Abstract
1. In voltage clamped myelinated nerve fibres, the K+ conductance has been calculated from current recordings obtained in low and high K+ media, taking into account the changes in EK resulting from accumulation of depletion of K+ ions near to nodal membrane. 2. At the end of a depolarization, the instantaneous K+ current reverses at a potential (instantaneous reversal potential) differing from the Nernst potential calculated using the external and internal bulk concentrations (theoretical Nernst potential). During a depolarization, EK, as estimated from the instantaneous reversal potential, changes continuously. This change depends on the size, the duration and the direction of the time dependent K+ current. The variation of EK is attributed to continuous changes in K+ concentration near the membrane during voltage pulses which turn on the K+ conductance. 3. The chord conductance [GK = IK/(E-EK), as calculated using the instantaneous reversal potential values for EK, has been analysed as a function of time and membrane potential. As previously reported it increases with the initial K+ concentration in the external medium. 4. The time course of the K+ current depends on both the kinetics of the conductance increase and the rate of change in the driving force for K. The kinetics of the conductance increase can satisfactorily be described by a single exponential function following a delay after the onset of the depolarizing voltage clamp pulse. 5. This delay increases when the holding potential is made more negative. It decreases with membrane depolarization and it is independent of the external K+ concentration. At a given membrane potential, the turning