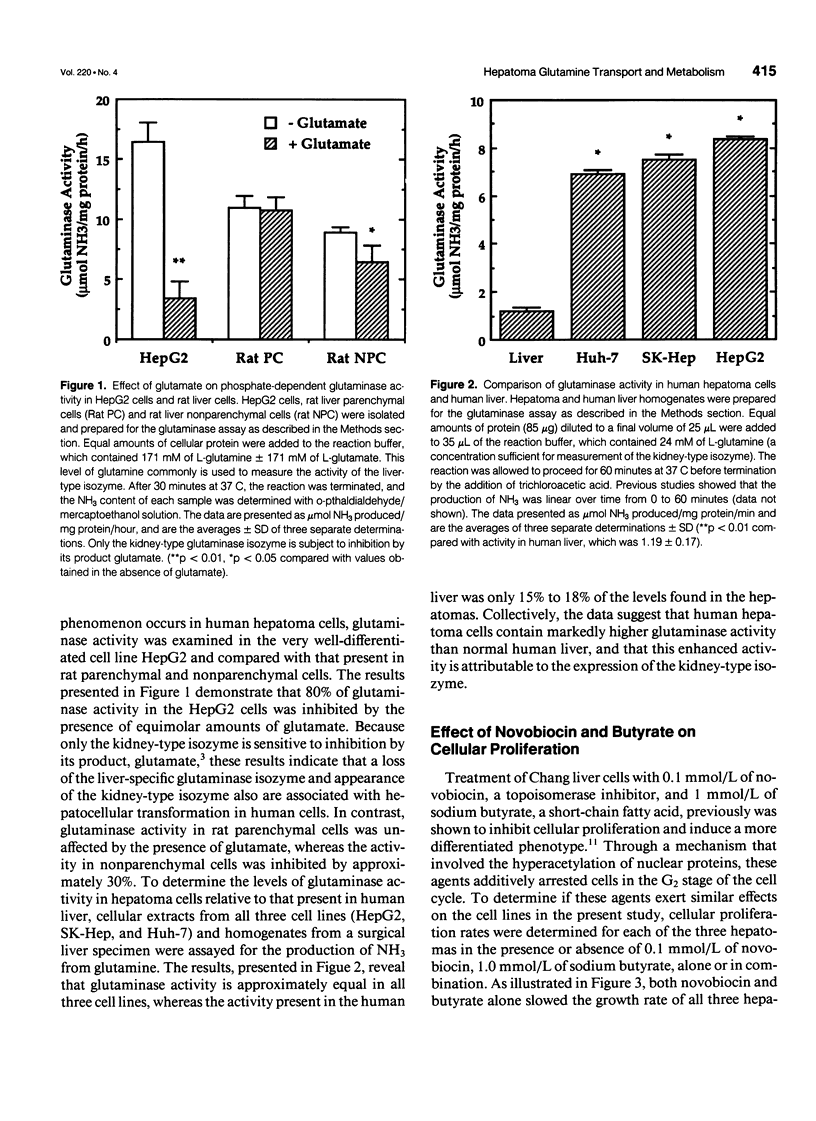
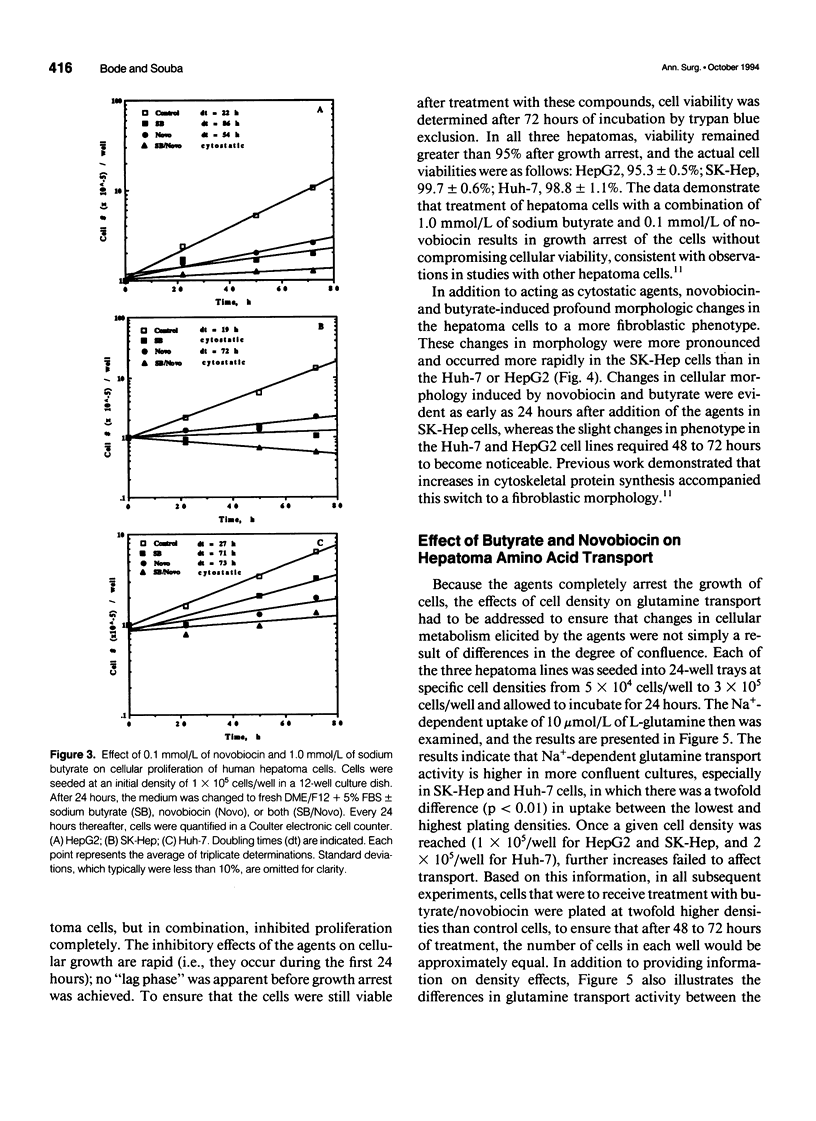
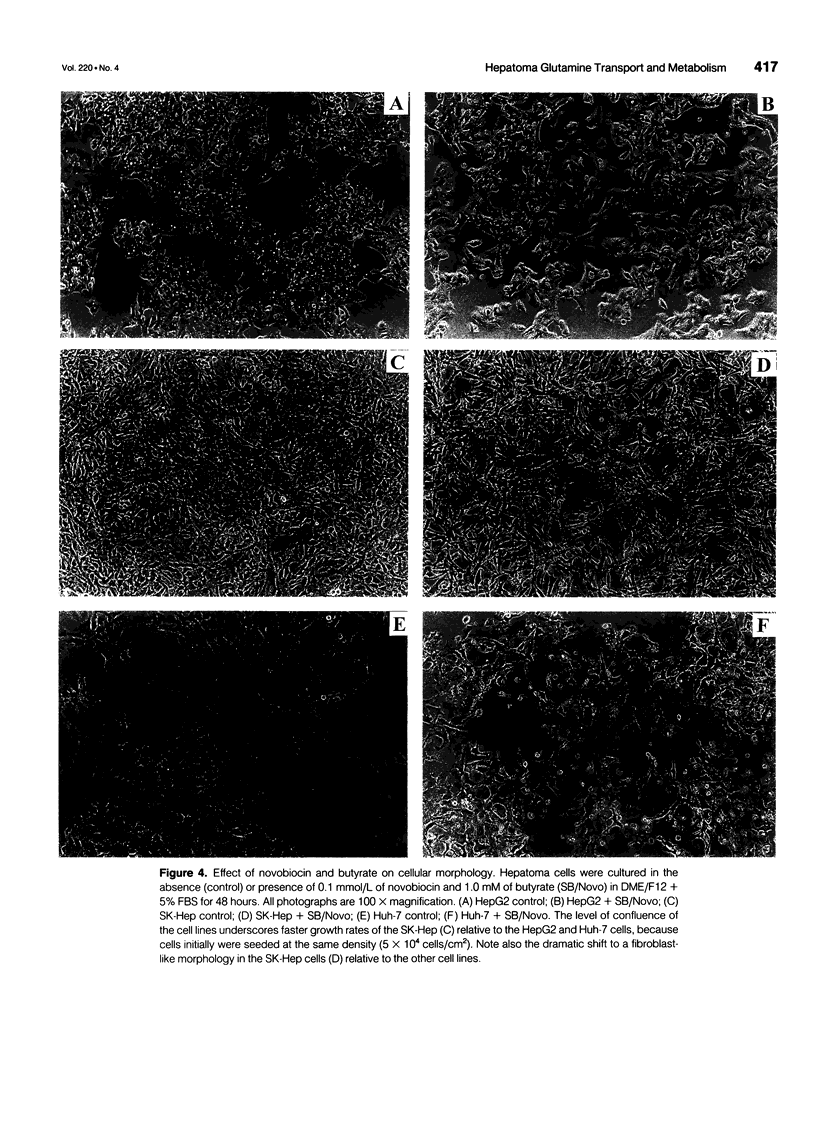
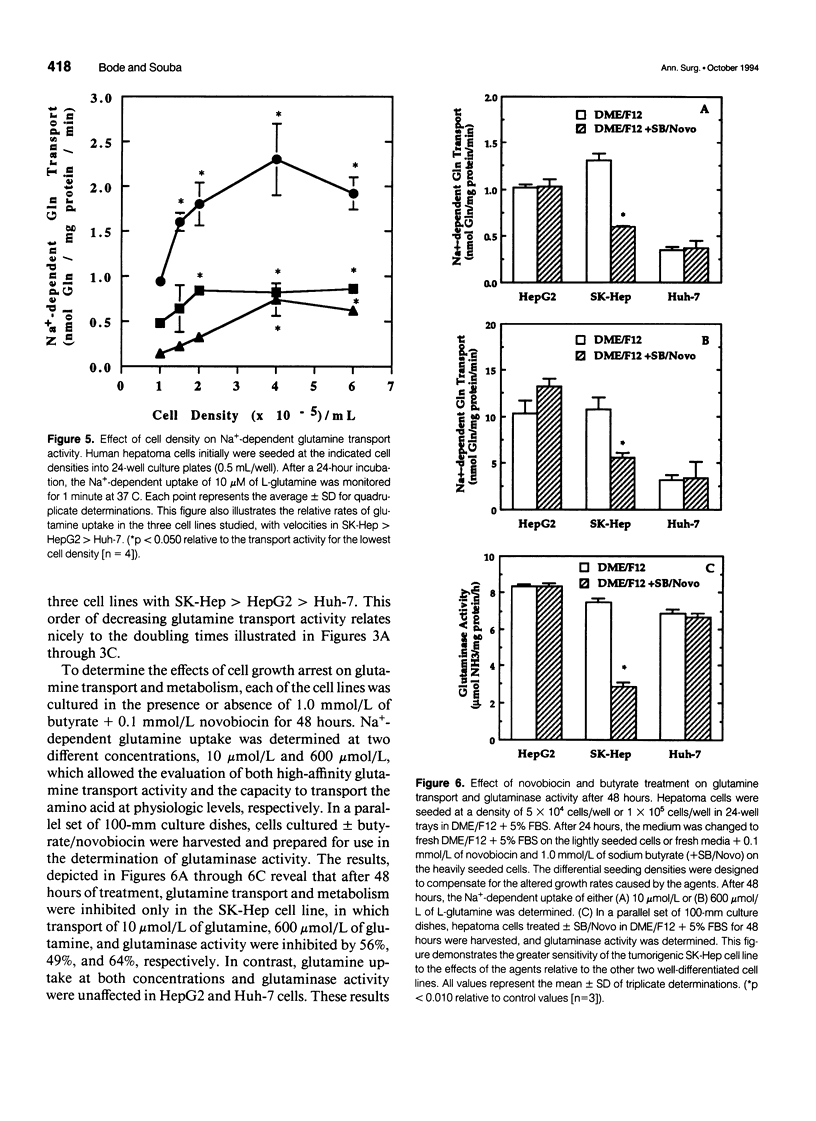
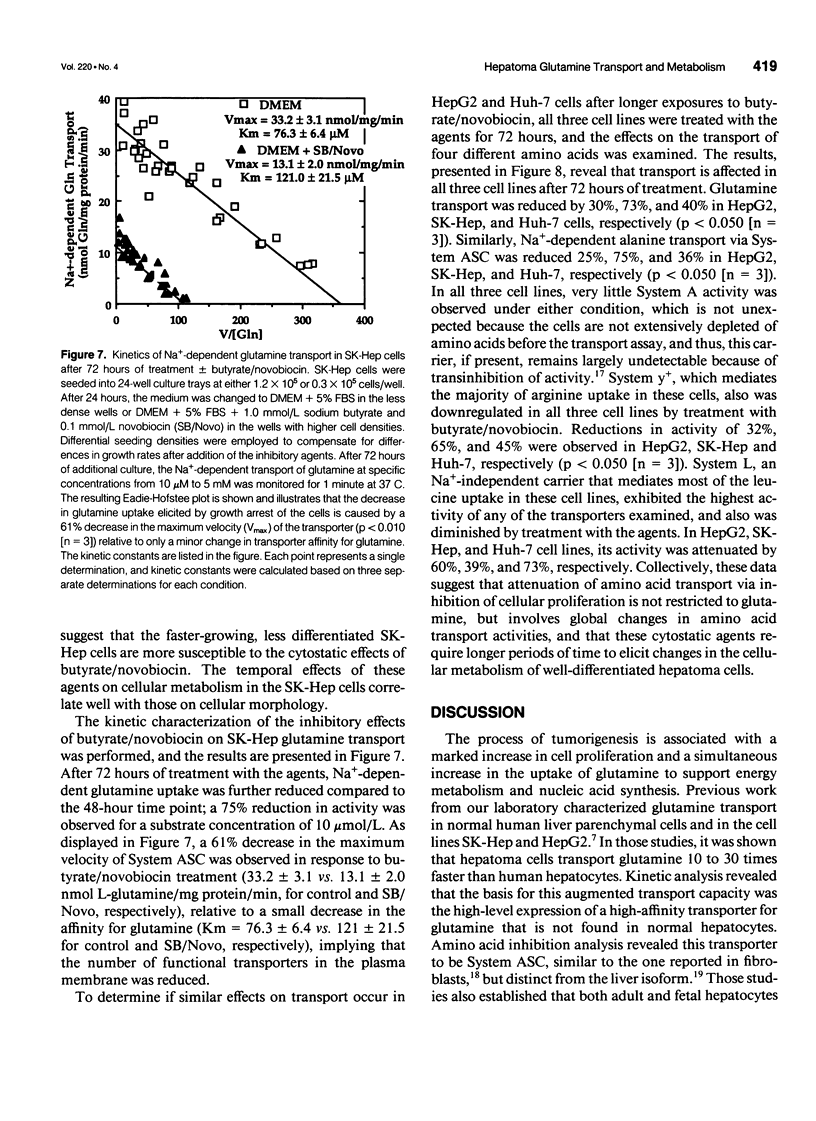
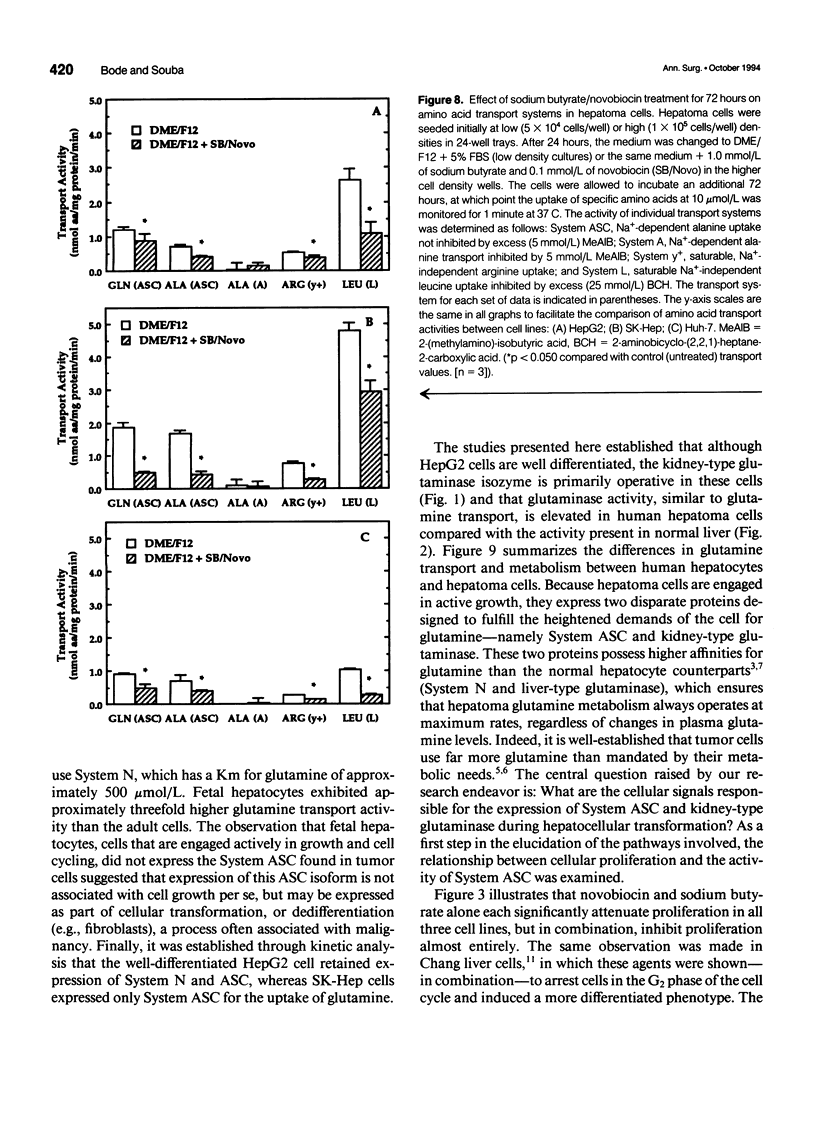
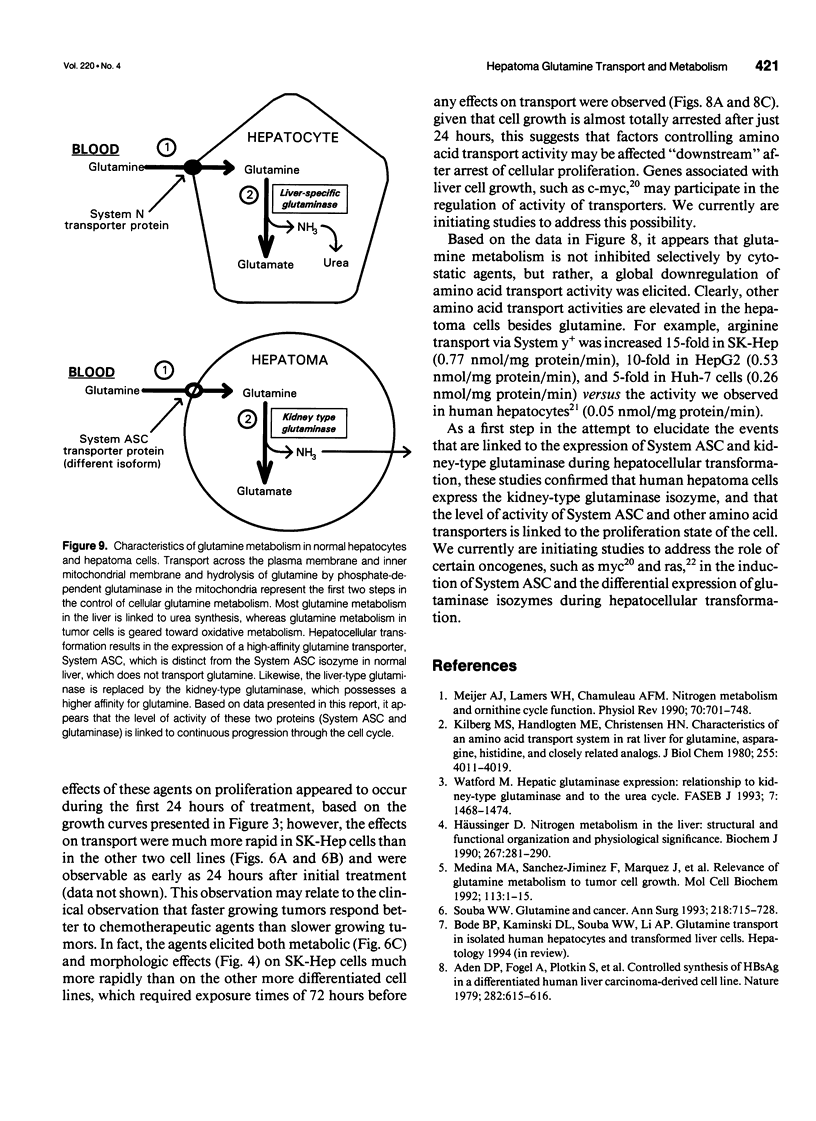
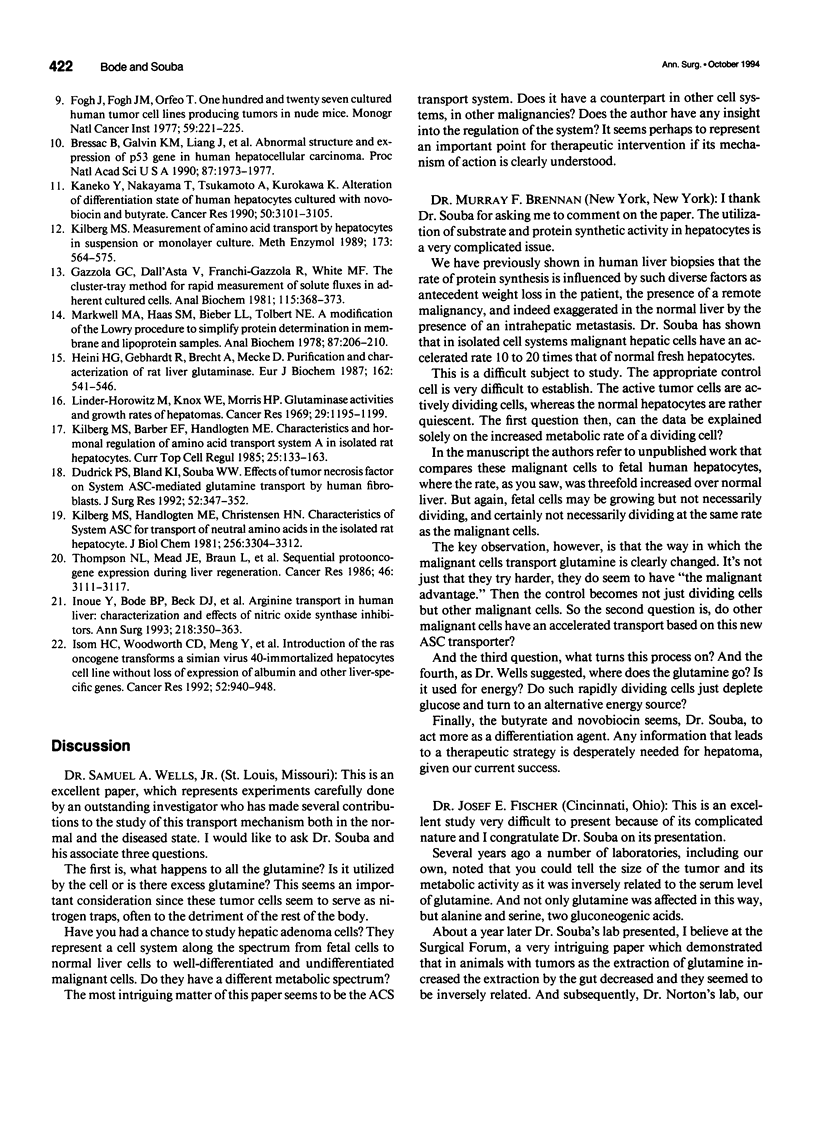
Images in this article
Selected References
These references are in PubMed. This may not be the complete list of references from this article.
- Aden D. P., Fogel A., Plotkin S., Damjanov I., Knowles B. B. Controlled synthesis of HBsAg in a differentiated human liver carcinoma-derived cell line. Nature. 1979 Dec 6;282(5739):615–616. doi: 10.1038/282615a0. [DOI] [PubMed] [Google Scholar]
