Abstract
1. The magnesium content of human red blood cells was controlled by varying the magnesium concentration in the medium in the presence of the ionophore A23187. The new magnesium levels attained were very stable, which allowed the magnesium dependence of the sodium pump to be investigated.
2. The effects of magnesium were shown to occur at the inner surface of the red cell membrane for the range of magnesium concentrations tested (10-7 to 6 × 10-3 m).
3. At intracellular ionized magnesium concentrations below 0.8 mm the activation of ouabain-sensitive sodium—potassium exchange by internal ionized magnesium could be resolved into two or three components: (a) a small component, about 5% of the maximum flux, which is apparently independent of the ionized magnesium concentration below 2 μm, (b) a saturating component with a K½ of between 30 and 45 μm, and possibly (c) a component which increases linearly with ionized magnesium concentration and which only becomes apparent at concentrations above 0.1 mm.
4. At intracellular ionized magnesium concentrations below 0.8 mm, activation of ouabain-sensitive sodium—sodium exchange by internal ionized magnesium could be resolved into two components: (a) a small component, about 6% of the maximal flux, which is apparently independent of the ionized magnesium concentration below 2 μm, and (b) a saturating component with a K½ of about 9 μm. At ionized magnesium concentrations between about 0.2 and 0.8 mm the rate of sodium—sodium exchange remained constant at the maximal level.
