Abstract
The electrophysiological properties of the high- and low-threshold Ca spikes described in inferior olivary neurones were analysed in detail. 1. During hyperpolarization the low- and high-threshold Ca action potentials can coexist as two distinct spikes, demonstrating non-mutual exclusion. 2. The high-threshold Ca spike shows a lack of refractoriness, is generated remotely from the site of recording and is composed of several all-or-none components, the last two properties suggesting a dendritic origin. 3. Hyperpolarization of the neurones allows the activation of the low-threshold Ca spike, which has activation properties resembling those of the early K conductance described in invertebrates. This low-threshold Ca spike shows refractoriness. 4. The relation between membrane polarization and low-threshold Ca spike is S-shaped. Low-threshold Ca spikes become apparent at -70 mV and have a maximum rate of rise (saturation) at polarization levels more negative than -85 mV. Thus, hyperpolarization removes a voltage-dependent Ca inactivation which is present at normal resting membrane potential (-65 mV). 5. Replacement of extracellular Ca by Ba or addition of tetraethylammonium to the bath corroborates the lack of fast inactivation for the high-threshold Ca spike and the inactivation properties of the low-threshold Ca conductance. It also demonstrates that the duration of the after-depolarization is determined by an interplay between inward Ca current and both voltage-dependent and Ca-dependent K currents. 6. Extracellular recordings from single cells indicate that the Na-dependent spike and the low-threshold Ca action potential are somatic in origin, while the high-threshold Ca spike (after-depolarization) and the hyperpolarization that follows are apparently located in the dendrites. 