Abstract
1. The transport of the non-metabolized sugar, 3-O-methylglucose, has been studied in the squid axon under conditions where the intracellular environment of the axon is controlled by internal dialysis. 2. Sugar transport is passive, shows saturation kinetics and is asymmetric. At 15 degrees C, the Michaelis and velocity constants for exit are approximately four times those for uptake. The asymmetry of transport is increased by raising the temperature. 3. Sugar uptake is not affected by intracellular sugar levels as high as 100 mM. Sugar exit is, however, reduced by external sugars although the apparent Km for exit is unaffected. 4. The kinetics of sugar exit under exchange conditions are determined by the kinetics of sugar uptake. These results can be accounted for by the asymmetric mobile-carrier and simultaneous-carrier models for transport. 5. Both sugar uptake and exit are reduced in the absence of ATPi. Kinetic analysis of transport under these conditions show that the capacity of the system to transport sugar is unchanged but that the affinity of the system for sugar is reduced. Internal cyclic AMP, AMP, ADP or GTP (2 mM) do not mimic this action of ATP. The hydrolysable analogue of ATP, alpha, beta-methylene-5-ATP (2 mM), (but not the nonhydrolysable analogue beta, gamma-methylene-5-ATP, 2 mM) has an ATP-like action on sugar transport. 6. Transport is unaffected by internal Ca2+ concentrations in the range 4 X 10(-8)--9 X 10(-7) M.