5. The intracellular concentration of ATP decreased and the ADP concentration increased as the magnesium content of the cells was reduced from the normal level. A small increase in ATP and a small decrease in ADP was seen when the magnesium content was increased above the normal level. The variation in the ATP: ADP ratio from 2.5 at very low magnesium levels to about 6 at normal magnesium levels can account, at least in part, for the different K½ values of sodium—potassium and sodium—sodium exchange.
6. When the concentration of ionized magnesium was increased above about 0.8 mm both sodium—potassium and sodium—sodium exchange were inhibited. Sodium—sodium exchange was more strongly inhibited than sodium—potassium exchange.
7. The possible sites of action of magnesium in the sodium pump cycle are discussed.
Full text
PDF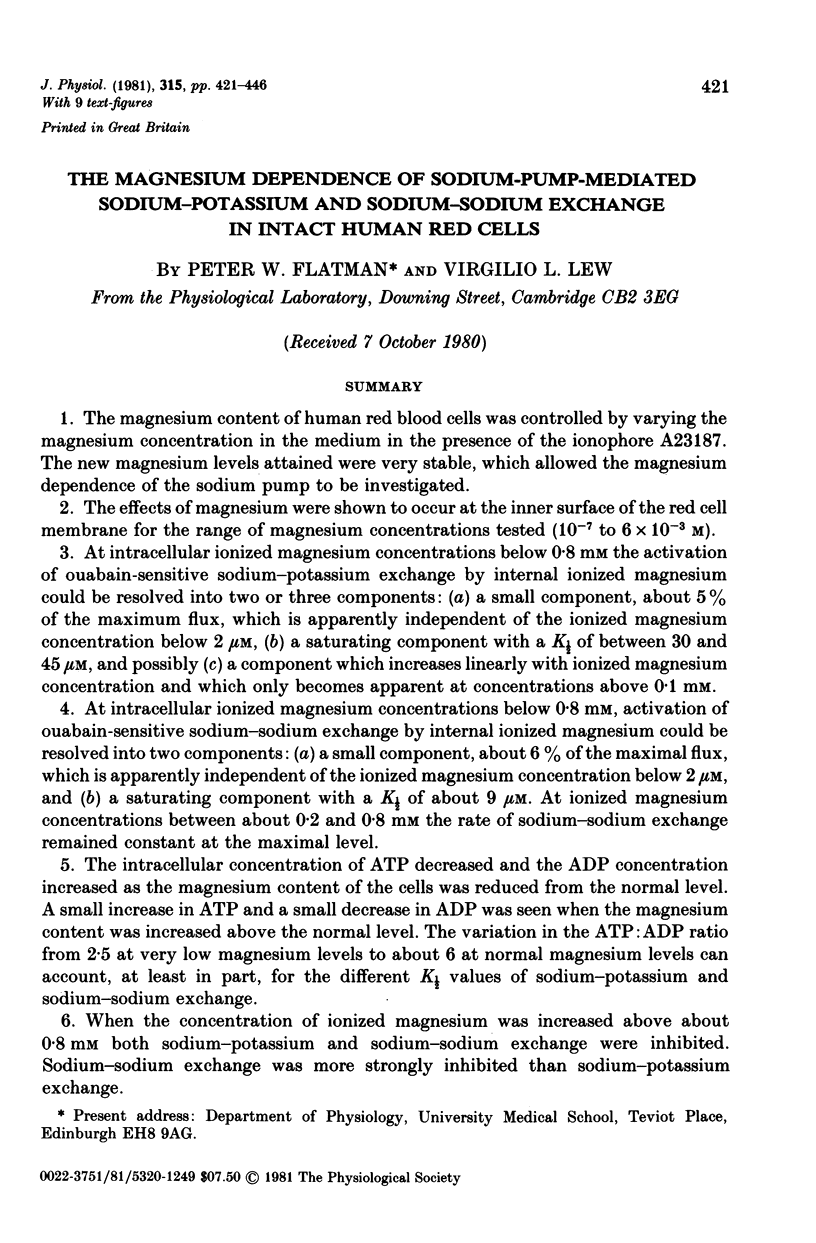
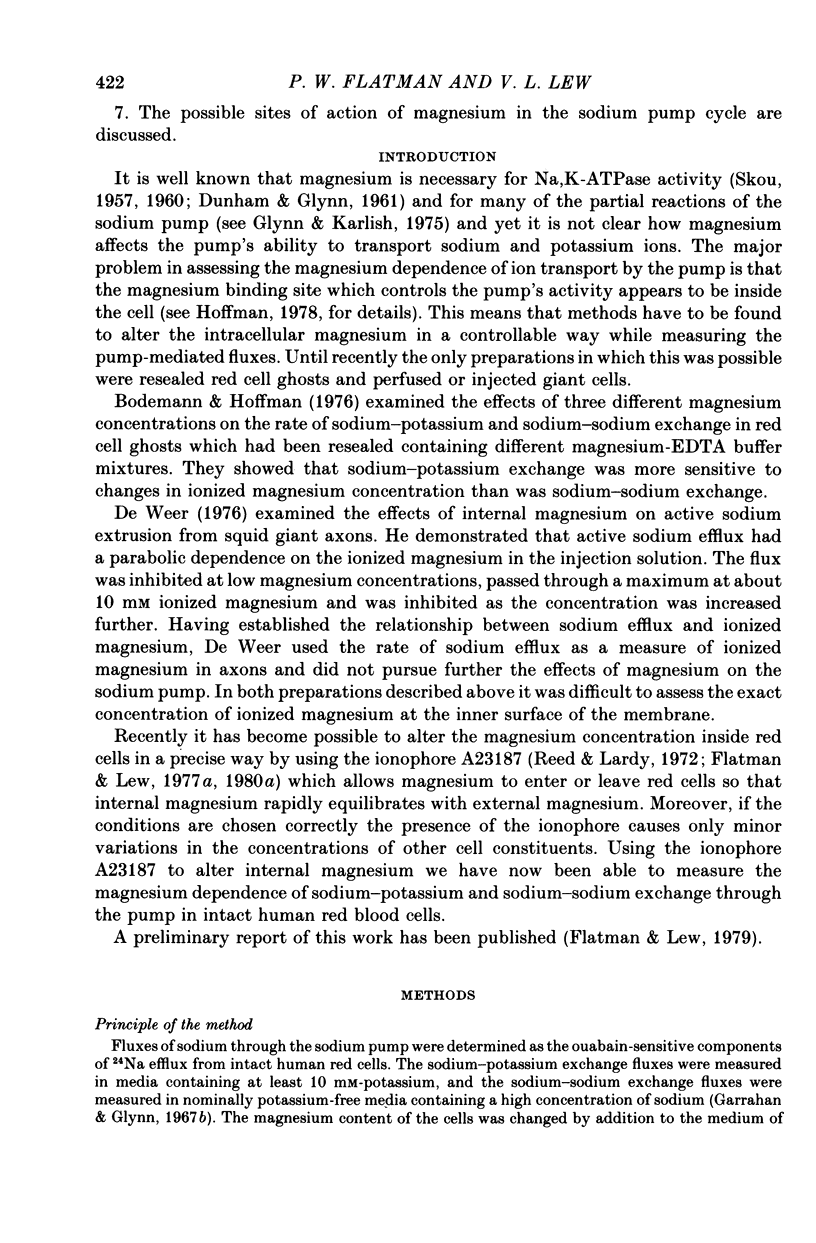
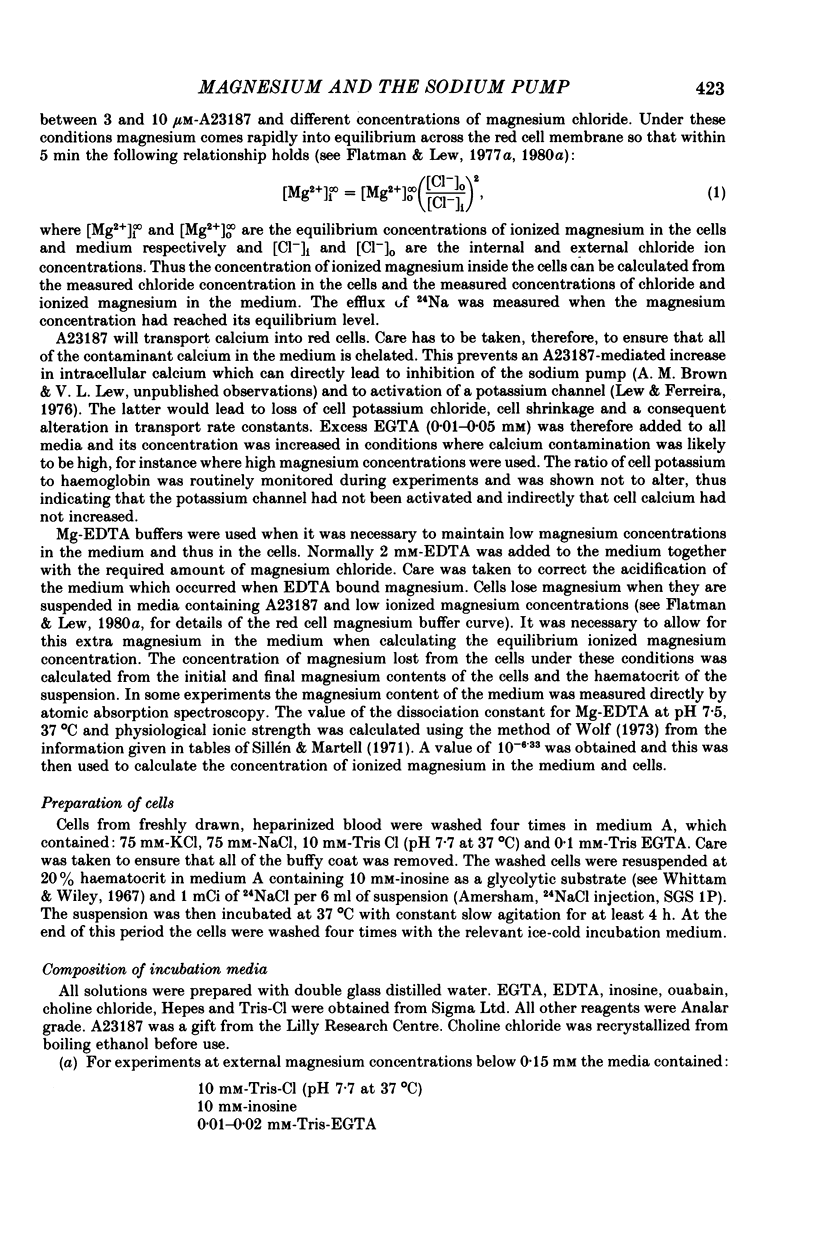
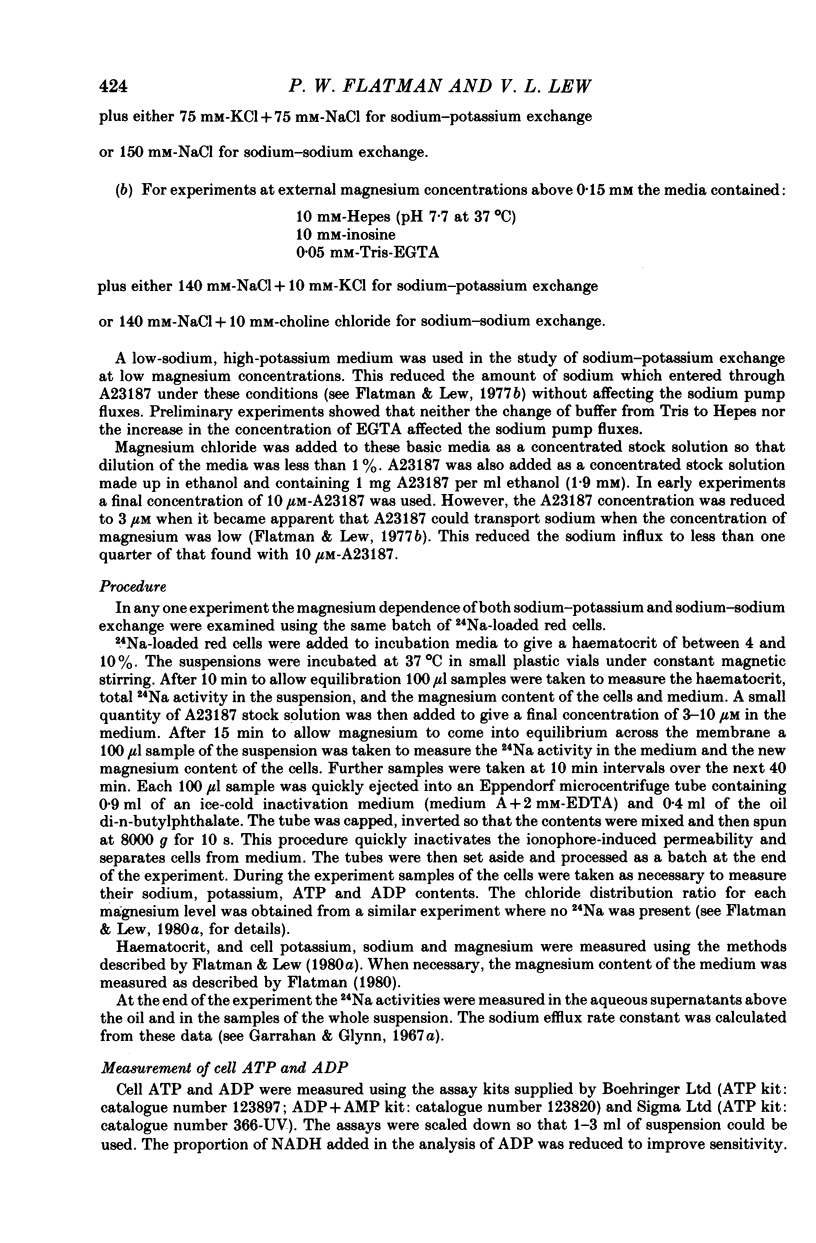
