Abstract
Both chemical and physical effects of red cells have been implicated in the spontaneous aggregation of platelets in sheared whole blood (WB). To determine whether the chemical effect is due to ADP leaking from the red cells, a previously described technique for measuring the concentration and size of single platelets and aggregates was used to study the shear-induced aggregation of platelets in WB flowing through 1.19-mm-diameter polyethylene tubing in the presence and absence of the ADP scavenger enzyme system phosphocreatine-creatine phosphokinase (CP-CPK). Significant spontaneous aggregation was observed at mean tube shear rates, (G) = 41.9 and 335 s-1 (42% and 13% decrease in single platelets after a mean transit time (t) = 43 s, compared to 89 and 95% decrease with 0.2 microM ADP). The addition of CP-CPK, either at the time of, or 30 min before each run, completely abolished aggregation. In the presence of 0.2 microM ADP, CP-CPK caused a reversal of aggregation at (t) = 17 s after 30% of single cells had aggregated. To determine whether red cells exert a physical effect by increasing the time of interaction of two colliding platelets (thereby increasing the proportion of collisions resulting in the formation of aggregates), an optically transparent suspension of 40% reconstituted red cell ghosts in serum containing 2.5-micron-diameter latex spheres (3 x 10(5)/microliters) flowing through 100-microns-diameter tubes was used as a model of platelets in blood, and the results were compared with those obtained in a control suspension of latex spheres in serum alone. Two-body collisions between microspheres in the interior of the flowing ghost cell or serum suspensions at shear rates from 5 to 90 s-1 were recorded on cine film. The films were subsequently analyzed, and the measured doublet lifetime, tau meas, was compared with that predicted by theory in the absence of interactions with other particles, tau theor. The mean (tau meas/tau theor) for doublets in ghost cell suspensions was 1.614 +/- 1.795 (SD; n = 320), compared to a value of 1.001 +/- 0.312 (n = 90) for doublets in serum. Whereas 11% of doublets in ghost cell suspensions had lifetimes from 2.5 to 5 times greater than predicted, in serum, no doublets had lifetimes greater than 1.91 times that predicted. There was no statistically significant correlation between tau meas/tau theor and shear rate, but the values of tau meas/tau theor for low-angle collisions in ghost cell suspensions were significantly greater than for high-angle collisions.
Full text
PDF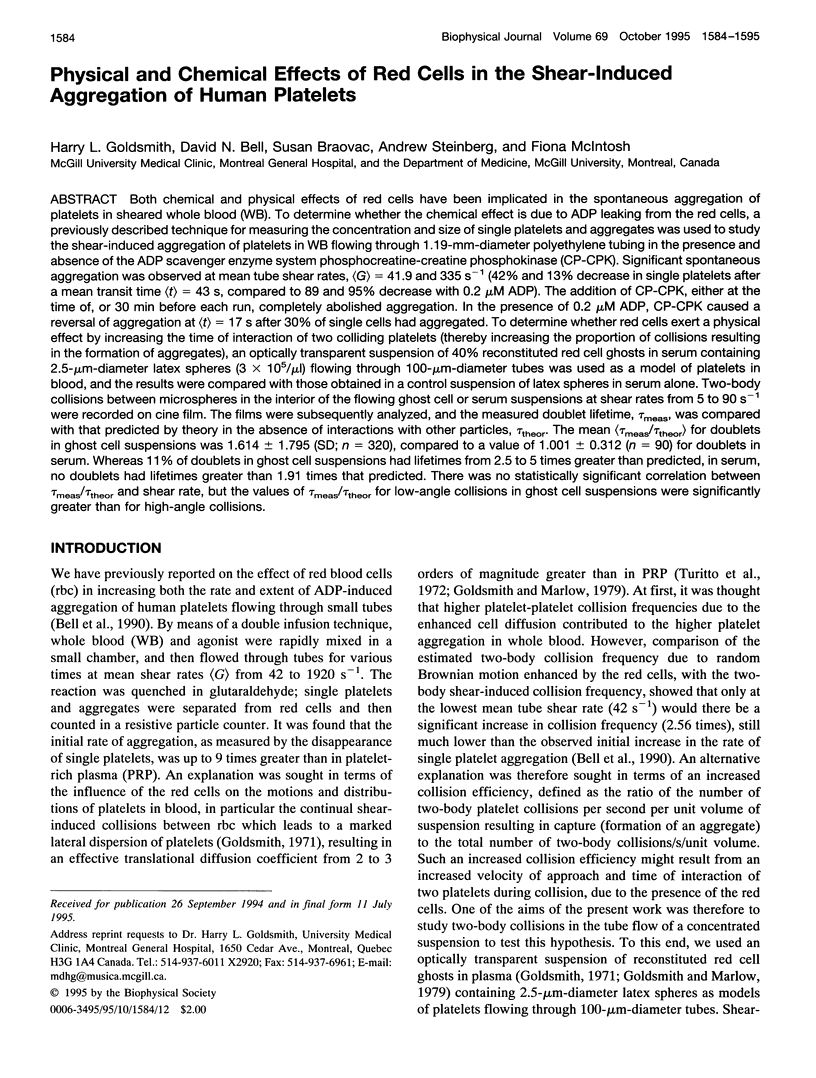
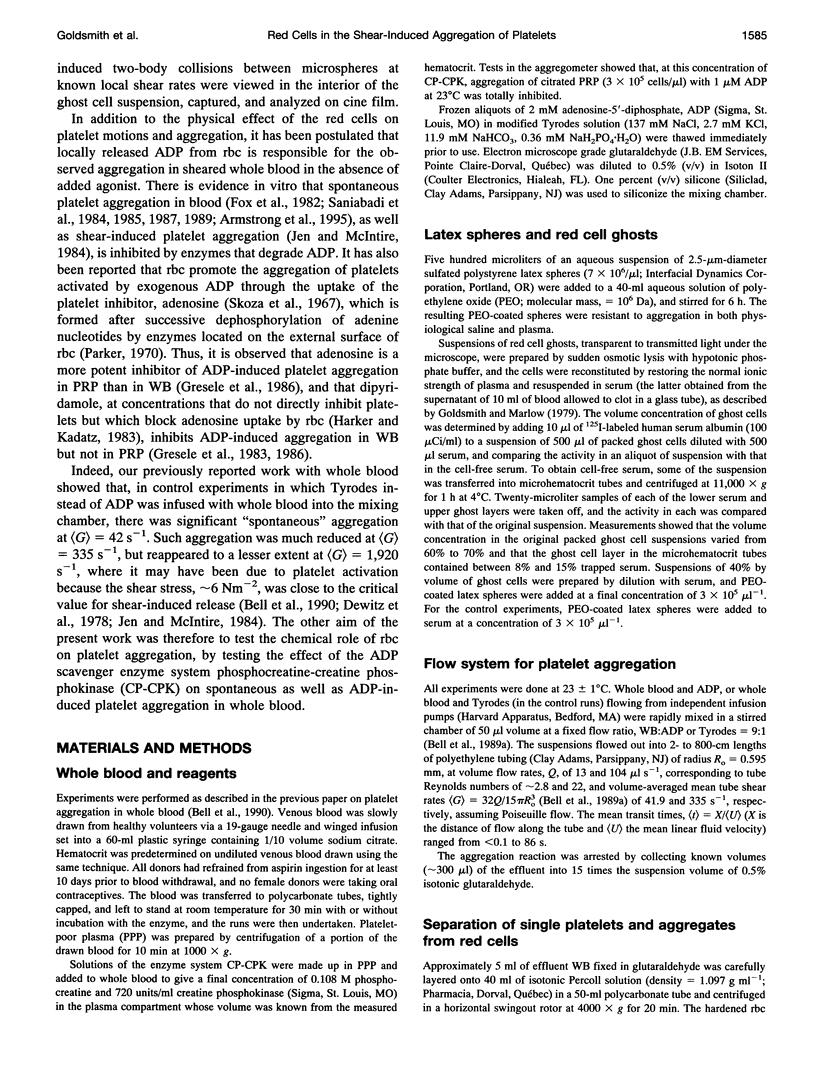
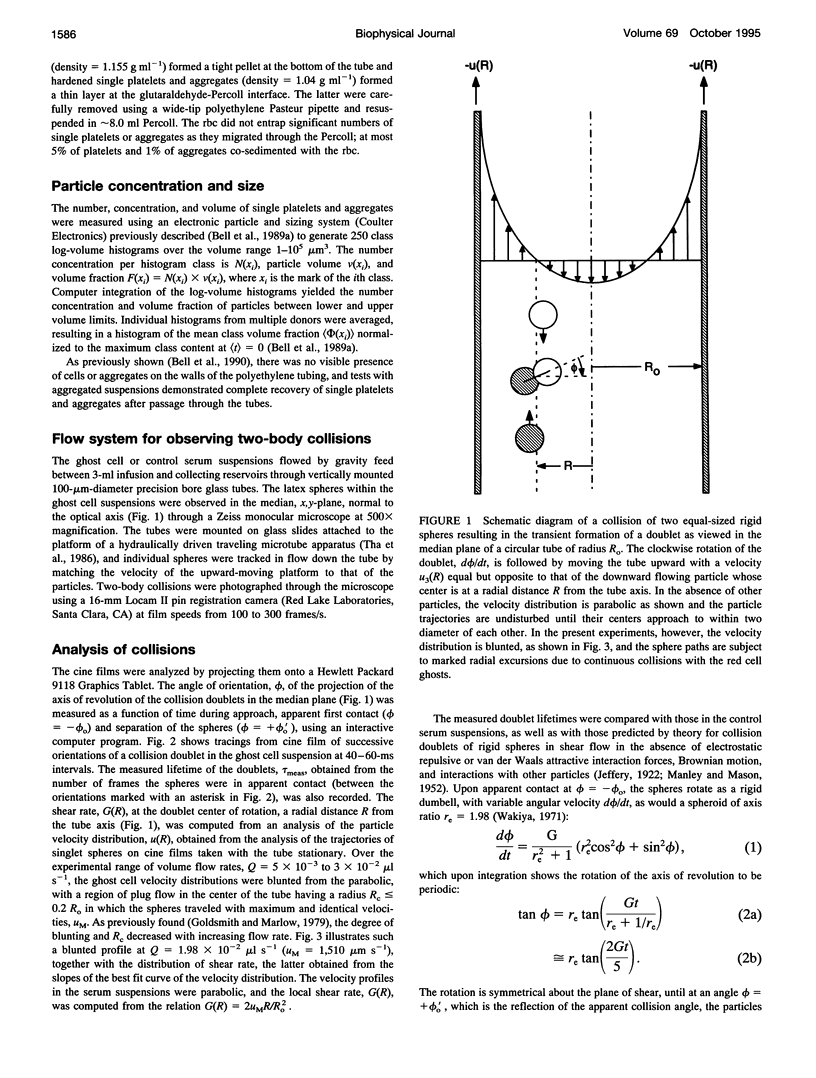
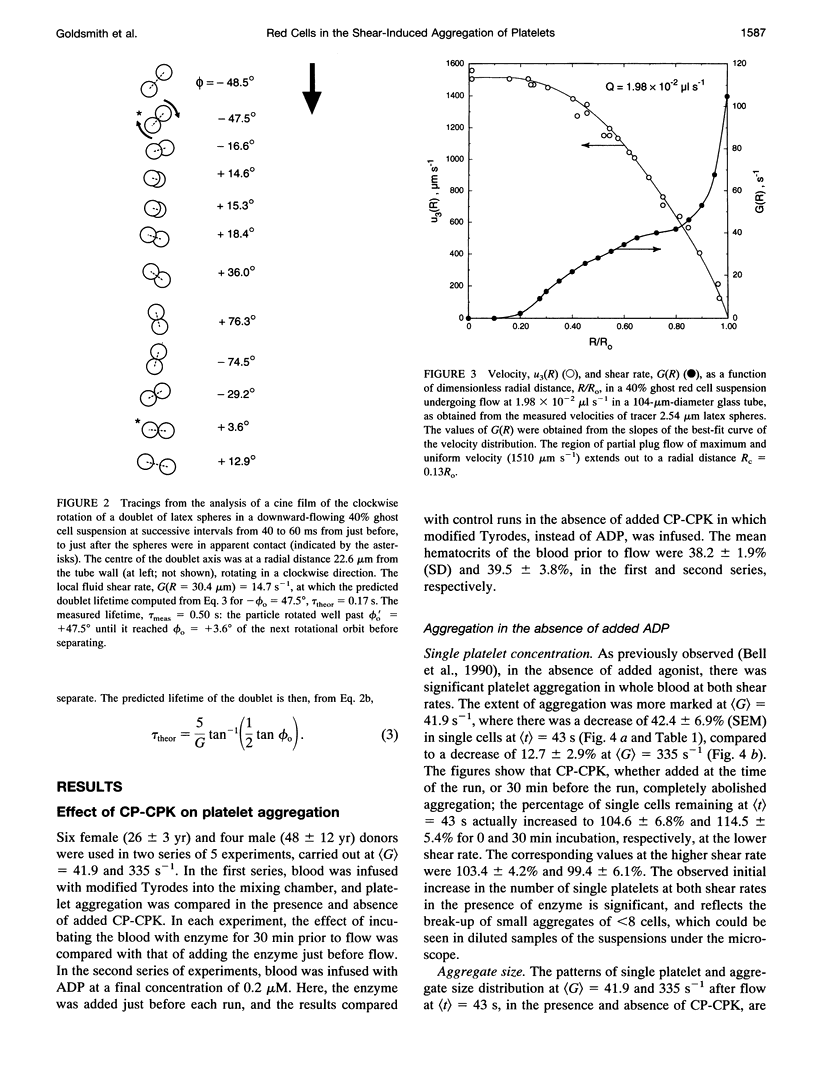
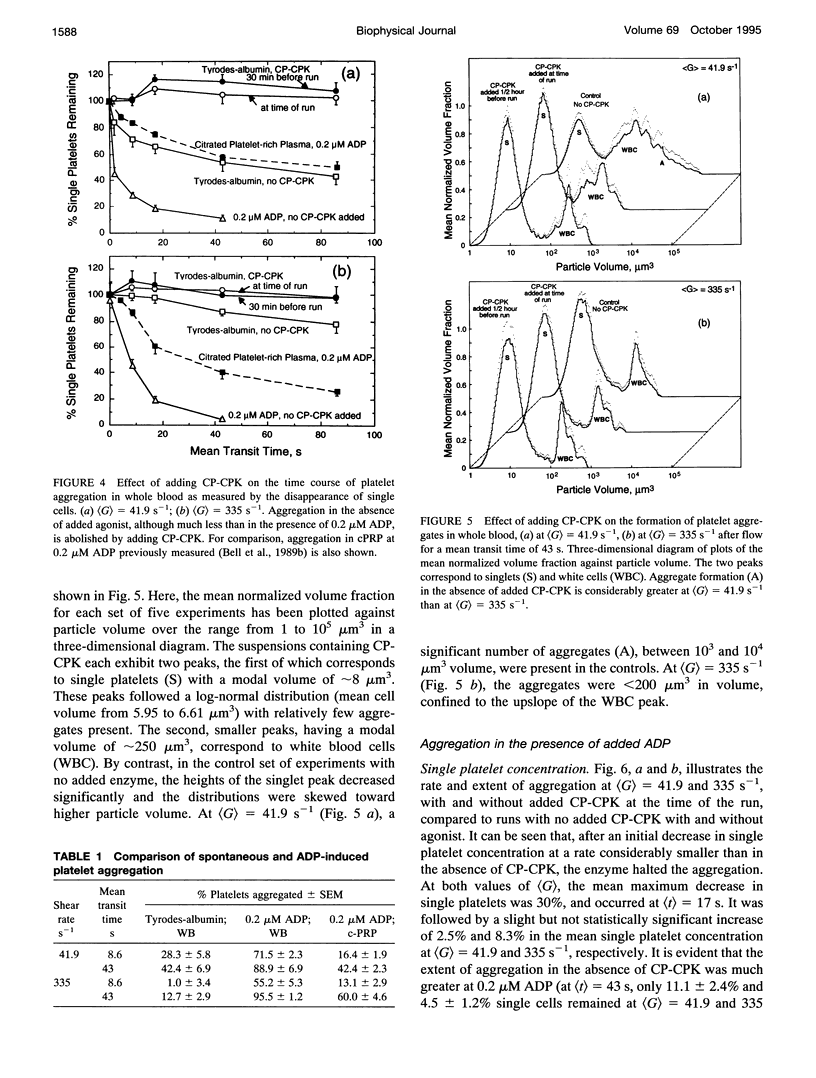
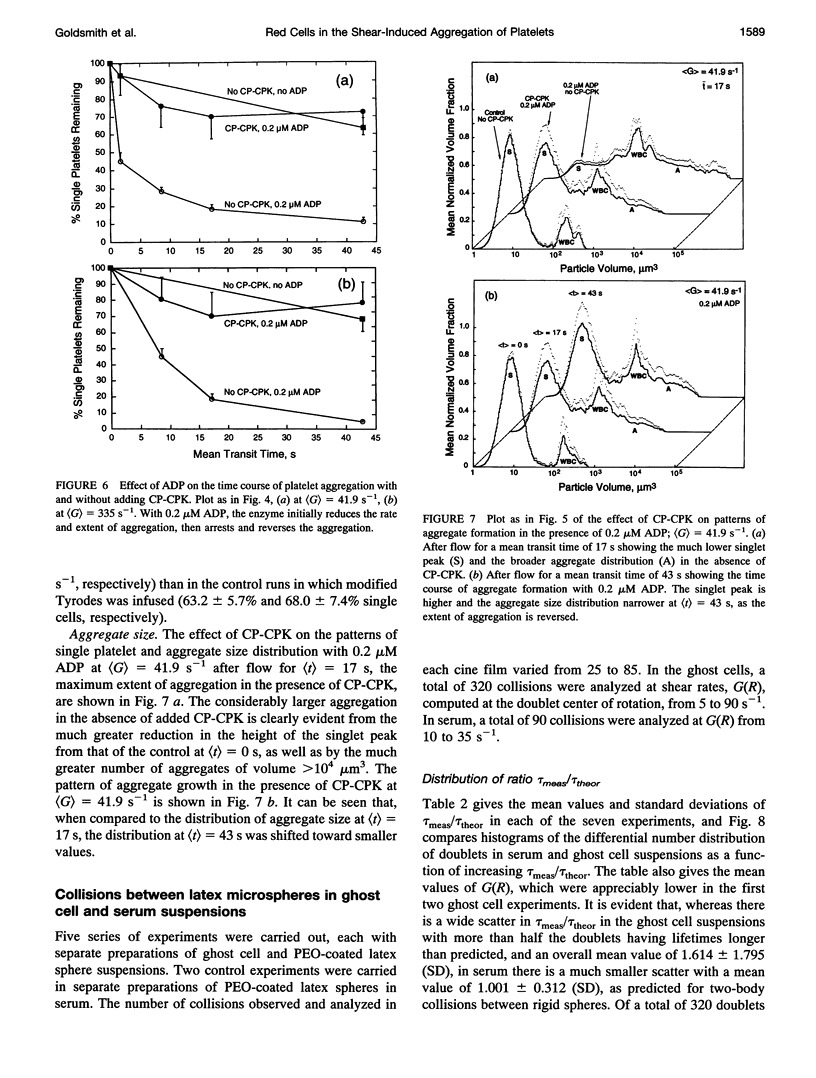