- Bressac B., Galvin K. M., Liang T. J., Isselbacher K. J., Wands J. R., Ozturk M. Abnormal structure and expression of p53 gene in human hepatocellular carcinoma. Proc Natl Acad Sci U S A. 1990 Mar;87(5):1973–1977. doi: 10.1073/pnas.87.5.1973. [DOI] [PMC free article] [PubMed] [Google Scholar]
- Dudrick P. S., Bland K. I., Souba W. W. Effects of tumor necrosis factor on system ASC-mediated glutamine transport by human fibroblasts. J Surg Res. 1992 Apr;52(4):347–352. doi: 10.1016/0022-4804(92)90114-f. [DOI] [PubMed] [Google Scholar]
- Fogh J., Fogh J. M., Orfeo T. One hundred and twenty-seven cultured human tumor cell lines producing tumors in nude mice. J Natl Cancer Inst. 1977 Jul;59(1):221–226. doi: 10.1093/jnci/59.1.221. [DOI] [PubMed] [Google Scholar]
- Gazzola G. C., Dall'Asta V., Franchi-Gazzola R., White M. F. The cluster-tray method for rapid measurement of solute fluxes in adherent cultured cells. Anal Biochem. 1981 Aug;115(2):368–374. doi: 10.1016/0003-2697(81)90019-1. [DOI] [PubMed] [Google Scholar]
- Haüssinger D. Nitrogen metabolism in liver: structural and functional organization and physiological relevance. Biochem J. 1990 Apr 15;267(2):281–290. doi: 10.1042/bj2670281. [DOI] [PMC free article] [PubMed] [Google Scholar]