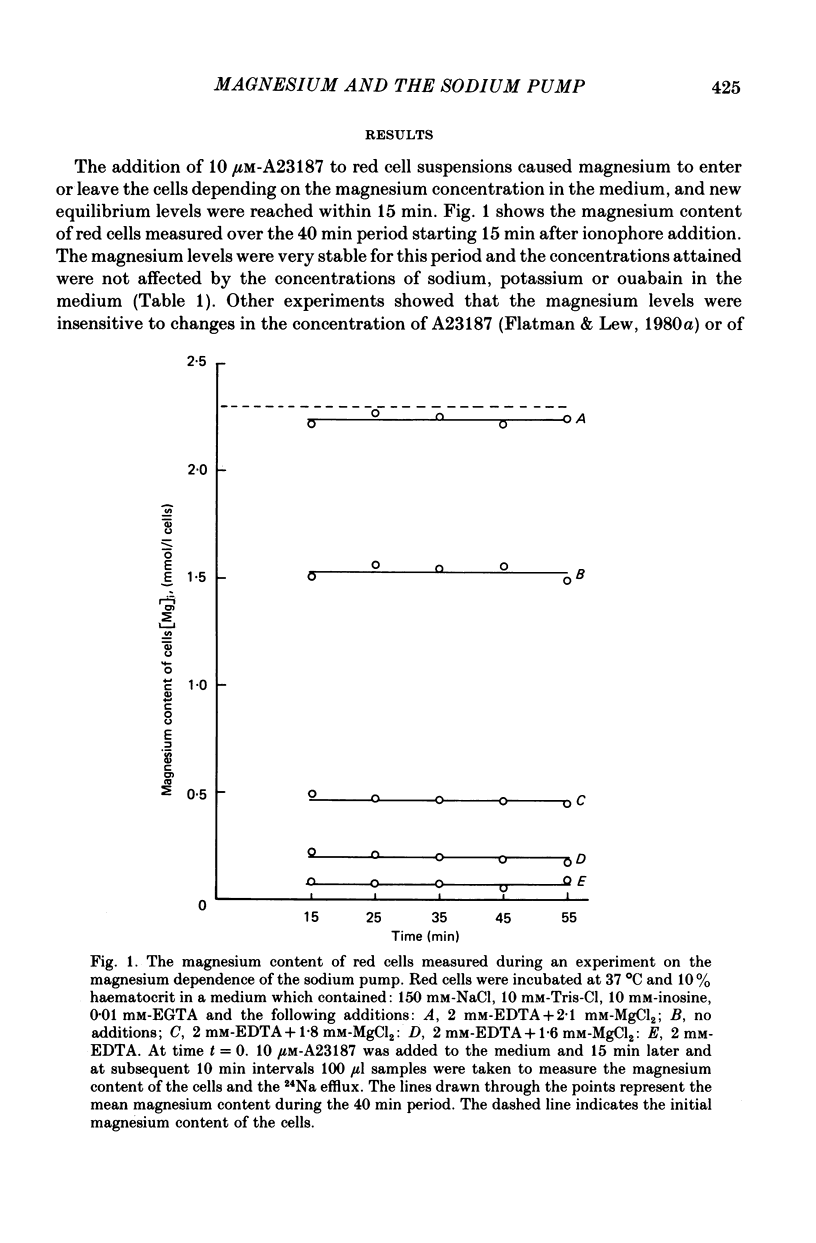
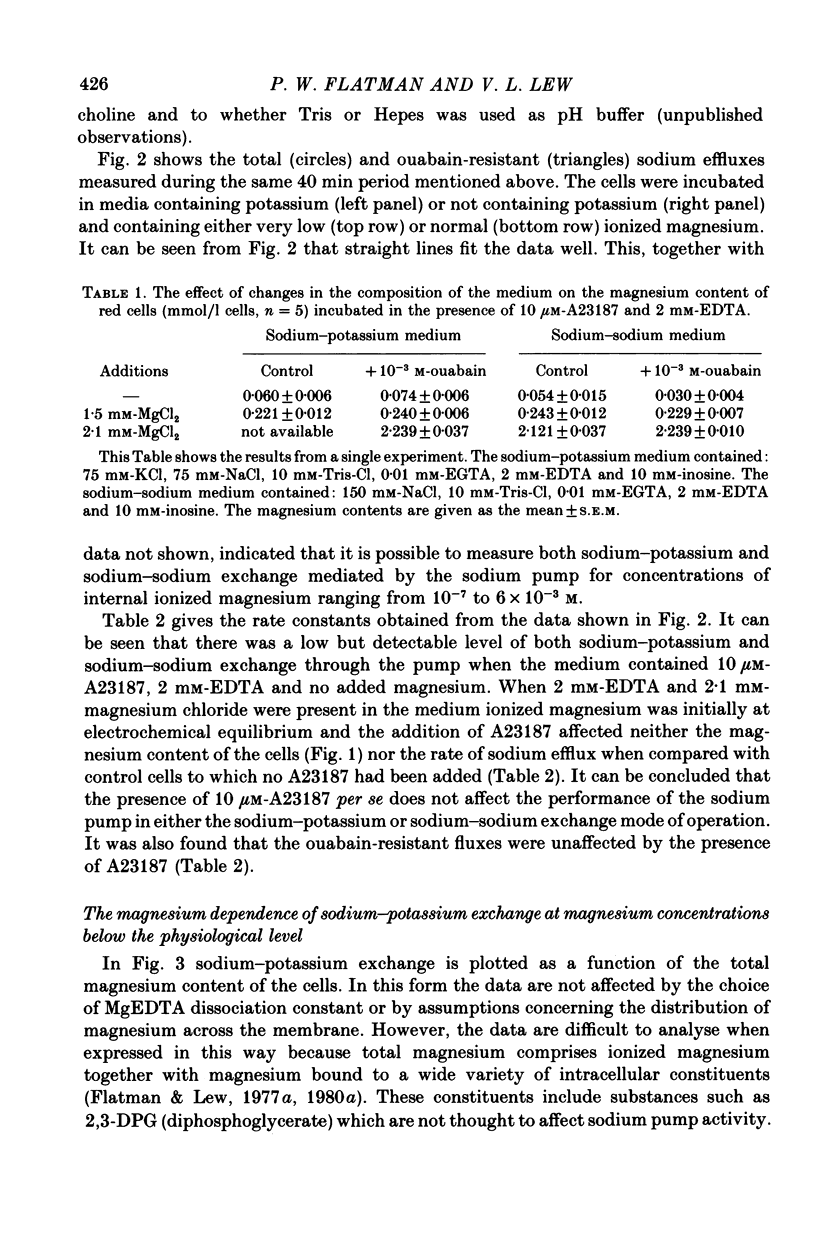
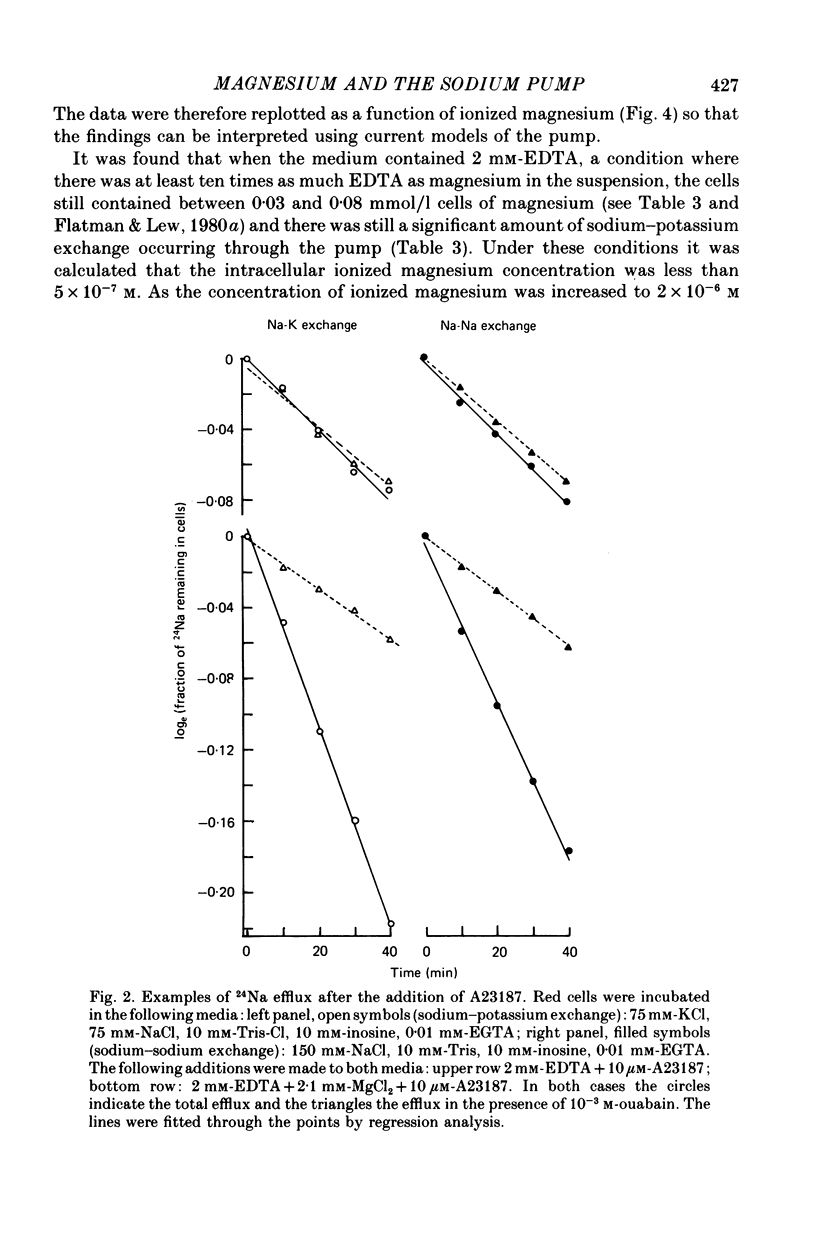
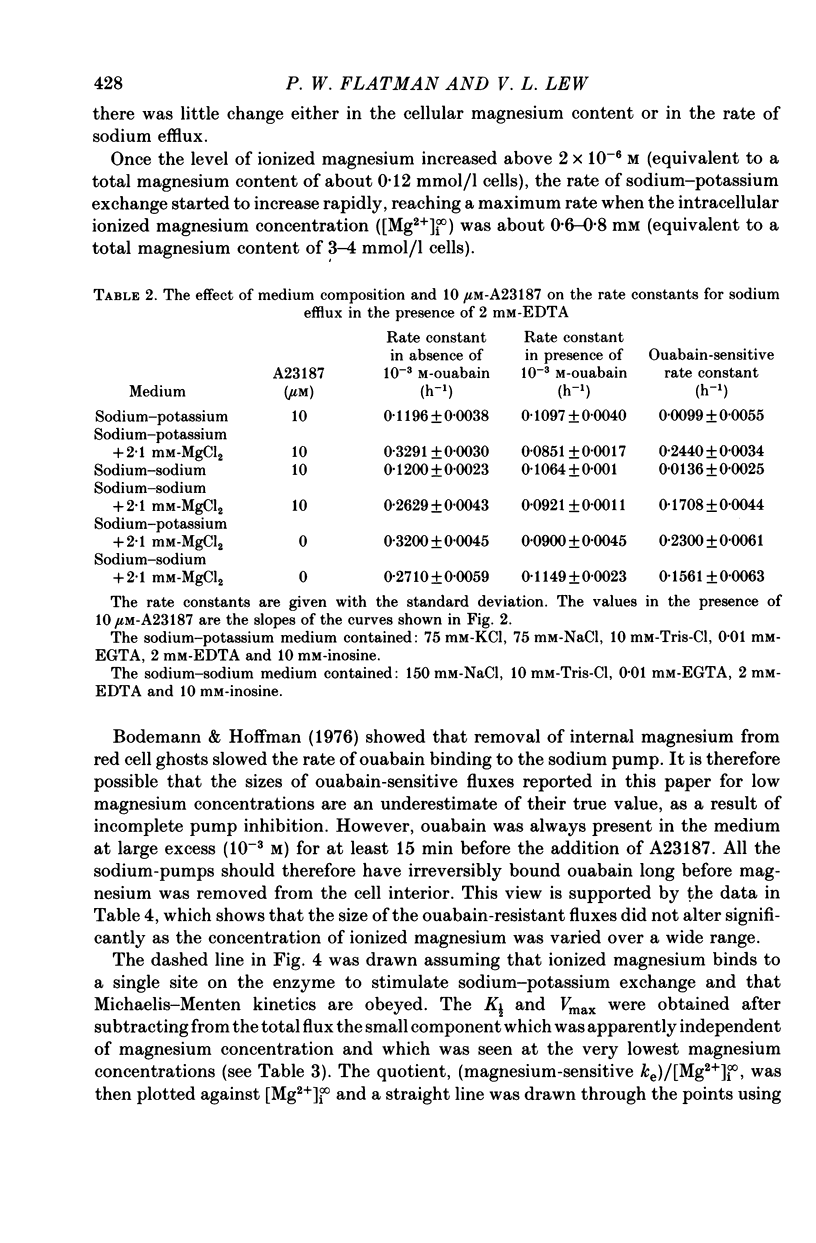
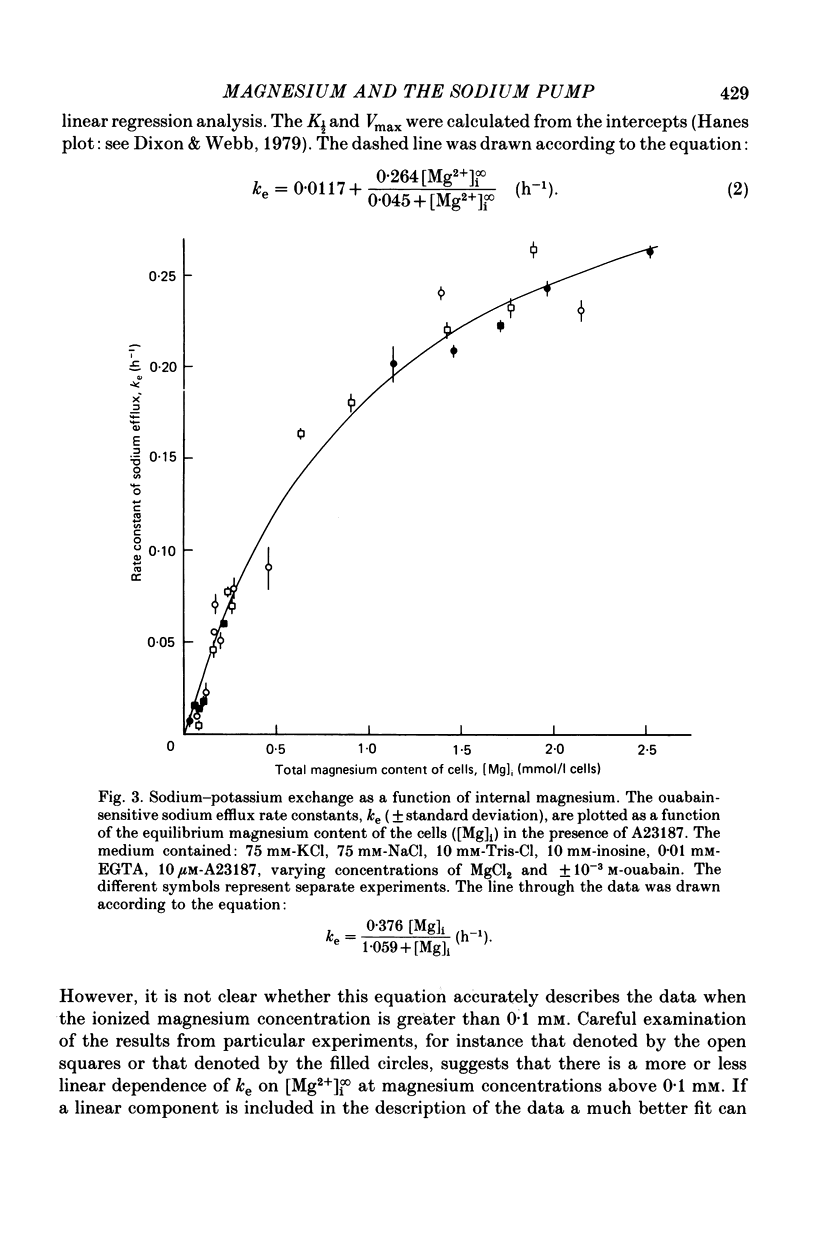
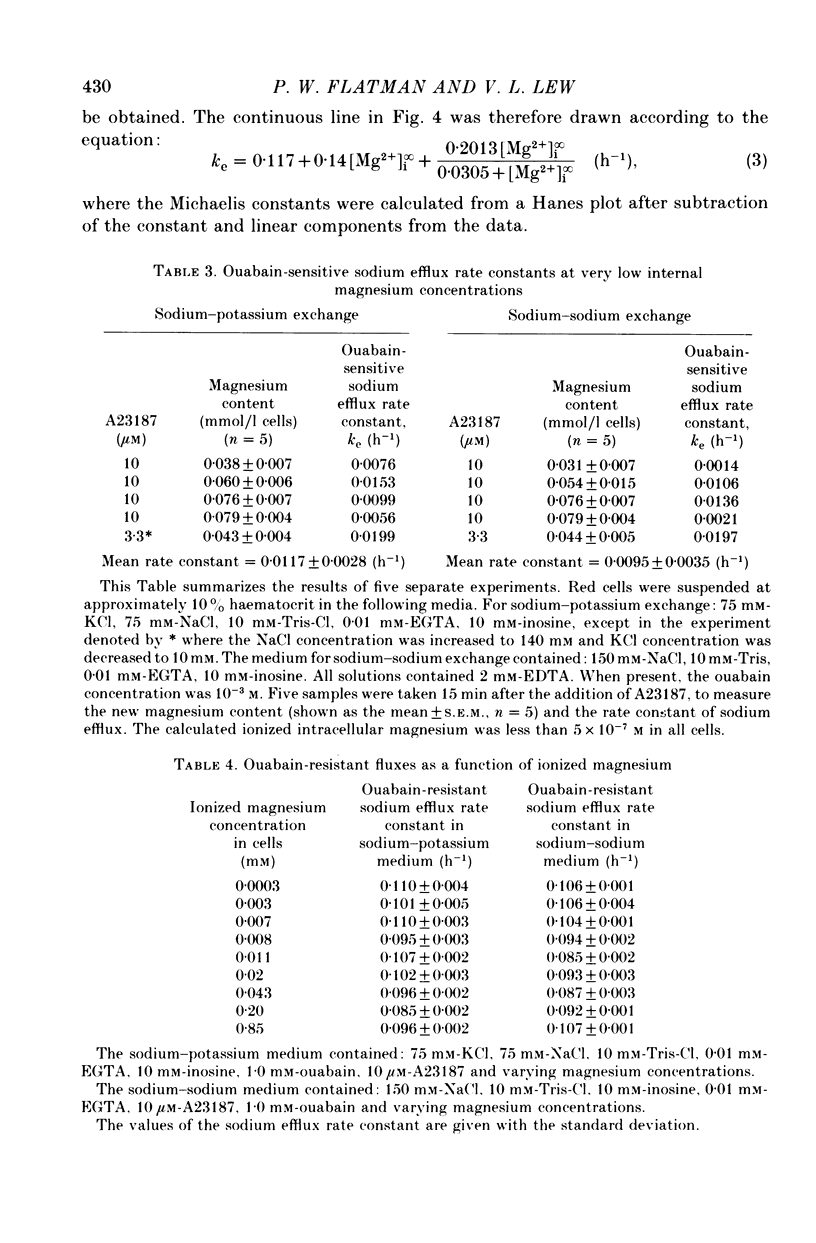
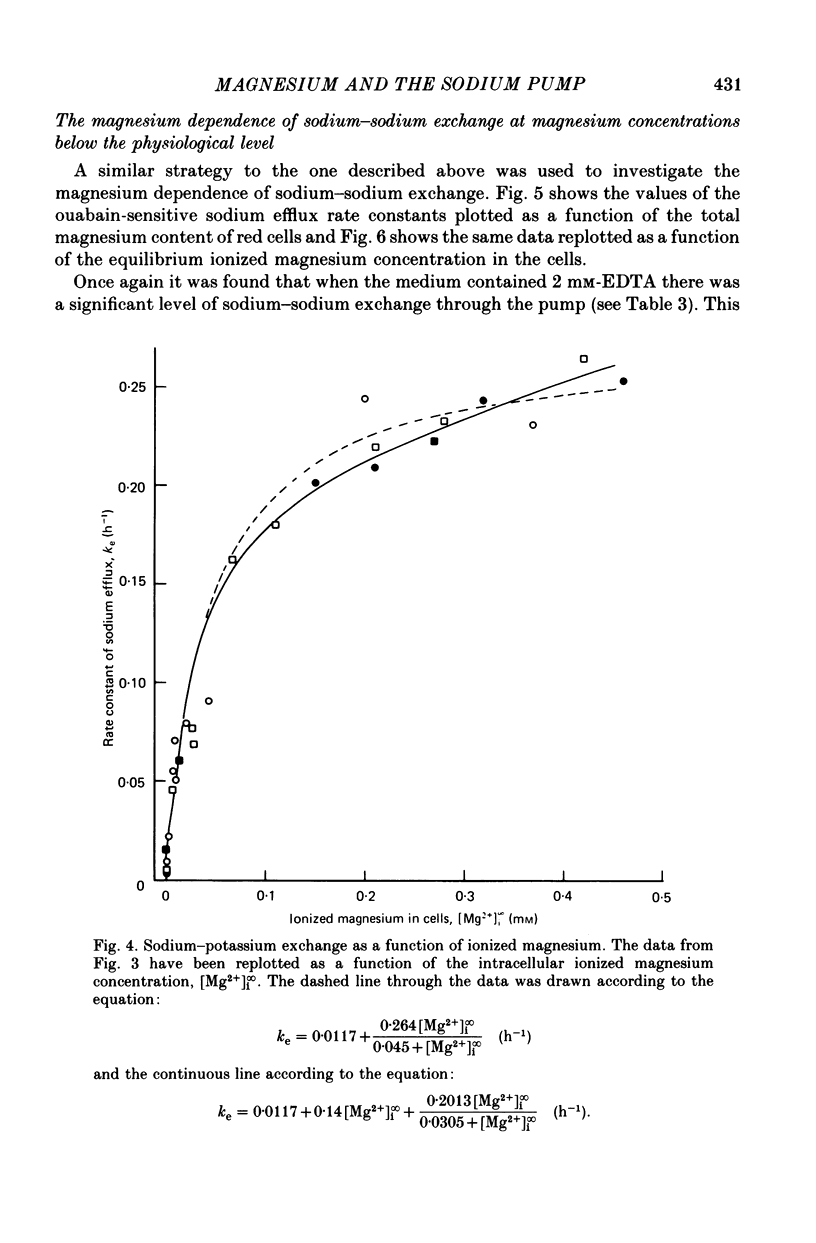
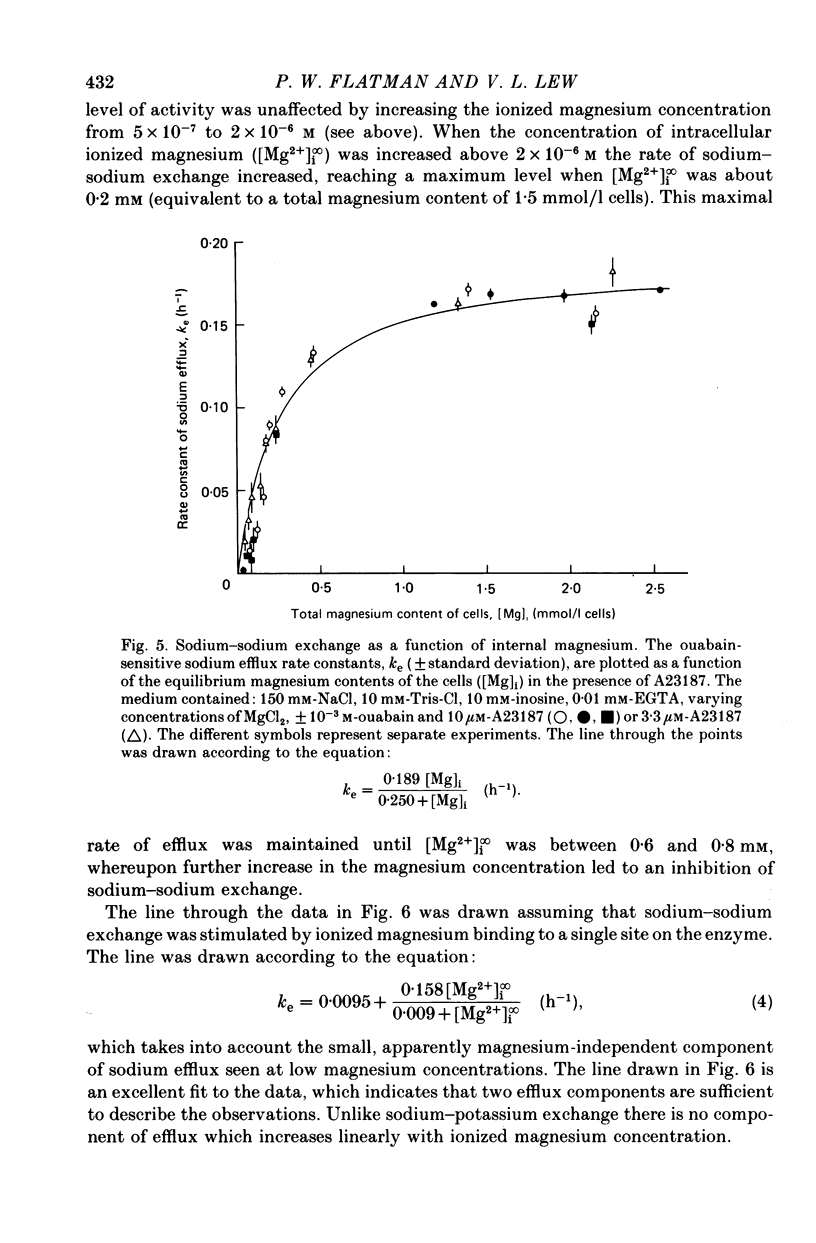
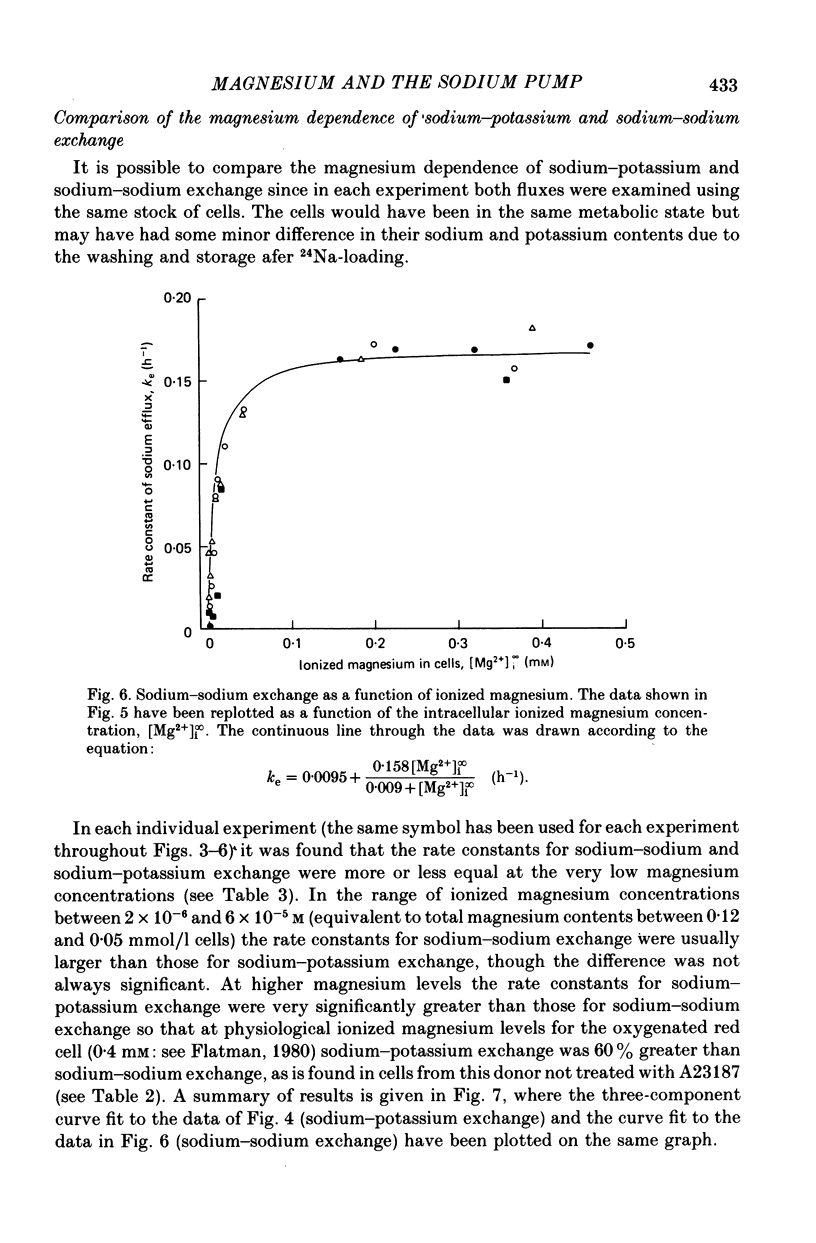
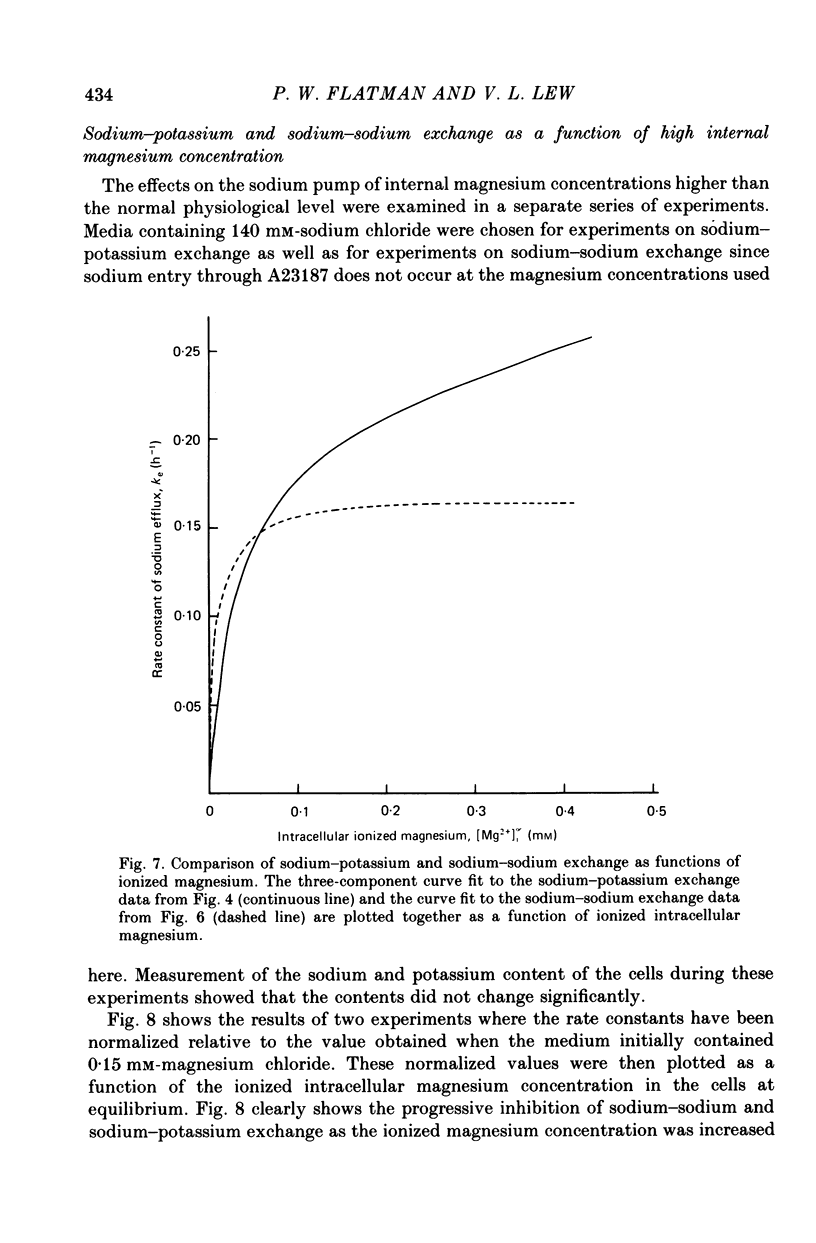
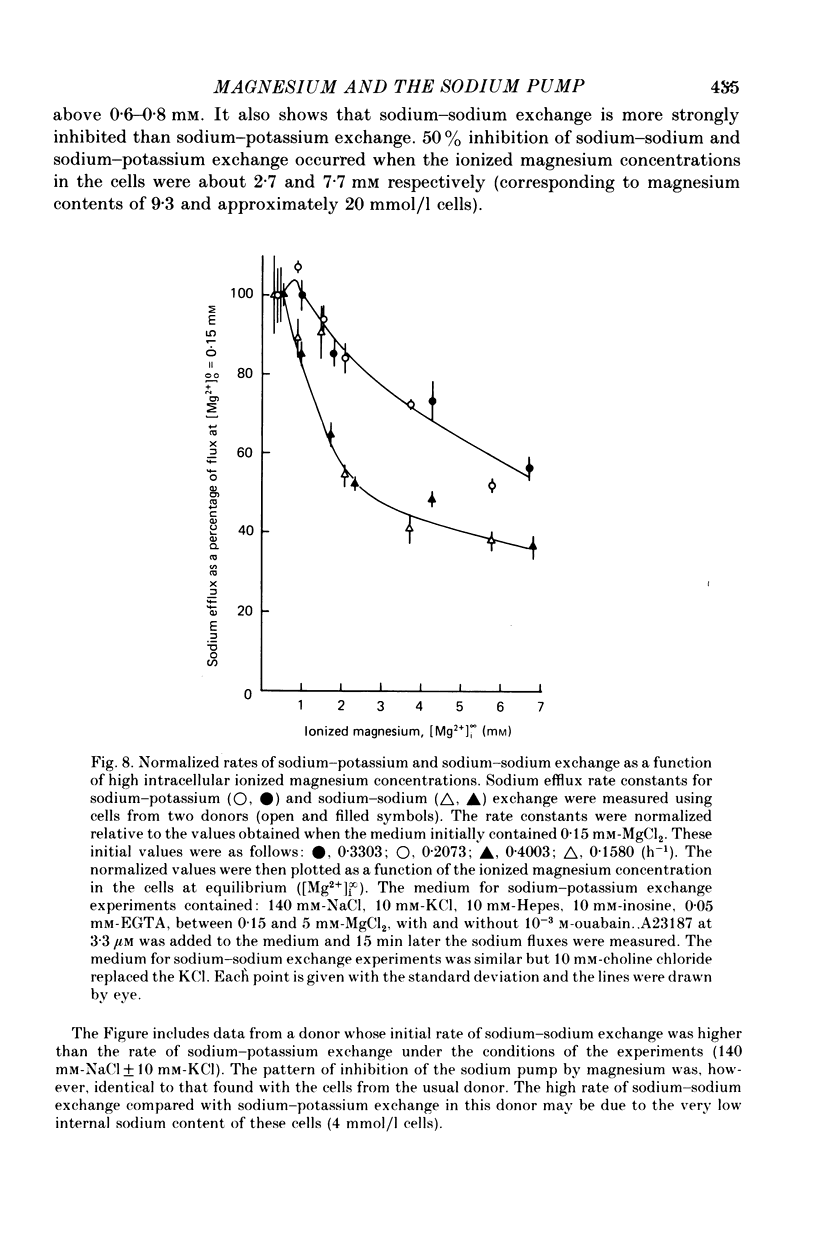
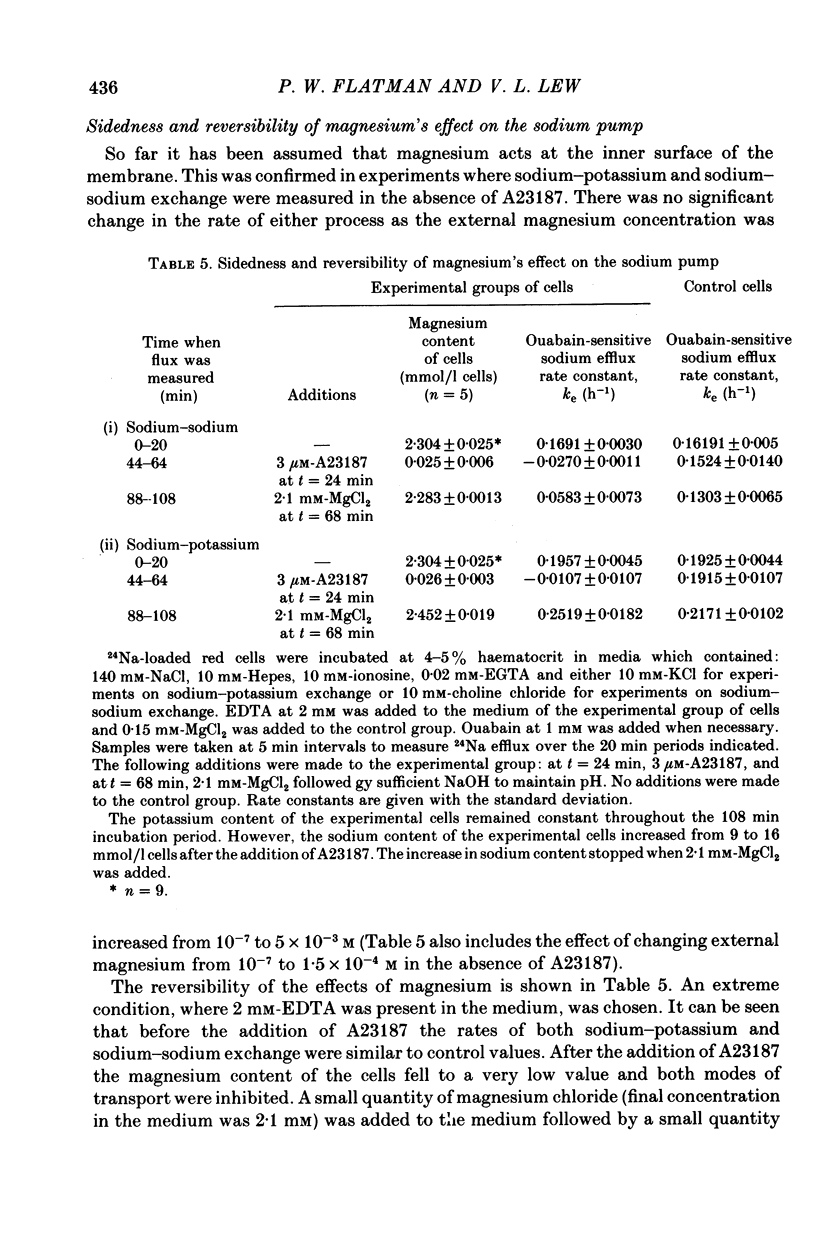
