Abstract
Confocal laser fluorescence microscopy was used to study in real time under nearly physiological conditions the equilibration and exchange characteristics of several different fluorescently labeled molecules into chemically skinned, unfixed skeletal muscle fibers of rabbit psoas. The time required for equilibration was found to vary widely from a few minutes up to several days. Specific interactions of molecules with myofibrillar structures seem to slow down equilibration significantly. Time for equilibration, therefore, cannot simply be predicted from diffusion parameters in solution. Specific interactions resulted in characteristic labeling patterns for molecules like creatine kinase (muscle type), pyruvate kinase, actin-binding IgG, and others. For the very slowly equilibrating Rh-NEM-S1, changes in affinity upon binding to actin in the absence of calcium and subsequent slow cooperative activation, beginning at the free end of the filament at the H-zone, were observed. In the presence of calcium, however, binding of Rh-NEM-S1 was homogeneous along the whole actin filament from the very beginning of equilibration. The dissociation properties of the dynamic interactions were analyzed using a chase protocol. Even molecules that bind with rather high affinity and that can be removed only by applying extreme experimental conditions like Rh-phalloidine or Rh-troponin could be displaced easily by unlabeled homologous molecules.
Full text
PDF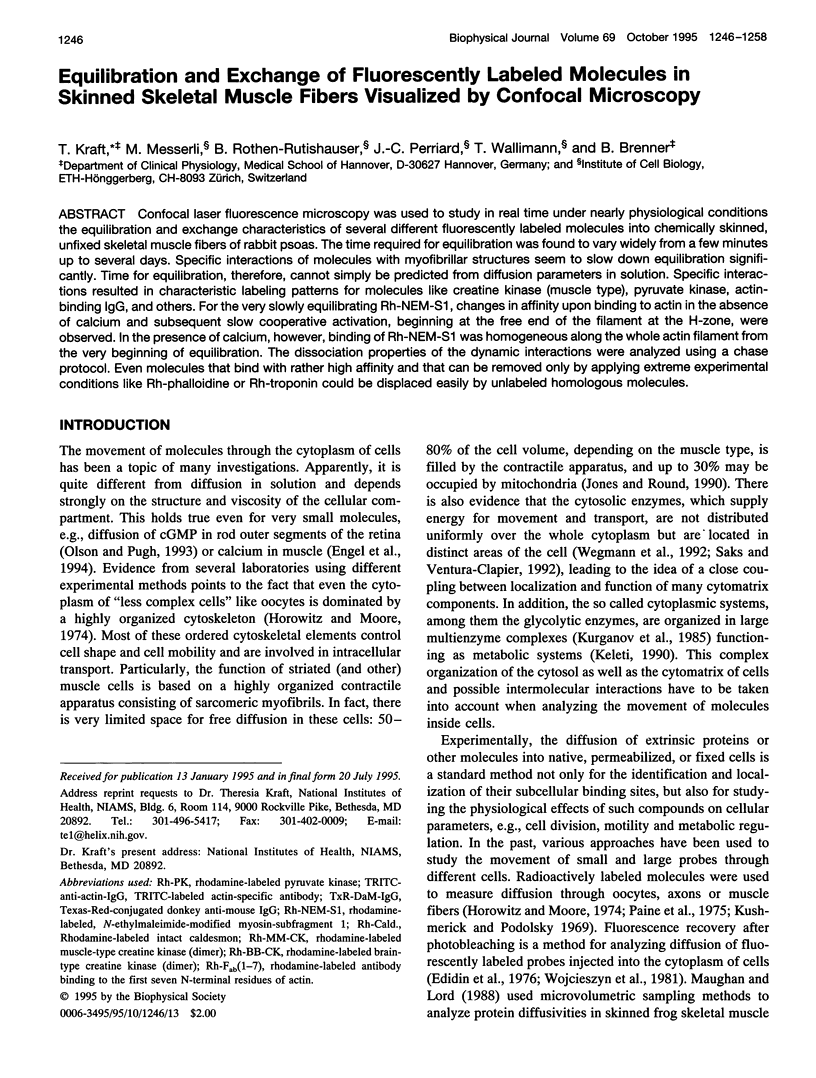
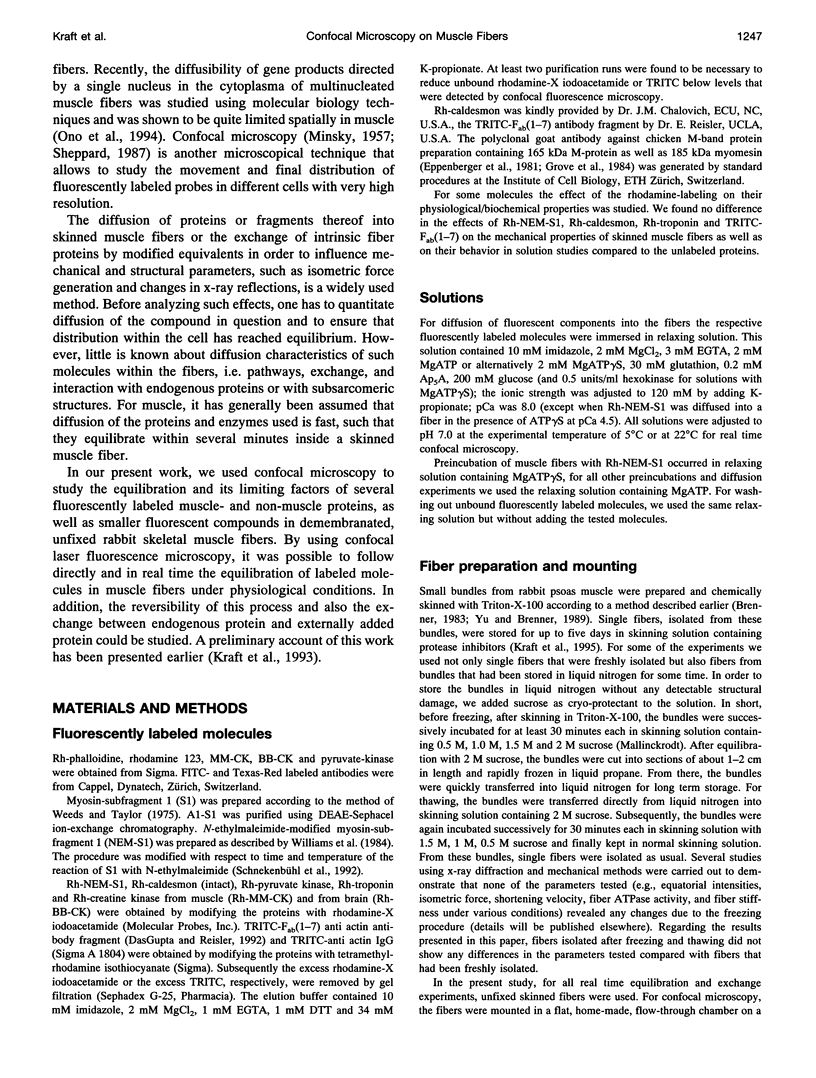
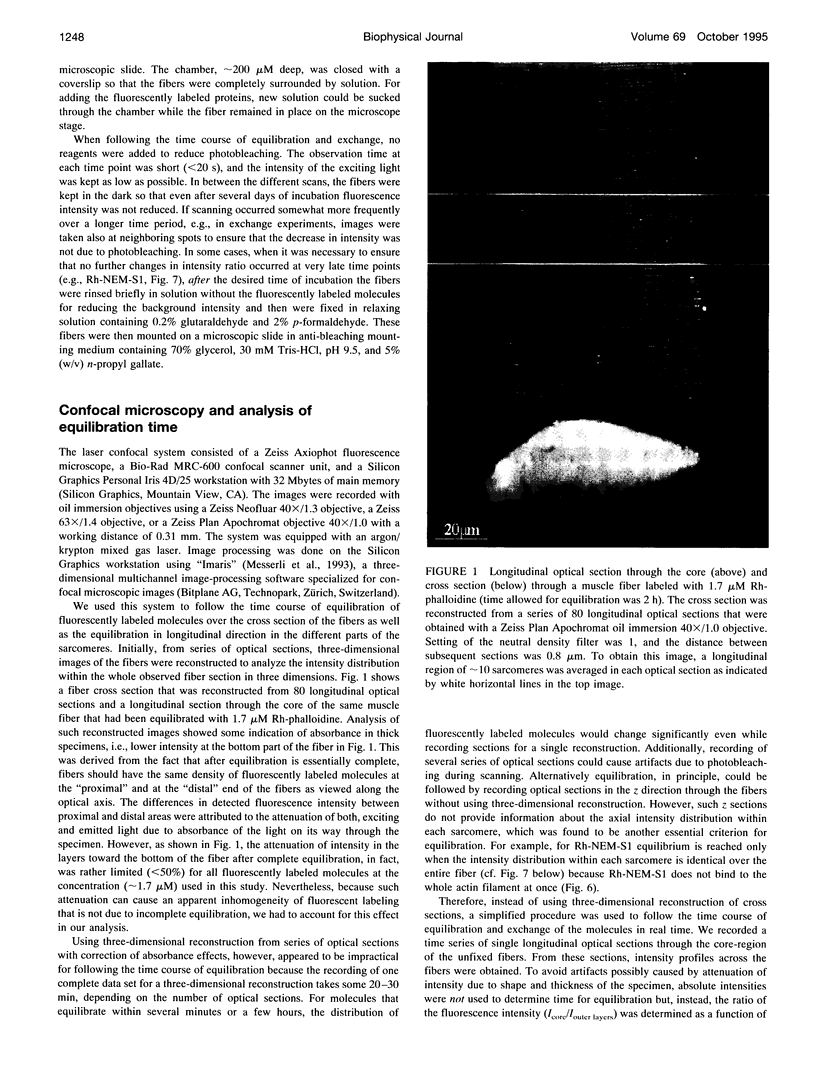
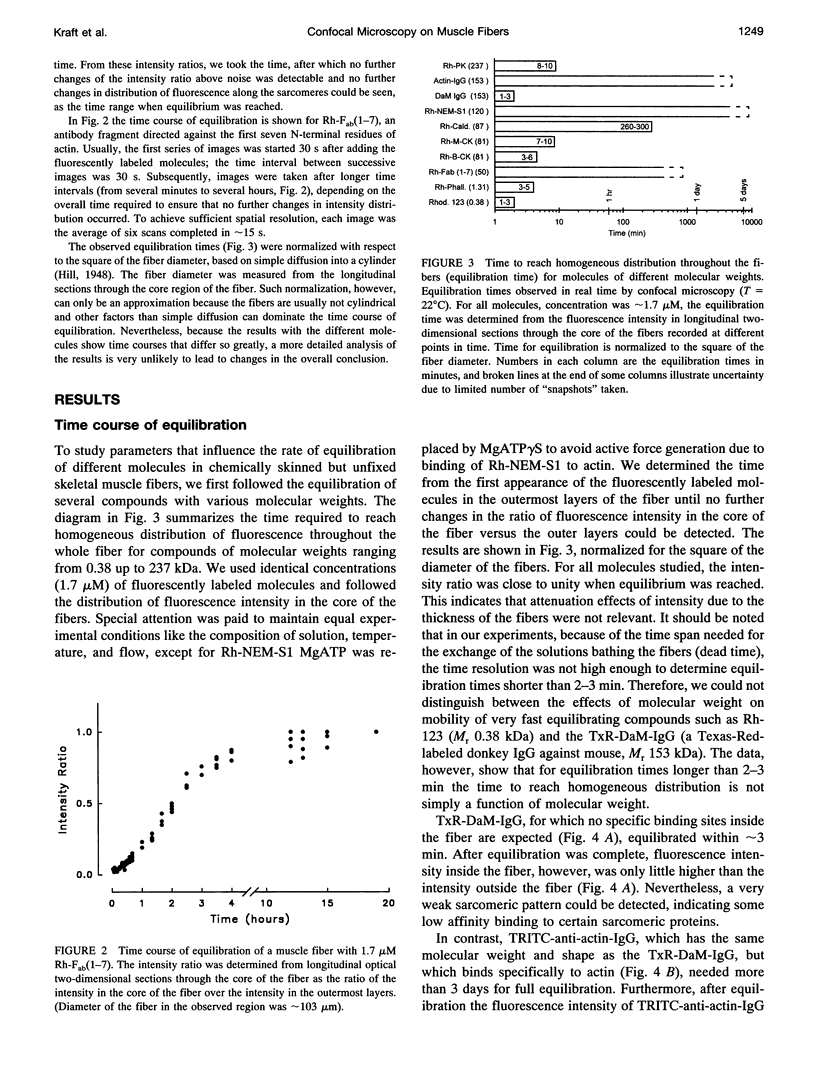
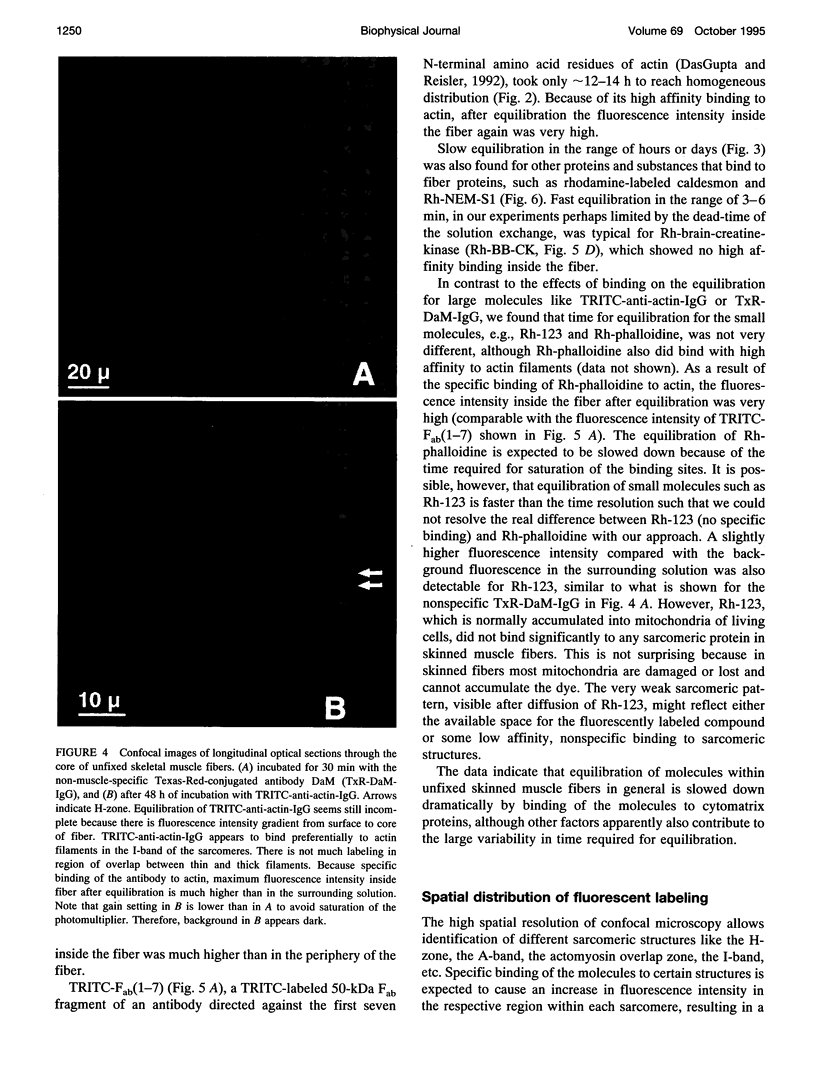
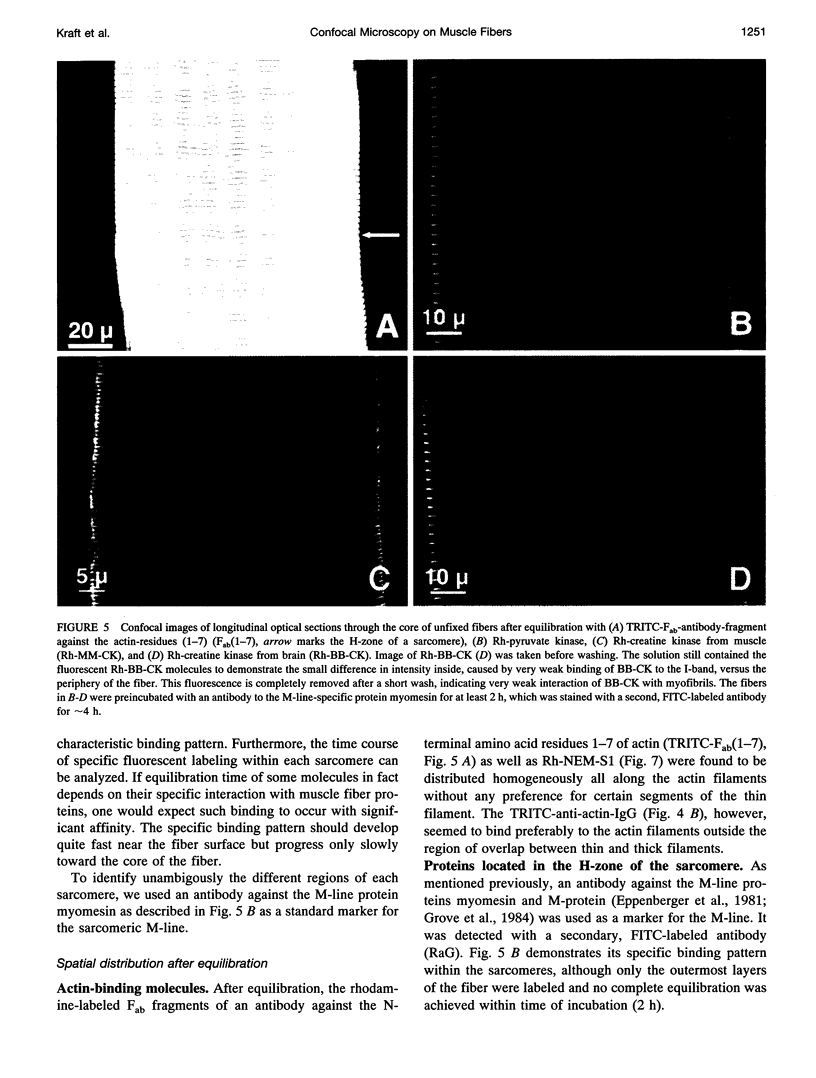
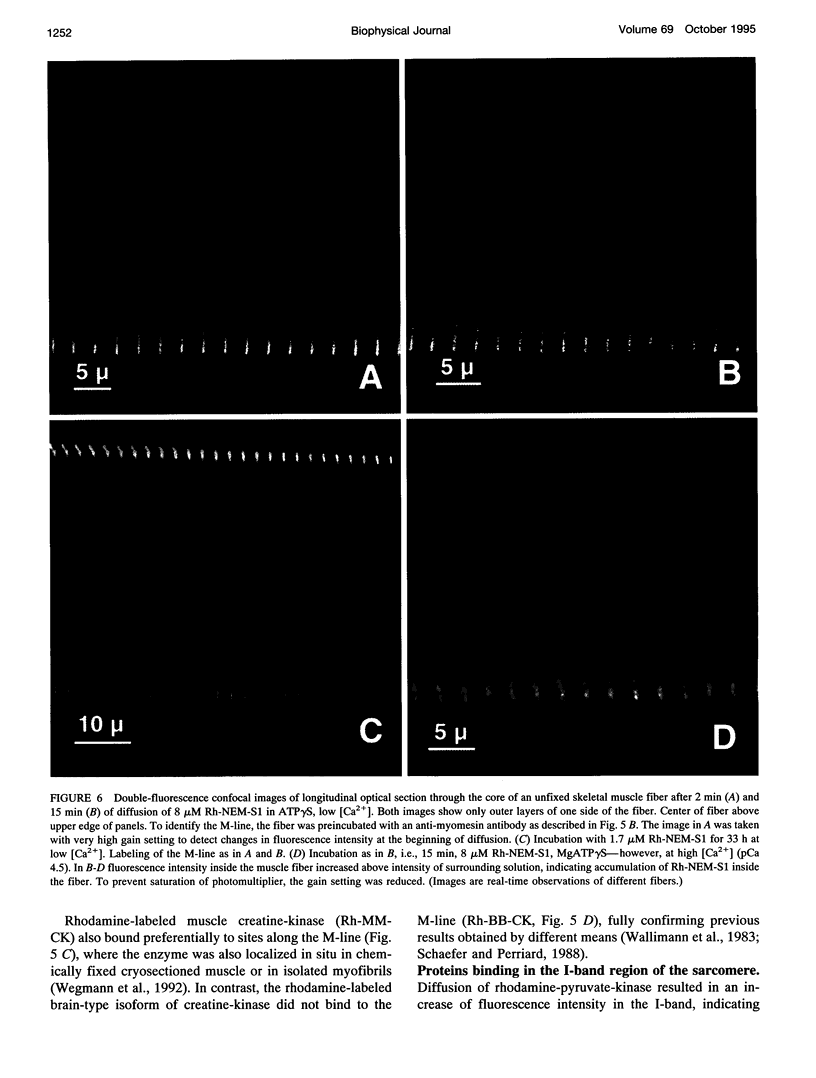
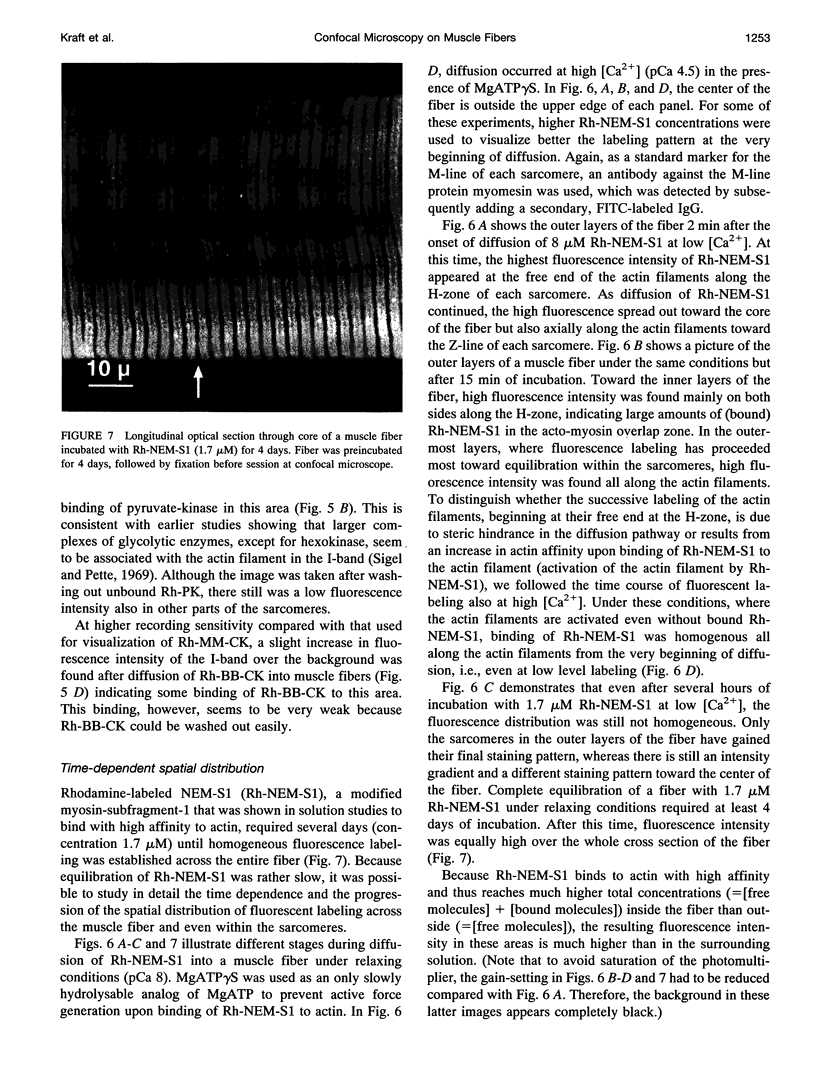
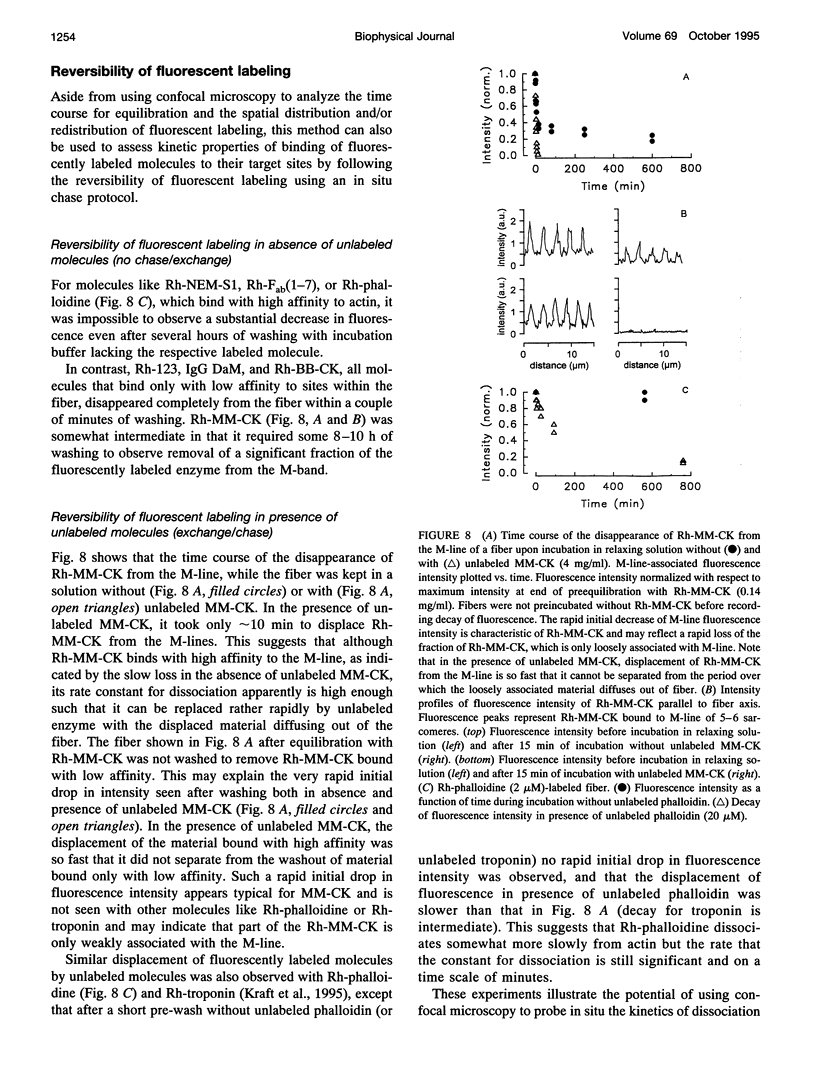
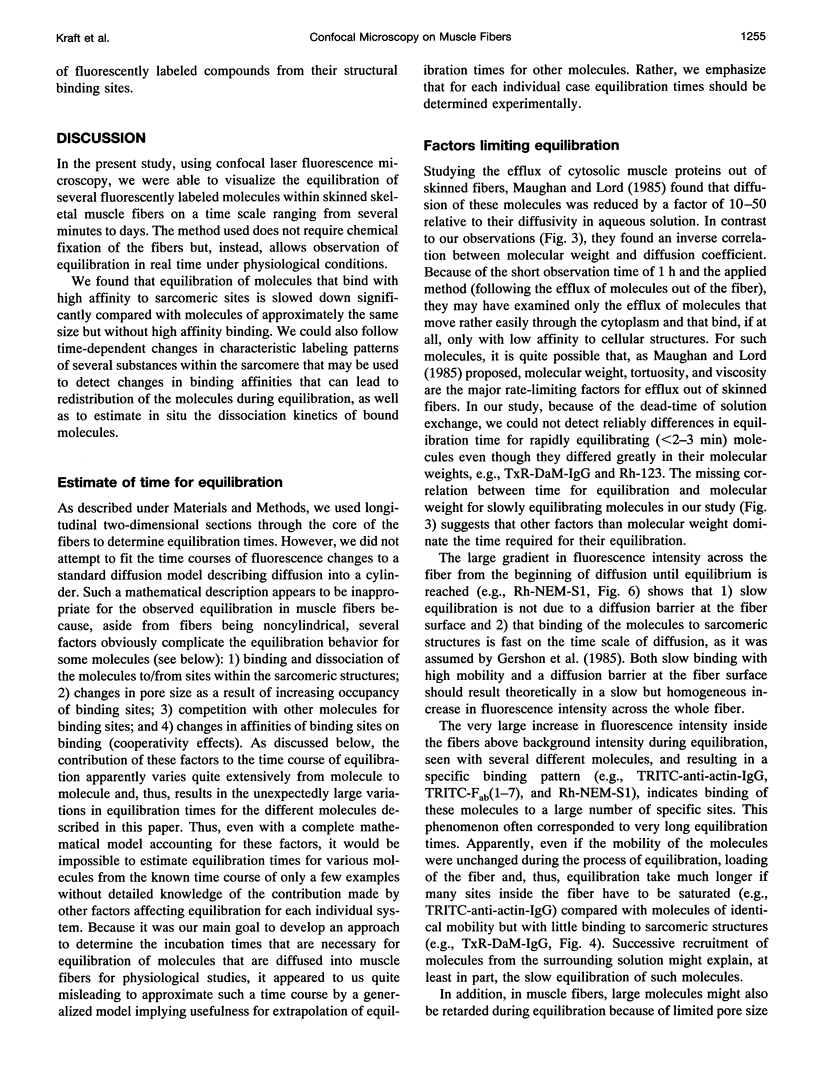
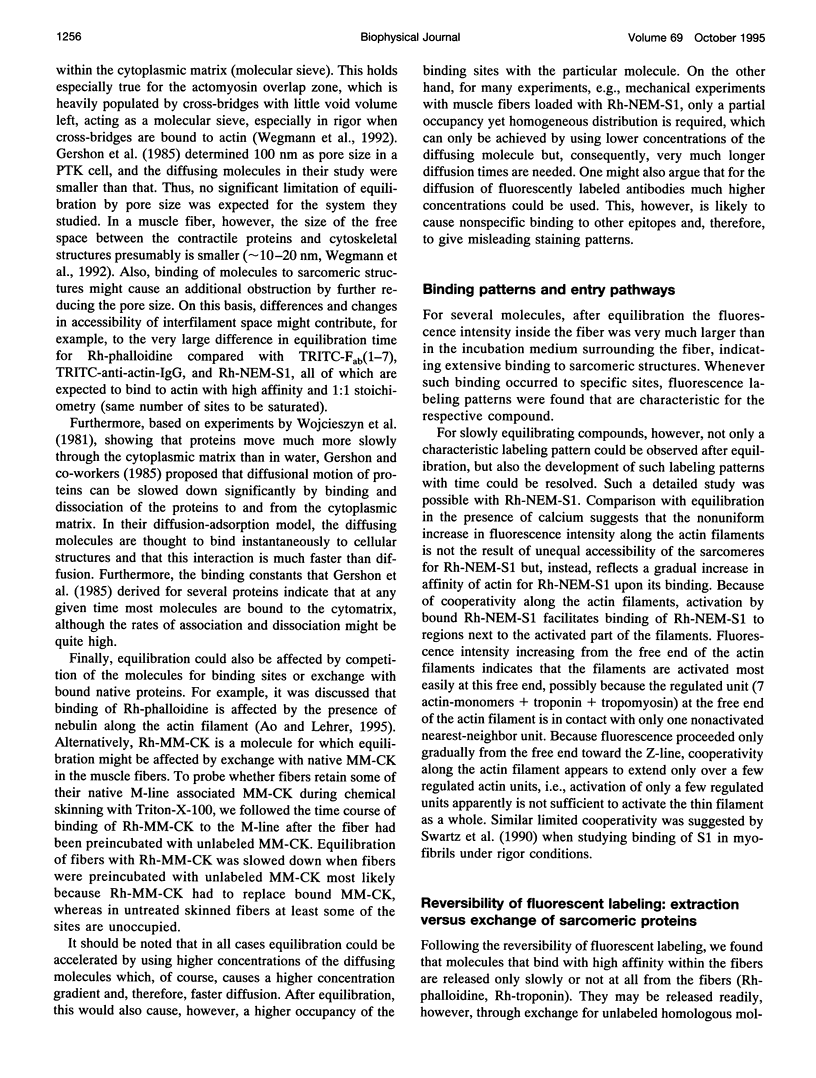
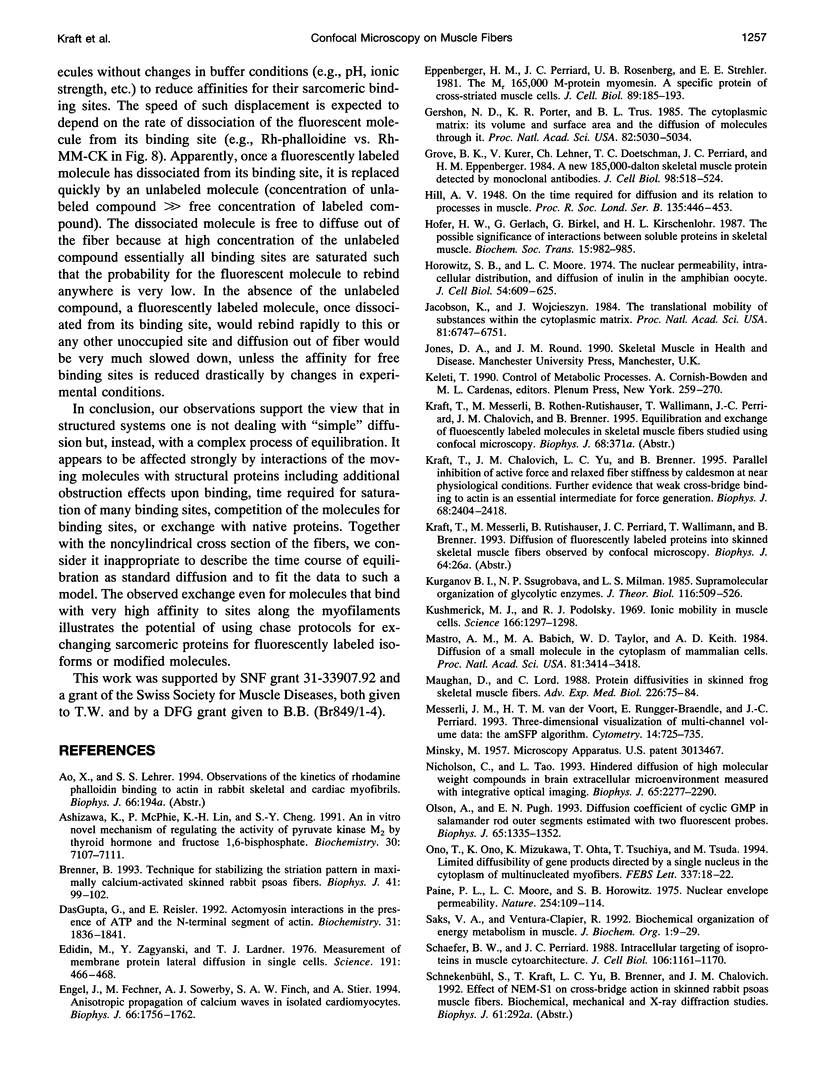
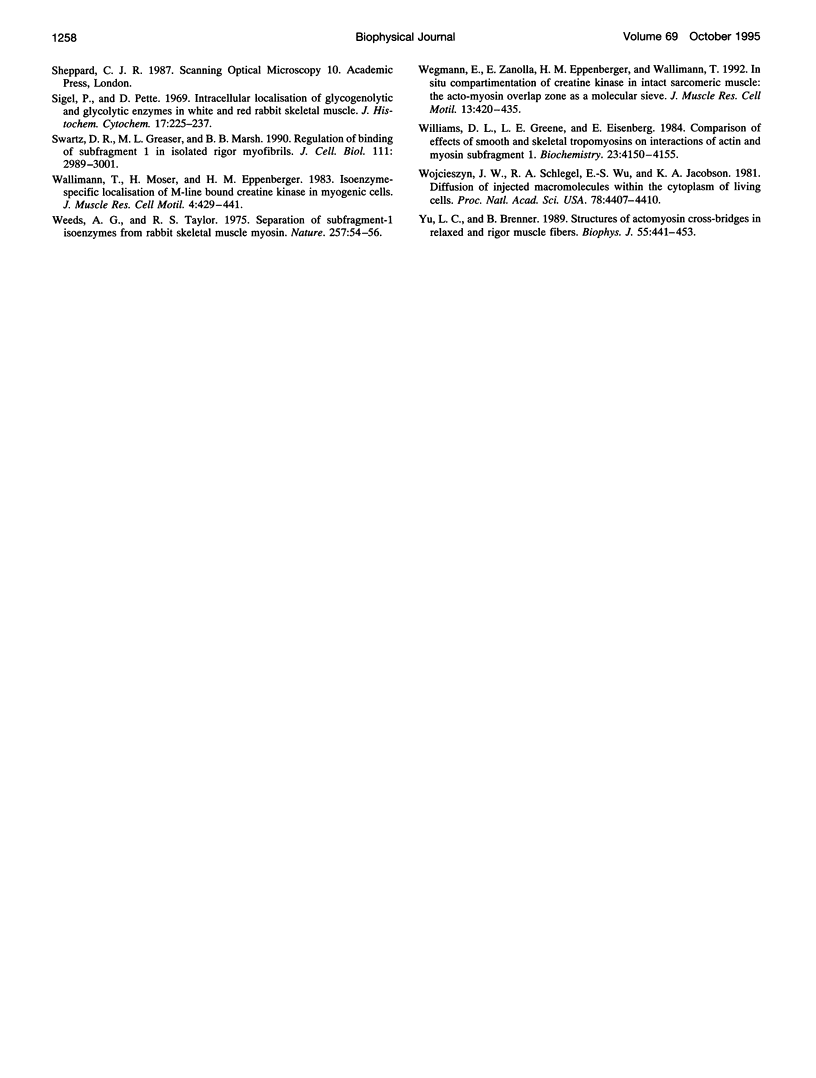
Images in this article
Selected References
These references are in PubMed. This may not be the complete list of references from this article.
- Ashizawa K., McPhie P., Lin K. H., Cheng S. Y. An in vitro novel mechanism of regulating the activity of pyruvate kinase M2 by thyroid hormone and fructose 1, 6-bisphosphate. Biochemistry. 1991 Jul 23;30(29):7105–7111. doi: 10.1021/bi00243a010. [DOI] [PubMed] [Google Scholar]
