Abstract
Once formed, fusion pores rapidly enlarge to semi-stable conductance values. The membranes lining the fusion pore are continuous bilayer structures, so variations of conductance in time reflect bending and stretching of membranes. We therefore modeled the evolution of fusion pores using the theory of the mechanics of deforming homogeneous membranes. We calculated the changes in length and width of theoretical fusion pores according to standard dynamical equations of motion. Theoretical fusion pores quickly achieve semi-stable dimensions, which correspond to energy minima located in a canyon between energy barriers. The height of the barrier preventing pore expansion diminishes along the dimensions of length and width. The bottom of the canyon slopes gently downward along increasing length. As a consequence, theoretical fusion pores slowly lengthen and widen as the dimensions migrate along the bottom of the canyon, until the barrier vanishes and the pore rapidly enlarges. The dynamics of growth is sensitive to tension, spontaneous curvature, bending elasticity, and mobilities. This sensitivity can account for the quantitative differences in pore evolution observed in two experimental systems: HA-expressing cells fusing to planar bilayer membranes and beige mouse mast cell degranulation. We conclude that the mechanics of membranes could cause the phenomenon of stagewise growth of fusion pores.
Full text
PDF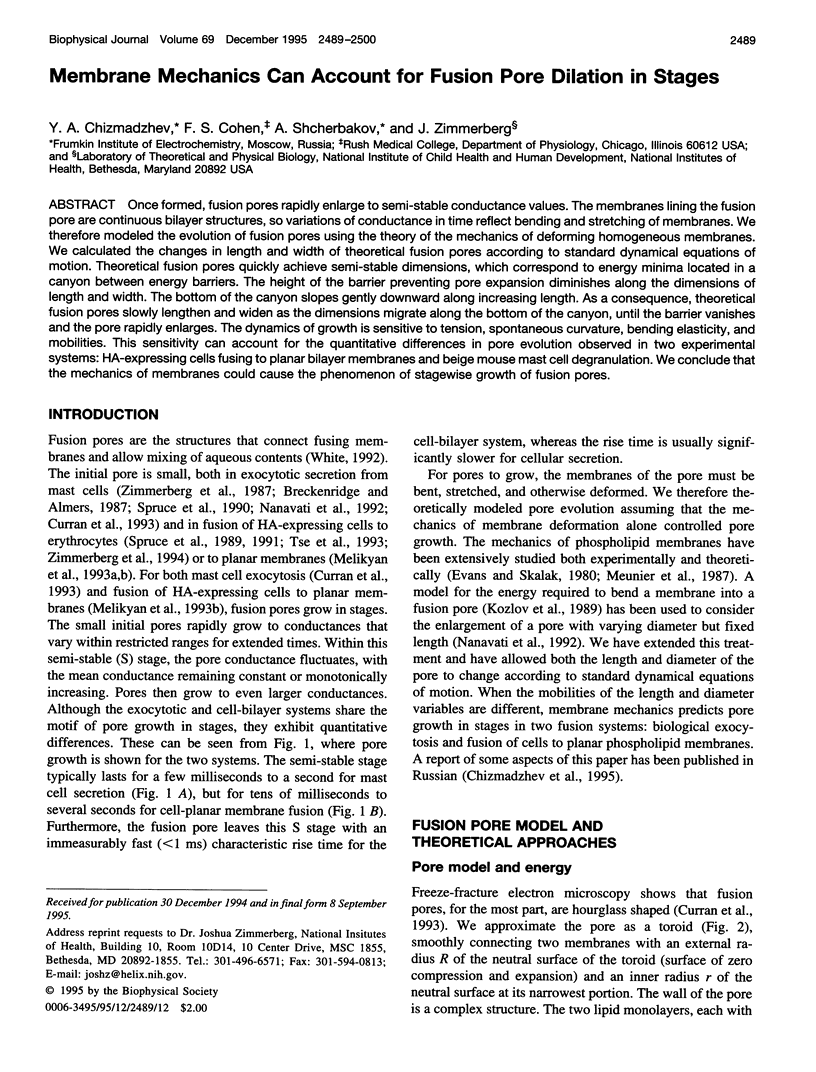