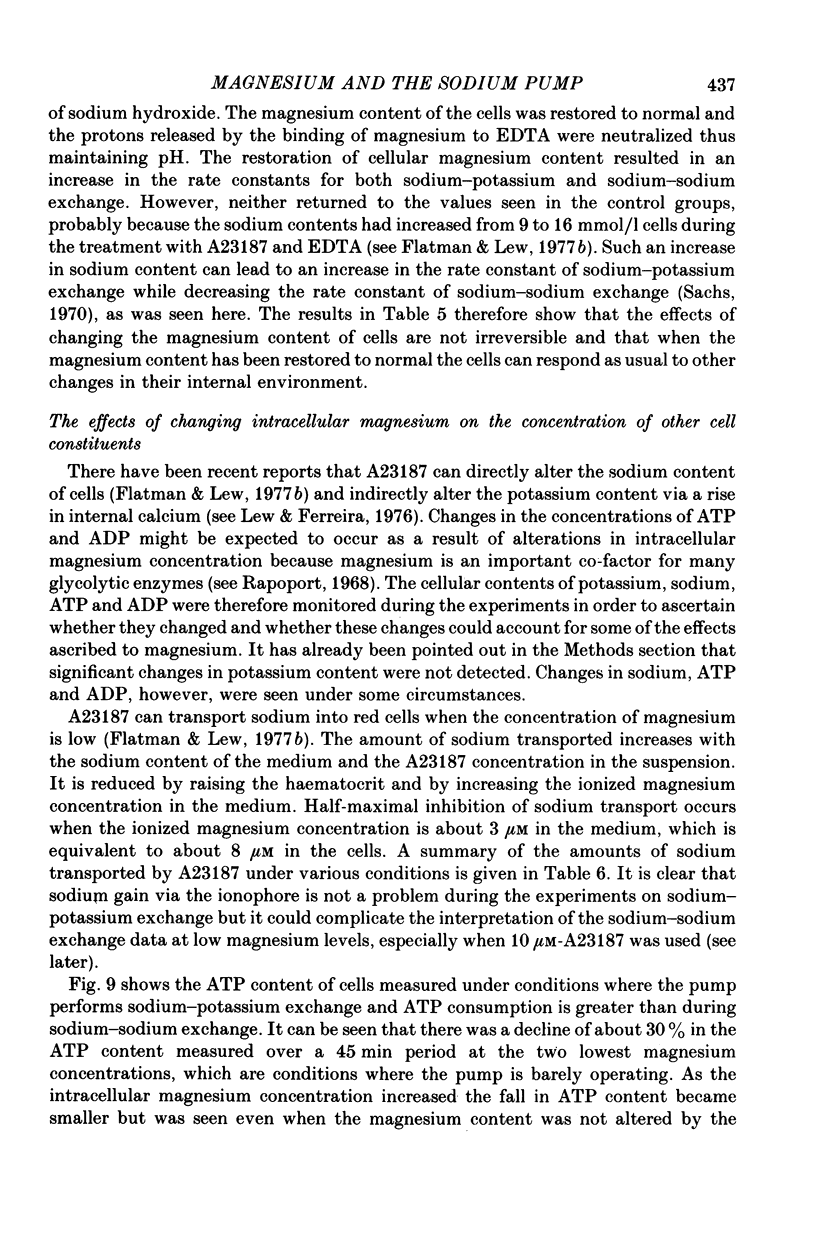
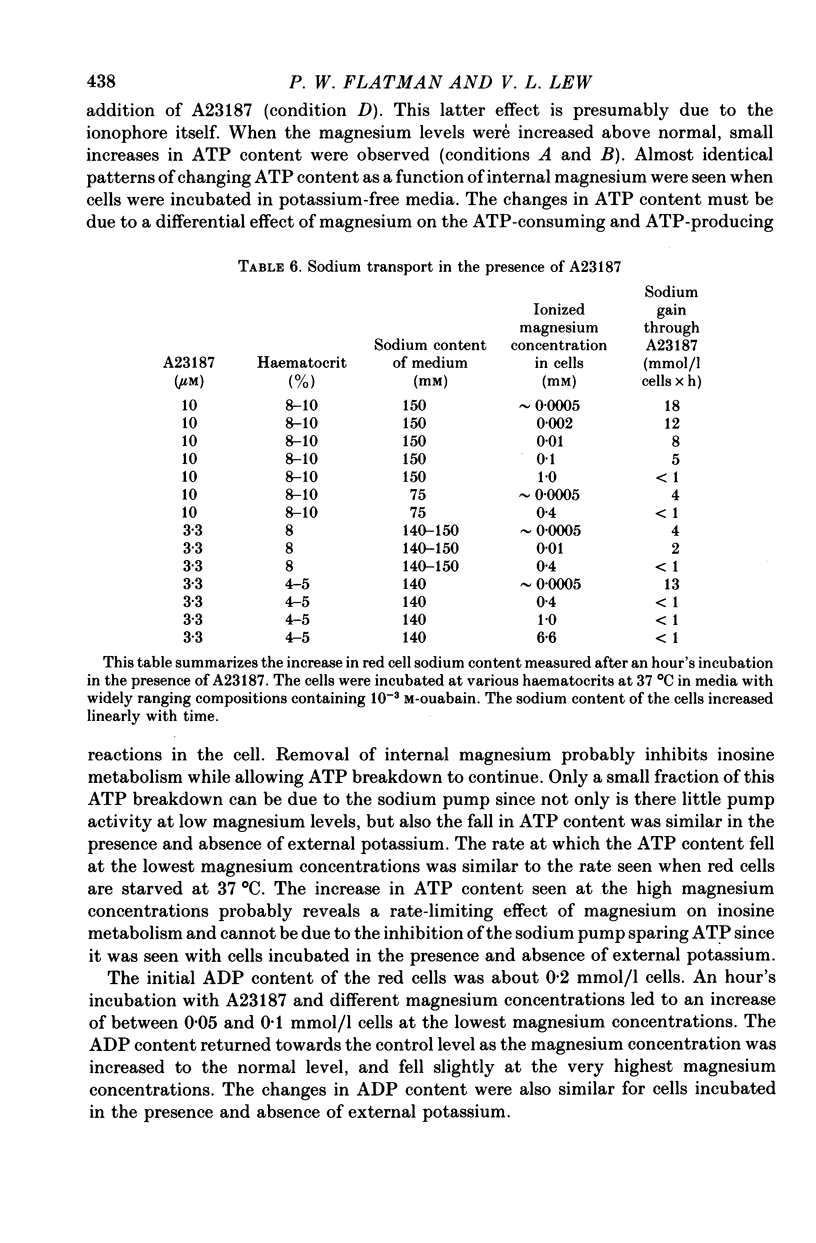
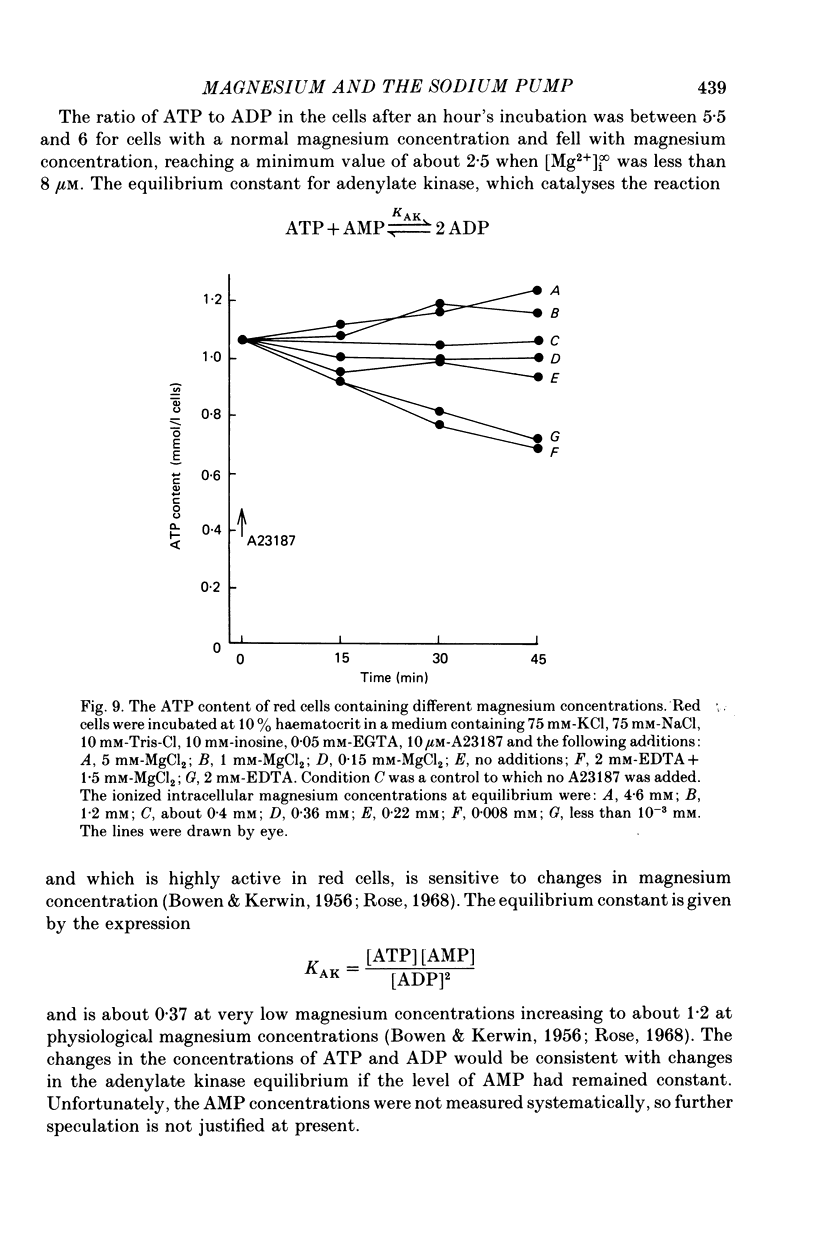
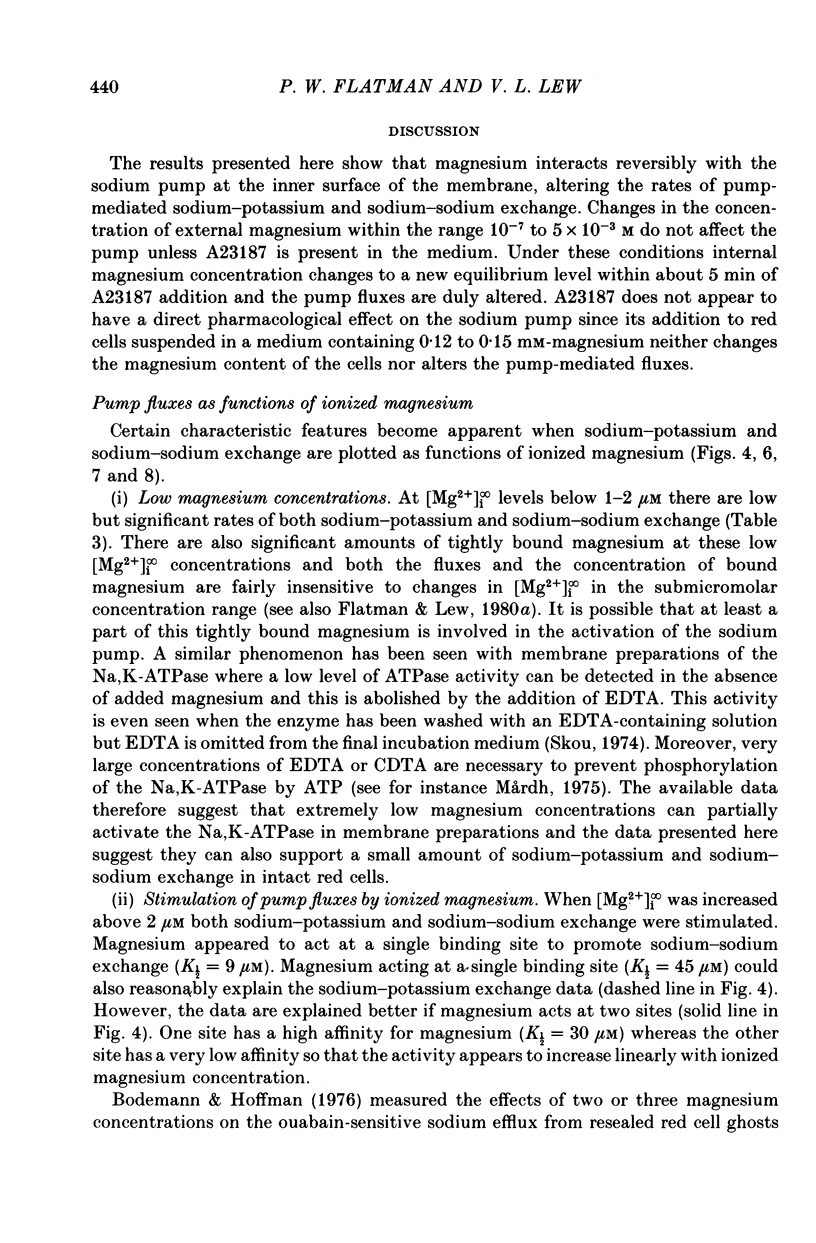
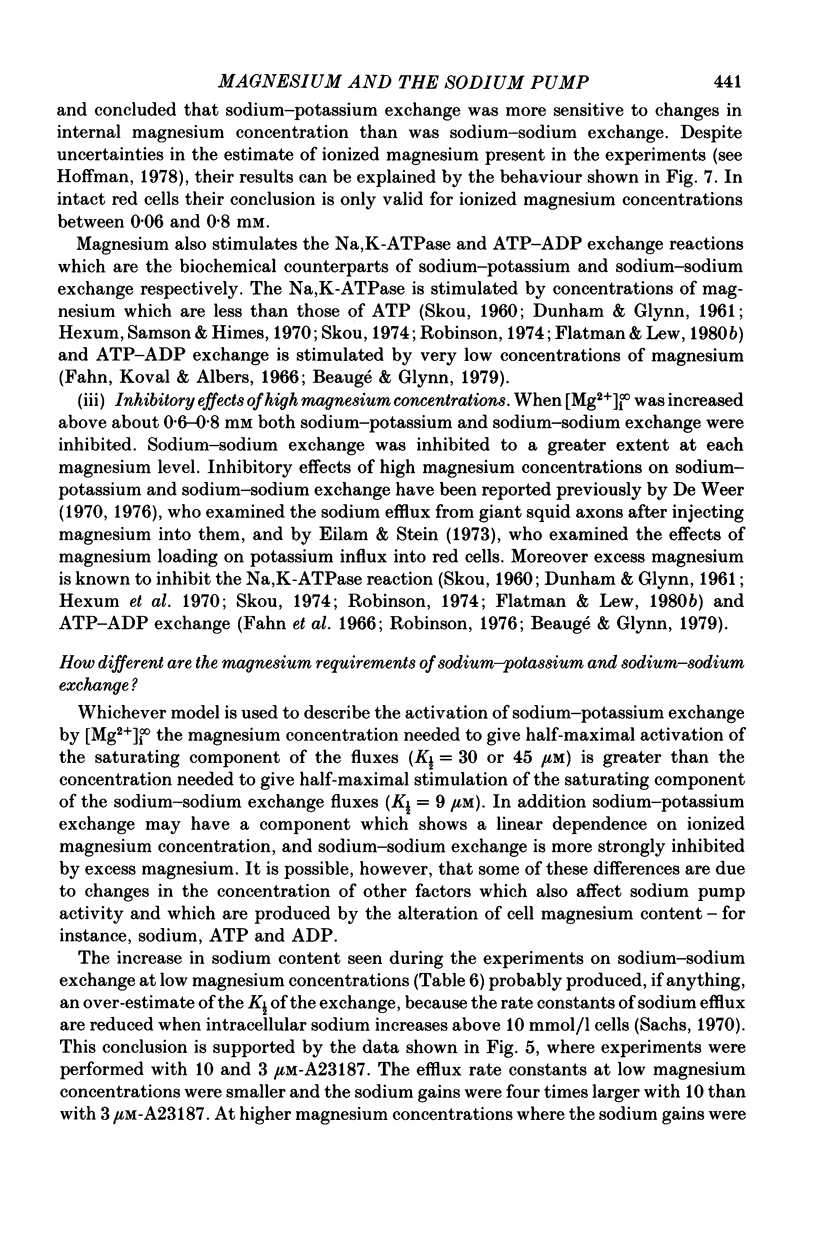
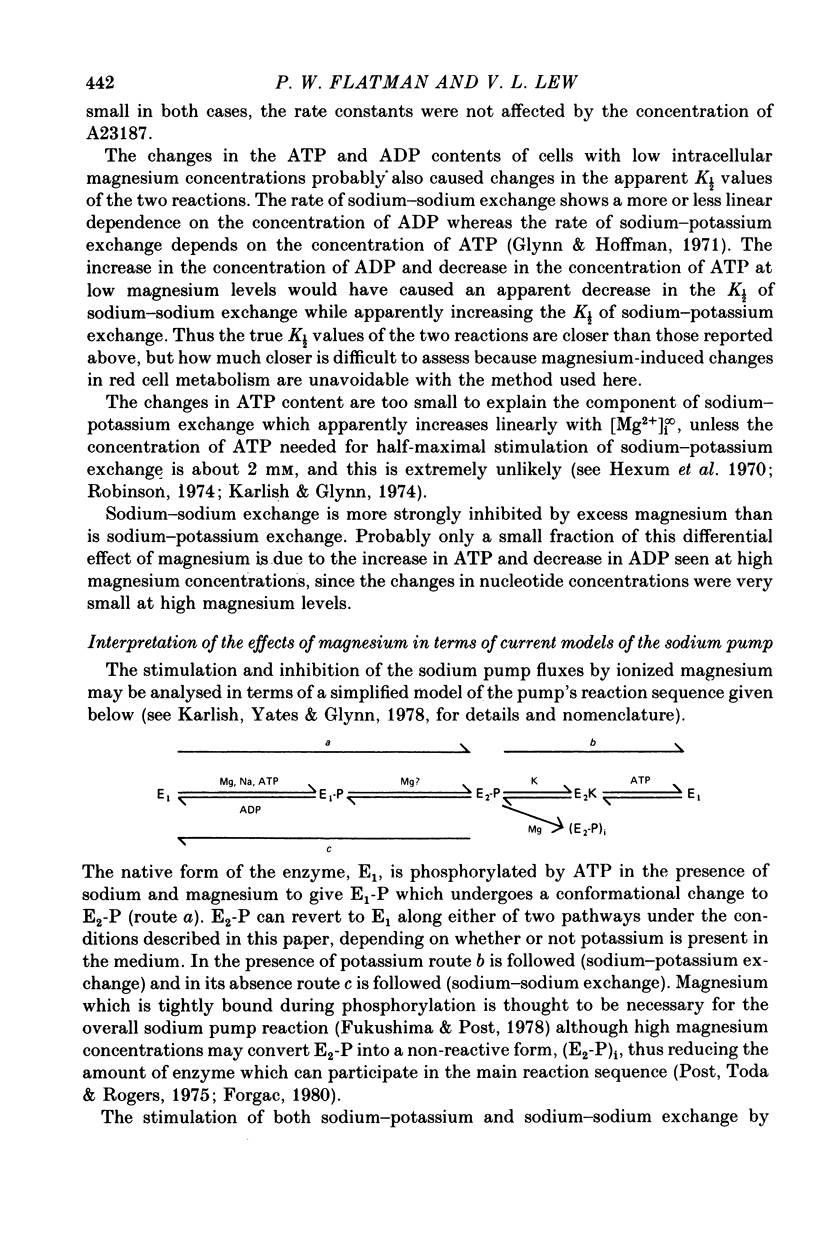
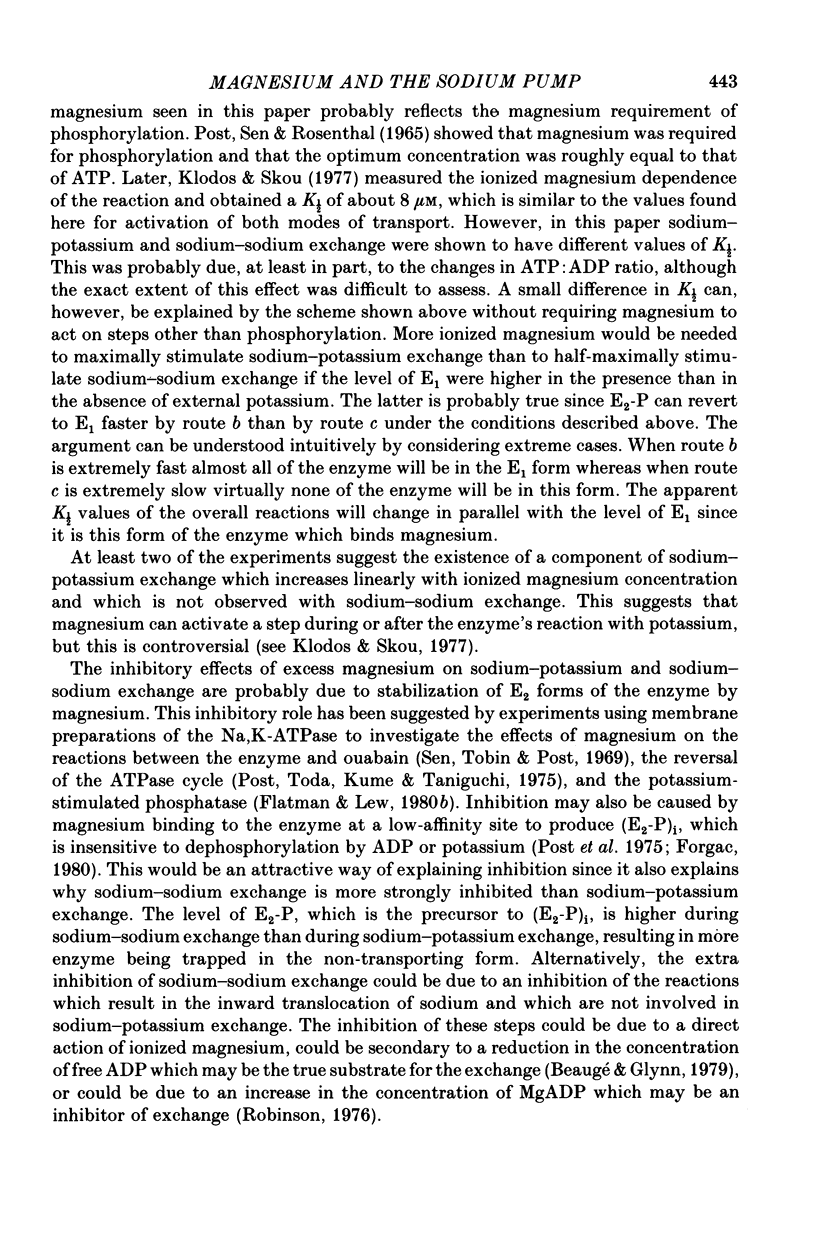
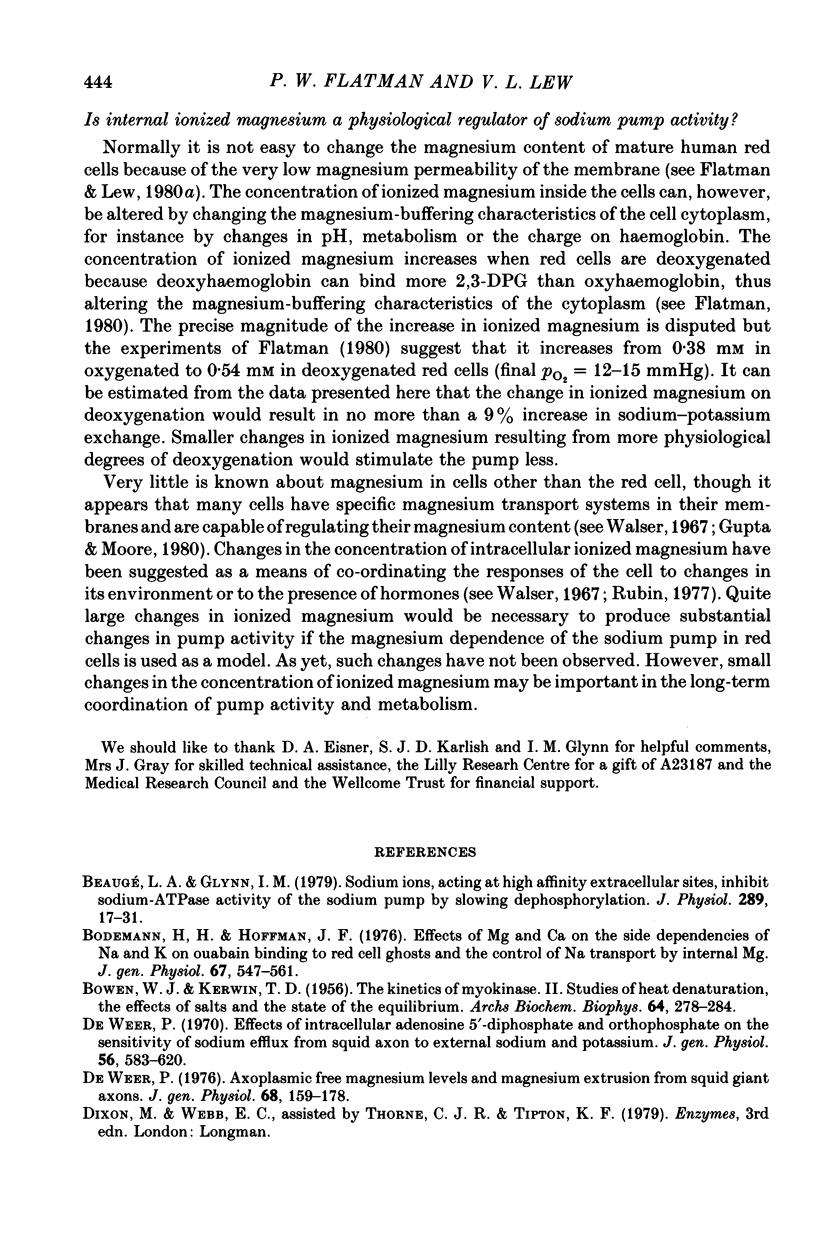
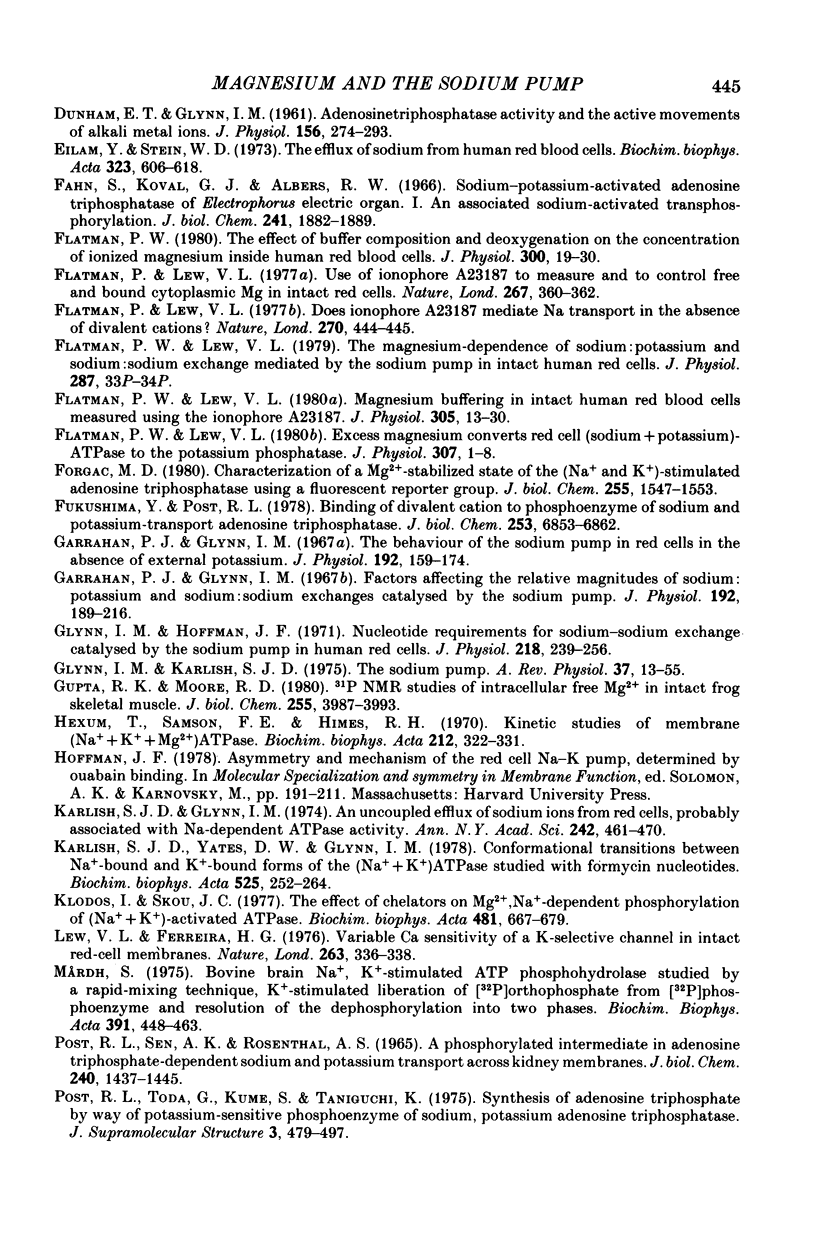
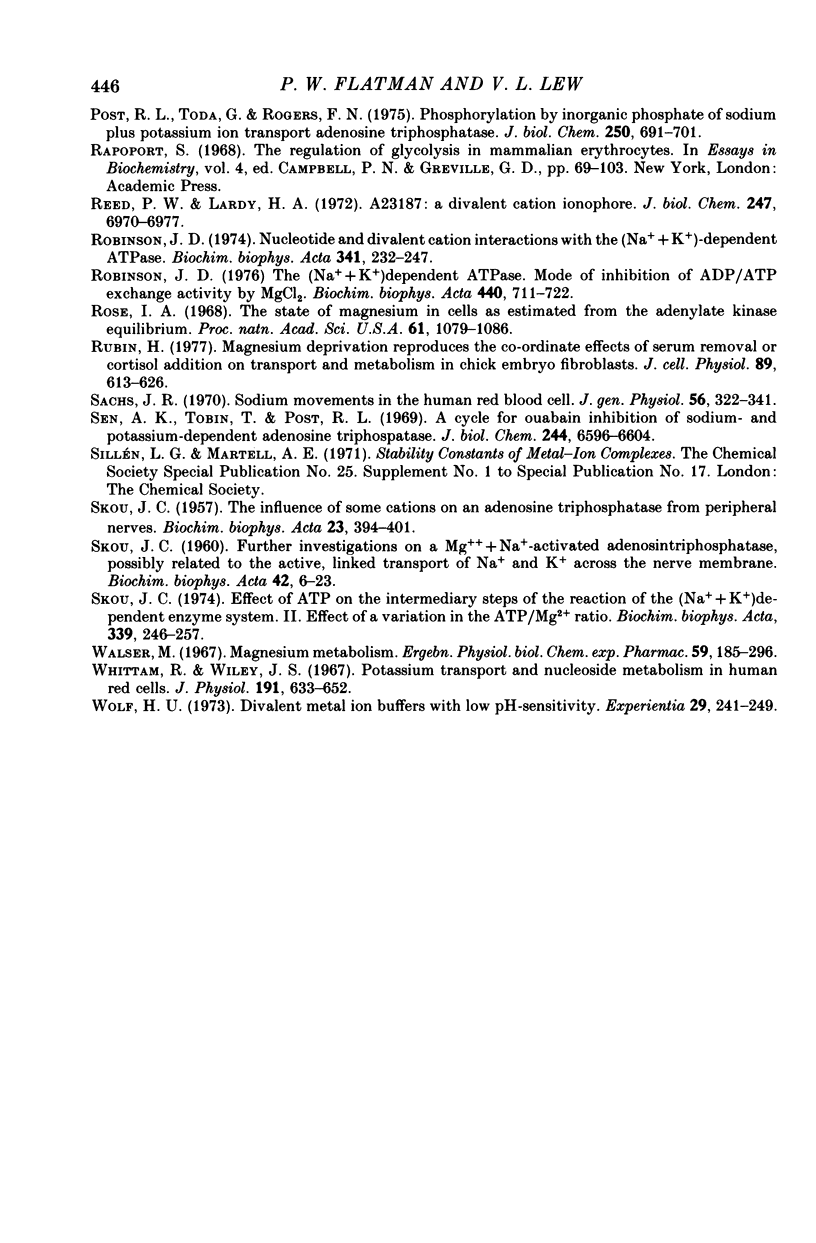
Selected References
These references are in PubMed. This may not be the complete list of references from this article.