- Brenner B. Technique for stabilizing the striation pattern in maximally calcium-activated skinned rabbit psoas fibers. Biophys J. 1983 Jan;41(1):99–102. doi: 10.1016/S0006-3495(83)84411-7. [DOI] [PMC free article] [PubMed] [Google Scholar]
- DasGupta G., Reisler E. Actomyosin interactions in the presence of ATP and the N-terminal segment of actin. Biochemistry. 1992 Feb 18;31(6):1836–1841. doi: 10.1021/bi00121a036. [DOI] [PubMed] [Google Scholar]
- Edidin M., Zagyansky Y., Lardner T. J. Measurement of membrane protein lateral diffusion in single cells. Science. 1976 Feb 6;191(4226):466–468. doi: 10.1126/science.1246629. [DOI] [PubMed] [Google Scholar]
- Engel J., Fechner M., Sowerby A. J., Finch S. A., Stier A. Anisotropic propagation of Ca2+ waves in isolated cardiomyocytes. Biophys J. 1994 Jun;66(6):1756–1762. doi: 10.1016/S0006-3495(94)80997-X. [DOI] [PMC free article] [PubMed] [Google Scholar]
- Eppenberger H. M., Perriard J. C., Rosenberg U. B., Strehler E. E. The Mr 165,000 M-protein myomesin: a specific protein of cross-striated muscle cells. J Cell Biol. 1981 May;89(2):185–193. doi: 10.1083/jcb.89.2.185. [DOI] [PMC free article] [PubMed] [Google Scholar]