Full text
PDF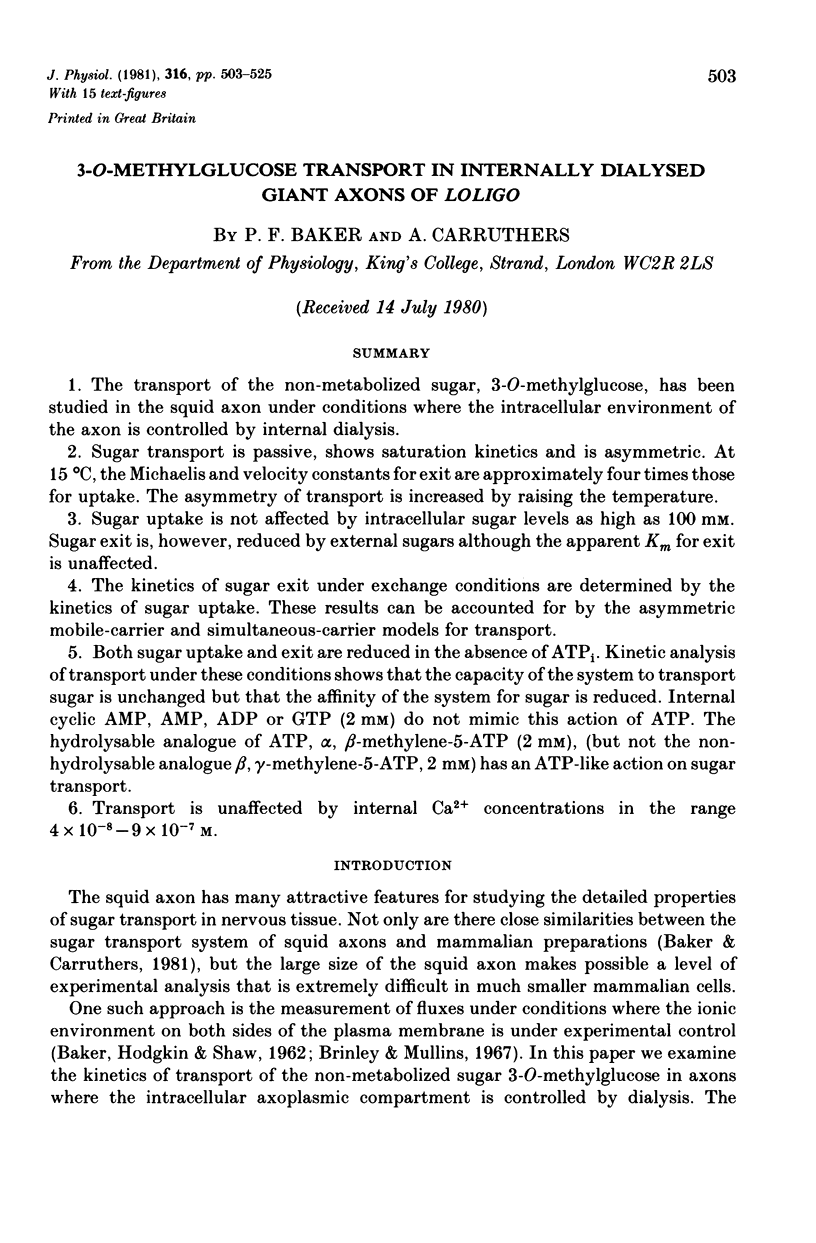
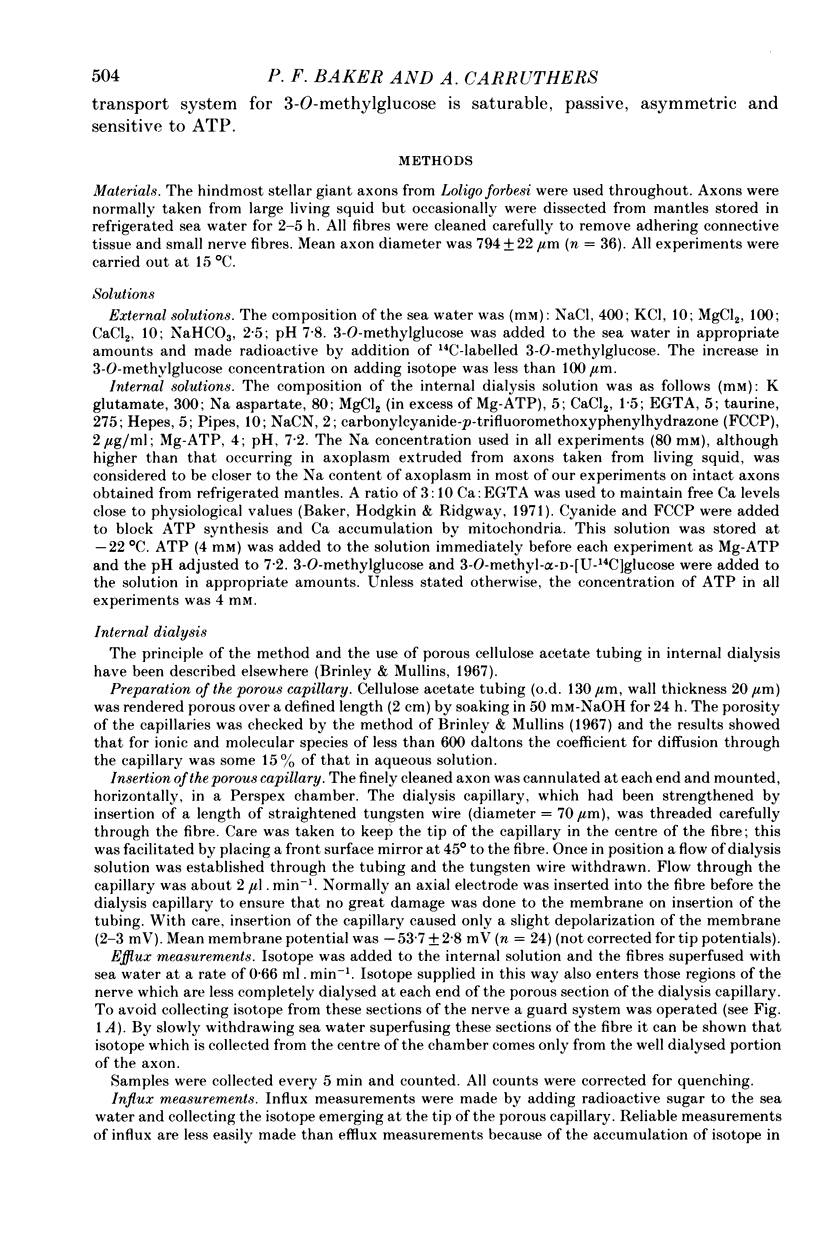
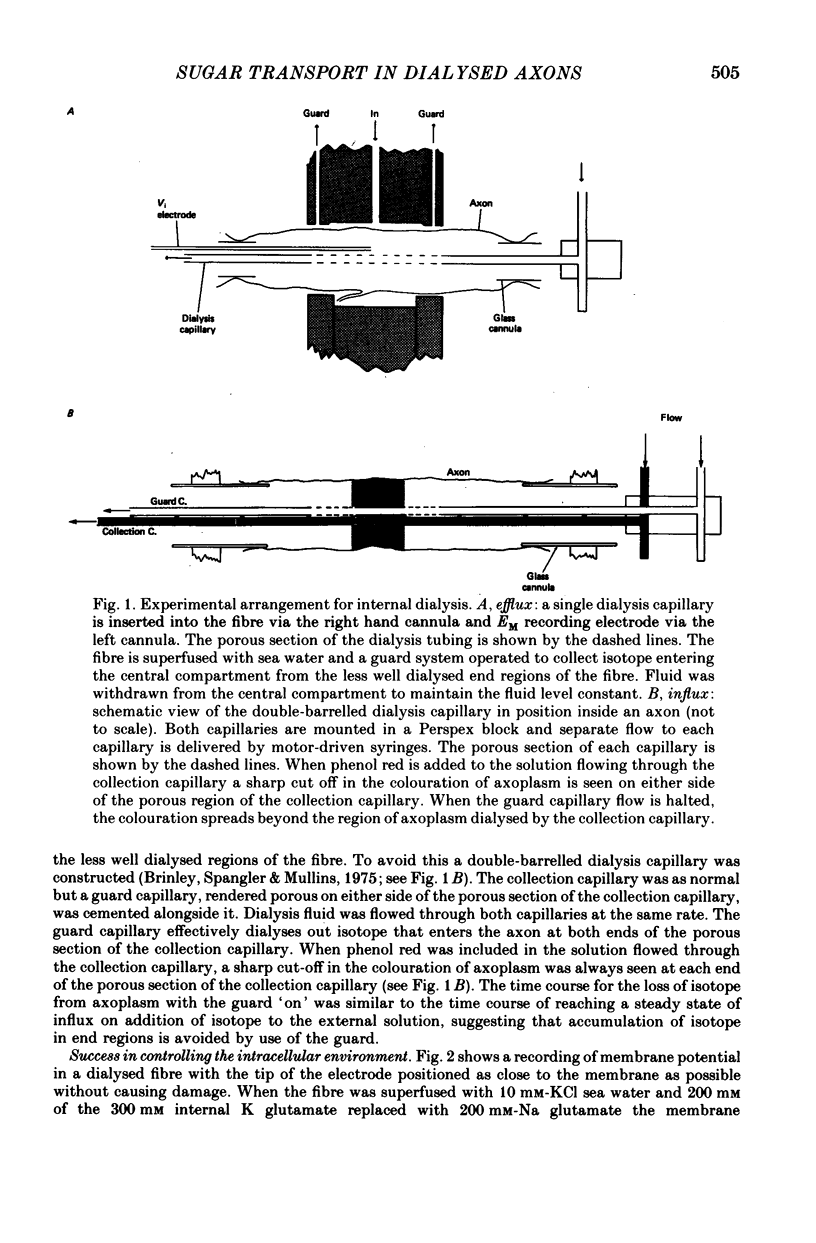
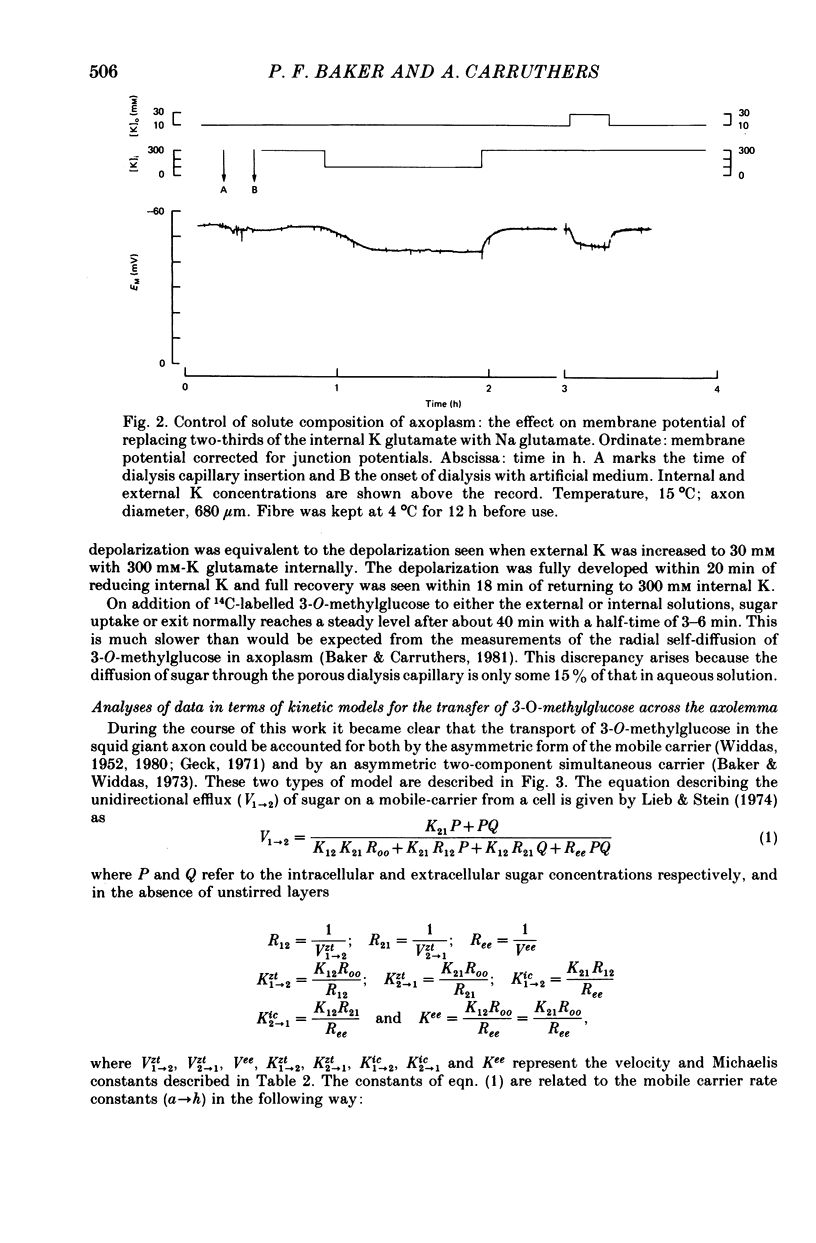
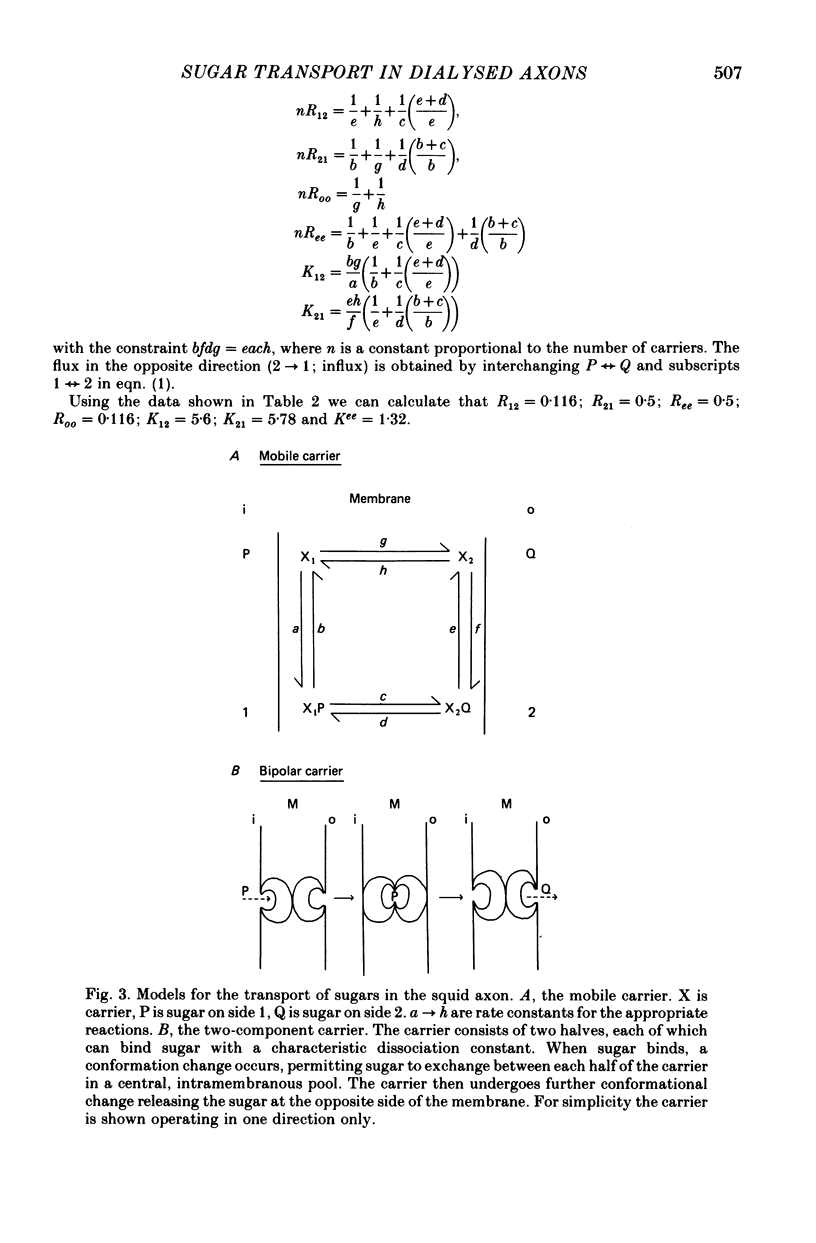
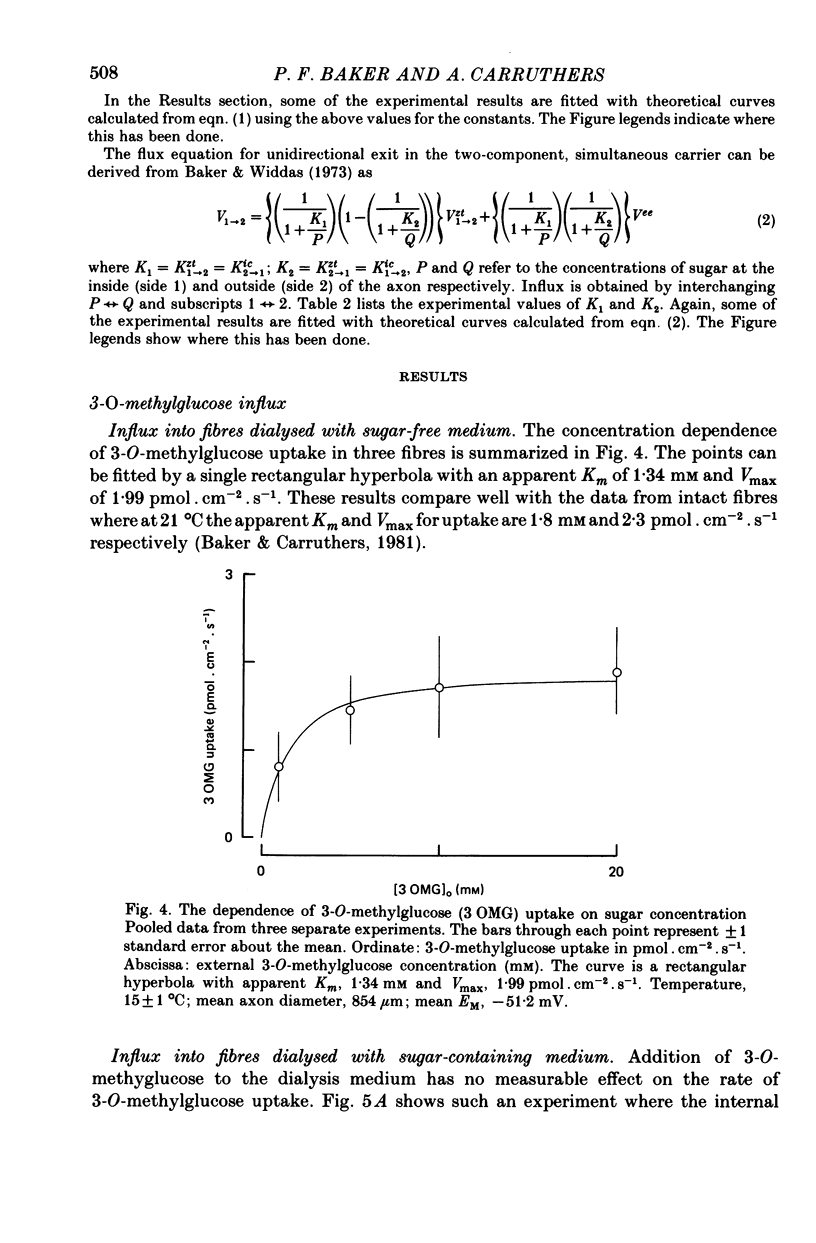
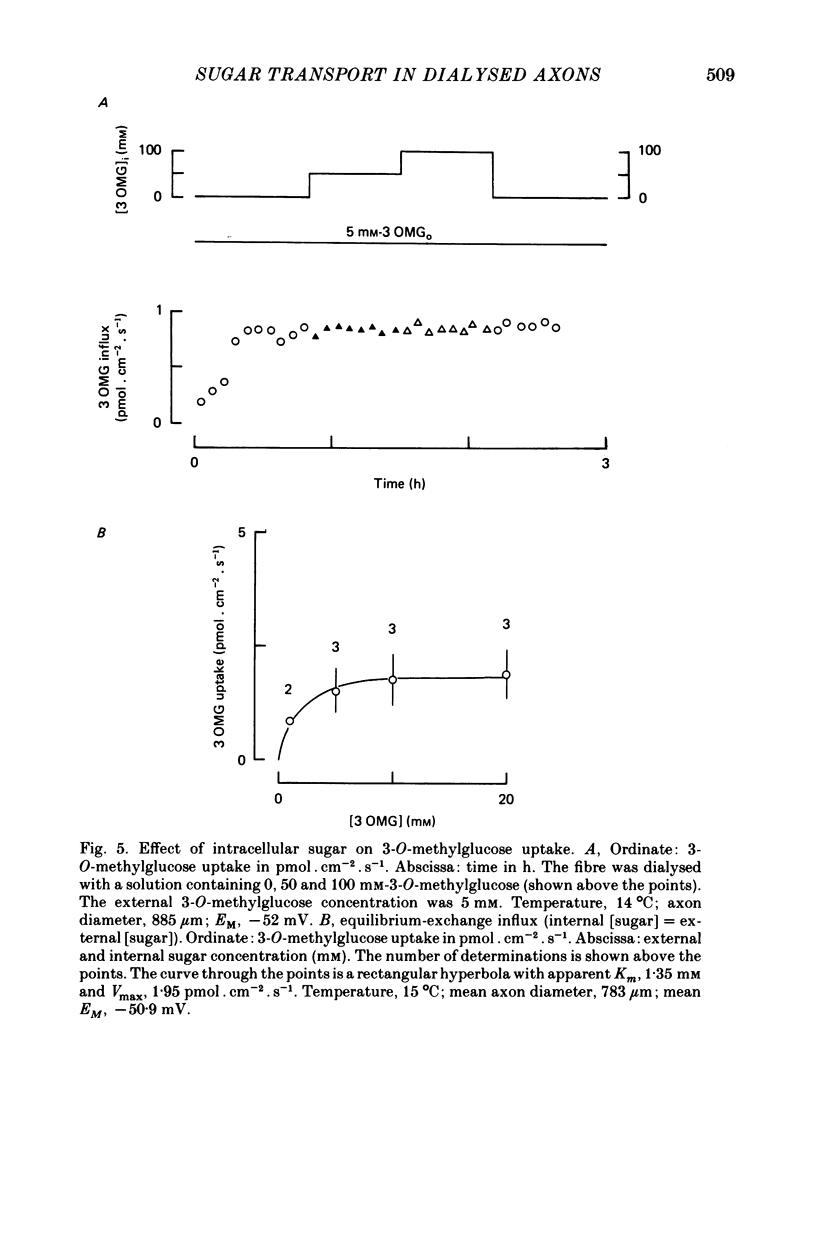
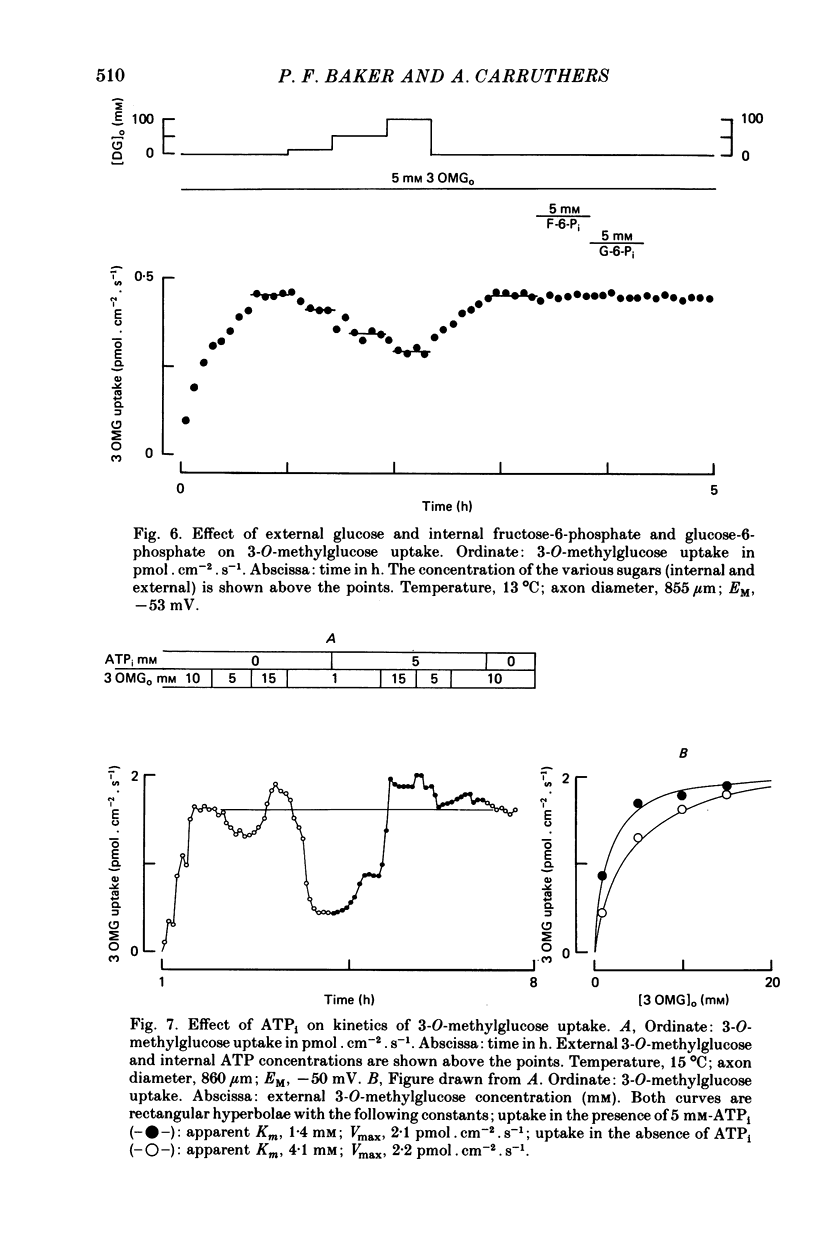
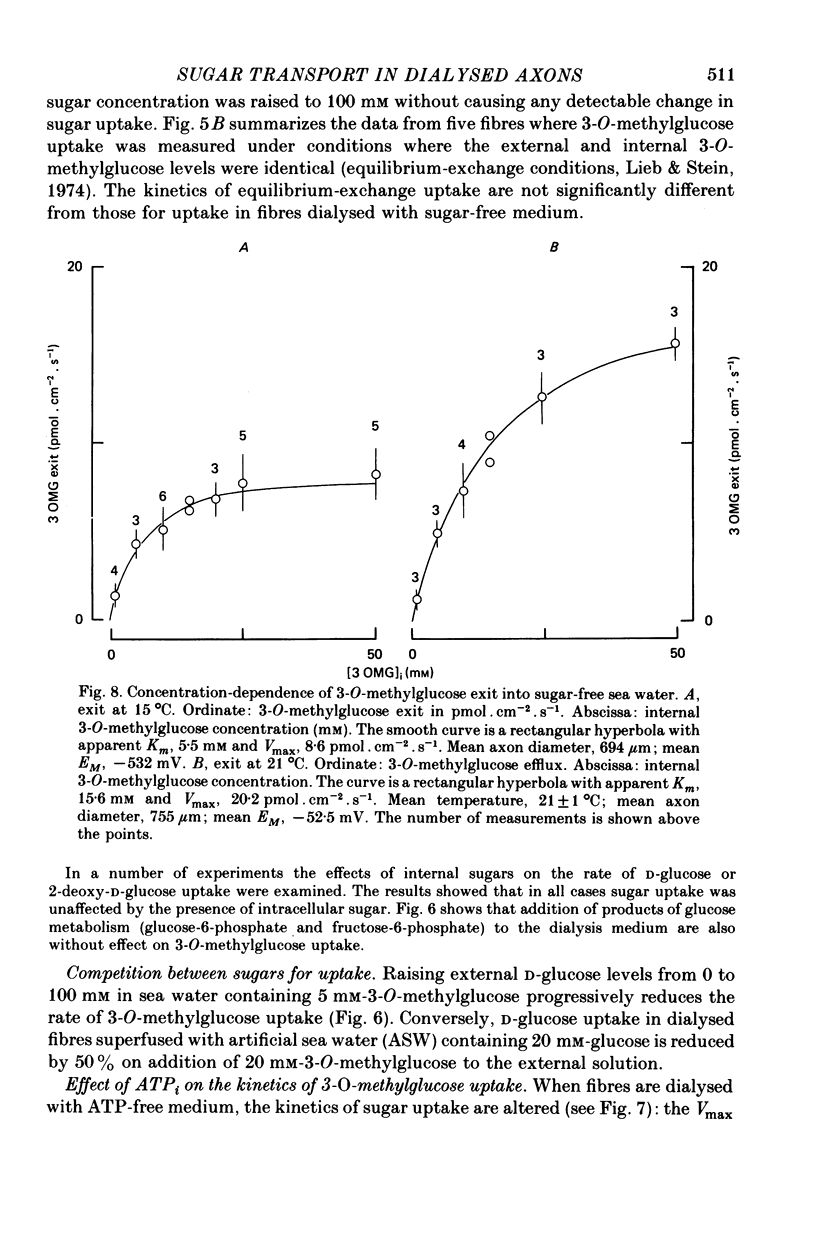