- BOWEN W. J., KERWIN T. D. The kinetics of myokinase. II. Studies of heat denaturation, the effects of salts and the state of equilibrium. Arch Biochem Biophys. 1956 Oct;64(2):278–284. doi: 10.1016/0003-9861(56)90270-3. [DOI] [PubMed] [Google Scholar]
- Beaugé L. A., Glynn I. M. Sodium ions, acting at high-affinity extracellular sites, inhibit sodium-ATPase activity of the sodium pump by slowing dephosphorylation. J Physiol. 1979 Apr;289:17–31. doi: 10.1113/jphysiol.1979.sp012722. [DOI] [PMC free article] [PubMed] [Google Scholar]
- Bodemann H. H., Hoffman J. F. Effects of Mg and Ca on the side dependencies of Na and K on ouabain binding to red blood cell ghosts and the control of Na transport by internal Mg. J Gen Physiol. 1976 May;67(5):547–561. doi: 10.1085/jgp.67.5.547. [DOI] [PMC free article] [PubMed] [Google Scholar]
- DUNHAM E. T., GLYNN I. M. Adenosinetriphosphatase activity and the active movements of alkali metal ions. J Physiol. 1961 Apr;156:274–293. doi: 10.1113/jphysiol.1961.sp006675. [DOI] [PMC free article] [PubMed] [Google Scholar]
- De Weer P. Axoplasmic free magnesium levels and magnesium extrusion from squid giant axons. J Gen Physiol. 1976 Aug;68(2):159–178. doi: 10.1085/jgp.68.2.159. [DOI] [PMC free article] [PubMed] [Google Scholar]
- De Weer P. Effects of intracellular adenosine-5'-diphosphate and orthophosphate on the sensitivity of sodium efflux from squid axon to external sodium and potassium. J Gen Physiol. 1970 Nov;56(5):583–620. doi: 10.1085/jgp.56.5.583. [DOI] [PMC free article] [PubMed] [Google Scholar]
- Eilam Y., Stein W. D. The efflux of sodium from human red blood cells. Biochim Biophys Acta. 1973 Nov 16;323(4):606–619. doi: 10.1016/0005-2736(73)90169-7. [DOI] [PubMed] [Google Scholar]
- Fahn S., Koval G. J., Albers R. W. Sodium-potassium-activated adenosine triphosphatase of Electrophorus electric organ. I. An associated sodium-activated transphosphorylation. J Biol Chem. 1966 Apr 25;241(8):1882–1889. [PubMed] [Google Scholar]
- Flatman P. W., Lew V. L. Excess magnesium converts red cell (sodium+potassium) ATPase to the potassium phosphatase. J Physiol. 1980 Oct;307:1–8. doi: 10.1113/jphysiol.1980.sp013419. [DOI] [PMC free article] [PubMed] [Google Scholar]
- Flatman P. W., Lew V. L. Magnesium buffering in intact human red blood cells measured using the ionophore A23187. J Physiol. 1980 Aug;305:13–30. doi: 10.1113/jphysiol.1980.sp013346. [DOI] [PMC free article] [PubMed] [Google Scholar]
- Flatman P. W., Lew V. L. The magnesium-dependence of sodium:potassium and sodium:sodium exchange mediated by the sodium pump in intact human red cells [proceedings]. J Physiol. 1979 Feb;287:33P–34P. [PubMed] [Google Scholar]
- Flatman P. W. The effect of buffer composition and deoxygenation on the concentration of ionized magnesium inside human red blood cells. J Physiol. 1980 Mar;300:19–30. doi: 10.1113/jphysiol.1980.sp013148. [DOI] [PMC free article] [PubMed] [Google Scholar]
- Flatman P., Lew V. L. Does ionophore A23187 mediate Na transport in the absence of divalent cations? Nature. 1977 Dec 1;270(5636):444–445. doi: 10.1038/270444a0. [DOI] [PubMed] [Google Scholar]
- Flatman P., Lew V. L. Use of ionophore A23187 to measure and to control free and bound cytoplasmic Mg in intact red cells. Nature. 1977 May 26;267(5609):360–362. doi: 10.1038/267360a0. [DOI] [PubMed] [Google Scholar]
- Forgac M. D. Characterization of a Mg2+-stabilized state of the (Na+ and K+)--stimulated adenosine triphosphatase using a fluorescent reporter group. J Biol Chem. 1980 Feb 25;255(4):1547–1553. [PubMed] [Google Scholar]
- Fukushima Y., Post R. L. Binding of divalent cation to phosphoenzyme of sodium- and potassium-transport adenosine triphosphatase. J Biol Chem. 1978 Oct 10;253(19):6853–6862. [PubMed] [Google Scholar]
- Garrahan P. J., Glynn I. M. Facftors affecting the relative magnitudes of the sodium:potassium and sodium:sodium exchanges catalysed by the sodium pump. J Physiol. 1967 Sep;192(1):189–216. doi: 10.1113/jphysiol.1967.sp008296. [DOI] [PMC free article] [PubMed] [Google Scholar]
- Garrahan P. J., Glynn I. M. The behaviour of the sodium pump in red cells in the absence of external potassium. J Physiol. 1967 Sep;192(1):159–174. doi: 10.1113/jphysiol.1967.sp008294. [DOI] [PMC free article] [PubMed] [Google Scholar]
- Glynn I. M., Hoffman J. F. Nucleotide requirements for sodium-sodium exchange catalysed by the sodium pump in human red cells. J Physiol. 1971 Oct;218(1):239–256. doi: 10.1113/jphysiol.1971.sp009612. [DOI] [PMC free article] [PubMed] [Google Scholar]
- Glynn I. M., Karlish S. J. The sodium pump. Annu Rev Physiol. 1975;37:13–55. doi: 10.1146/annurev.ph.37.030175.000305. [DOI] [PubMed] [Google Scholar]
- Gupta R. K., Moore R. D. 31P NMR studies of intracellular free Mg2+ in intact frog skeletal muscle. J Biol Chem. 1980 May 10;255(9):3987–3993. [PubMed] [Google Scholar]
- Hexum T., Samson F. E., Jr, Himes R. H. Kinetic studies of membrane (Na+-K+-Mg2+)-ATPase. Biochim Biophys Acta. 1970 Aug 15;212(2):322–331. doi: 10.1016/0005-2744(70)90213-5. [DOI] [PubMed] [Google Scholar]
- Karlish S. J., Glynn I. M. An uncoupled efflux of sodium ions from human red cells, probably associated with Na-dependent ATPase activity. Ann N Y Acad Sci. 1974;242(0):461–470. doi: 10.1111/j.1749-6632.1974.tb19110.x. [DOI] [PubMed] [Google Scholar]
- Karlish S. J., Yates D. W., Glynn I. M. Conformational transitions between Na+-bound and K+-bound forms of (Na+ + K+)-ATPase, studied with formycin nucleotides. Biochim Biophys Acta. 1978 Jul 7;525(1):252–264. doi: 10.1016/0005-2744(78)90219-x. [DOI] [PubMed] [Google Scholar]
- Klodos I., Skou J. C. The effect of chelators on Mg2+, Na+-dependent phosphorylation of (Na+ + K+)-activated ATPase. Biochim Biophys Acta. 1977 Apr 12;481(2):667–679. doi: 10.1016/0005-2744(77)90300-x. [DOI] [PubMed] [Google Scholar]
- Lew V. L., Ferreira H. G. Variable Ca sensitivity of a K-selective channel in intact red-cell membranes. Nature. 1976 Sep 23;263(5575):336–338. doi: 10.1038/263336a0. [DOI] [PubMed] [Google Scholar]
- Mårdh S. Bovine brain Na+,K+-stimulated ATP phosphohydrolase studied by a rapid-mixing technique. K+-stimulated liberation of [32P] orthophosphate from [32P] phosphoenzyme and resolution of the dephosphorylation into two phases. Biochim Biophys Acta. 1975 Jun 24;391(2):448–463. doi: 10.1016/0005-2744(75)90269-7. [DOI] [PubMed] [Google Scholar]
- POST R. L., SEN A. K., ROSENTHAL A. S. A PHOSPHORYLATED INTERMEDIATE IN ADENOSINE TRIPHOSPHATE-DEPENDENT SODIUM AND POTASSIUM TRANSPORT ACROSS KIDNEY MEMBRANES. J Biol Chem. 1965 Mar;240:1437–1445. [PubMed] [Google Scholar]
- Post R. L., Toda G., Kume S., Taniguchi K. Synthesis of adenosine triphosphate by way of potassium-sensitive phosphoenzyme of sodium, potassium adenosine triphosphatase. J Supramol Struct. 1975;3(5-6):479–497. doi: 10.1002/jss.400030508. [DOI] [PubMed] [Google Scholar]
- Post R. L., Toda G., Rogers F. N. Phosphorylation by inorganic phosphate of sodium plus potassium ion transport adenosine triphosphatase. Four reactive states. J Biol Chem. 1975 Jan 25;250(2):691–701. [PubMed] [Google Scholar]
- Rapoport S. The regulation of glycolysis in mammalian erythrocytes. Essays Biochem. 1968;4:69–103. [PubMed] [Google Scholar]
- Reed P. W., Lardy H. A. A23187: a divalent cation ionophore. J Biol Chem. 1972 Nov 10;247(21):6970–6977. [PubMed] [Google Scholar]
- Robinson J. D. Nucleotide and divalent cation interactions with the (Na+ plus K+)-dependent ATPase. Biochim Biophys Acta. 1974 Mar 21;341(1):232–247. doi: 10.1016/0005-2744(74)90084-9. [DOI] [PubMed] [Google Scholar]
- Robinson J. D. The (Na + K+)-dependent ATPase. Mode of inhibition of ADP/ATP exchange activity by MgC12. Biochim Biophys Acta. 1976 Sep 13;440(3):711–722. doi: 10.1016/0005-2728(76)90053-0. [DOI] [PubMed] [Google Scholar]
- Rose I. A. The state of magnesium in cells as estimated from the adenylate kinase equilibrium. Proc Natl Acad Sci U S A. 1968 Nov;61(3):1079–1086. doi: 10.1073/pnas.61.3.1079. [DOI] [PMC free article] [PubMed] [Google Scholar]
- Rubin H. Magnesium deprivation reproduces the coordinate effects of serum removal or cortisol addition on transport and metabolism in chick embryo fibroblasts. J Cell Physiol. 1976 Dec;89(4):613–625. doi: 10.1002/jcp.1040890418. [DOI] [PubMed] [Google Scholar]
- SKOU J. C. The influence of some cations on an adenosine triphosphatase from peripheral nerves. Biochim Biophys Acta. 1957 Feb;23(2):394–401. doi: 10.1016/0006-3002(57)90343-8. [DOI] [PubMed] [Google Scholar]
- Sachs J. R. Sodium movements in the human red blood cell. J Gen Physiol. 1970 Sep;56(3):322–341. doi: 10.1085/jgp.56.3.322. [DOI] [PMC free article] [PubMed] [Google Scholar]
- Sen A. K., Tobin T. A cycle for ouabain inhibition of sodium- and potassium-dependent adenosine triphosphatase. J Biol Chem. 1969 Dec 25;244(24):6596–6604. [PubMed] [Google Scholar]
- Skou J. C. Effect of ATP on the intermediary steps of the reaction of the (Na+ plusK+)-dependent enzyme system. II. Effect of a variation in the ATP-Mg2+ ratio. Biochim Biophys Acta. 1974 Mar 15;339(2):246–257. doi: 10.1016/0005-2736(74)90322-8. [DOI] [PubMed] [Google Scholar]
- Walser M. Magnesium metabolism. Ergeb Physiol. 1967;59:185–296. doi: 10.1007/BF02269144. [DOI] [PubMed] [Google Scholar]
- Whittam R., Wiley J. S. Potassium transport and nucleoside metabolism in human red cells. J Physiol. 1967 Aug;191(3):633–652. doi: 10.1113/jphysiol.1967.sp008272. [DOI] [PMC free article] [PubMed] [Google Scholar]