- Gershon N. D., Porter K. R., Trus B. L. The cytoplasmic matrix: its volume and surface area and the diffusion of molecules through it. Proc Natl Acad Sci U S A. 1985 Aug;82(15):5030–5034. doi: 10.1073/pnas.82.15.5030. [DOI] [PMC free article] [PubMed] [Google Scholar]
- Grove B. K., Kurer V., Lehner C., Doetschman T. C., Perriard J. C., Eppenberger H. M. A new 185,000-dalton skeletal muscle protein detected by monoclonal antibodies. J Cell Biol. 1984 Feb;98(2):518–524. doi: 10.1083/jcb.98.2.518. [DOI] [PMC free article] [PubMed] [Google Scholar]
- Hofer H. W., Gerlach G., Birkel G., Kirschenlohr H. L. The possible significance of interactions between soluble proteins in skeletal muscle. Biochem Soc Trans. 1987 Oct;15(5):982–984. doi: 10.1042/bst0150982. [DOI] [PubMed] [Google Scholar]
- Horowitz S. B. The permeability of the amphibian oocyte nucleus, in situ. J Cell Biol. 1972 Sep;54(3):609–625. doi: 10.1083/jcb.54.3.609. [DOI] [PMC free article] [PubMed] [Google Scholar]
- Jacobson K., Wojcieszyn J. The translational mobility of substances within the cytoplasmic matrix. Proc Natl Acad Sci U S A. 1984 Nov;81(21):6747–6751. doi: 10.1073/pnas.81.21.6747. [DOI] [PMC free article] [PubMed] [Google Scholar]