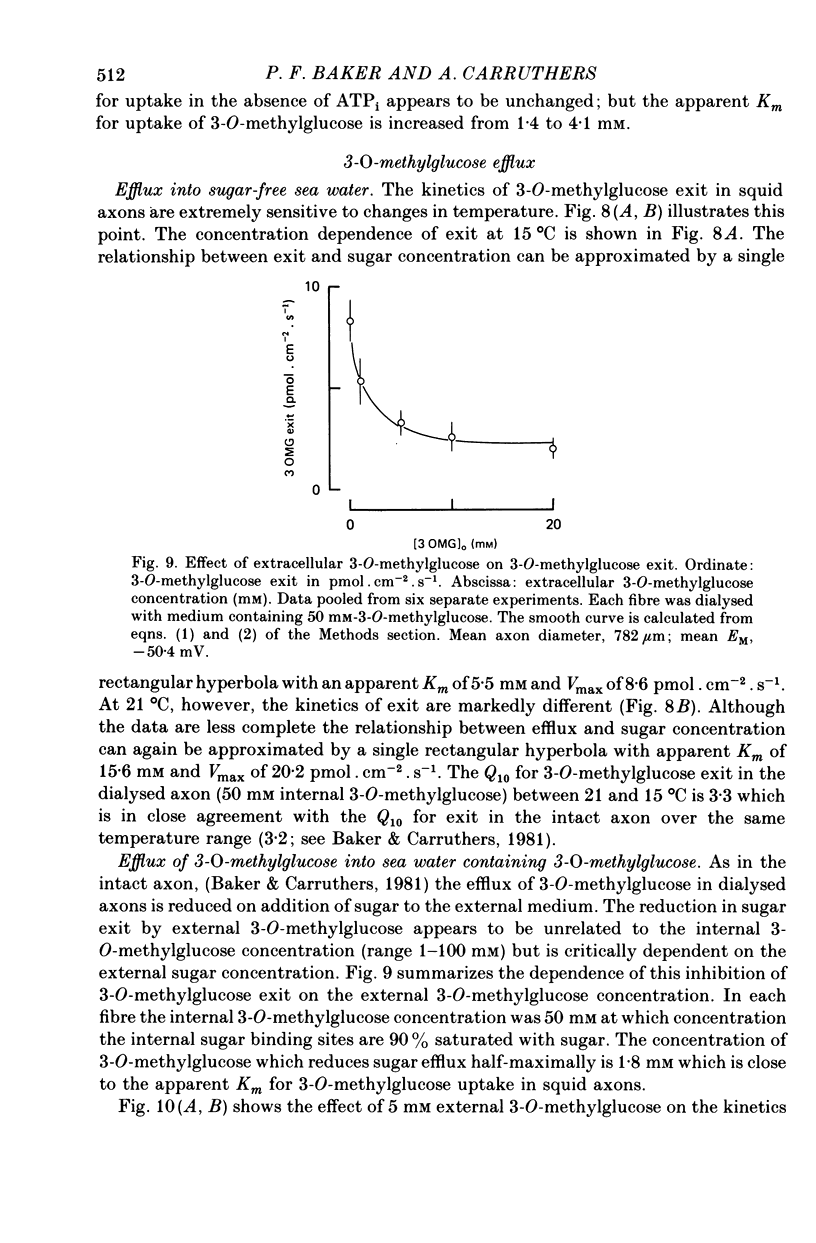
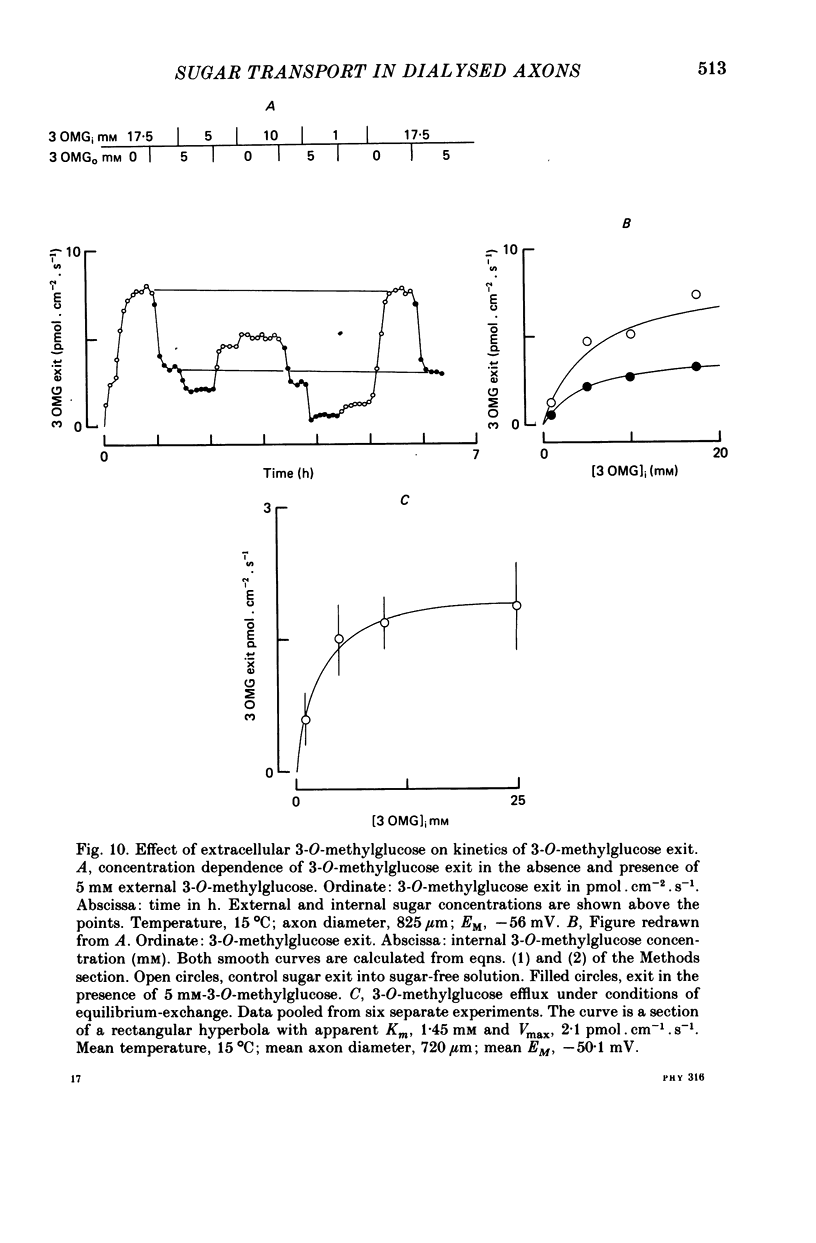
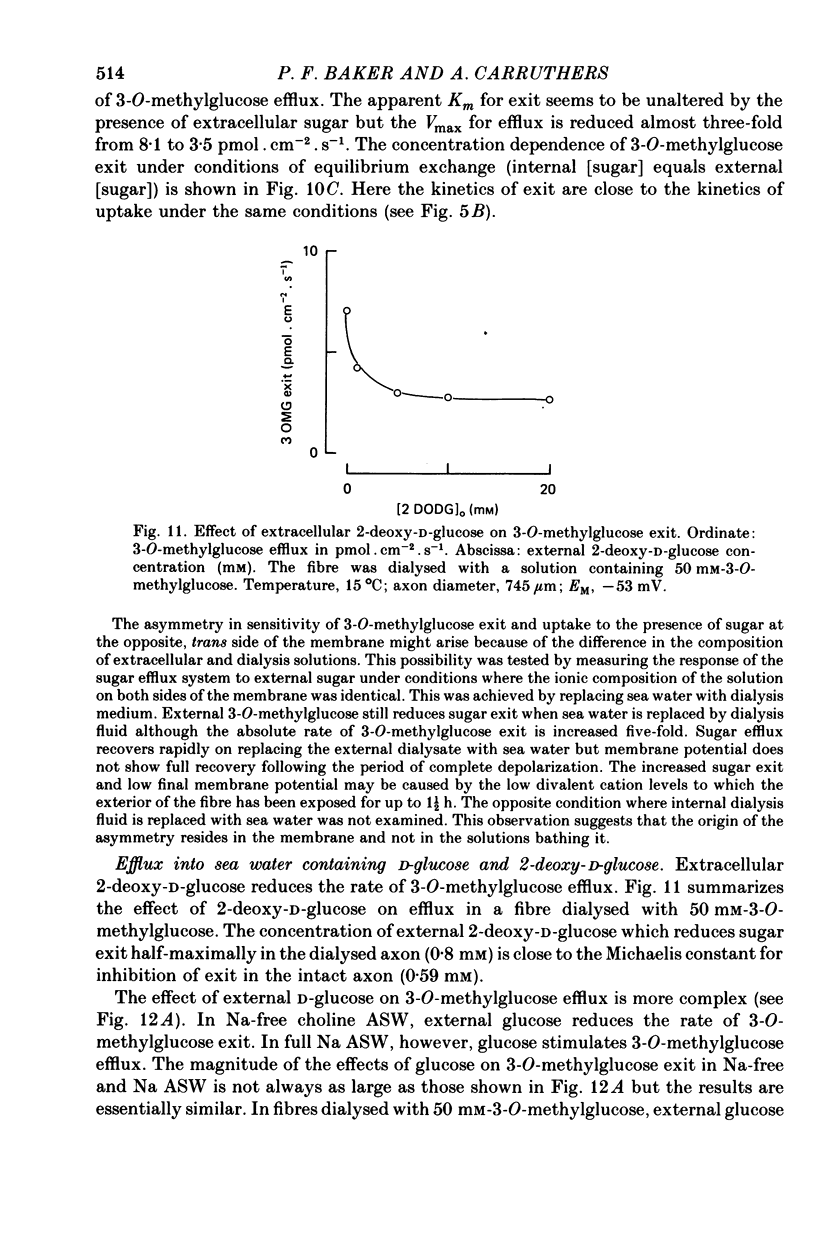
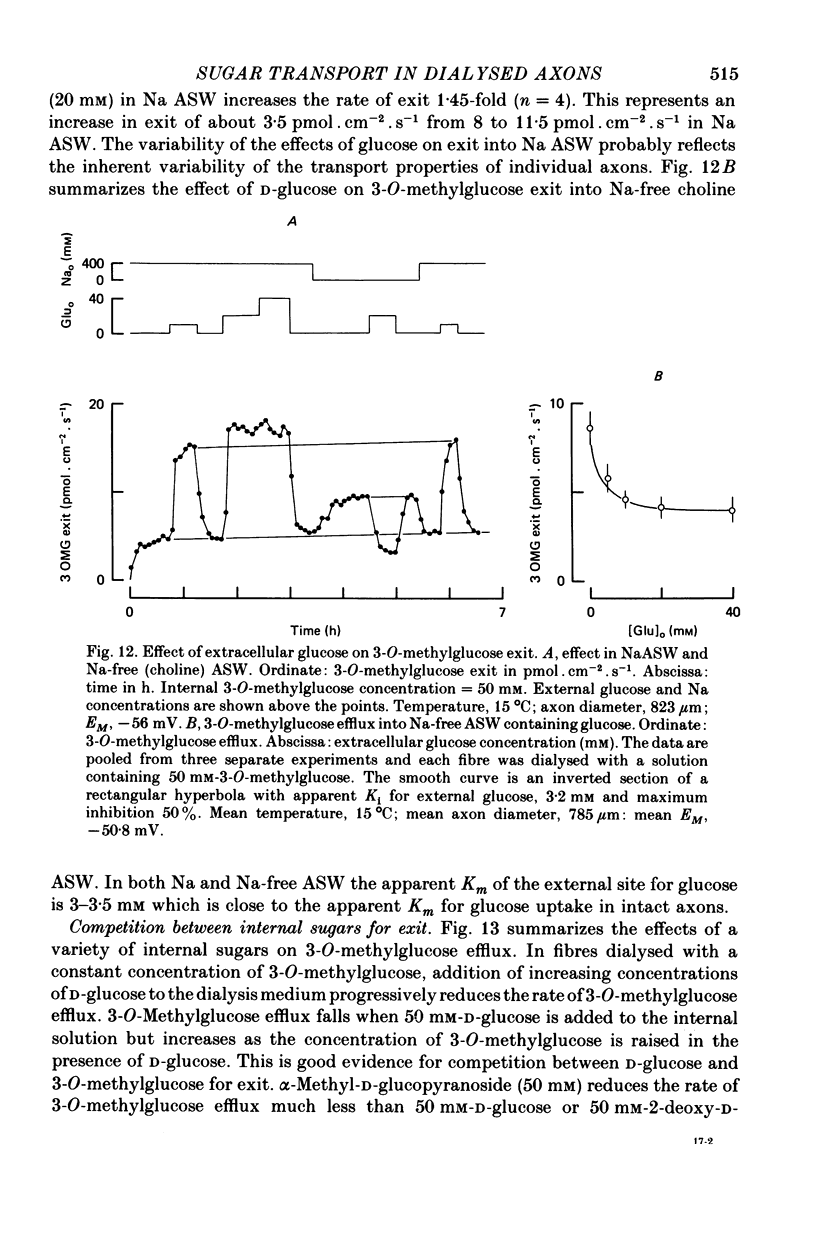
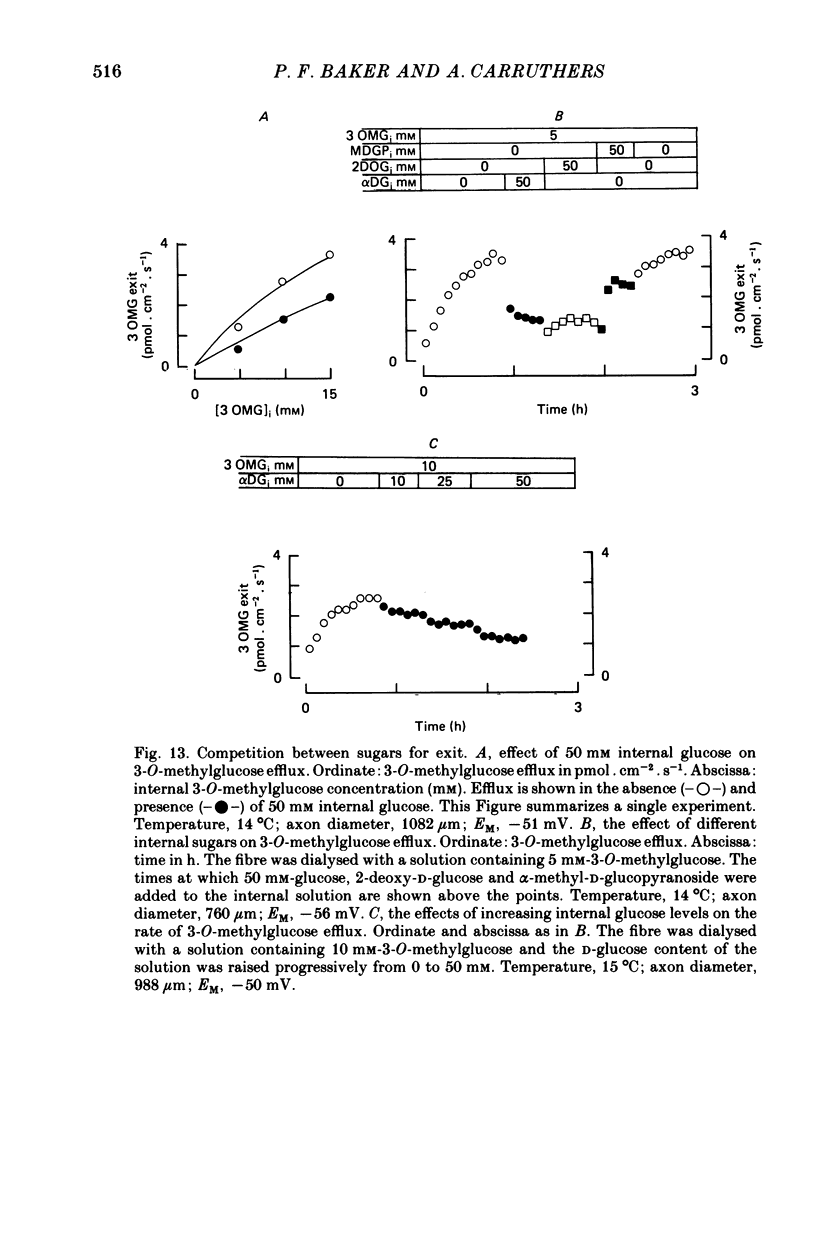
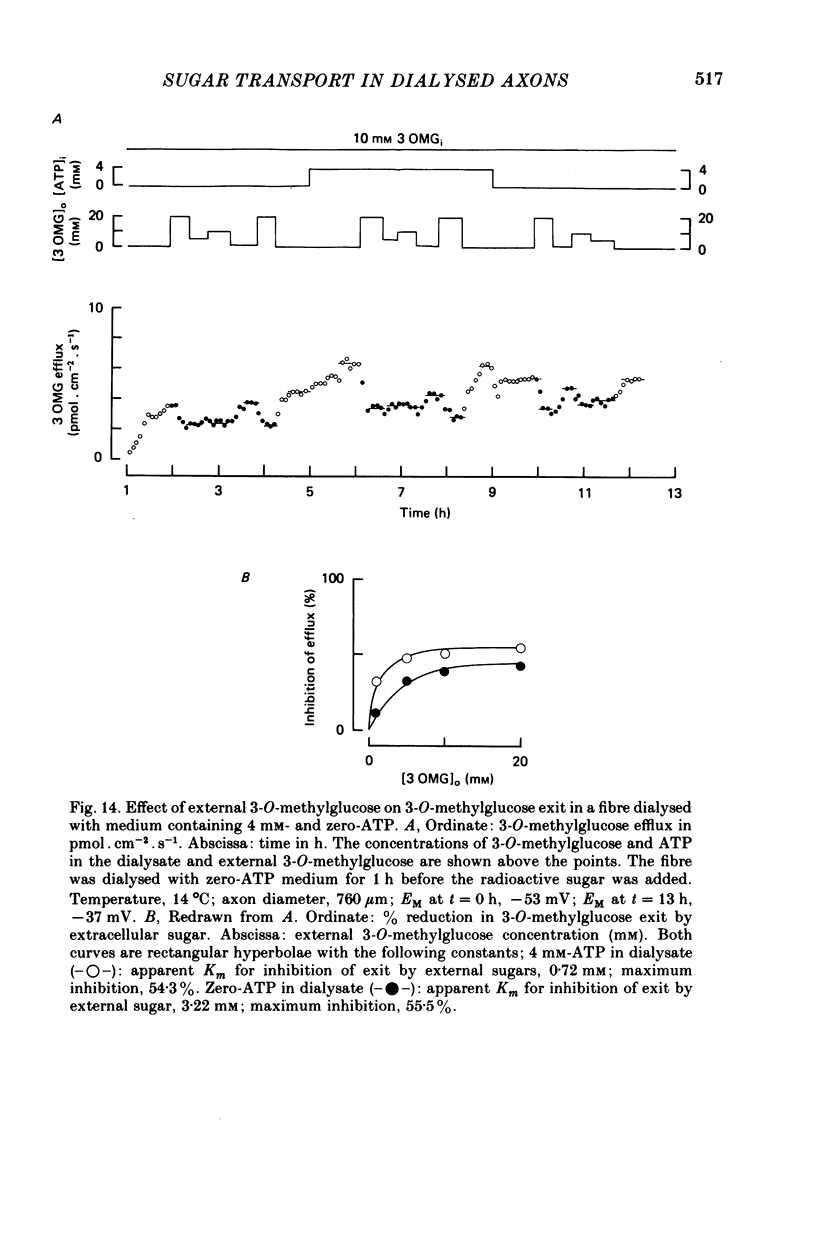
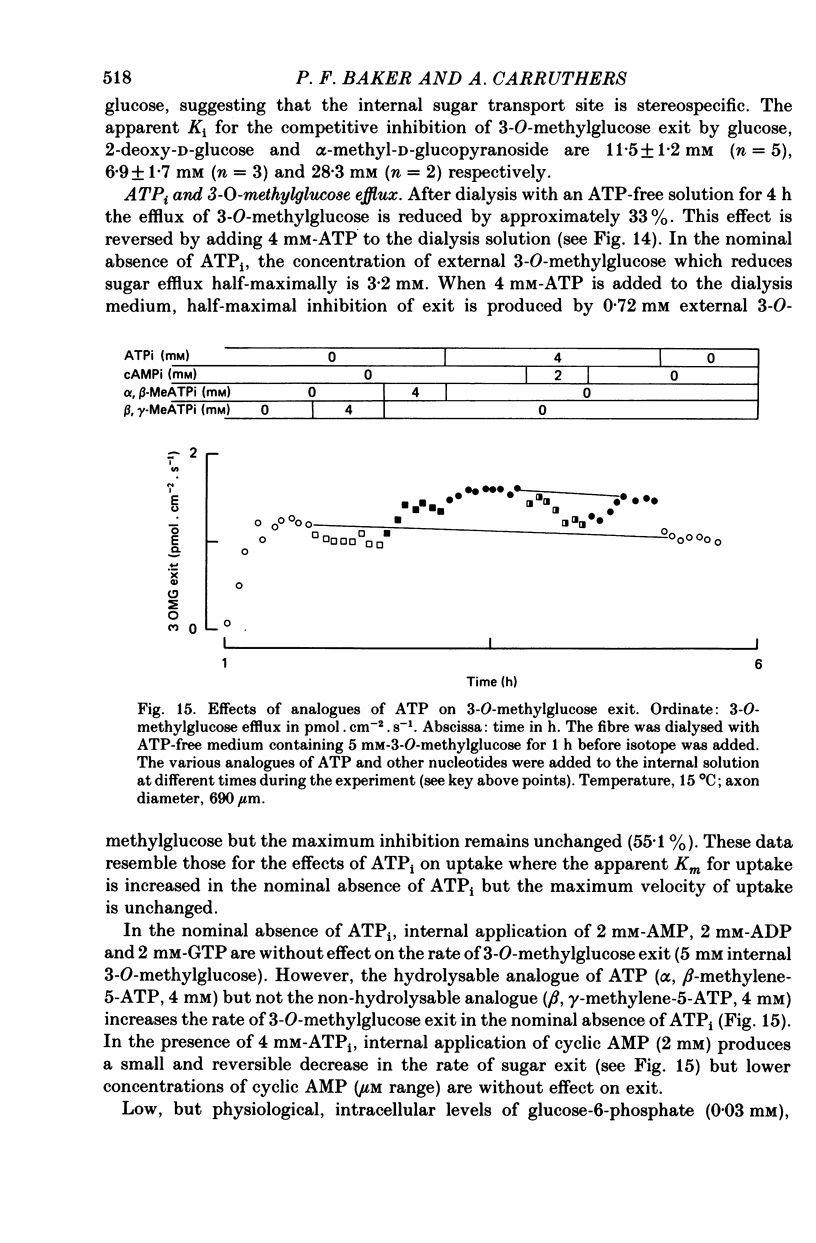
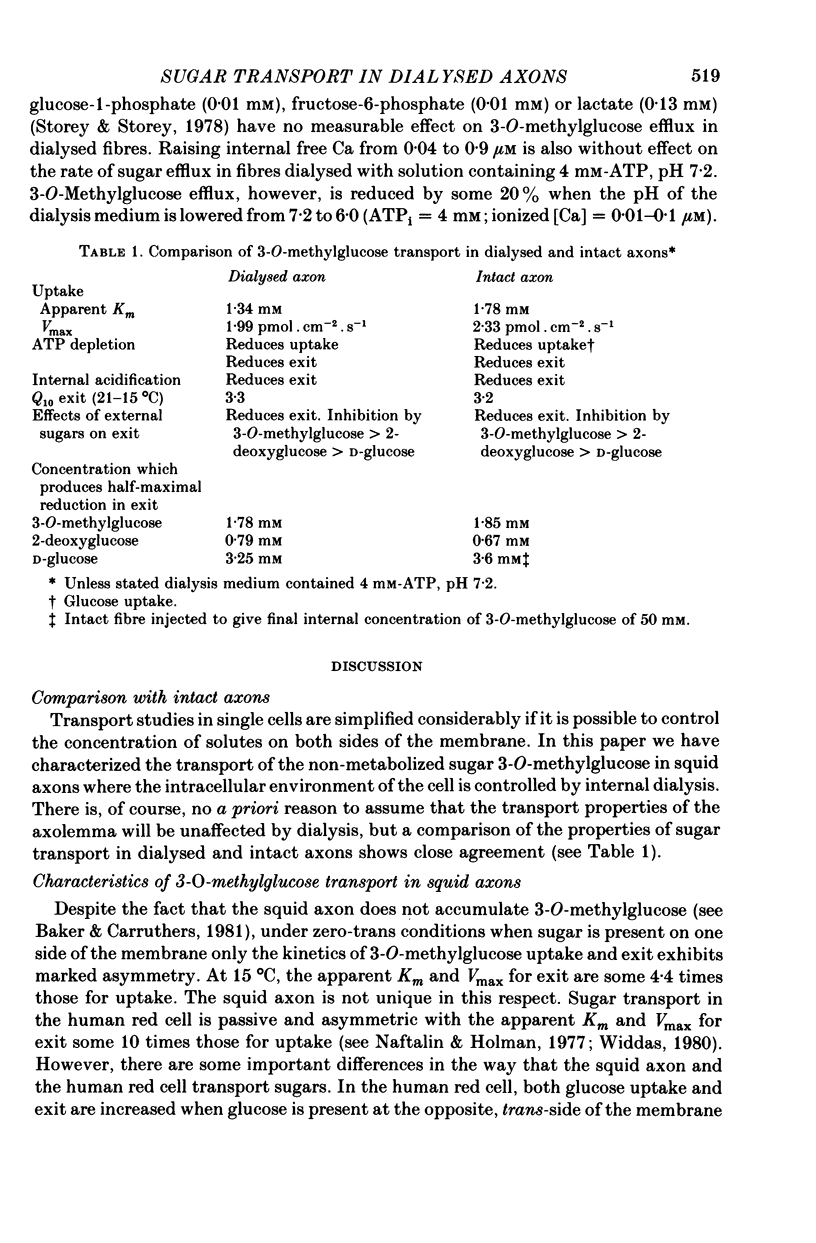
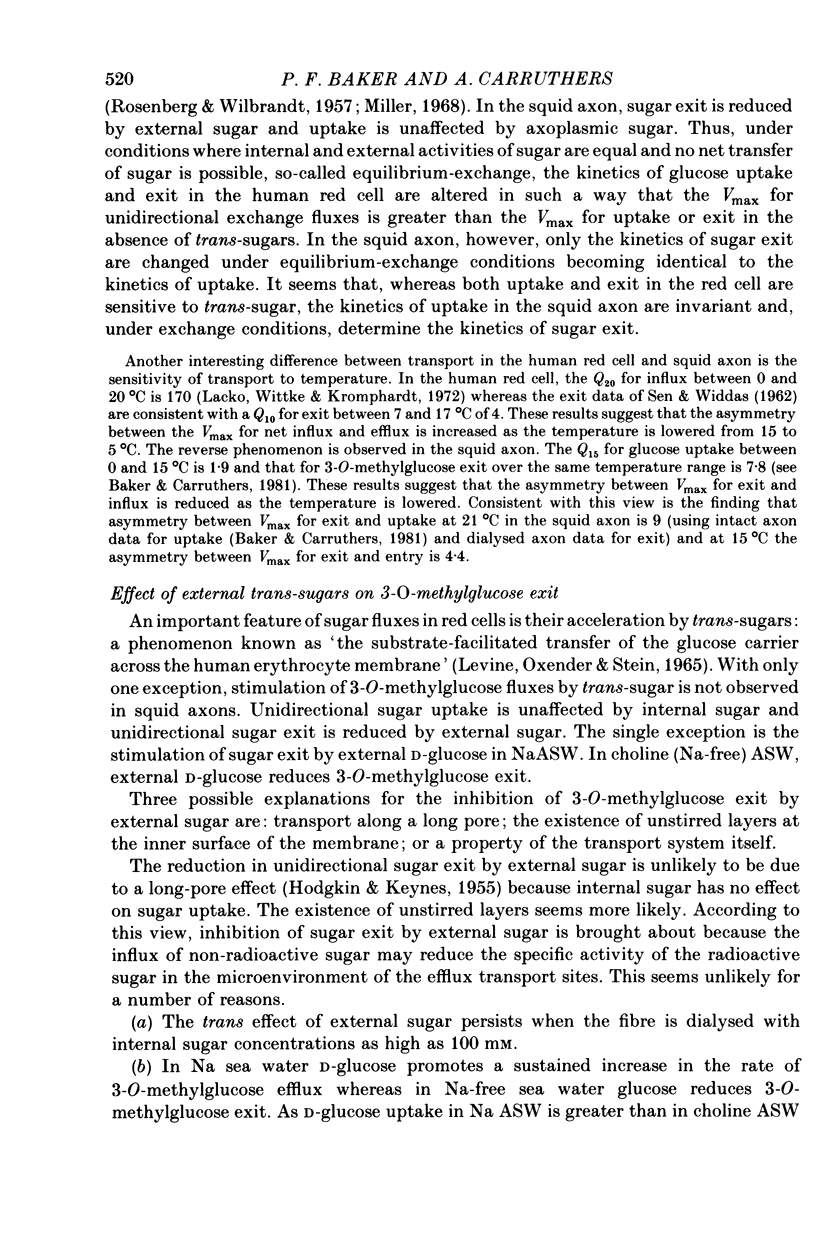