on of the K+ conductance is found to be faster at high than at low external K+ concentrations. 6. At repolarization the turning off of the conductance cannot be described by a single exponential function. It is faster at low than at high external K+ concentrations. 7. The results suggest that the change in K+ conductance proceeds in a multi-step transition or (and) that the K+ conductance is determined by several types of K+ channels.
Full text
PDF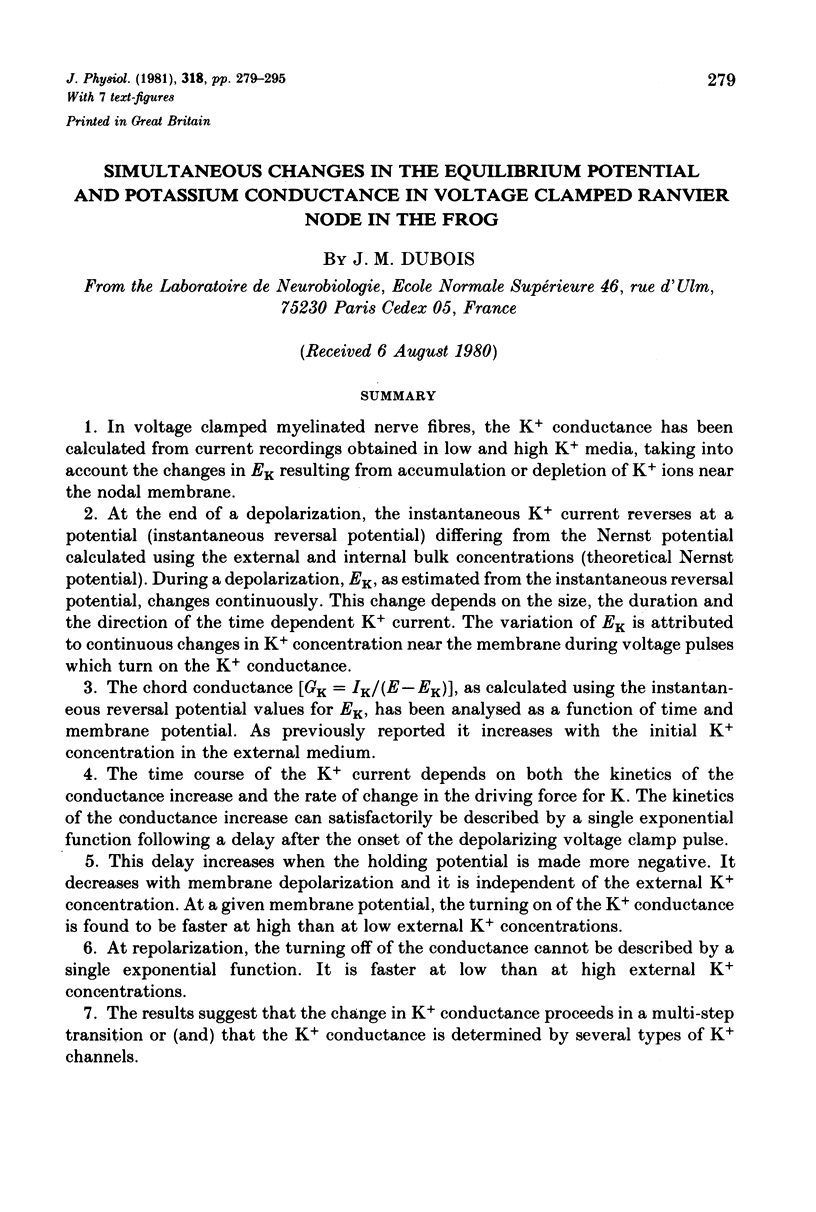
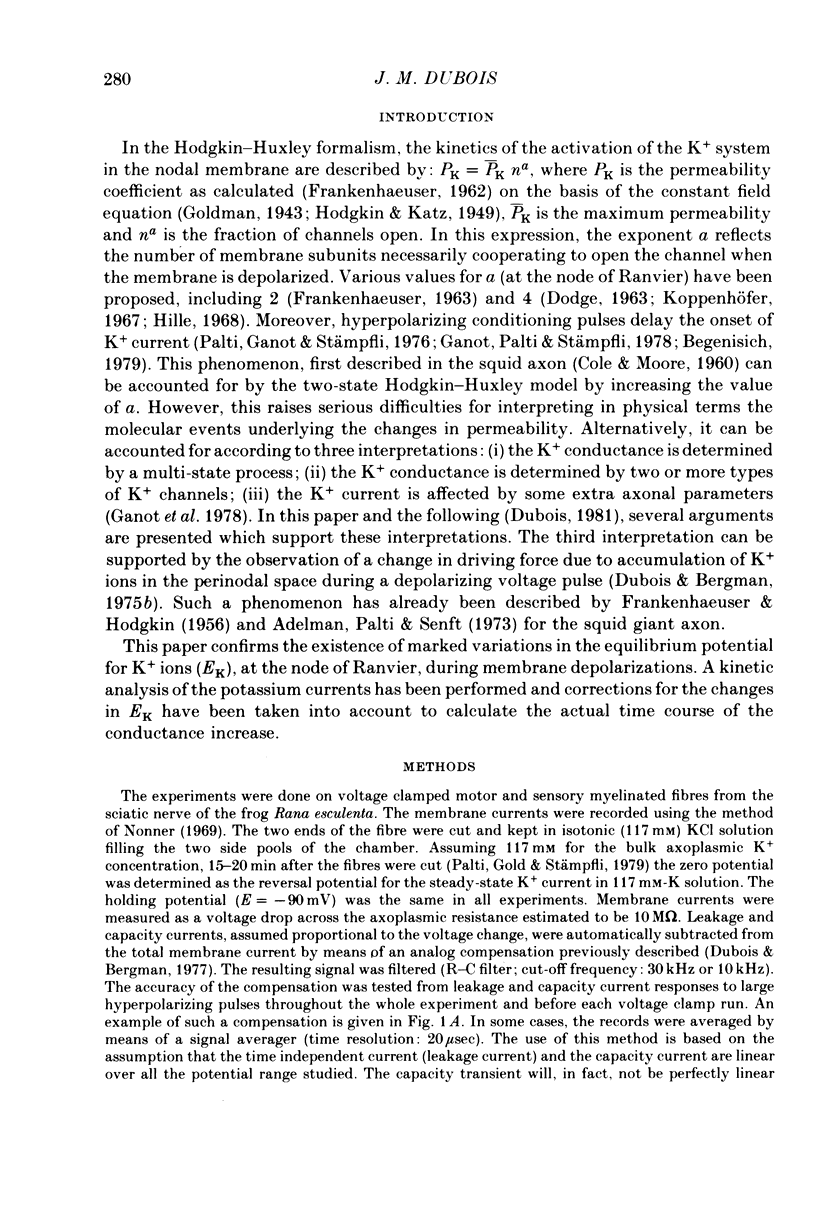
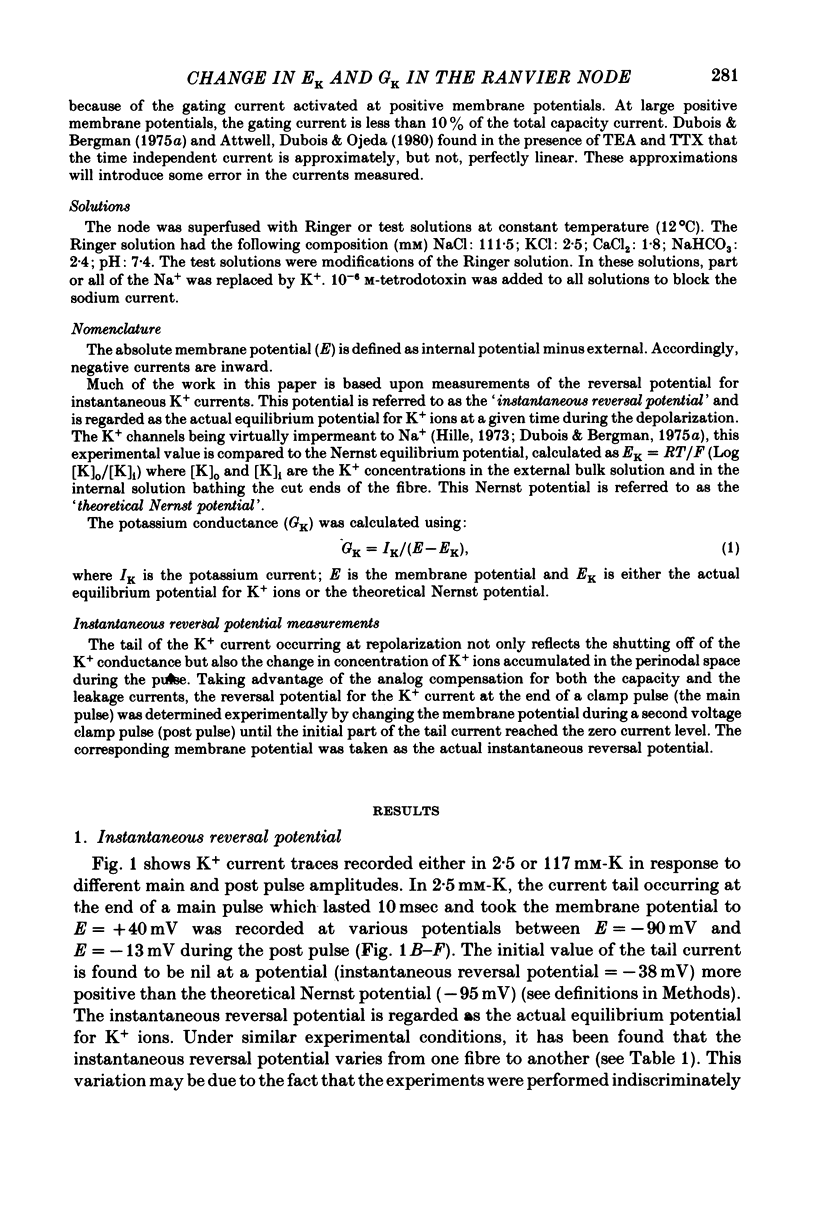
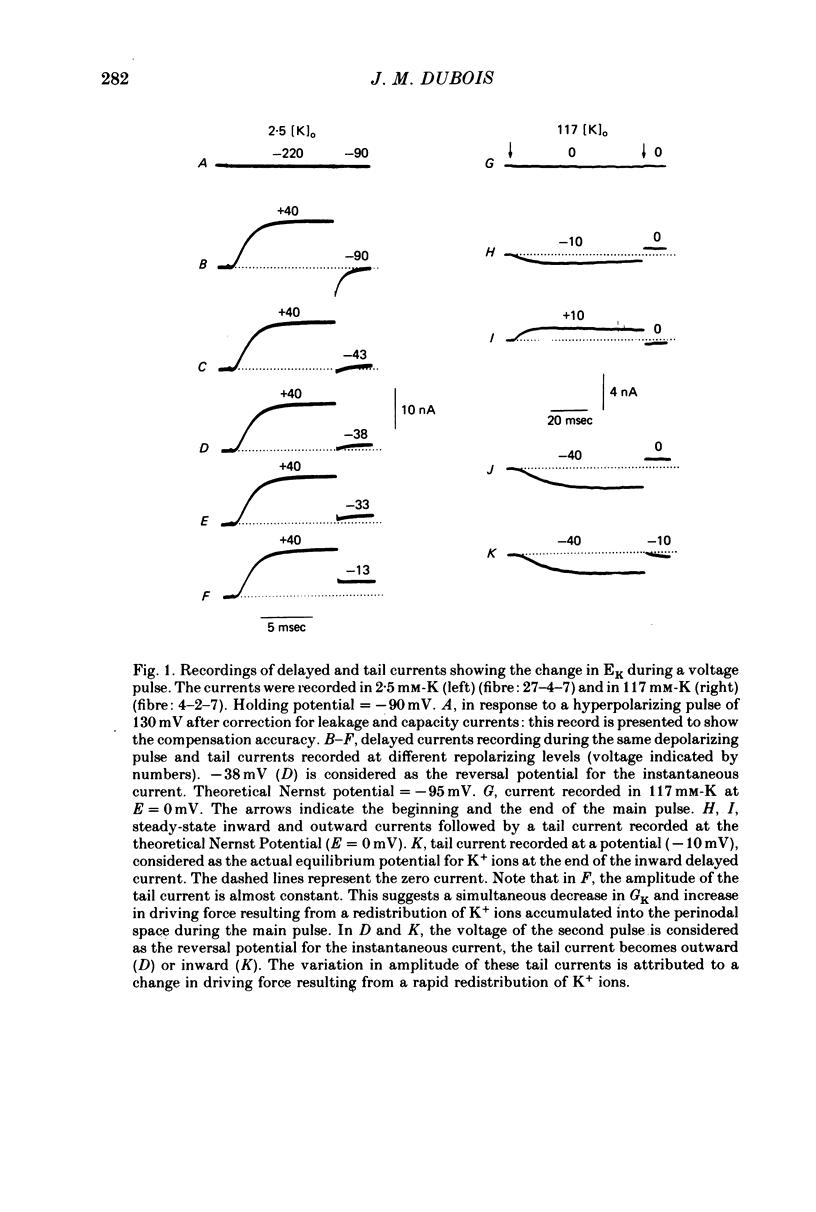
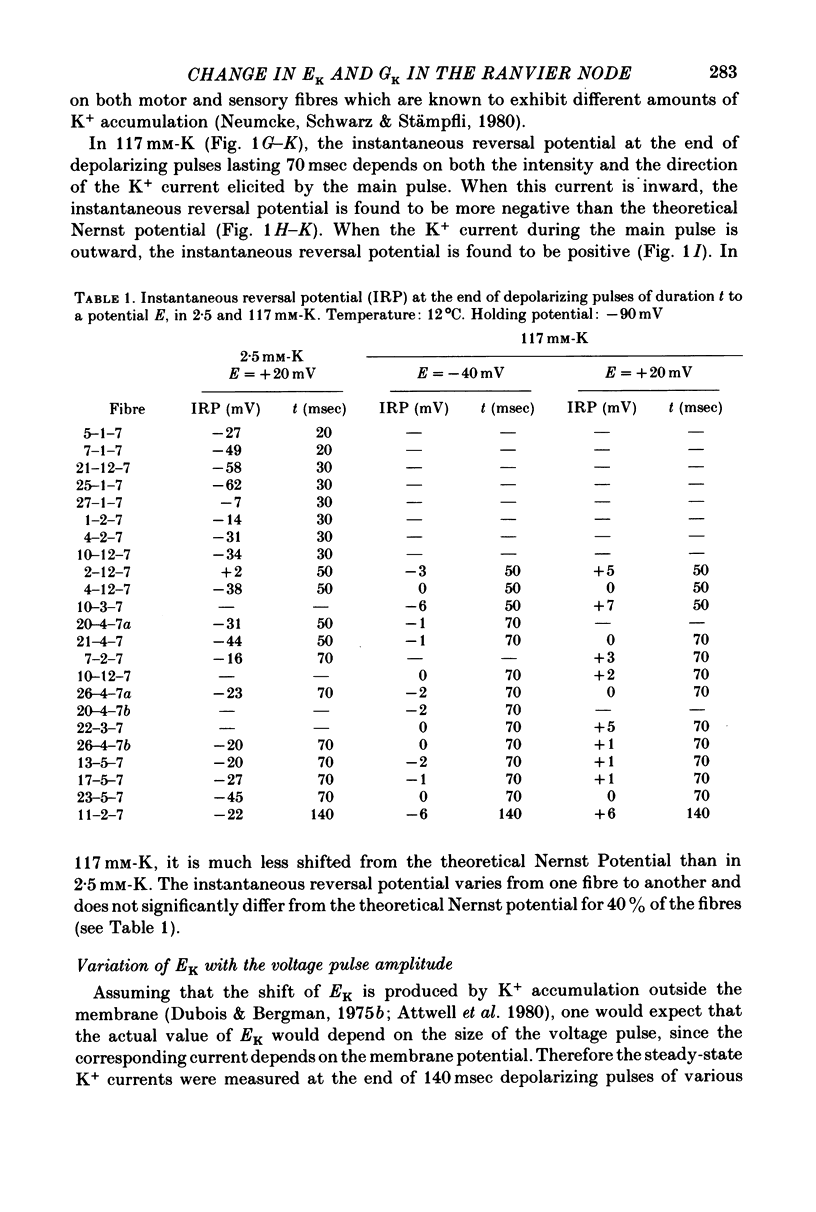
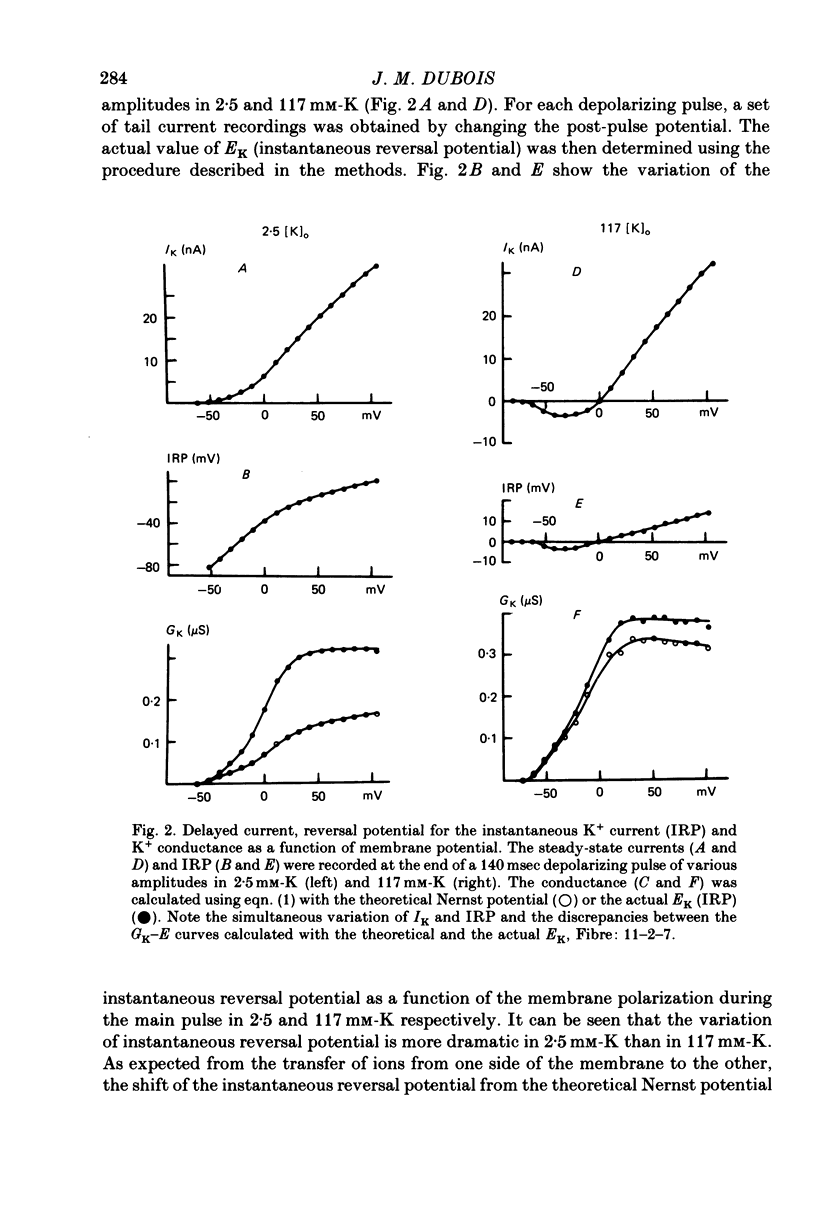
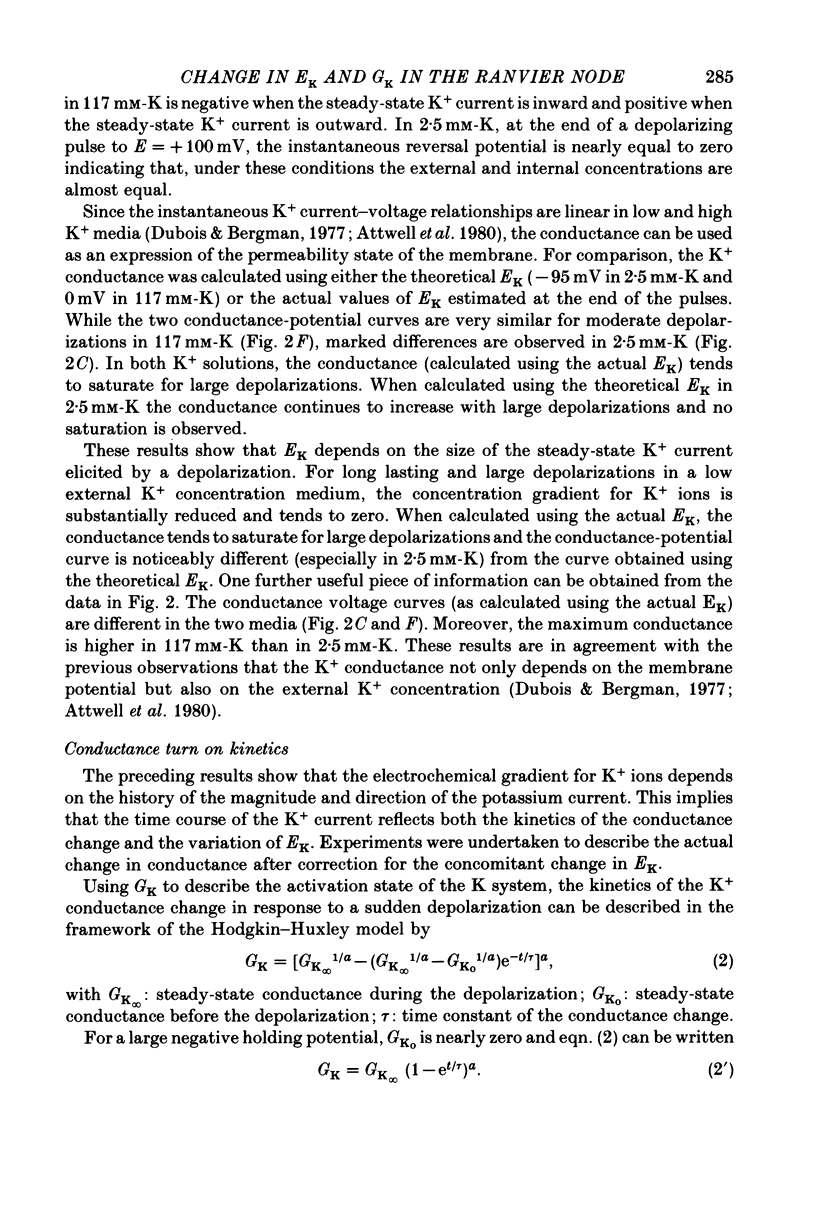
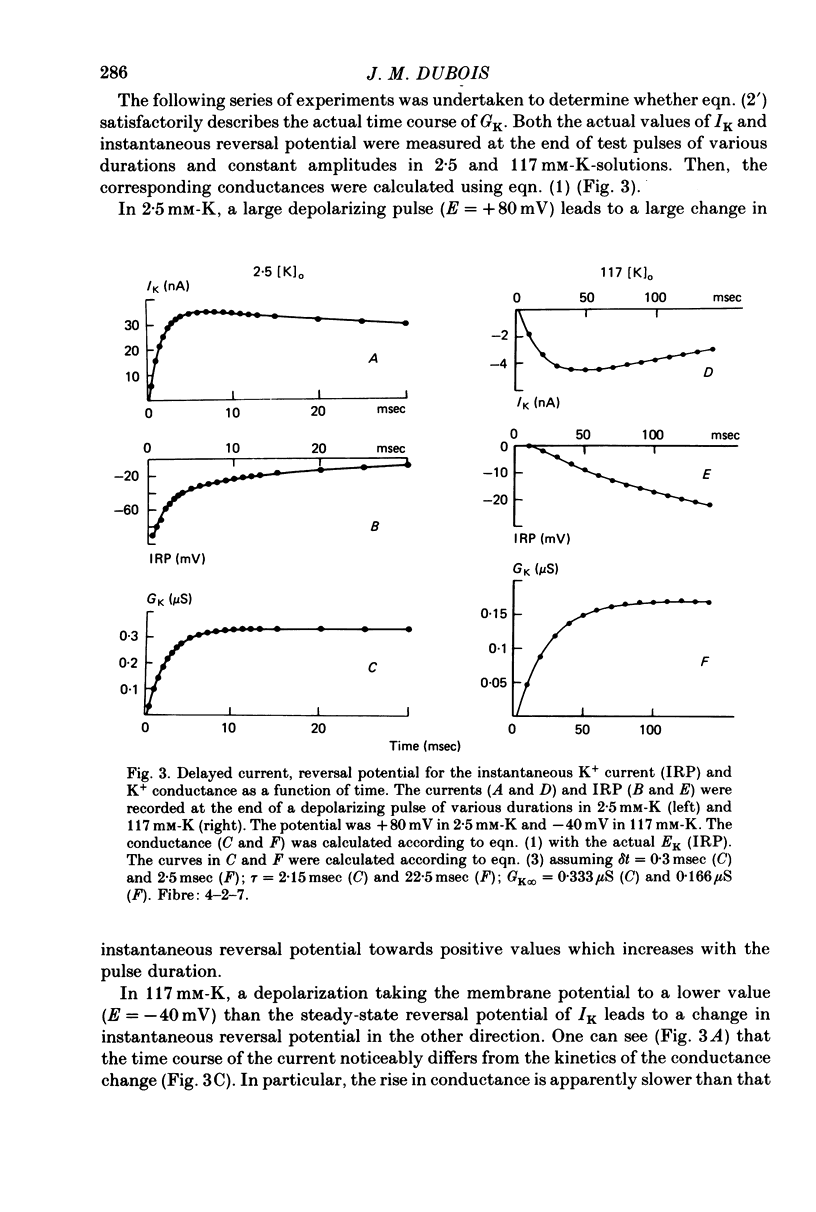
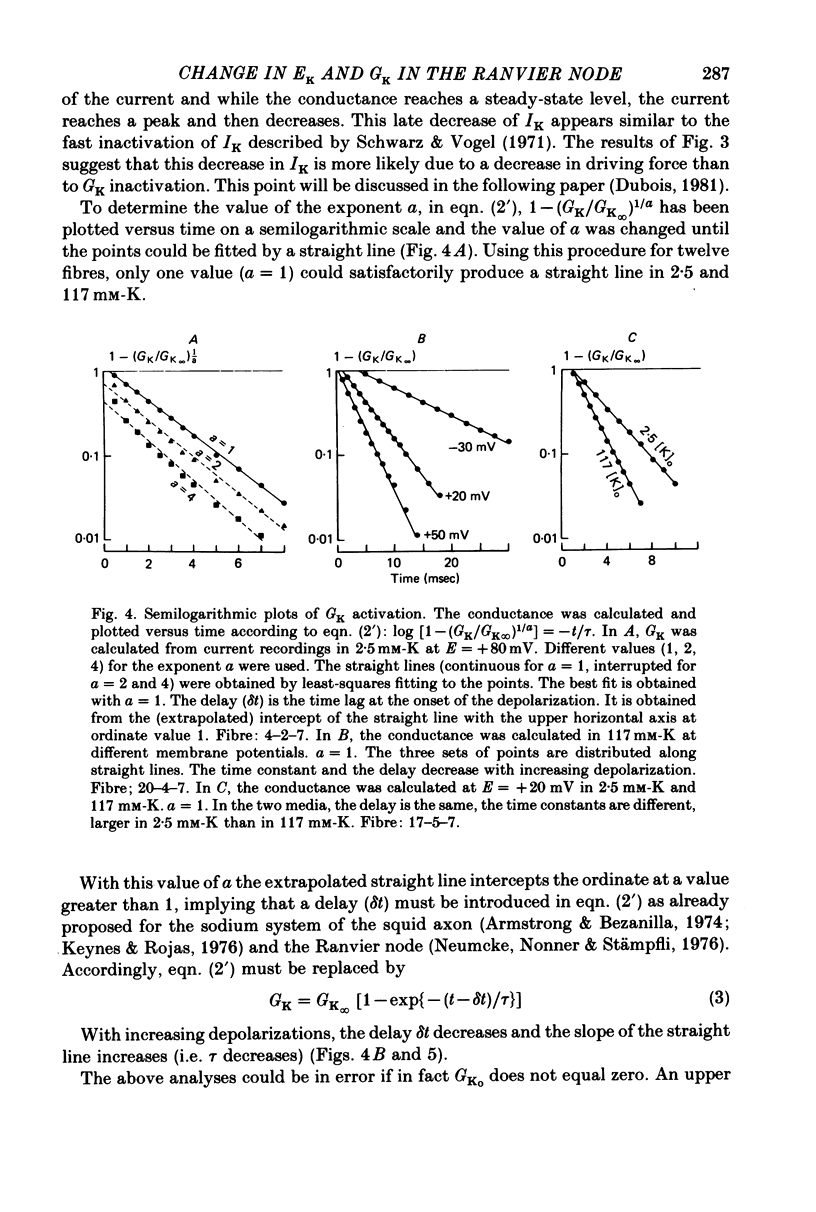
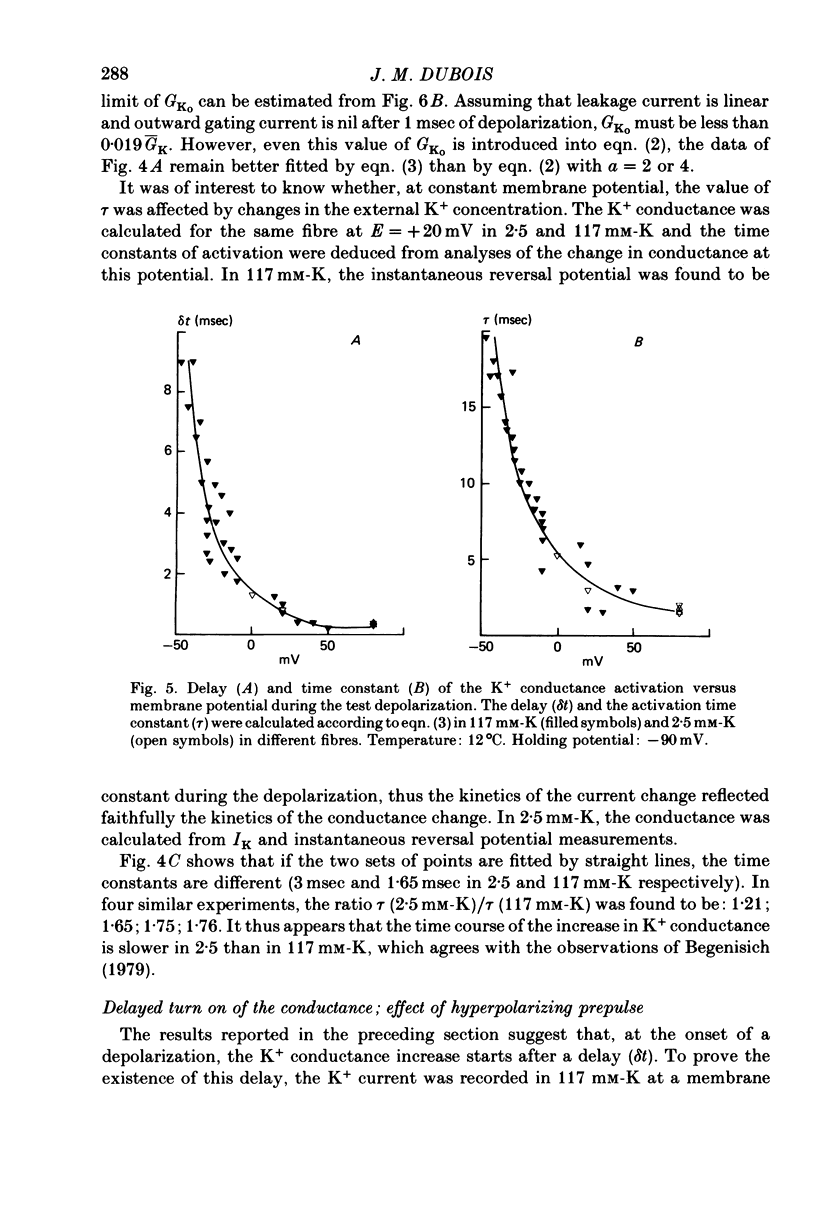