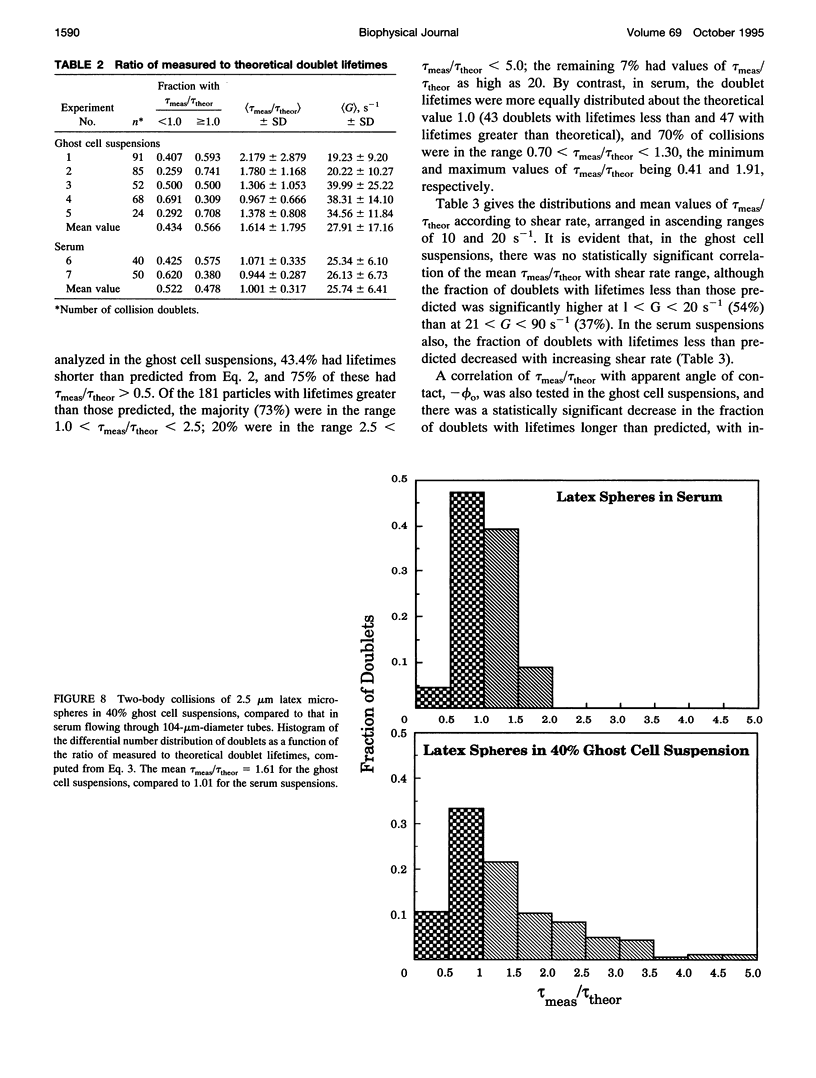
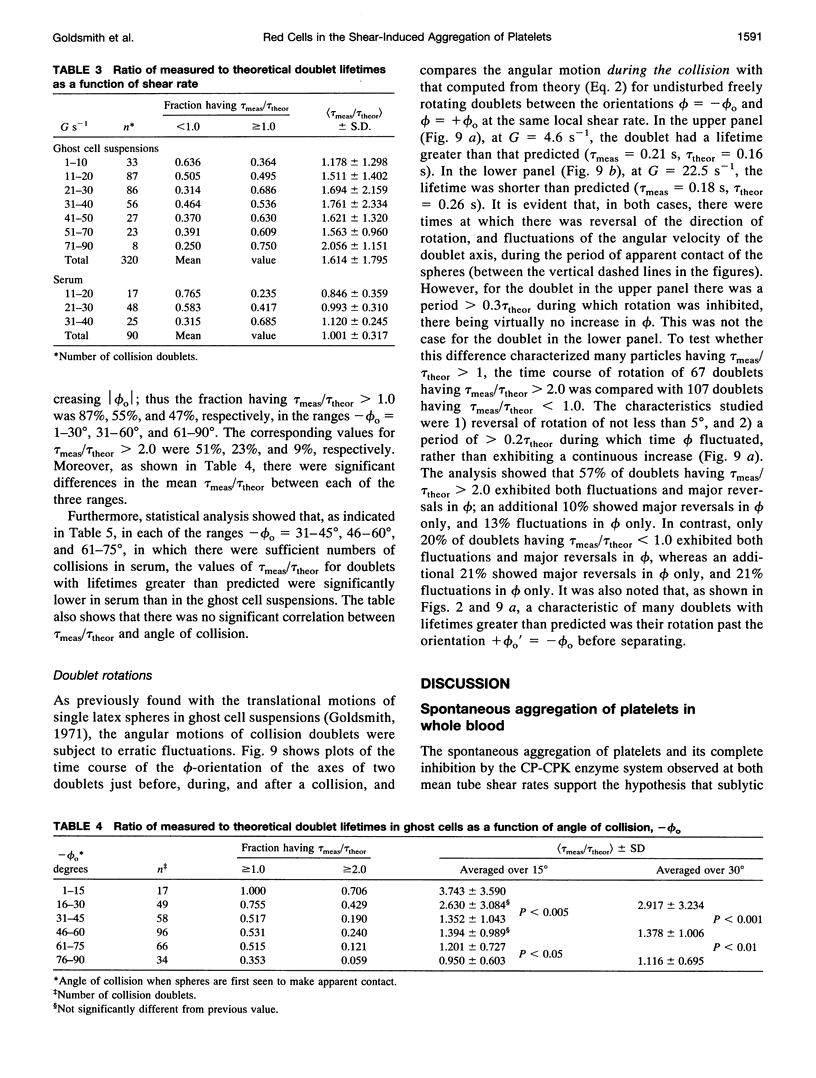
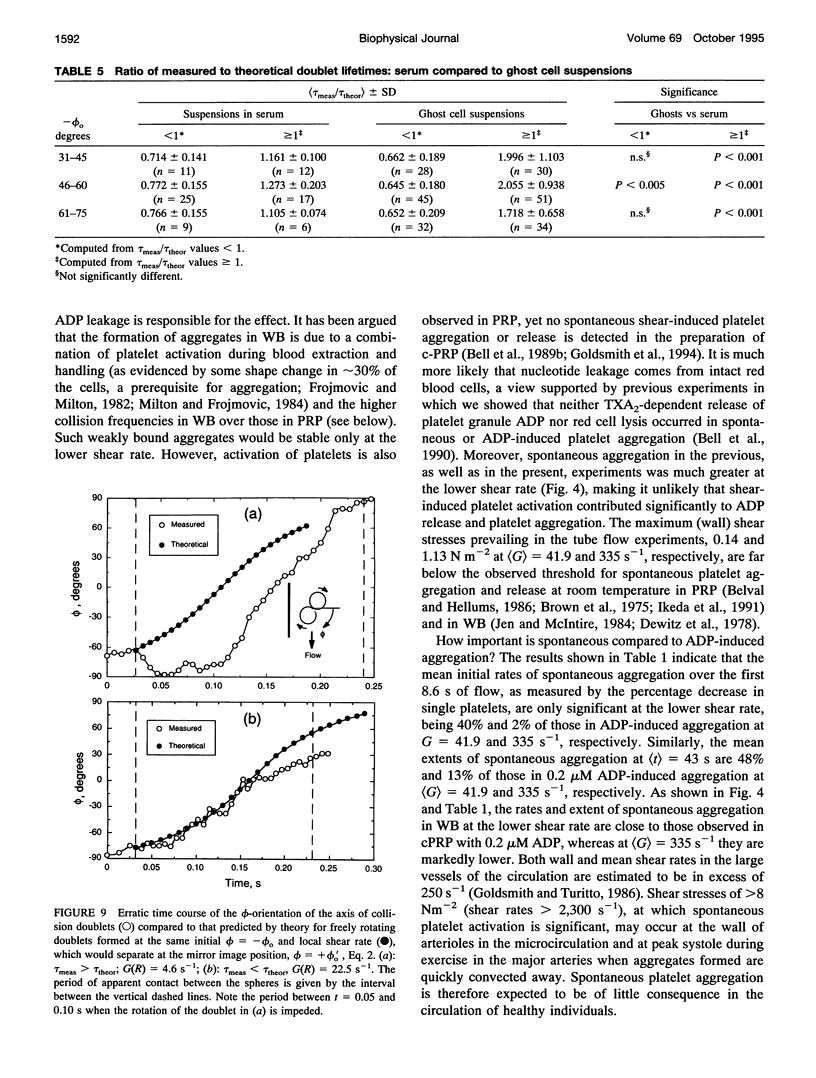
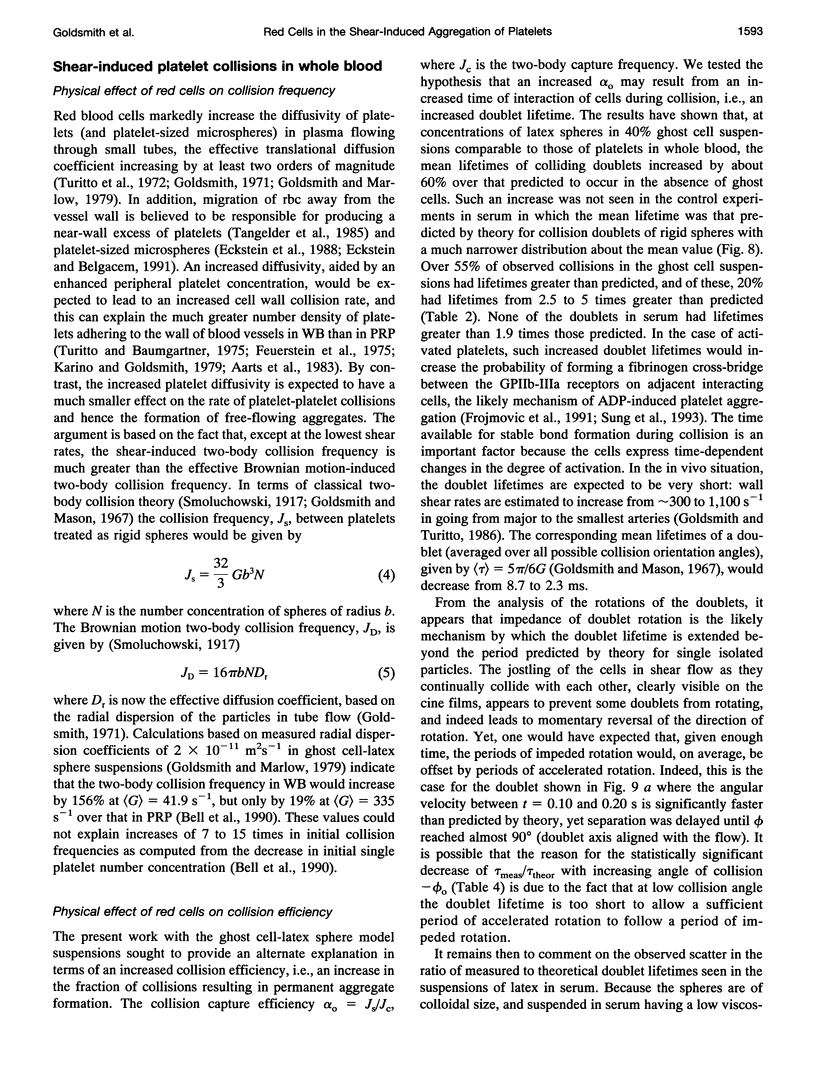
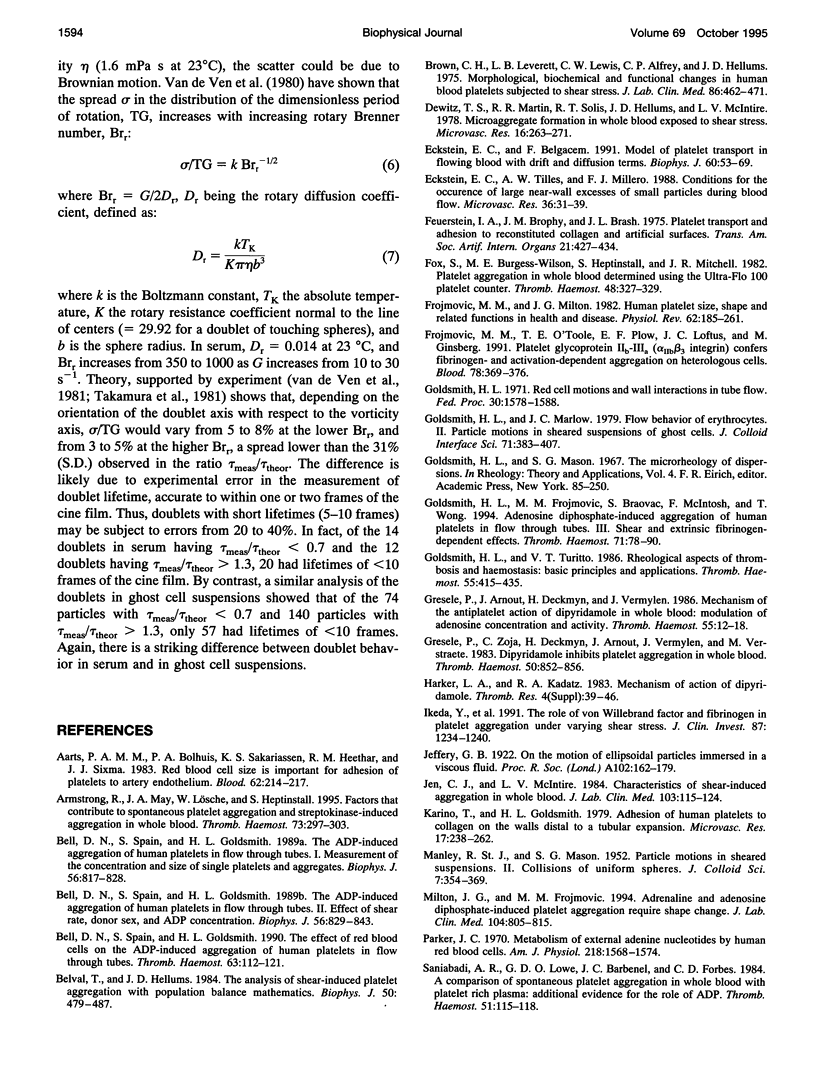
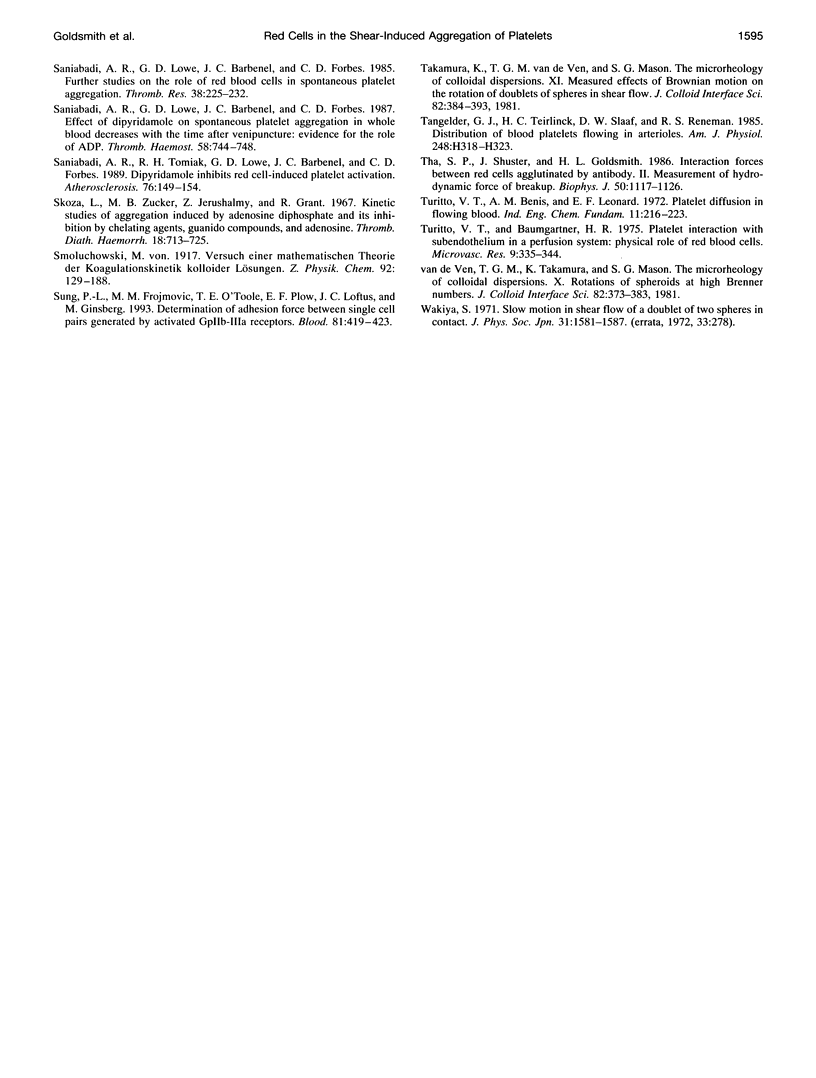
Selected References
These references are in PubMed. This may not be the complete list of references from this article.
- Aarts P. A., Bolhuis P. A., Sakariassen K. S., Heethaar R. M., Sixma J. J. Red blood cell size is important for adherence of blood platelets to artery subendothelium. Blood. 1983 Jul;62(1):214–217. [PubMed] [Google Scholar]
- Armstrong R., May J. A., Lösche W., Heptinstall S. Factors that contribute to spontaneous platelet aggregation and streptokinase-induced aggregation in whole blood. Thromb Haemost. 1995 Feb;73(2):297–303. [PubMed] [Google Scholar]
- Bell D. N., Spain S., Goldsmith H. L. Adenosine diphosphate-induced aggregation of human platelets in flow through tubes. I. Measurement of concentration and size of single platelets and aggregates. Biophys J. 1989 Nov;56(5):817–828. doi: 10.1016/S0006-3495(89)82728-6. [DOI] [PMC free article] [PubMed] [Google Scholar]
- Bell D. N., Spain S., Goldsmith H. L. Adenosine diphosphate-induced aggregation of human platelets in flow through tubes. II. Effect of shear rate, donor sex, and ADP concentration. Biophys J. 1989 Nov;56(5):829–843. doi: 10.1016/S0006-3495(89)82729-8. [DOI] [PMC free article] [PubMed] [Google Scholar]