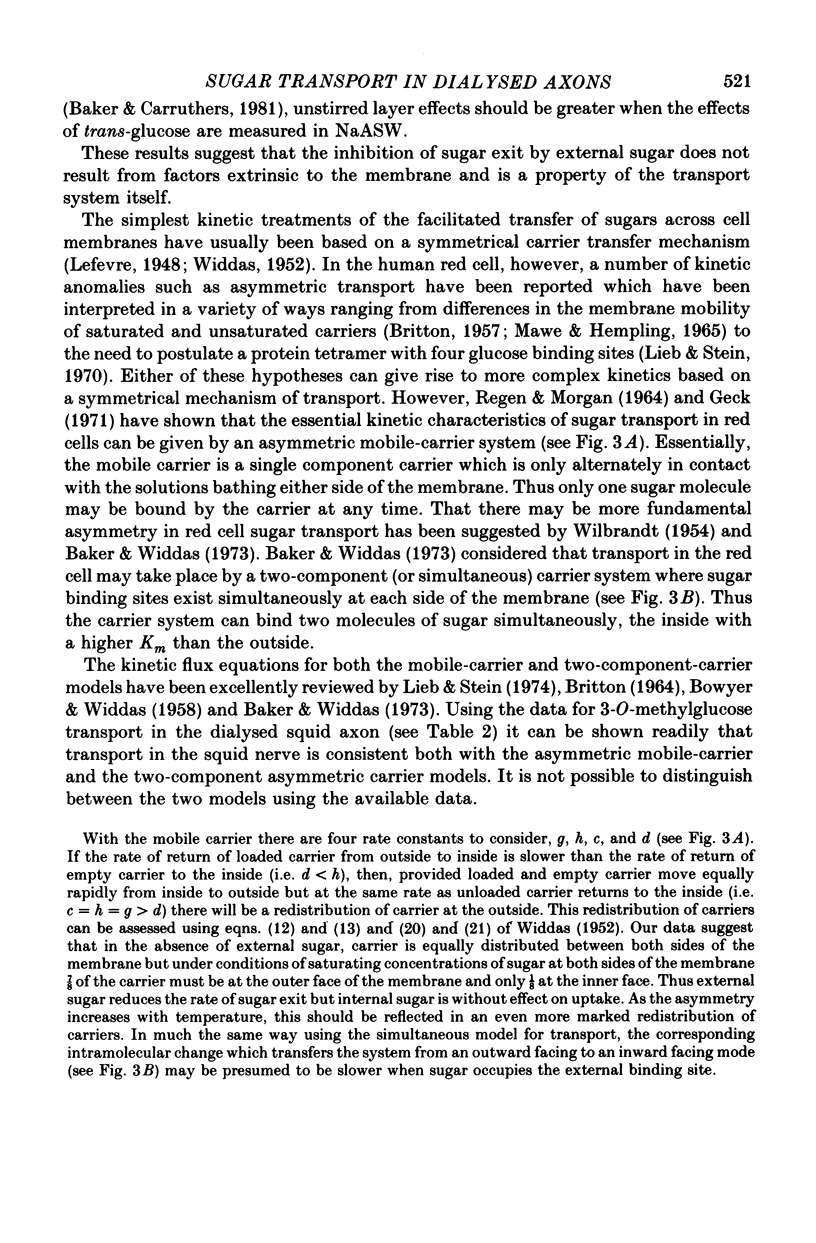
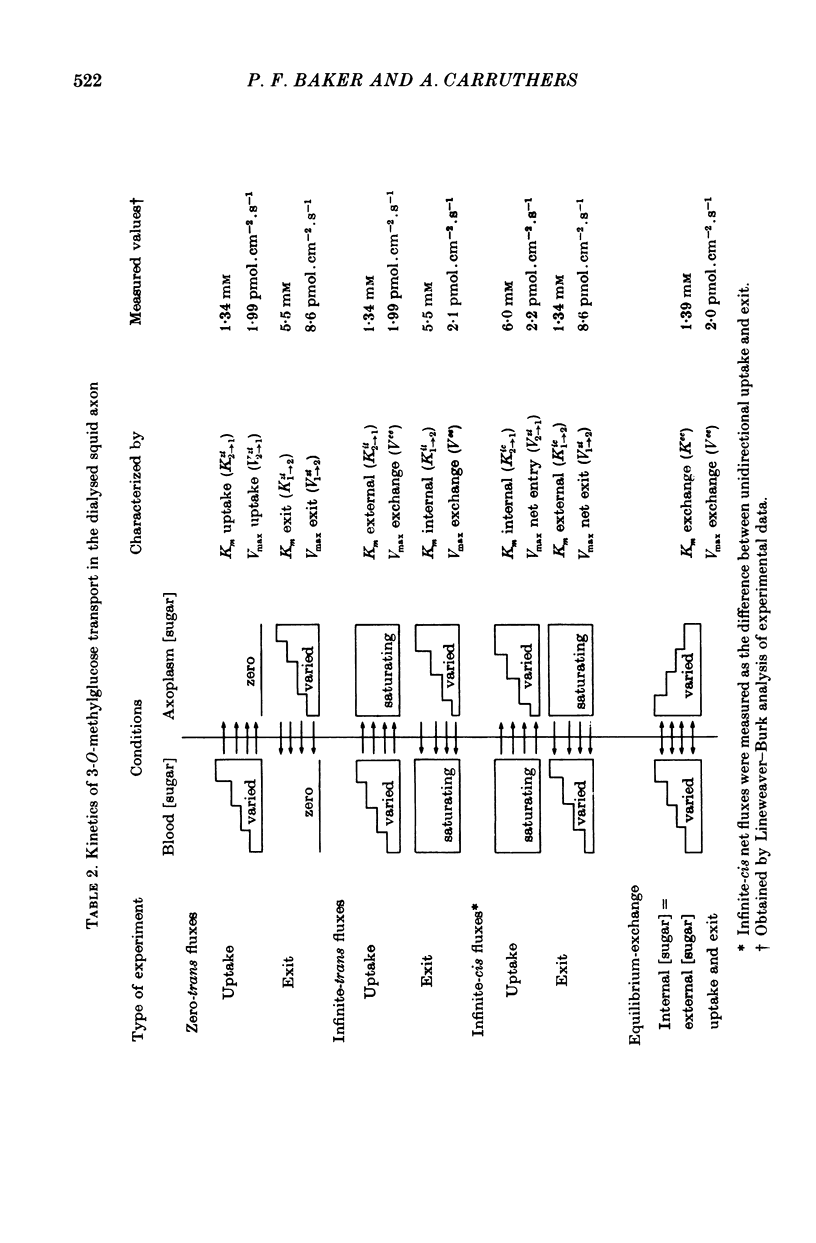
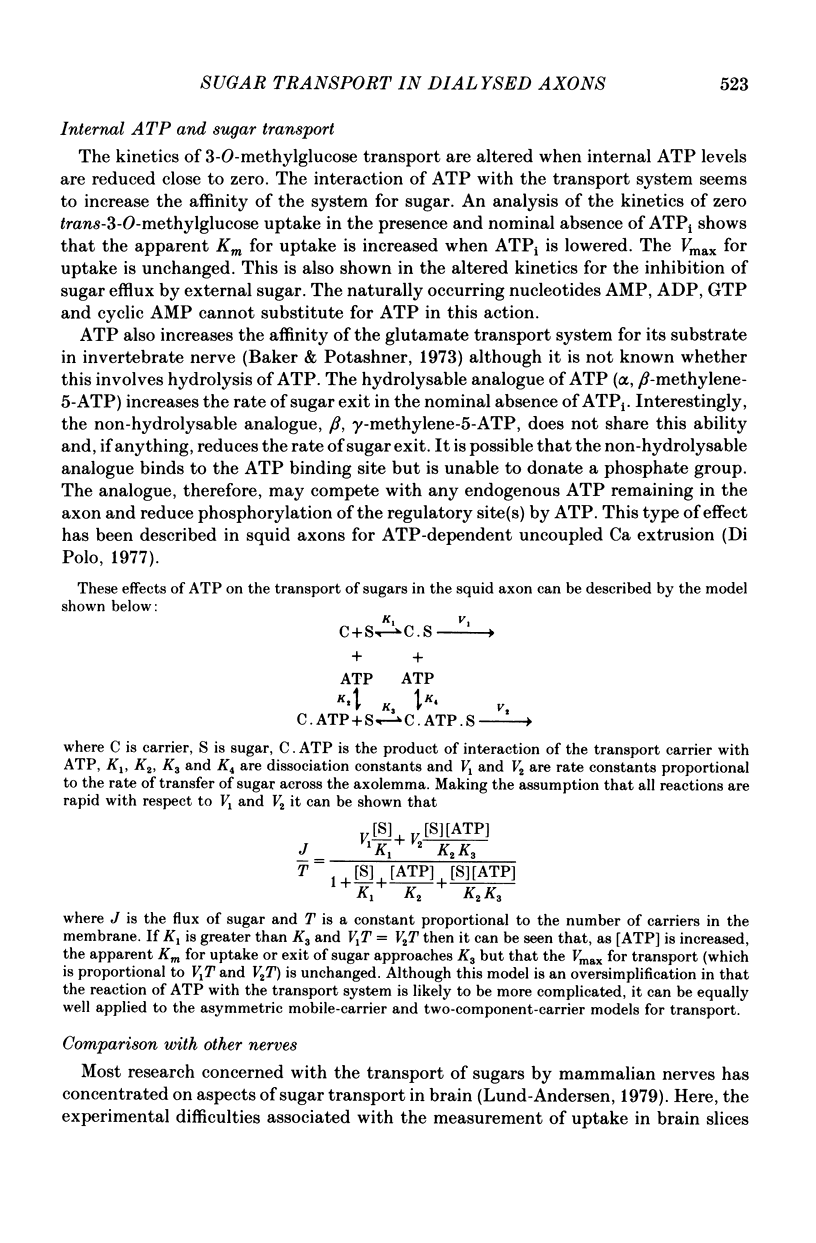
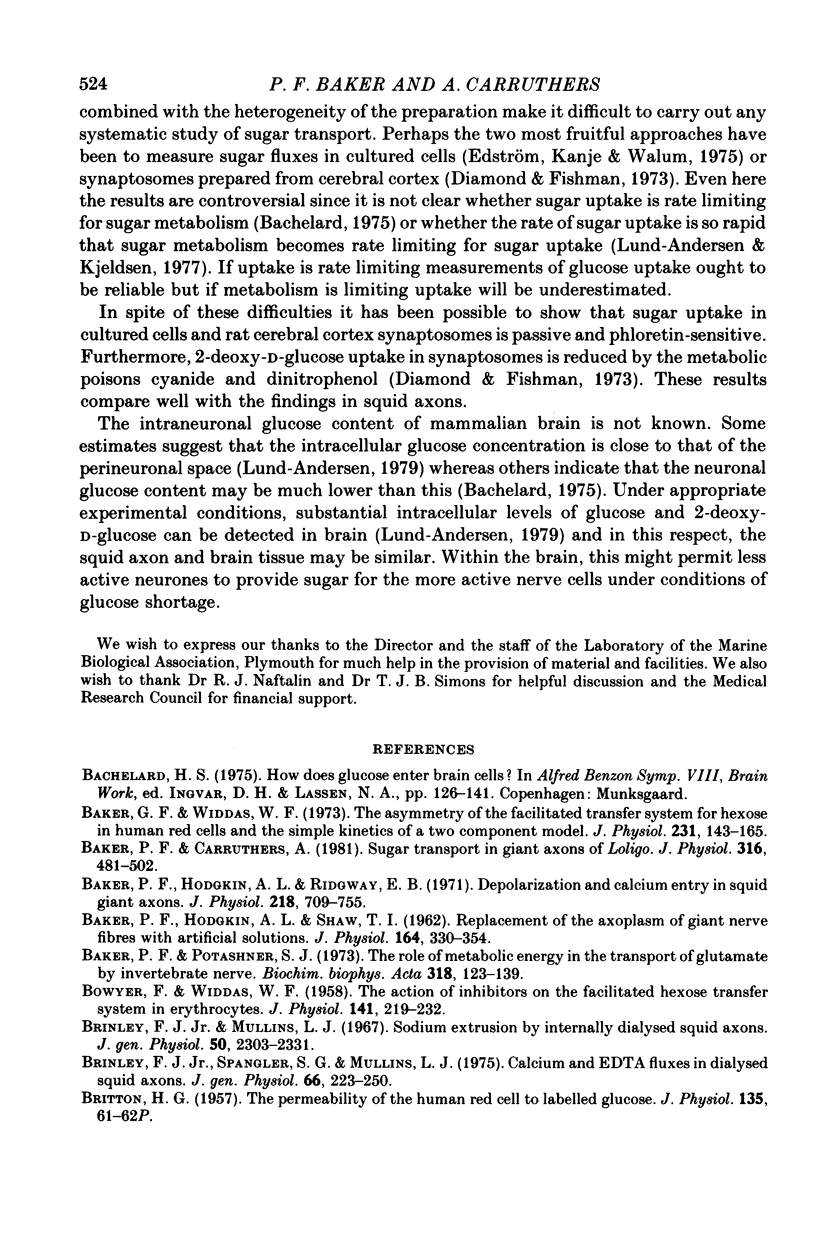
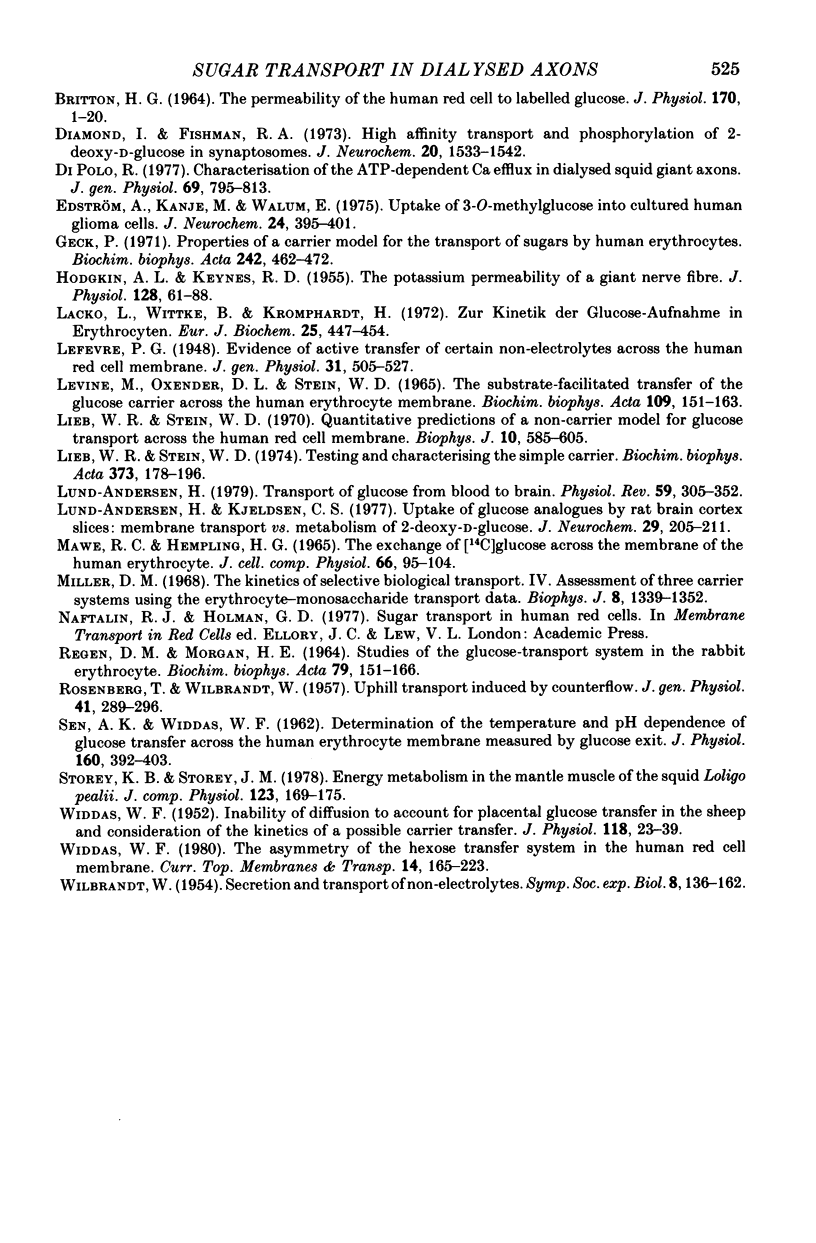
Images in this article
Selected References
These references are in PubMed. This may not be the complete list of references from this article.