- Heini H. G., Gebhardt R., Brecht A., Mecke D. Purification and characterization of rat liver glutaminase. Eur J Biochem. 1987 Feb 2;162(3):541–546. doi: 10.1111/j.1432-1033.1987.tb10673.x. [DOI] [PubMed] [Google Scholar]
- Inoue Y., Bode B. P., Beck D. J., Li A. P., Bland K. I., Souba W. W. Arginine transport in human liver. Characterization and effects of nitric oxide synthase inhibitors. Ann Surg. 1993 Sep;218(3):350–363. doi: 10.1097/00000658-199309000-00014. [DOI] [PMC free article] [PubMed] [Google Scholar]
- Isom H. C., Woodworth C. D., Meng Y., Kreider J., Miller T., Mengel L. Introduction of the ras oncogene transforms a simian virus 40-immortalized hepatocyte cell line without loss of expression of albumin and other liver-specific genes. Cancer Res. 1992 Feb 15;52(4):940–948. [PubMed] [Google Scholar]
- Kaneko Y., Nakayama T., Tsukamoto A., Kurokawa K. Alteration of differentiation state of human hepatocytes cultured with novobiocin and butyrate. Cancer Res. 1990 May 15;50(10):3101–3105. [PubMed] [Google Scholar]
- Kilberg M. S., Barber E. F., Handlogten M. E. Characteristics and hormonal regulation of amino acid transport system A in isolated rat hepatocytes. Curr Top Cell Regul. 1985;25:133–163. doi: 10.1016/b978-0-12-152825-6.50009-6. [DOI] [PubMed] [Google Scholar]
- Kilberg M. S., Handlogten M. E., Christensen H. N. Characteristics of an amino acid transport system in rat