- Bell D. N., Spain S., Goldsmith H. L. The effect of red blood cells on the ADP-induced aggregation of human platelets in flow through tubes. Thromb Haemost. 1990 Feb 19;63(1):112–121. [PubMed] [Google Scholar]
- Belval T. K., Hellums J. D. Analysis of shear-induced platelet aggregation with population balance mathematics. Biophys J. 1986 Sep;50(3):479–487. doi: 10.1016/S0006-3495(86)83485-3. [DOI] [PMC free article] [PubMed] [Google Scholar]
- Brown C. H., 3rd, Leverett L. B., Lewis C. W., Alfrey C. P., Jr, Hellums J. D. Morphological, biochemical, and functional changes in human platelets subjected to shear stress. J Lab Clin Med. 1975 Sep;86(3):462–471. [PubMed] [Google Scholar]
- Dewitz T. S., Martin R. R., Solis R. T., Hellums J. D., McIntire L. V. Microaggregate formation in whole blood exposed to shear stress. Microvasc Res. 1978 Sep;16(2):263–271. doi: 10.1016/0026-2862(78)90059-6. [DOI] [PubMed] [Google Scholar]
- Eckstein E. C., Belgacem F. Model of platelet transport in flowing blood with drift and diffusion terms. Biophys J. 1991 Jul;60(1):53–69. doi: 10.1016/S0006-3495(91)82030-6. [DOI] [PMC free article] [PubMed] [Google Scholar]
- Eckstein E. C., Tilles A. W., Millero F. J., 3rd Conditions for the occurrence of large near-wall excesses of small particles during blood flow. Microvasc Res. 1988 Jul;36(1):31–39. doi: 10.1016/0026-2862(88)90036-2. [DOI] [PubMed] [Google Scholar]