- BAKER P. F., HODGKIN A. L., SHAW T. I. Replacement of the axoplasm of giant nerve fibres with artificial solutions. J Physiol. 1962 Nov;164:330–354. doi: 10.1113/jphysiol.1962.sp007025. [DOI] [PMC free article] [PubMed] [Google Scholar]
- BOWYER F., WIDDAS W. F. The action of inhibitors on the facilitated hexose transfer system in erythrocytes. J Physiol. 1958 Apr 30;141(2):219–232. doi: 10.1113/jphysiol.1958.sp005969. [DOI] [PMC free article] [PubMed] [Google Scholar]
- BRITTON H. G. PERMEABILITY OF THE HUMAN RED CELL TO LABELLED GLUCOSE. J Physiol. 1964 Jan;170:1–20. doi: 10.1113/jphysiol.1964.sp007310. [DOI] [PMC free article] [PubMed] [Google Scholar]
- BRITTON H. G. The permeability of the human red cell to labelled glucose. J Physiol. 1957 Feb 15;135(2):61–2P. [PubMed] [Google Scholar]
- Baker G. F., Widdas W. F. The asymmetry of the facilitated transfer system for hexoses in human red cells and the simple kinetics of a two component model. J Physiol. 1973 May;231(1):143–165. doi: 10.1113/jphysiol.1973.sp010225. [DOI] [PMC free article] [PubMed] [Google Scholar]
- Baker P. F., Carruthers A. Sugar transport in giant axons of Loligo. J Physiol. 1981 Jul;316:481–502. doi: 10.1113/jphysiol.1981.sp013802. [DOI] [PMC free article] [PubMed] [Google Scholar]
- Baker P. F., Potashner S. J. The role of metabolic energy in the transport of glutamate by invertebrate nerve. Biochim Biophys Acta. 1973 Aug 9;318(1):123–139. doi: 10.1016/0005-2736(73)90342-8. [DOI] [PubMed] [Google Scholar]
- Brinley F. J., Jr, Mullins L. J. Sodium extrusion by internally dialyzed squid axons. J Gen Physiol. 1967 Nov;50(10):2303–2331. doi: 10.1085/jgp.50.10.2303. [DOI] [PMC free article] [PubMed] [Google Scholar]
- Brinley F. J., Jr, Spangler S. G., Mullins L. J. Calcium and EDTA fluxes in dialyzed squid axons. J Gen Physiol. 1975 Aug;66(2):223–250. doi: 10.1085/jgp.66.2.223. [DOI] [PMC free article] [PubMed] [Google Scholar]
- Diamond I., Fishman R. A. High-affinity transport and phosphorylation of 2-deoxy-D-glucose in synaptosomes. J Neurochem. 1973 Jun;20(6):1533–1542. doi: 10.1111/j.1471-4159.1973.tb00271.x. [DOI] [PubMed] [Google Scholar]
- Edström A., Kanje M., Walum E. Uptake of 3-O-methyl-D-glucose into cultured human glioma cells. J Neurochem. 1975 Feb;24(2):395–401. doi: 10.1111/j.1471-4159.1975.tb11893.x. [DOI] [PubMed] [Google Scholar]
- Geck P. Eigenschaften eines asymmetrischen Carrier-Modells für den Zuckertrnasport am menschlichen Erythrozyten. Biochim Biophys Acta. 1971 Aug 13;241(2):462–472. doi: 10.1016/0005-2736(71)90045-9. [DOI] [PubMed] [Google Scholar]
- HODGKIN A. L., KEYNES R. D. The potassium permeability of a giant nerve fibre. J Physiol. 1955 Apr 28;128(1):61–88. doi: 10.1113/jphysiol.1955.sp005291. [DOI] [PMC free article] [PubMed] [Google Scholar]
- Lacko L., Wittke B., Kromphardt H. Zur Kinetik der Glucose-Aufnahme in Erythrocyten. Effekt der Trans-Konzentraion. Eur J Biochem. 1972 Feb;25(3):447–454. doi: 10.1111/j.1432-1033.1972.tb01714.x. [DOI] [PubMed] [Google Scholar]
- LeFEVRE P. G. Evidence of active transfer of certain non-electrolytes across the human red cell membrane. J Gen Physiol. 1948 Jul 20;31(6):505–527. doi: 10.1085/jgp.31.6.505. [DOI] [PMC free article] [PubMed] [Google Scholar]
- Levine M., Oxender D. L., Stein W. D. The substrate-facilitated transport of the glucose carrier across the human erythrocyte membrane. Biochim Biophys Acta. 1965 Sep 27;109(1):151–163. doi: 10.1016/0926-6585(65)90099-3. [DOI] [PubMed] [Google Scholar]
- Lieb W. R., Stein W. D. Quantitative predictions of a noncarrier model for glucose transport across the human red cell membrane. Biophys J. 1970 Jul;10(7):585–609. doi: 10.1016/s0006-3495(70)86322-6. [DOI] [PMC free article] [PubMed] [Google Scholar]
- Lieb W. R., Stein W. D. Testing and characterizing the simple carrier. Biochim Biophys Acta. 1974 Dec 10;373(2):178–196. doi: 10.1016/0005-2736(74)90144-8. [DOI] [PubMed] [Google Scholar]
- Lund-Andersen H., Kjeldsen C. S. Uptake of glucose analogues by rat brain cortex slices: membrane transport versus metabolism of 2-deoxy-D-glucose. J Neurochem. 1977 Aug;29(2):205–211. doi: 10.1111/j.1471-4159.1977.tb09610.x. [DOI] [PubMed] [Google Scholar]
- Lund-Andersen H. Transport of glucose from blood to brain. Physiol Rev. 1979 Apr;59(2):305–352. doi: 10.1152/physrev.1979.59.2.305. [DOI] [PubMed] [Google Scholar]
- Mawe R. C., Hempling H. G. The exchange of C14 glucose across the membrane of the human erythrocyte. J Cell Physiol. 1965 Aug;66(1):95–103. doi: 10.1002/jcp.1030660110. [DOI] [PubMed] [Google Scholar]
- Miller D. M. The kinetics of selective biological transport. IV. Assessment of three carrier systems using the erythrocyte-monosaccharide transport data. Biophys J. 1968 Nov;8(11):1339–1352. doi: 10.1016/S0006-3495(68)86560-9. [DOI] [PMC free article] [PubMed] [Google Scholar]
- REGEN D. M., MORGAN H. E. STUDIES OF THE GLUCOSE-TRANSPORT SYSTEM IN THE RABBIT ERYTHROCYTE. Biochim Biophys Acta. 1964 Jan 27;79:151–166. doi: 10.1016/0926-6577(64)90048-8. [DOI] [PubMed] [Google Scholar]
- ROSENBERG T., WILBRANDT W. Uphill transport induced by counterflow. J Gen Physiol. 1957 Nov 20;41(2):289–296. doi: 10.1085/jgp.41.2.289. [DOI] [PMC free article] [PubMed] [Google Scholar]
- SEN A. K., WIDDAS W. F. Determination of the temperature and pH dependence of glucose transfer across the human erythrocyte membrane measured by glucose exit. J Physiol. 1962 Mar;160:392–403. doi: 10.1113/jphysiol.1962.sp006854. [DOI] [PMC free article] [PubMed] [Google Scholar]
- WIDDAS W. F. Inability of diffusion to account for placental glucose transfer in the sheep and consideration of the kinetics of a possible carrier transfer. J Physiol. 1952 Sep;118(1):23–39. doi: 10.1113/jphysiol.1952.sp004770. [DOI] [PMC free article] [PubMed] [Google Scholar]