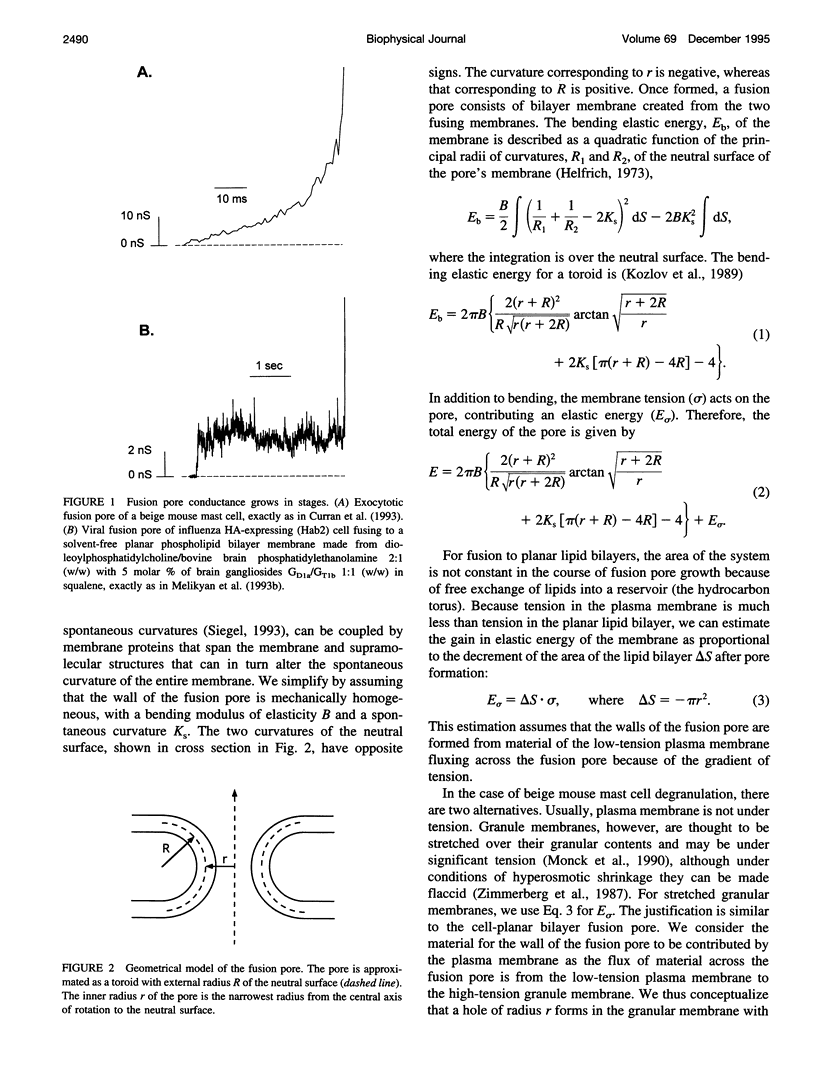
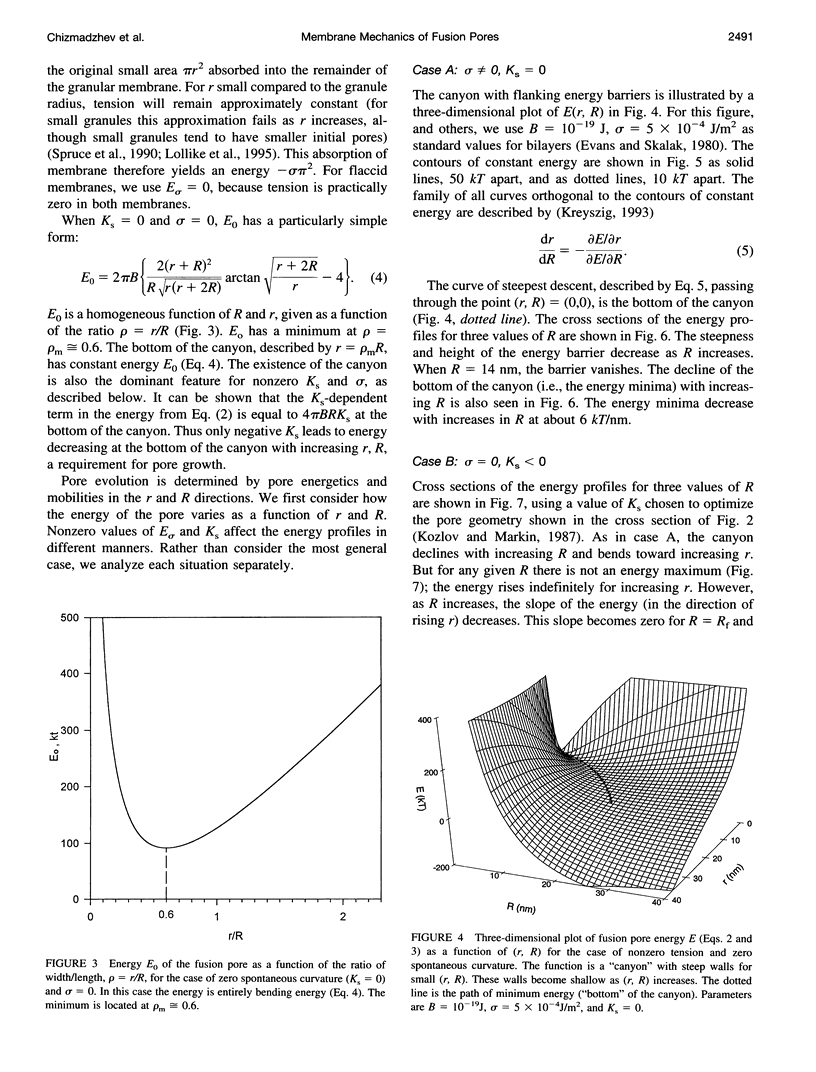
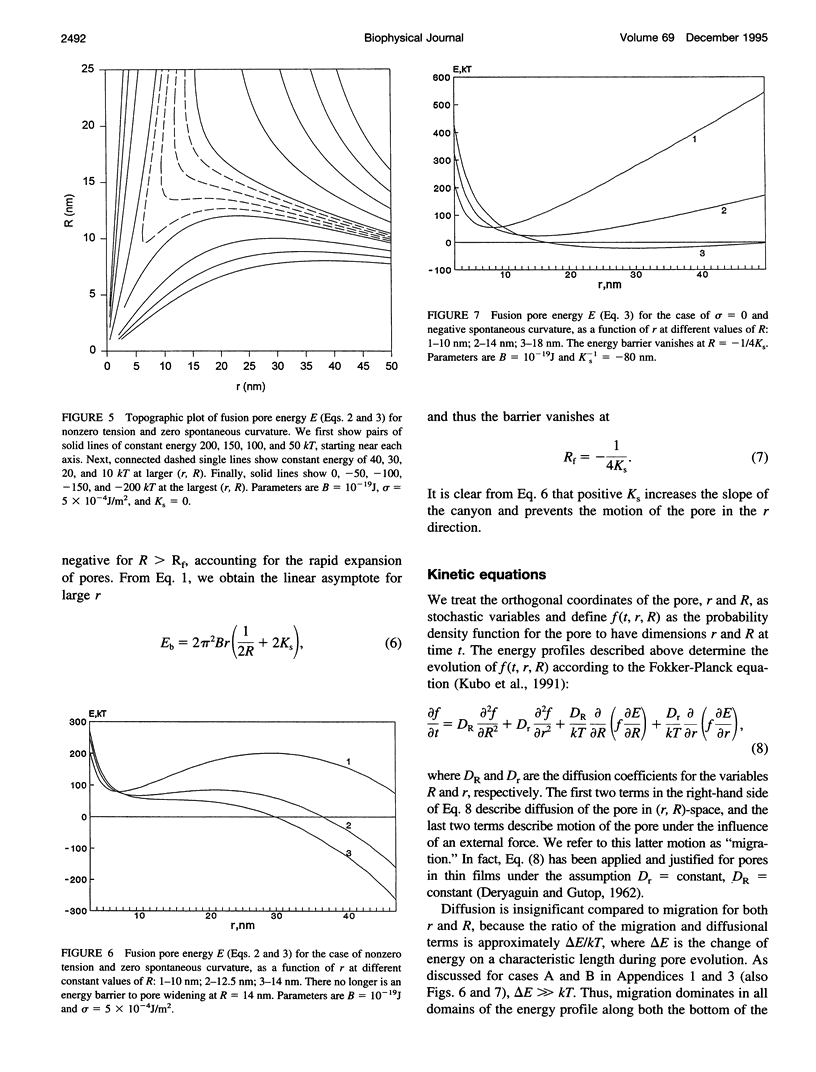
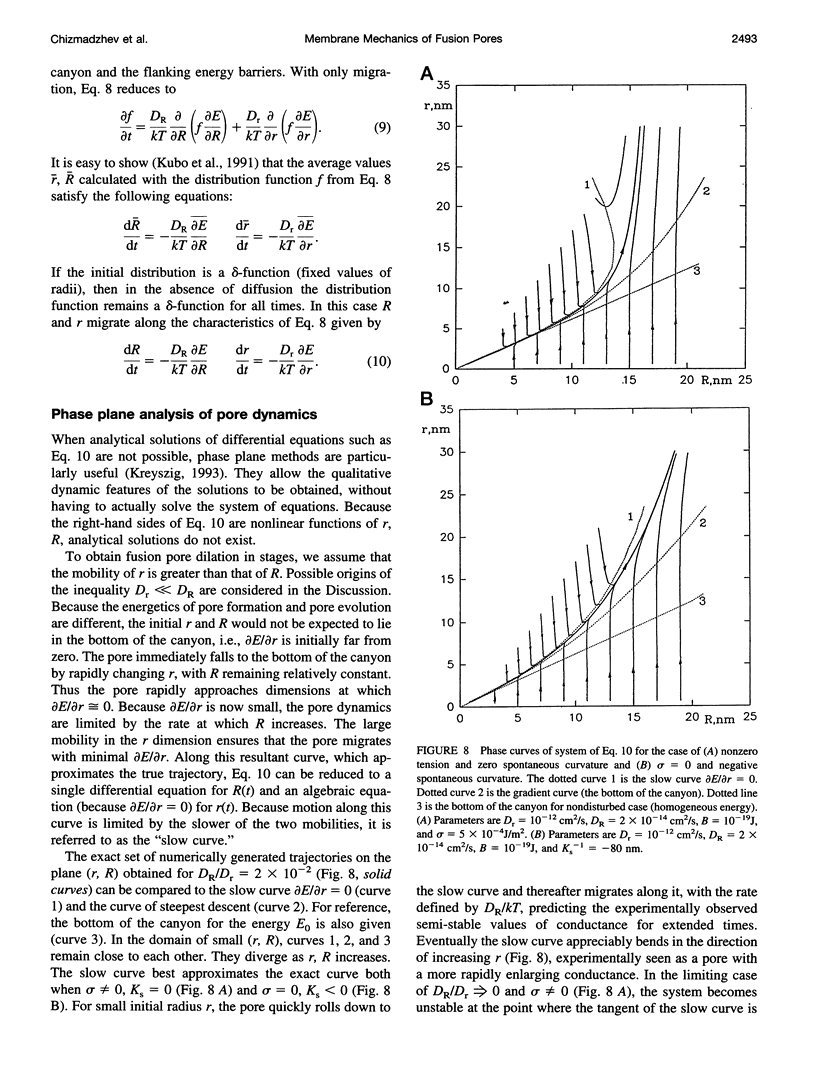
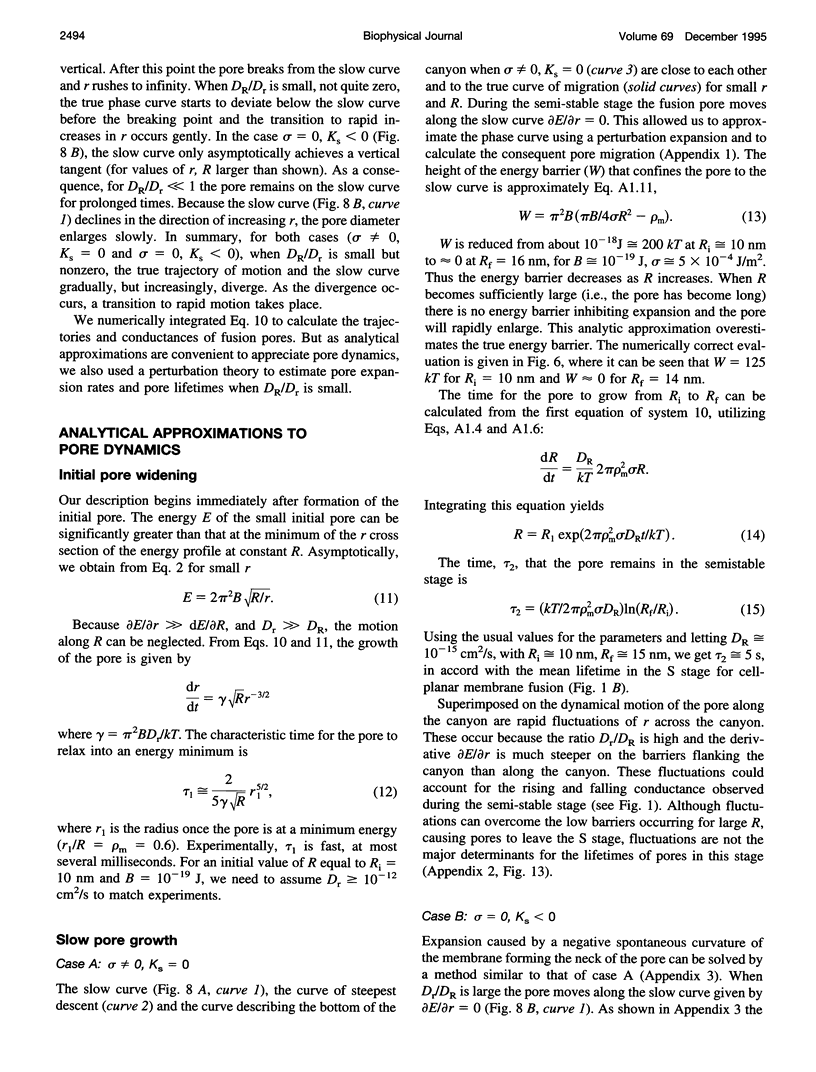
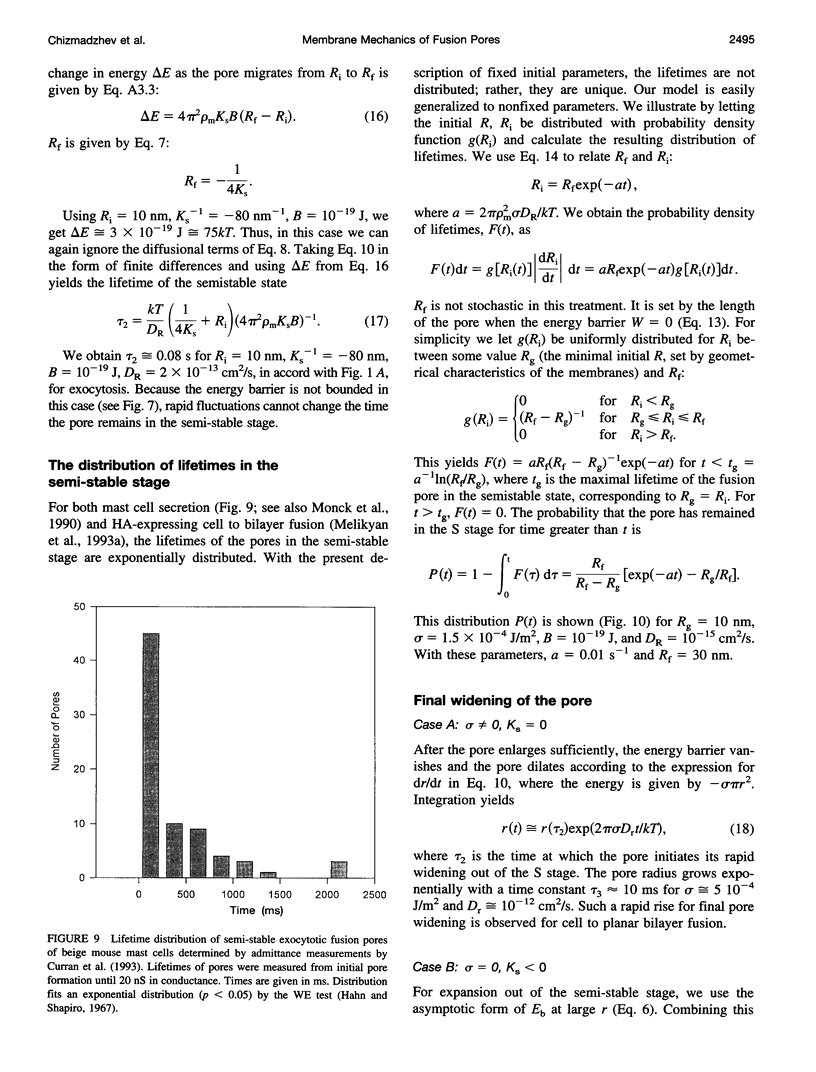
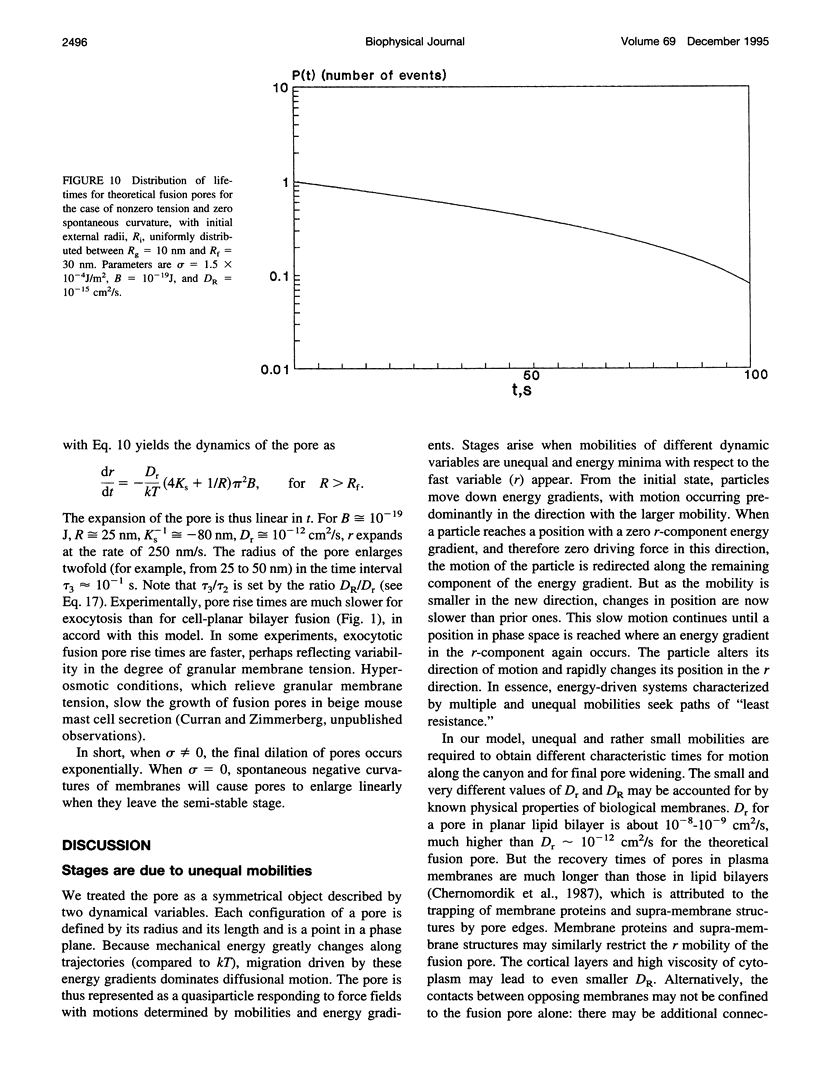