- Feuerstein I. A., Brophy J. M., Brash J. L. Platelet transport and adhesion to reconstituted collagen and artificial surfaces,. Trans Am Soc Artif Intern Organs. 1975;21:427–435. [PubMed] [Google Scholar]
- Fox S. C., Burgess-Wilson M., Heptinstall S., Mitchell J. R. Platelet aggregation in whole blood determined using the Ultra-Flo 100 Platelet Counter. Thromb Haemost. 1982 Dec 27;48(3):327–329. [PubMed] [Google Scholar]
- Frojmovic M. M., Milton J. G. Human platelet size, shape, and related functions in health and disease. Physiol Rev. 1982 Jan;62(1):185–261. doi: 10.1152/physrev.1982.62.1.185. [DOI] [PubMed] [Google Scholar]
- Frojmovic M. M., O'Toole T. E., Plow E. F., Loftus J. C., Ginsberg M. H. Platelet glycoprotein IIb-IIIa (alpha IIb beta 3 integrin) confers fibrinogen- and activation-dependent aggregation on heterologous cells. Blood. 1991 Jul 15;78(2):369–376. [PubMed] [Google Scholar]
- Goldsmith H. L., Frojmovic M. M., Braovac S., McIntosh F., Wong T. Adenosine diphosphate-induced aggregation of human platelets in flow through tubes: III. Shear and extrinsic fibrinogen-dependent effects. Thromb Haemost. 1994 Jan;71(1):78–90. [PubMed] [Google Scholar]
- Goldsmith H. L. Red cell motions and wall interactions in tube flow. Fed Proc. 1971 Sep-Oct;30(5):1578–1590. [PubMed] [Google Scholar]