- Kraft T., Chalovich J. M., Yu L. C., Brenner B. Parallel inhibition of active force and relaxed fiber stiffness by caldesmon fragments at physiological ionic strength and temperature conditions: additional evidence that weak cross-bridge binding to actin is an essential intermediate for force generation. Biophys J. 1995 Jun;68(6):2404–2418. doi: 10.1016/S0006-3495(95)80423-6. [DOI] [PMC free article] [PubMed] [Google Scholar]
- Kurganov B. I., Sugrobova N. P., Mil'man L. S. Supramolecular organization of glycolytic enzymes. J Theor Biol. 1985 Oct 21;116(4):509–526. doi: 10.1016/s0022-5193(85)80086-2. [DOI] [PubMed] [Google Scholar]
- Kushmerick M. J., Podolsky R. J. Ionic mobility in muscle cells. Science. 1969 Dec 5;166(3910):1297–1298. doi: 10.1126/science.166.3910.1297. [DOI] [PubMed] [Google Scholar]
- Mastro A. M., Babich M. A., Taylor W. D., Keith A. D. Diffusion of a small molecule in the cytoplasm of mammalian cells. Proc Natl Acad Sci U S A. 1984 Jun;81(11):3414–3418. doi: 10.1073/pnas.81.11.3414. [DOI] [PMC free article] [PubMed] [Google Scholar]