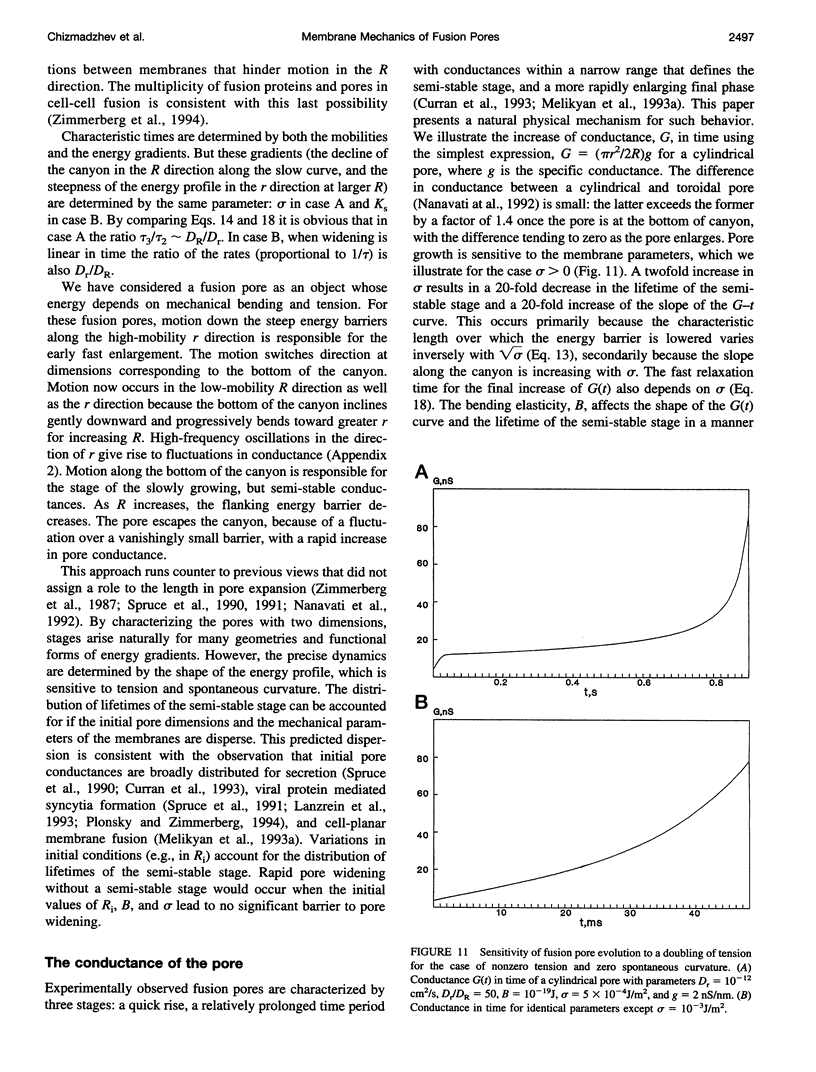
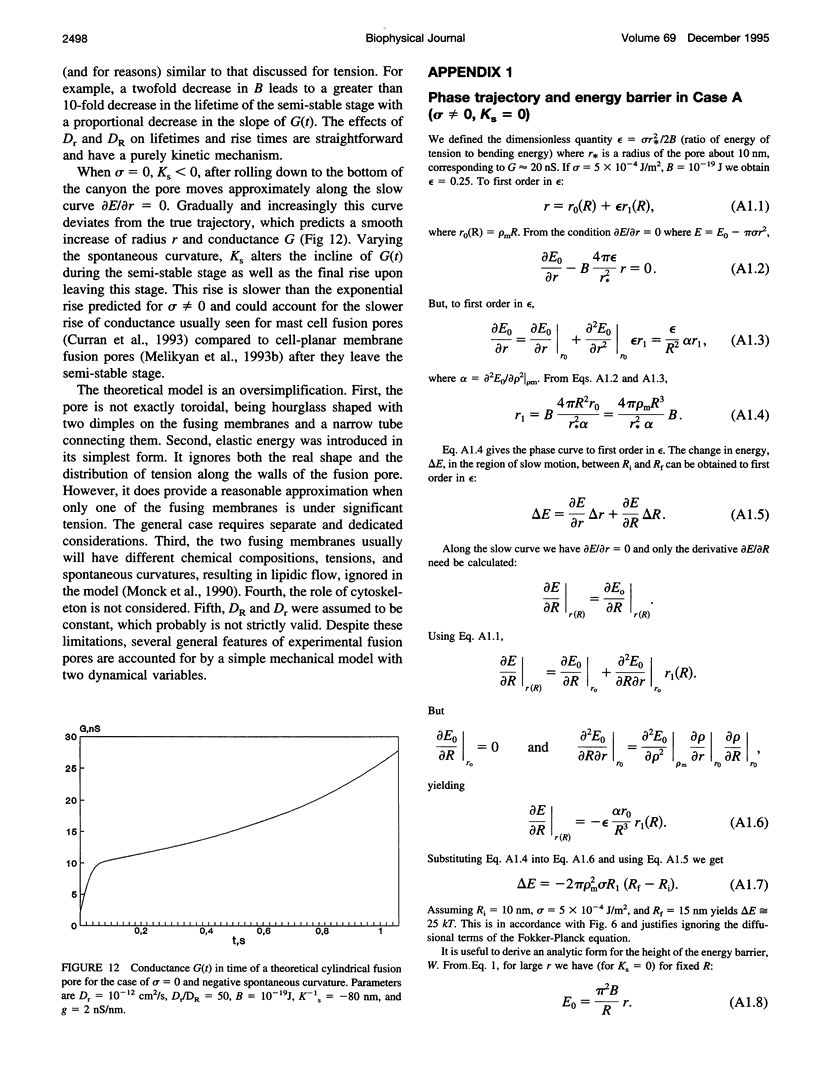
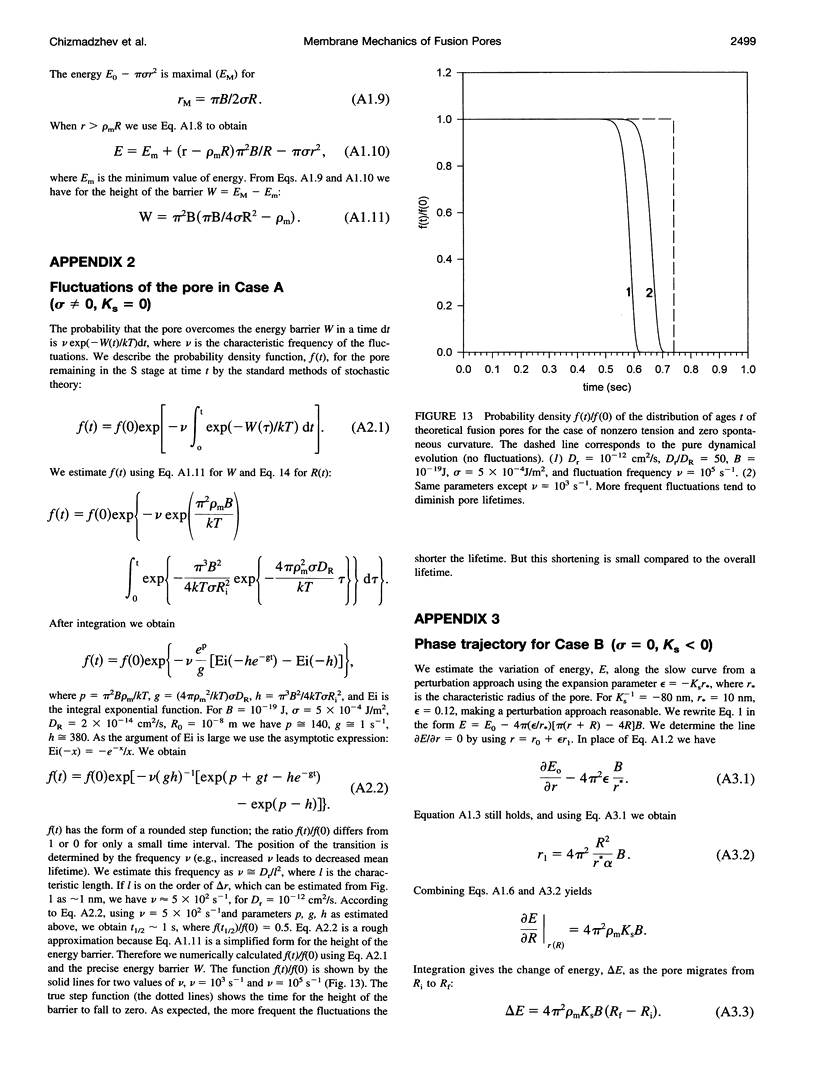
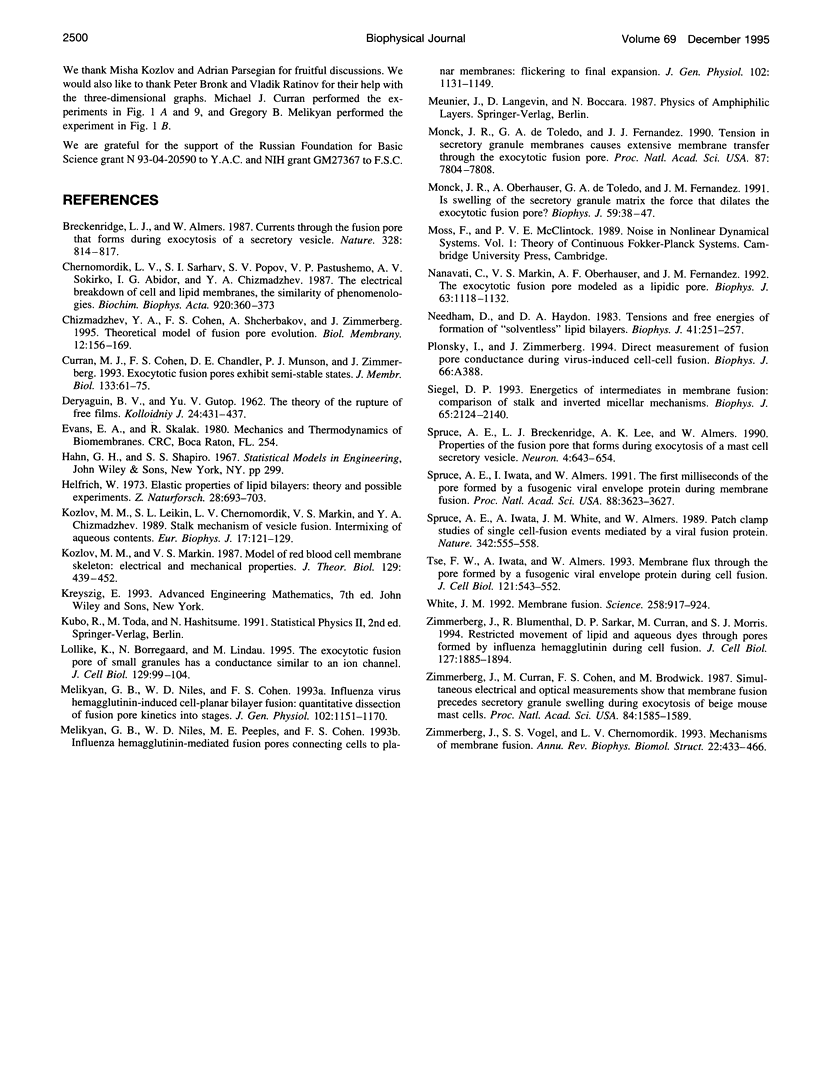
Images in this article
Selected References
These references are in PubMed. This may not be the complete list of references from this article.