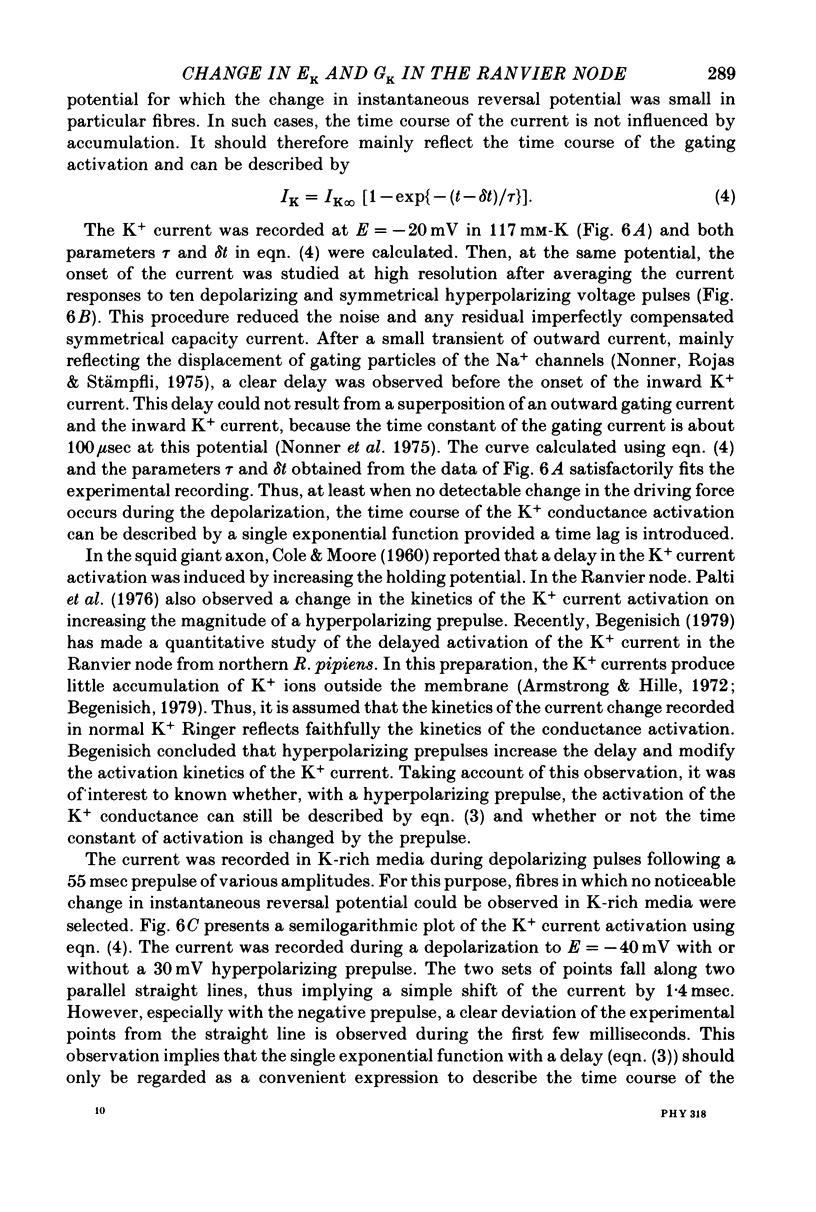
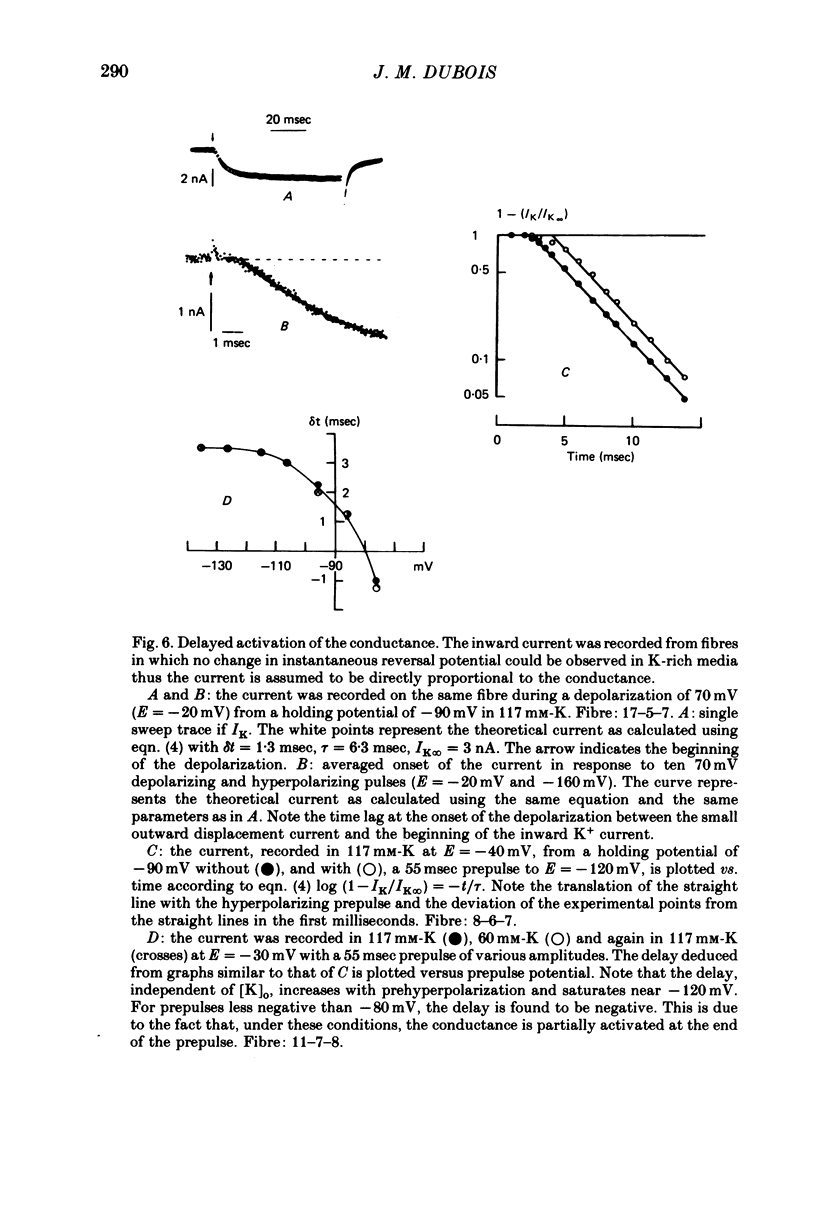
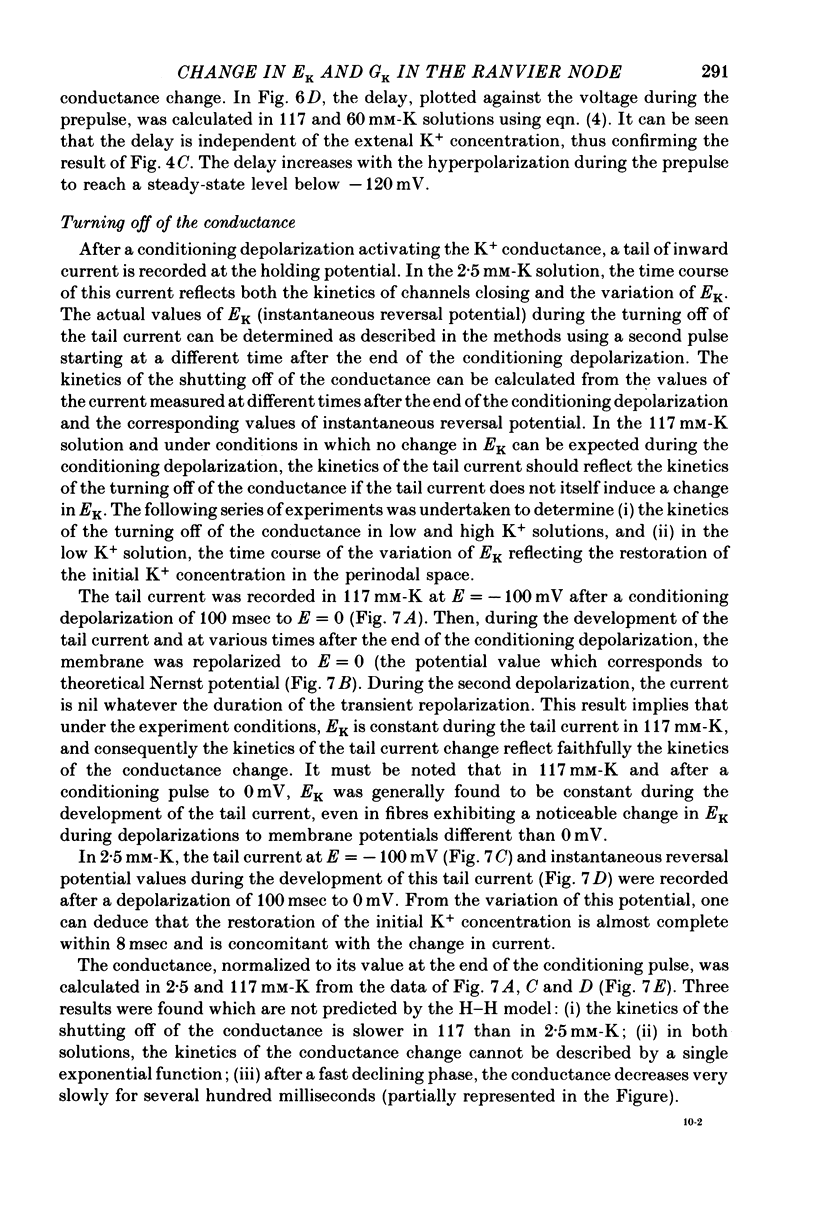
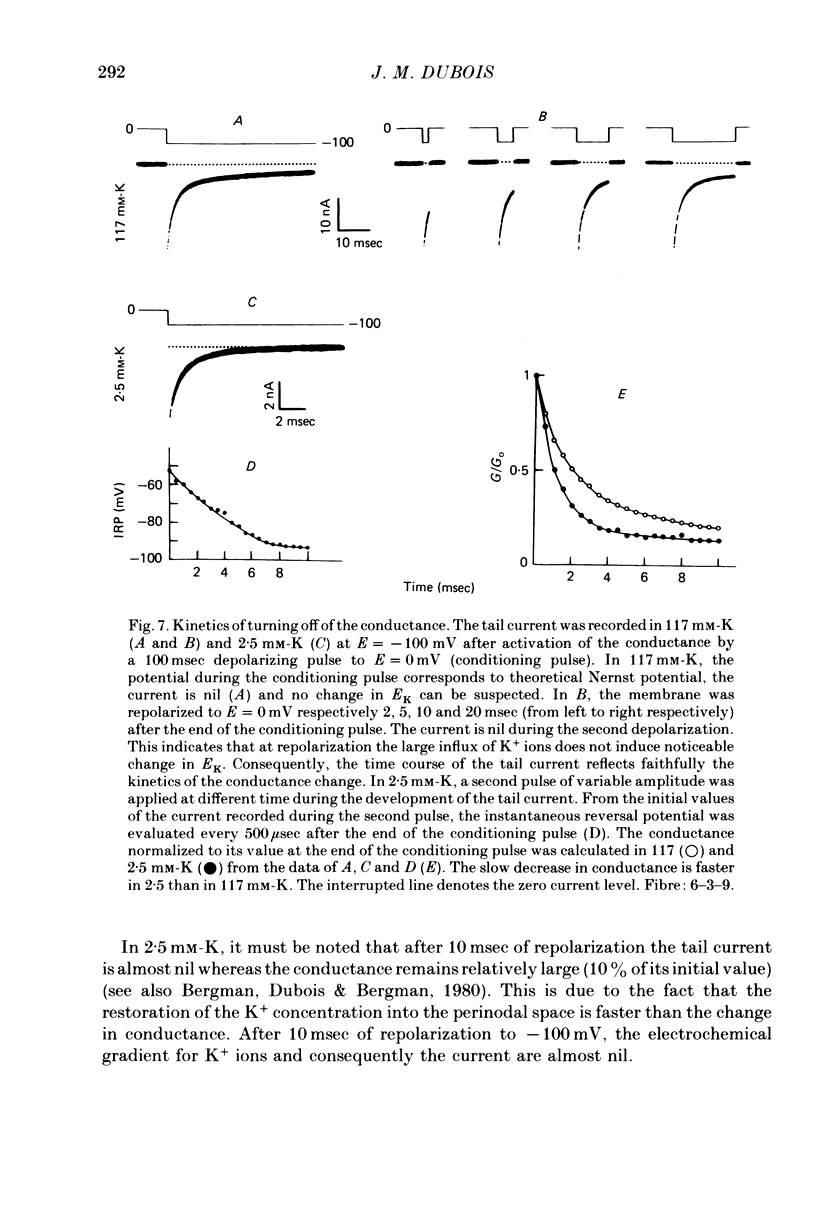
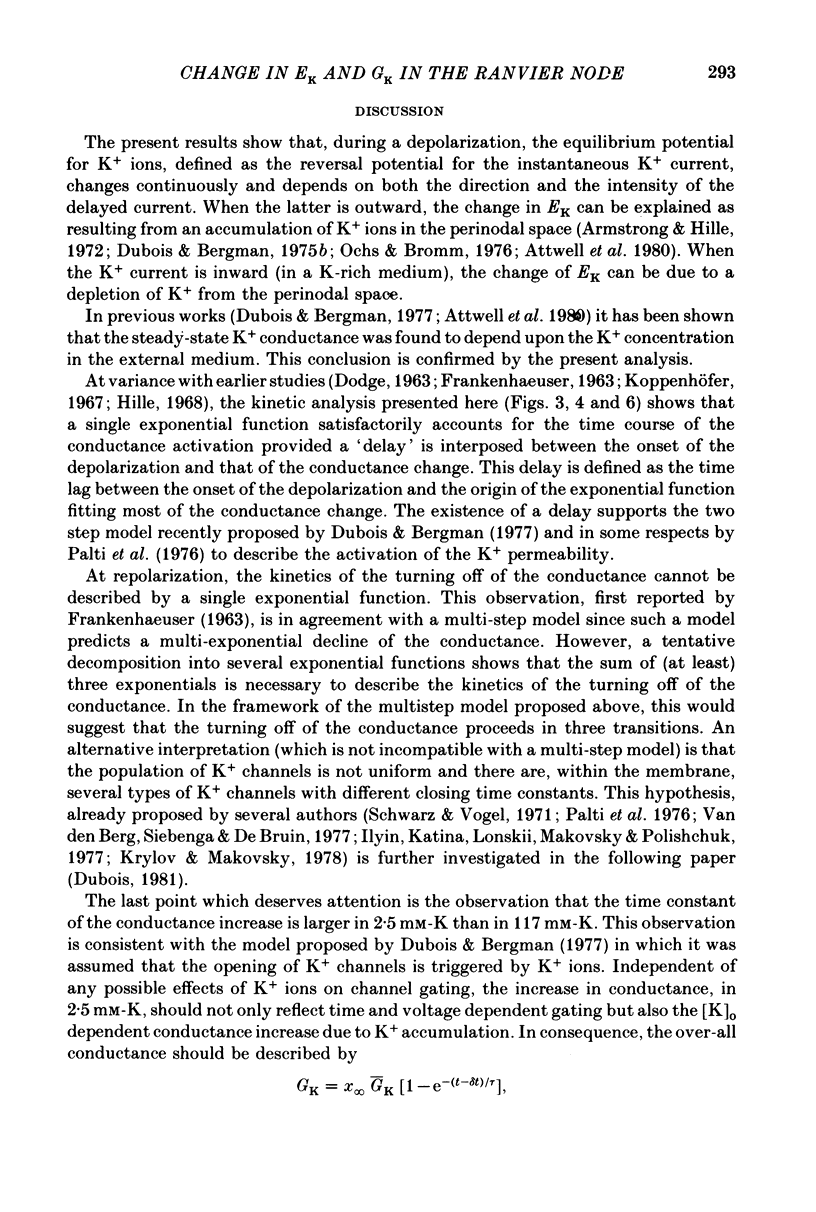
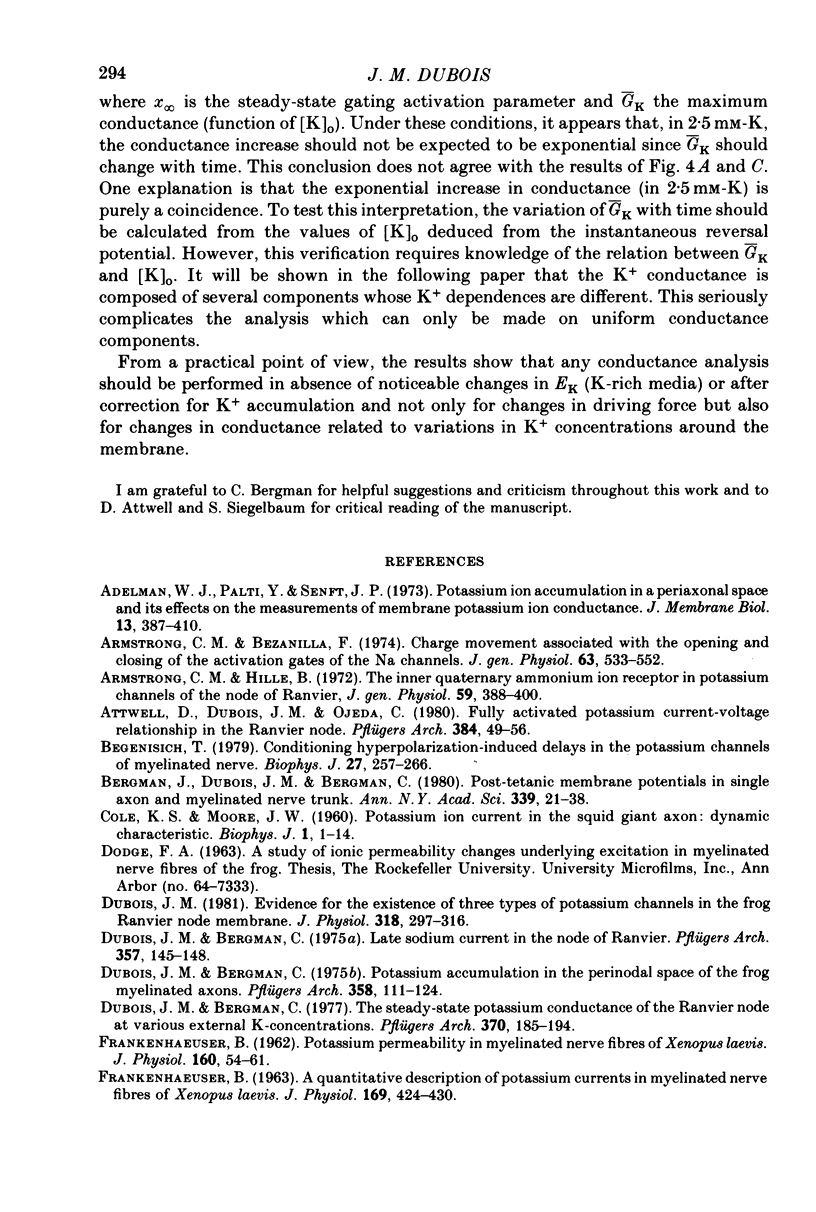
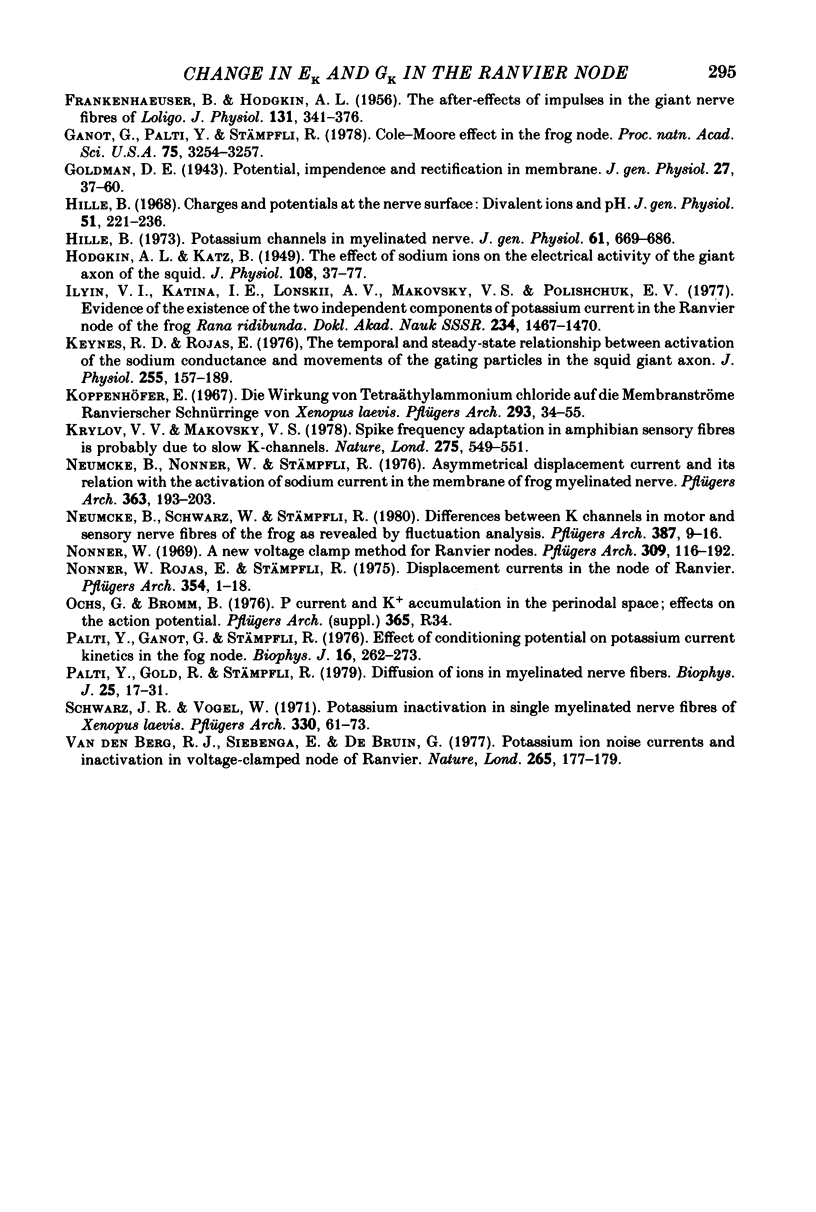
Selected References
These references are in PubMed. This may not be the complete list of references from this article.