- Maughan D., Lord C. Protein diffusivities in skinned frog skeletal muscle fibers. Adv Exp Med Biol. 1988;226:75–84. [PubMed] [Google Scholar]
- Messerli J. M., van der Voort H. T., Rungger-Brändle E., Perriard J. C. Three-dimensional visualization of multi-channel volume data: the amSFP algorithm. Cytometry. 1993 Oct;14(7):725–735. doi: 10.1002/cyto.990140705. [DOI] [PubMed] [Google Scholar]
- Nicholson C., Tao L. Hindered diffusion of high molecular weight compounds in brain extracellular microenvironment measured with integrative optical imaging. Biophys J. 1993 Dec;65(6):2277–2290. doi: 10.1016/S0006-3495(93)81324-9. [DOI] [PMC free article] [PubMed] [Google Scholar]
- Olson A., Pugh E. N., Jr Diffusion coefficient of cyclic GMP in salamander rod outer segments estimated with two fluorescent probes. Biophys J. 1993 Sep;65(3):1335–1352. doi: 10.1016/S0006-3495(93)81177-9. [DOI] [PMC free article] [PubMed] [Google Scholar]
- Ono T., Ono K., Mizukawa K., Ohta T., Tsuchiya T., Tsuda M. Limited diffusibility of gene products directed by a single nucleus in the cytoplasm of multinucleated myofibres. FEBS Lett. 1994 Jan 3;337(1):18–22. doi: 10.1016/0014-5793(94)80621-7. [DOI] [PubMed] [Google Scholar]