- Goldsmith H. L., Turitto V. T. Rheological aspects of thrombosis and haemostasis: basic principles and applications. ICTH-Report--Subcommittee on Rheology of the International Committee on Thrombosis and Haemostasis. Thromb Haemost. 1986 Jun 30;55(3):415–435. [PubMed] [Google Scholar]
- Gresele P., Arnout J., Deckmyn H., Vermylen J. Mechanism of the antiplatelet action of dipyridamole in whole blood: modulation of adenosine concentration and activity. Thromb Haemost. 1986 Feb 28;55(1):12–18. [PubMed] [Google Scholar]
- Gresele P., Zoja C., Deckmyn H., Arnout J., Vermylen J., Verstraete M. Dipyridamole inhibits platelet aggregation in whole blood. Thromb Haemost. 1983 Dec 30;50(4):852–856. [PubMed] [Google Scholar]
- Harker L. A., Kadatz R. A. Mechanism of action of dipyridamole. Thromb Res Suppl. 1983;4:39–46. doi: 10.1016/0049-3848(83)90356-0. [DOI] [PubMed] [Google Scholar]
- Ikeda Y., Handa M., Kawano K., Kamata T., Murata M., Araki Y., Anbo H., Kawai Y., Watanabe K., Itagaki I. The role of von Willebrand factor and fibrinogen in platelet aggregation under varying shear stress. J Clin Invest. 1991 Apr;87(4):1234–1240. doi: 10.1172/JCI115124. [DOI] [PMC free article] [PubMed] [Google Scholar]
- Jen C. J., McIntire L. V. Characteristics of shear-induced aggregation in whole blood. J Lab Clin Med. 1984 Jan;103(1):115–124. [PubMed] [Google Scholar]