- Adelman W. J., Jr, Palti Y., Senft J. P. Potassium ion accumulation in a periaxonal space and its effect on the measurement of membrane potassium ion conductance. J Membr Biol. 1973 Nov 8;13(4):387–410. doi: 10.1007/BF01868237. [DOI] [PubMed] [Google Scholar]
- Armstrong C. M., Bezanilla F. Charge movement associated with the opening and closing of the activation gates of the Na channels. J Gen Physiol. 1974 May;63(5):533–552. doi: 10.1085/jgp.63.5.533. [DOI] [PMC free article] [PubMed] [Google Scholar]
- Armstrong C. M., Hille B. The inner quaternary ammonium ion receptor in potassium channels of the node of Ranvier. J Gen Physiol. 1972 Apr;59(4):388–400. doi: 10.1085/jgp.59.4.388. [DOI] [PMC free article] [PubMed] [Google Scholar]
- Attwell D., Dubois J. M., Ojeda C. Fully activated potassium current-voltage relationship in the Ranvier node: discrepancy between the results of two methods of analysis. Pflugers Arch. 1980 Mar;384(1):49–56. doi: 10.1007/BF00589513. [DOI] [PubMed] [Google Scholar]
- Begenisich T. Conditioning hyperpolarization-induced delays in the potassium channels of myelinated nerve. Biophys J. 1979 Aug;27(2):257–265. doi: 10.1016/S0006-3495(79)85215-7. [DOI] [PMC free article] [PubMed] [Google Scholar]
- Bergman J., Dubois J. M., Bergman C. Post-tetanic membrane potential in single axon and myelinated nerve trunk. Ann N Y Acad Sci. 1980;339:21–38. doi: 10.1111/j.1749-6632.1980.tb15965.x. [DOI] [PubMed] [Google Scholar]
- COLE K. S., MOORE J. W. Potassium ion current in the squid giant axon: dynamic characteristic. Biophys J. 1960 Sep;1:1–14. doi: 10.1016/s0006-3495(60)86871-3. [DOI] [PMC free article] [PubMed] [Google Scholar]
- Dubois J. M., Bergman C. Late sodium current in the node of Ranvier. Pflugers Arch. 1975;357(1-2):145–148. doi: 10.1007/BF00584552. [DOI] [PubMed] [Google Scholar]
- Dubois J. M., Bergman C. Potassium accumulation in the perinodal space of frog myelinated axons. Pflugers Arch. 1975 Jul 21;358(2):111–124. doi: 10.1007/BF00583922. [DOI] [PubMed] [Google Scholar]
- Dubois J. M., Bergman C. The steady-state potassium conductance of the Ranvier node at various external K-concentrations. Pflugers Arch. 1977 Aug 29;370(2):185–194. doi: 10.1007/BF00581693. [DOI] [PubMed] [Google Scholar]
- Dubois J. M. Evidence for the existence of three types of potassium channels in the frog Ranvier node membrane. J Physiol. 1981 Sep;318:297–316. doi: 10.1113/jphysiol.1981.sp013865. [DOI] [PMC free article] [PubMed] [Google Scholar]
- FRANKENHAEUSER B. A QUANTITATIVE DESCRIPTION OF POTASSIUM CURRENTS IN MYELINATED NERVE FIBRES OF XENOPUS LAEVIS. J Physiol. 1963 Nov;169:424–430. doi: 10.1113/jphysiol.1963.sp007268. [DOI] [PMC free article] [PubMed] [Google Scholar]
- FRANKENHAEUSER B., HODGKIN A. L. The after-effects of impulses in the giant nerve fibres of Loligo. J Physiol. 1956 Feb 28;131(2):341–376. doi: 10.1113/jphysiol.1956.sp005467. [DOI] [PMC free article] [PubMed] [Google Scholar]
- FRANKENHAEUSER B. Potassium permeability in myelinated nerve fibres of Xenopus laevis. J Physiol. 1962 Jan;160:54–61. doi: 10.1113/jphysiol.1962.sp006834. [DOI] [PMC free article] [PubMed] [Google Scholar]
- Ganot G., Palti Y., Staempfli R. Cole-Moore effect in the frog node. Proc Natl Acad Sci U S A. 1978 Jul;75(7):3254–3257. doi: 10.1073/pnas.75.7.3254. [DOI] [PMC free article] [PubMed] [Google Scholar]
- HODGKIN A. L., KATZ B. The effect of sodium ions on the electrical activity of giant axon of the squid. J Physiol. 1949 Mar 1;108(1):37–77. doi: 10.1113/jphysiol.1949.sp004310. [DOI] [PMC free article] [PubMed] [Google Scholar]
- Hille B. Charges and potentials at the nerve surface. Divalent ions and pH. J Gen Physiol. 1968 Feb;51(2):221–236. doi: 10.1085/jgp.51.2.221. [DOI] [PMC free article] [PubMed] [Google Scholar]
- Hille B. Potassium channels in myelinated nerve. Selective permeability to small cations. J Gen Physiol. 1973 Jun;61(6):669–686. doi: 10.1085/jgp.61.6.669. [DOI] [PMC free article] [PubMed] [Google Scholar]
- Il'in V. I., Katina I. E., Lonskii A. V., Makovskii V. S., Polishchuk E. V. Dannye o sushchestvovanii dvukh nezavisimykh komponent kalievogo toka v membrane perekhvata Ranv'e liagushki Rana ridibunda. Dokl Akad Nauk SSSR. 1977;234(6):1467–1470. [PubMed] [Google Scholar]
- Keynes R. D., Rojas E. The temporal and steady-state relationships between activation of the sodium conductance and movement of the gating particles in the squid giant axon. J Physiol. 1976 Feb;255(1):157–189. doi: 10.1113/jphysiol.1976.sp011274. [DOI] [PMC free article] [PubMed] [Google Scholar]
- Krylov B. V., Makovsky V. S. Spike frequency adaptation in amphibian sensory fibres is probably due to slow K channels. Nature. 1978 Oct 12;275(5680):549–551. doi: 10.1038/275549a0. [DOI] [PubMed] [Google Scholar]
- Neumcke B., Nonner W., Stämpfli R. Asymmetrical displacement current and its relation with the activation of sodium current in the membrane of frog myelinated nerve. Pflugers Arch. 1976 Jun 22;363(3):193–203. doi: 10.1007/BF00594601. [DOI] [PubMed] [Google Scholar]
- Neumcke B., Schwarz W., Stämpfli R. Differences between K channels in motor and sensory nerve fibres of the frog as revealed by fluctuation analysis. Pflugers Arch. 1980 Aug;387(1):9–16. doi: 10.1007/BF00580838. [DOI] [PubMed] [Google Scholar]
- Nonner W. A new voltage clamp method for Ranvier nodes. Pflugers Arch. 1969;309(2):176–192. doi: 10.1007/BF00586967. [DOI] [PubMed] [Google Scholar]
- Nonner W., Rojas E., Stämpfli H. Displacement currents in the node of Ranvier. Voltage and time dependence. Pflugers Arch. 1975;354(1):1–18. doi: 10.1007/BF00584499. [DOI] [PubMed] [Google Scholar]
- Palti Y., Ganot G., Stämpfli R. Effect of conditioning potential on potassium current kinetics in the frog node. Biophys J. 1976 Mar;16(3):261–273. doi: 10.1016/S0006-3495(76)85686-X. [DOI] [PMC free article] [PubMed] [Google Scholar]
- Palti Y., Gold R., Stämpfli R. Diffusion of ions in myelinated nerve fibers. Biophys J. 1979 Jan;25(1):17–31. doi: 10.1016/S0006-3495(79)85275-3. [DOI] [PMC free article] [PubMed] [Google Scholar]
- Schwarz J. R., Vogel W. Potassium inactivation in single myelinated nerve fibres of Xenopus laevis. Pflugers Arch. 1971;330(1):61–73. doi: 10.1007/BF00588735. [DOI] [PubMed] [Google Scholar]
- van den Berg R. J., Siebenga E., de Bruin G. Potassium ion noise currents and inactivation in voltage-clamped node of Ranvier. Nature. 1977 Jan 13;265(5590):177–179. doi: 10.1038/265177a0. [DOI] [PubMed] [Google Scholar]