- Paine P. L., Moore L. C., Horowitz S. B. Nuclear envelope permeability. Nature. 1975 Mar 13;254(5496):109–114. doi: 10.1038/254109a0. [DOI] [PubMed] [Google Scholar]
- Schäfer B. W., Perriard J. C. Intracellular targeting of isoproteins in muscle cytoarchitecture. J Cell Biol. 1988 Apr;106(4):1161–1170. doi: 10.1083/jcb.106.4.1161. [DOI] [PMC free article] [PubMed] [Google Scholar]
- Sigel P., Pette D. Intracellular localization of glycogenolytic and glycolytic enzymes in white and red rabbit skeletal muscle: a gel film method for coupled enzyme reactions in histochemistry. J Histochem Cytochem. 1969 Apr;17(4):225–237. doi: 10.1177/17.4.225. [DOI] [PubMed] [Google Scholar]
- Swartz D. R., Greaser M. L., Marsh B. B. Regulation of binding of subfragment 1 in isolated rigor myofibrils. J Cell Biol. 1990 Dec;111(6 Pt 2):2989–3001. doi: 10.1083/jcb.111.6.2989. [DOI] [PMC free article] [PubMed] [Google Scholar]
- Wallimann T., Moser H., Eppenberger H. M. Isoenzyme-specific localization of M-line bound creatine kinase in myogenic cells. J Muscle Res Cell Motil. 1983 Aug;4(4):429–441. doi: 10.1007/BF00711948. [DOI] [PubMed] [Google Scholar]