- Karino T., Goldsmith H. L. Adhesion of human platelets to collagen on the walls distal to a tubular expansion. Microvasc Res. 1979 May;17(3 Pt 1):238–262. doi: 10.1016/s0026-2862(79)80002-3. [DOI] [PubMed] [Google Scholar]
- Milton J. G., Frojmovic M. M. Adrenaline and adenosine diphosphate-induced platelet aggregation require shape change. Importance of pseudopods. J Lab Clin Med. 1984 Nov;104(5):805–815. [PubMed] [Google Scholar]
- Parker J. C. Metabolism of external adenine nucleotides by human red blood cells. Am J Physiol. 1970 Jun;218(6):1568–1574. doi: 10.1152/ajplegacy.1970.218.6.1568. [DOI] [PubMed] [Google Scholar]
- Saniabadi A. R., Lowe G. D., Barbenel J. C., Forbes C. D. A comparison of spontaneous platelet aggregation in whole blood with platelet rich plasma: additional evidence for the role of ADP. Thromb Haemost. 1984 Feb 28;51(1):115–118. [PubMed] [Google Scholar]
- Saniabadi A. R., Lowe G. D., Barbenel J. C., Forbes C. D. Effect of dipyridamole on spontaneous platelet aggregation in whole blood decreases with the time after venepuncture: evidence for the role of ADP. Thromb Haemost. 1987 Aug 4;58(2):744–748. [PubMed] [Google Scholar]
- Saniabadi A. R., Lowe G. D., Barbenel J. C., Forbes C. D. Further studies on the role of red blood cells in spontaneous platelet aggregation. Thromb Res. 1985 May 1;38(3):225–232. doi: 10.1016/0049-3848(85)90150-1. [DOI] [PubMed] [Google Scholar]