- Breckenridge L. J., Almers W. Currents through the fusion pore that forms during exocytosis of a secretory vesicle. 1987 Aug 27-Sep 2Nature. 328(6133):814–817. doi: 10.1038/328814a0. [DOI] [PubMed] [Google Scholar]
- Chernomordik L. V., Sukharev S. I., Popov S. V., Pastushenko V. F., Sokirko A. V., Abidor I. G., Chizmadzhev Y. A. The electrical breakdown of cell and lipid membranes: the similarity of phenomenologies. Biochim Biophys Acta. 1987 Sep 3;902(3):360–373. doi: 10.1016/0005-2736(87)90204-5. [DOI] [PubMed] [Google Scholar]
- Curran M. J., Cohen F. S., Chandler D. E., Munson P. J., Zimmerberg J. Exocytotic fusion pores exhibit semi-stable states. J Membr Biol. 1993 Apr;133(1):61–75. doi: 10.1007/BF00231878. [DOI] [PubMed] [Google Scholar]
- Helfrich W. Elastic properties of lipid bilayers: theory and possible experiments. Z Naturforsch C. 1973 Nov-Dec;28(11):693–703. doi: 10.1515/znc-1973-11-1209. [DOI] [PubMed] [Google Scholar]
- Kozlov M. M., Leikin S. L., Chernomordik L. V., Markin V. S., Chizmadzhev Y. A. Stalk mechanism of vesicle fusion. Intermixing of aqueous contents. Eur Biophys J. 1989;17(3):121–129. doi: 10.1007/BF00254765. [DOI] [PubMed] [Google Scholar]
- Kozlov M. M., Markin V. S. Model of red blood cell membrane skeleton: electrical and mechanical properties. J Theor Biol. 1987 Dec 21;129(4):439–452. doi: 10.1016/s0022-5193(87)80023-1. [DOI] [PubMed] [Google Scholar]
- Lollike K., Borregaard N., Lindau M. The exocytotic fusion pore of small granules has a conductance similar to an ion channel. J Cell Biol. 1995 Apr;129(1):99–104. doi: 10.1083/jcb.129.1.99. [DOI] [PMC free article] [PubMed] [Google Scholar]
- Melikyan G. B., Niles W. D., Cohen F. S. Influenza virus hemagglutinin-induced cell-planar bilayer fusion: quantitative dissection of fusion pore kinetics into stages. J Gen Physiol. 1993 Dec;102(6):1151–1170. doi: 10.1085/jgp.102.6.1151. [DOI] [PMC free article] [PubMed] [Google Scholar]
- Melikyan G. B., Niles W. D., Peeples M. E., Cohen F. S. Influenza hemagglutinin-mediated fusion pores connecting cells to planar membranes: flickering to final expansion. J Gen Physiol. 1993 Dec;102(6):1131–1149. doi: 10.1085/jgp.102.6.1131. [DOI] [PMC free article] [PubMed] [Google Scholar]
- Monck J. R., Alvarez de Toledo G., Fernandez J. M. Tension in secretory granule membranes causes extensive membrane transfer through the exocytotic fusion pore. Proc Natl Acad Sci U S A. 1990 Oct;87(20):7804–7808. doi: 10.1073/pnas.87.20.7804. [DOI] [PMC free article] [PubMed] [Google Scholar]
- Monck J. R., Oberhauser A. F., Alvarez de Toledo G., Fernandez J. M. Is swelling of the secretory granule matrix the force that dilates the exocytotic fusion pore? Biophys J. 1991 Jan;59(1):39–47. doi: 10.1016/S0006-3495(91)82196-8. [DOI] [PMC free article] [PubMed] [Google Scholar]
- Nanavati C., Markin V. S., Oberhauser A. F., Fernandez J. M. The exocytotic fusion pore modeled as a lipidic pore. Biophys J. 1992 Oct;63(4):1118–1132. doi: 10.1016/S0006-3495(92)81679-X. [DOI] [PMC free article] [PubMed] [Google Scholar]
- Needham D., Haydon D. A. Tensions and free energies of formation of "solventless" lipid bilayers. Measurement of high contact angles. Biophys J. 1983 Mar;41(3):251–257. doi: 10.1016/S0006-3495(83)84435-X. [DOI] [PMC free article] [PubMed] [Google Scholar]
- Siegel D. P. Energetics of intermediates in membrane fusion: comparison of stalk and inverted micellar intermediate mechanisms. Biophys J. 1993 Nov;65(5):2124–2140. doi: 10.1016/S0006-3495(93)81256-6. [DOI] [PMC free article] [PubMed] [Google Scholar]
- Spruce A. E., Breckenridge L. J., Lee A. K., Almers W. Properties of the fusion pore that forms during exocytosis of a mast cell secretory vesicle. Neuron. 1990 May;4(5):643–654. doi: 10.1016/0896-6273(90)90192-i. [DOI] [PubMed] [Google Scholar]
- Spruce A. E., Iwata A., Almers W. The first milliseconds of the pore formed by a fusogenic viral envelope protein during membrane fusion. Proc Natl Acad Sci U S A. 1991 May 1;88(9):3623–3627. doi: 10.1073/pnas.88.9.3623. [DOI] [PMC free article] [PubMed] [Google Scholar]
- Spruce A. E., Iwata A., White J. M., Almers W. Patch clamp studies of single cell-fusion events mediated by a viral fusion protein. Nature. 1989 Nov 30;342(6249):555–558. doi: 10.1038/342555a0. [DOI] [PubMed] [Google Scholar]
- Tse F. W., Iwata A., Almers W. Membrane flux through the pore formed by a fusogenic viral envelope protein during cell fusion. J Cell Biol. 1993 May;121(3):543–552. doi: 10.1083/jcb.121.3.543. [DOI] [PMC free article] [PubMed] [Google Scholar]
- White J. M. Membrane fusion. Science. 1992 Nov 6;258(5084):917–924. doi: 10.1126/science.1439803. [DOI] [PubMed] [Google Scholar]
- Zimmerberg J., Blumenthal R., Sarkar D. P., Curran M., Morris S. J. Restricted movement of lipid and aqueous dyes through pores formed by influenza hemagglutinin during cell fusion. J Cell Biol. 1994 Dec;127(6 Pt 2):1885–1894. doi: 10.1083/jcb.127.6.1885. [DOI] [PMC free article] [PubMed] [Google Scholar]
- Zimmerberg J., Curran M., Cohen F. S., Brodwick M. Simultaneous electrical and optical measurements show that membrane fusion precedes secretory granule swelling during exocytosis of beige mouse mast cells. Proc Natl Acad Sci U S A. 1987 Mar;84(6):1585–1589. doi: 10.1073/pnas.84.6.1585. [DOI] [PMC free article] [PubMed] [Google Scholar]
- Zimmerberg J., Vogel S. S., Chernomordik L. V. Mechanisms of membrane fusion. Annu Rev Biophys Biomol Struct. 1993;22:433–466. doi: 10.1146/annurev.bb.22.060193.002245. [DOI] [PubMed] [Google Scholar]