- Weeds A. G., Taylor R. S. Separation of subfragment-1 isoenzymes from rabbit skeletal muscle myosin. Nature. 1975 Sep 4;257(5521):54–56. doi: 10.1038/257054a0. [DOI] [PubMed] [Google Scholar]
- Wegmann G., Zanolla E., Eppenberger H. M., Wallimann T. In situ compartmentation of creatine kinase in intact sarcomeric muscle: the acto-myosin overlap zone as a molecular sieve. J Muscle Res Cell Motil. 1992 Aug;13(4):420–435. doi: 10.1007/BF01738037. [DOI] [PubMed] [Google Scholar]
- Williams D. L., Jr, Greene L. E., Eisenberg E. Comparison of effects of smooth and skeletal muscle tropomyosins on interactions of actin and myosin subfragment 1. Biochemistry. 1984 Aug 28;23(18):4150–4155. doi: 10.1021/bi00313a022. [DOI] [PubMed] [Google Scholar]
- Wojcieszyn J. W., Schlegel R. A., Wu E. S., Jacobson K. A. Diffusion of injected macromolecules within the cytoplasm of living cells. Proc Natl Acad Sci U S A. 1981 Jul;78(7):4407–4410. doi: 10.1073/pnas.78.7.4407. [DOI] [PMC free article] [PubMed] [Google Scholar]
- Yu L. C., Brenner B. Structures of actomyosin crossbridges in relaxed and rigor muscle fibers. Biophys J. 1989 Mar;55(3):441–453. doi: 10.1016/S0006-3495(89)82838-3. [DOI] [PMC free article] [PubMed] [Google Scholar]