- Saniabadi A. R., Tomiak R. H., Lowe G. D., Barbenel J. C., Forbes C. D. Dipyridamole inhibits red cell-induced platelet activation. Atherosclerosis. 1989 Apr;76(2-3):149–154. doi: 10.1016/0021-9150(89)90098-1. [DOI] [PubMed] [Google Scholar]
- Skoza L., Zucker M. B., Jerushalmy Z., Grant R. Kinetic studies of platelet aggregation induced by adenosine diphosphate and its inhibition by chelating agents, guanidino compounds, and adenosine. Thromb Diath Haemorrh. 1967 Dec 31;18(3-4):713–725. [PubMed] [Google Scholar]
- Sung K. L., Frojmovic M. M., O'Toole T. E., Zhu C., Ginsberg M. H., Chien S. Determination of adhesion force between single cell pairs generated by activated GpIIb-IIIa receptors. Blood. 1993 Jan 15;81(2):419–423. [PubMed] [Google Scholar]
- Tangelder G. J., Teirlinck H. C., Slaaf D. W., Reneman R. S. Distribution of blood platelets flowing in arterioles. Am J Physiol. 1985 Mar;248(3 Pt 2):H318–H323. doi: 10.1152/ajpheart.1985.248.3.H318. [DOI] [PubMed] [Google Scholar]
- Tha S. P., Shuster J., Goldsmith H. L. Interaction forces between red cells agglutinated by antibody. II. Measurement of hydrodynamic force of breakup. Biophys J. 1986 Dec;50(6):1117–1126. doi: 10.1016/S0006-3495(86)83556-1. [DOI] [PMC free article] [PubMed] [Google Scholar]
- Turitto V. T., Baumgartner H. R. Platelet interaction with subendothelium in a perfusion system: physical role of red blood cells. Microvasc Res. 1975 May;9(3):335–344. doi: 10.1016/0026-2862(75)90070-9. [DOI] [PubMed] [Google Scholar]