liver for glutamine, asparagine, histidine, and closely related analogs. J Biol Chem. 1980 May 10;255(9):4011–4019. [PubMed] [Google Scholar]
- Kilberg M. S., Handlogten M. E., Christensen H. N. Characteristics of system ASC for transport of neutral amino acids in the isolated rat hepatocyte. J Biol Chem. 1981 Apr 10;256(7):3304–3312. [PubMed] [Google Scholar]
- Kilberg M. S. Measurement of amino acid transport by hepatocytes in suspension or monolayer culture. Methods Enzymol. 1989;173:564–575. doi: 10.1016/s0076-6879(89)73039-1. [DOI] [PubMed] [Google Scholar]
- Linder-Horowitz M., Knox W. E., Morris H. P. Glutaminase activities and growth rates of rat hepatomas. Cancer Res. 1969 Jun;29(6):1195–1199. [PubMed] [Google Scholar]
- Markwell M. A., Haas S. M., Bieber L. L., Tolbert N. E. A modification of the Lowry procedure to simplify protein determination in membrane and lipoprotein samples. Anal Biochem. 1978 Jun 15;87(1):206–210. doi: 10.1016/0003-2697(78)90586-9. [DOI] [PubMed] [Google Scholar]
- Medina M. A., Sánchez-Jiménez F., Márquez J., Rodríguez Quesada A., Núez de Castro I. Relevance of glutamine metabolism to tumor cell growth. Mol Cell Biochem. 1992 Jul 6;113(1):1–15. doi: 10.1007/BF00230880. [DOI] [PubMed] [Google Scholar]
- Meijer A. J., Lamers W. H., Chamuleau R. A. Nitrogen metabolism and ornithine cycle function. Physiol Rev. 1990 Jul;70(3):701–748. doi: 10.1152/physrev.1990.70.3.701. [DOI] [PubMed] [Google Scholar]
- Souba W. W. Glutamine and cancer. Ann Surg. 1993 Dec;218(6):715–728. doi: 10.1097/00000658-199312000-00004. [DOI] [PMC free article] [PubMed] [Google Scholar]
- Thompson N. L., Mead J. E., Braun L., Goyette M., Shank P. R., Fausto N. Sequential protooncogene expression during rat liver regeneration. Cancer Res. 1986 Jun;46(6):3111–3117. [PubMed] [Google Scholar]
- Watford M. Hepatic glutaminase expression: relationship to kidney-type glutaminase and to the urea cycle. FASEB J. 1993 Dec;7(15):1468–1474. doi: 10.1096/fasebj.7.15.8262331. [DOI] [PubMed] [Google Scholar]