7. The ionic conductances comprise the main components of the oscillatory behaviour of these cells. The sequence of events leading to oscillation entails initially a low-threshold Ca spike or Na spike, followed by an after-depolarization/after-hyperpolarization sequence and then a post-anodal exaltation product by a rebound low-threshold Ca spike.
Full text
PDF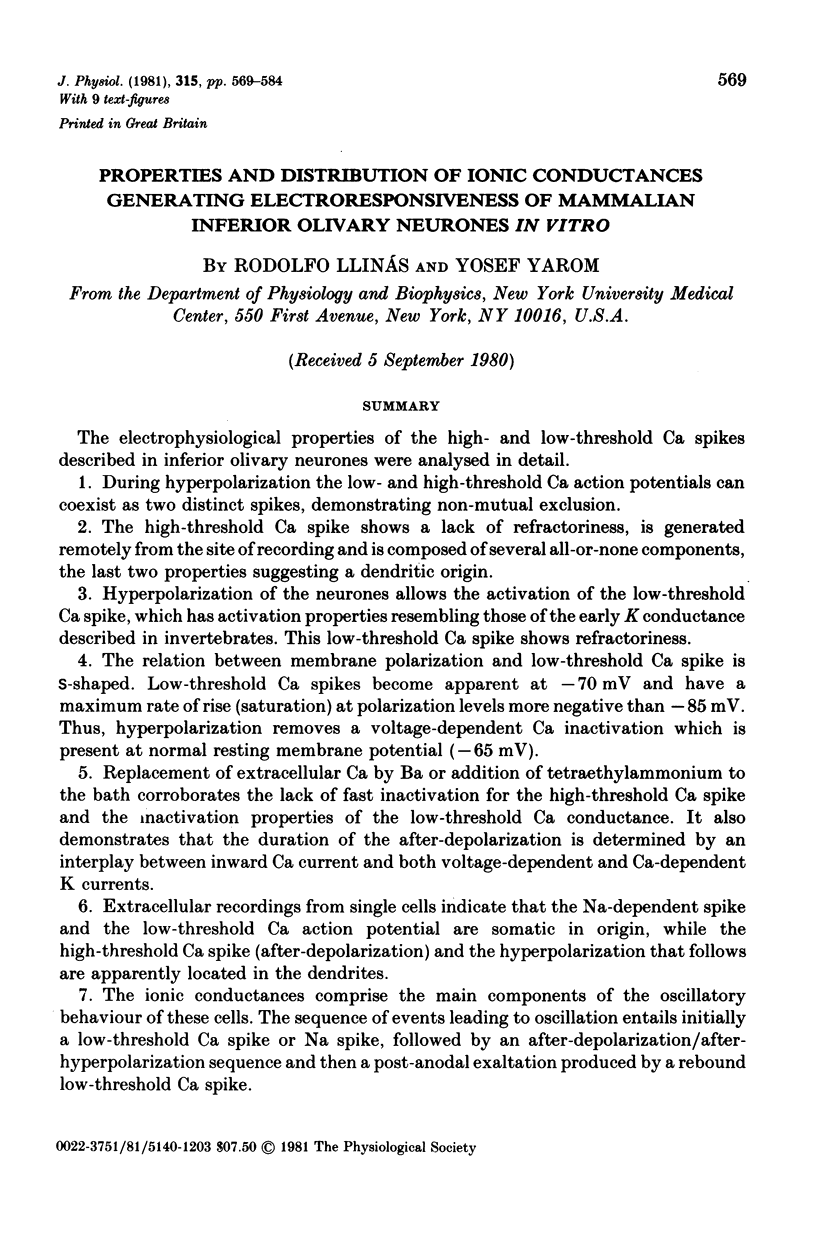
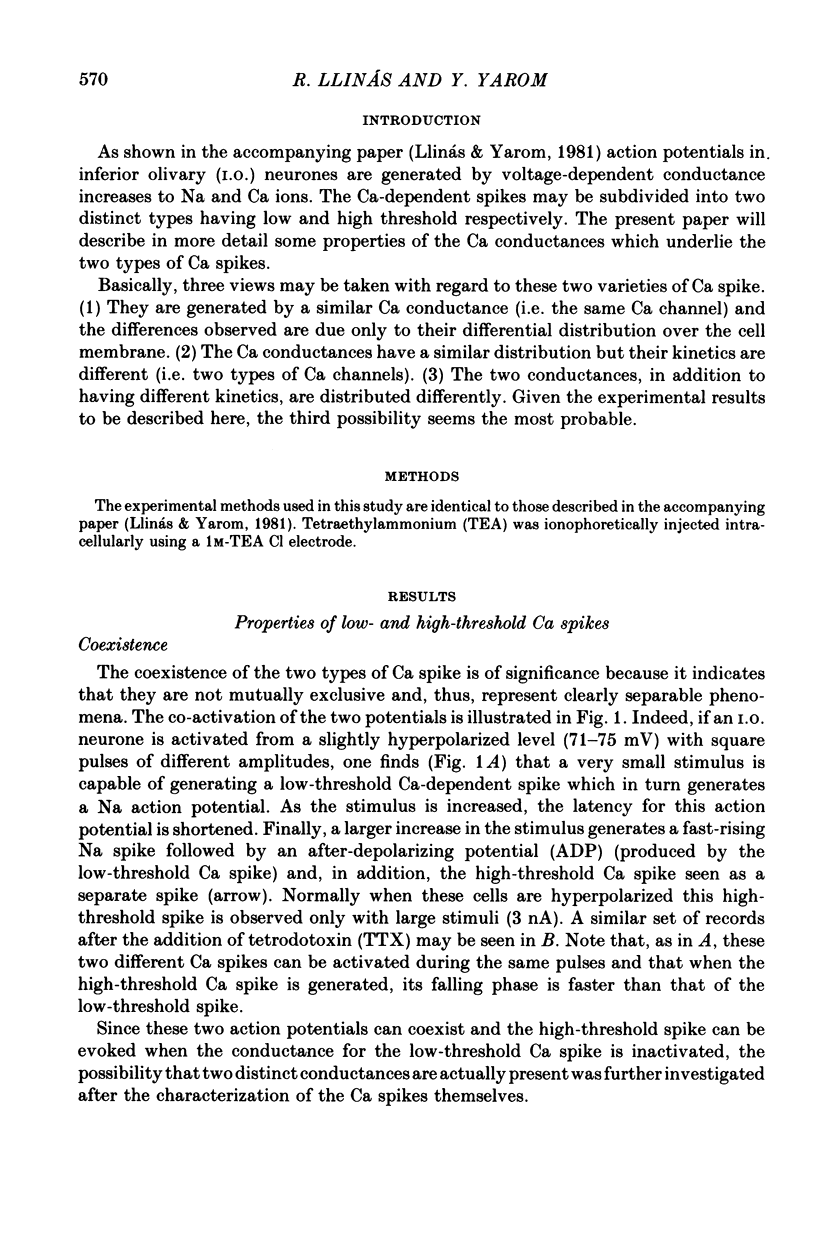
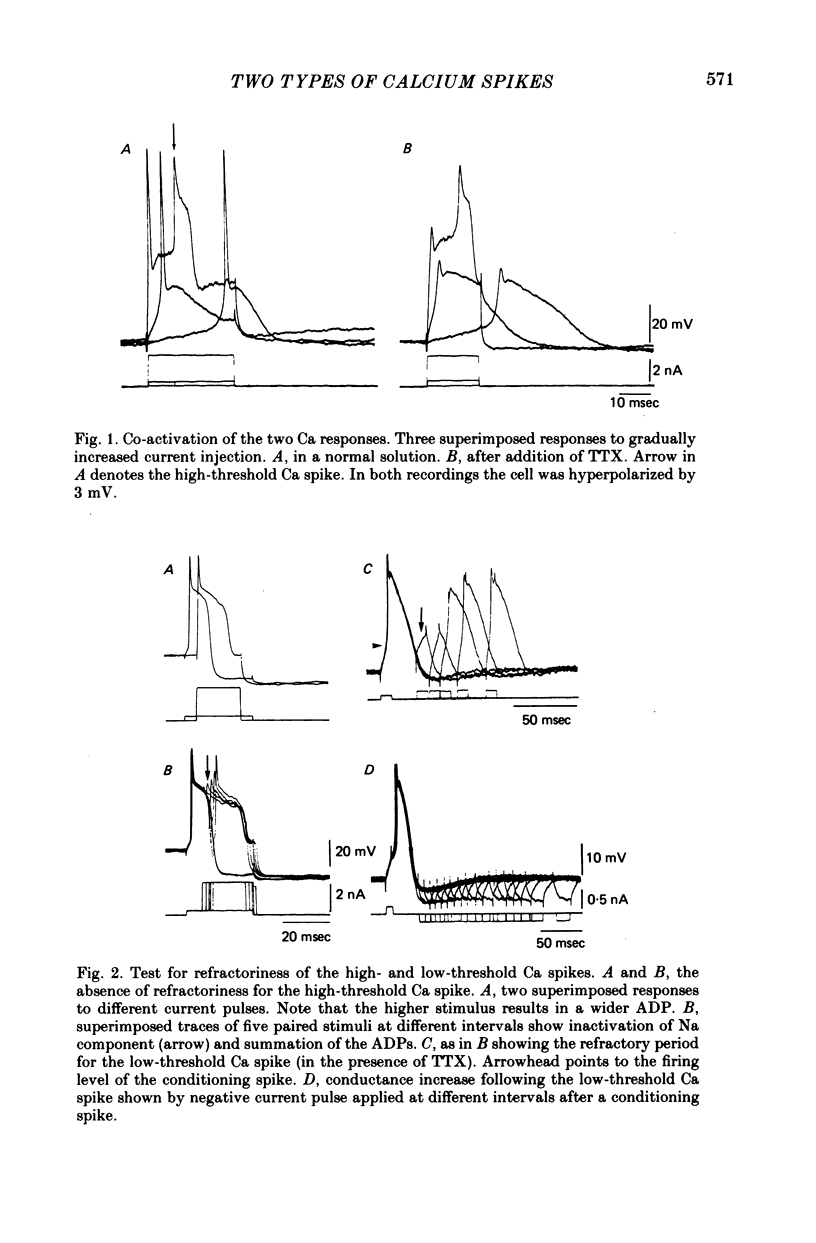
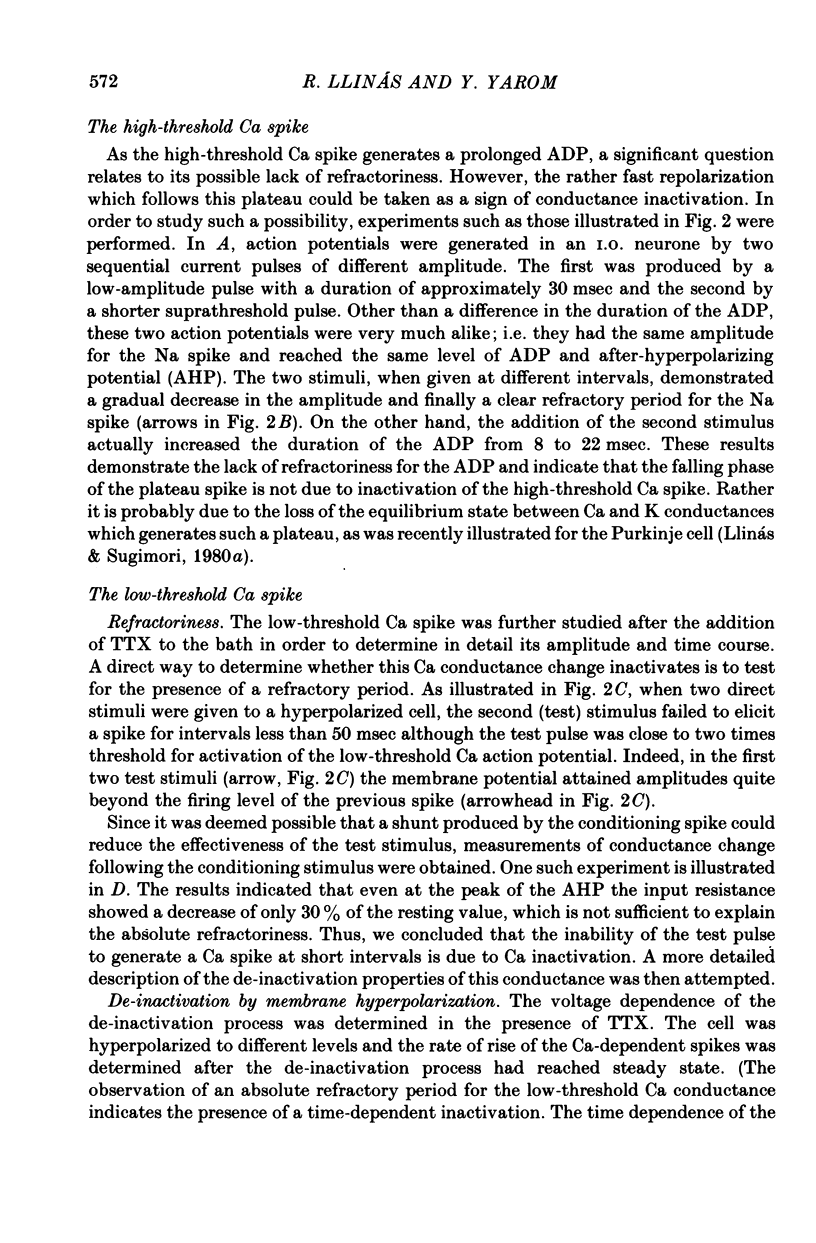
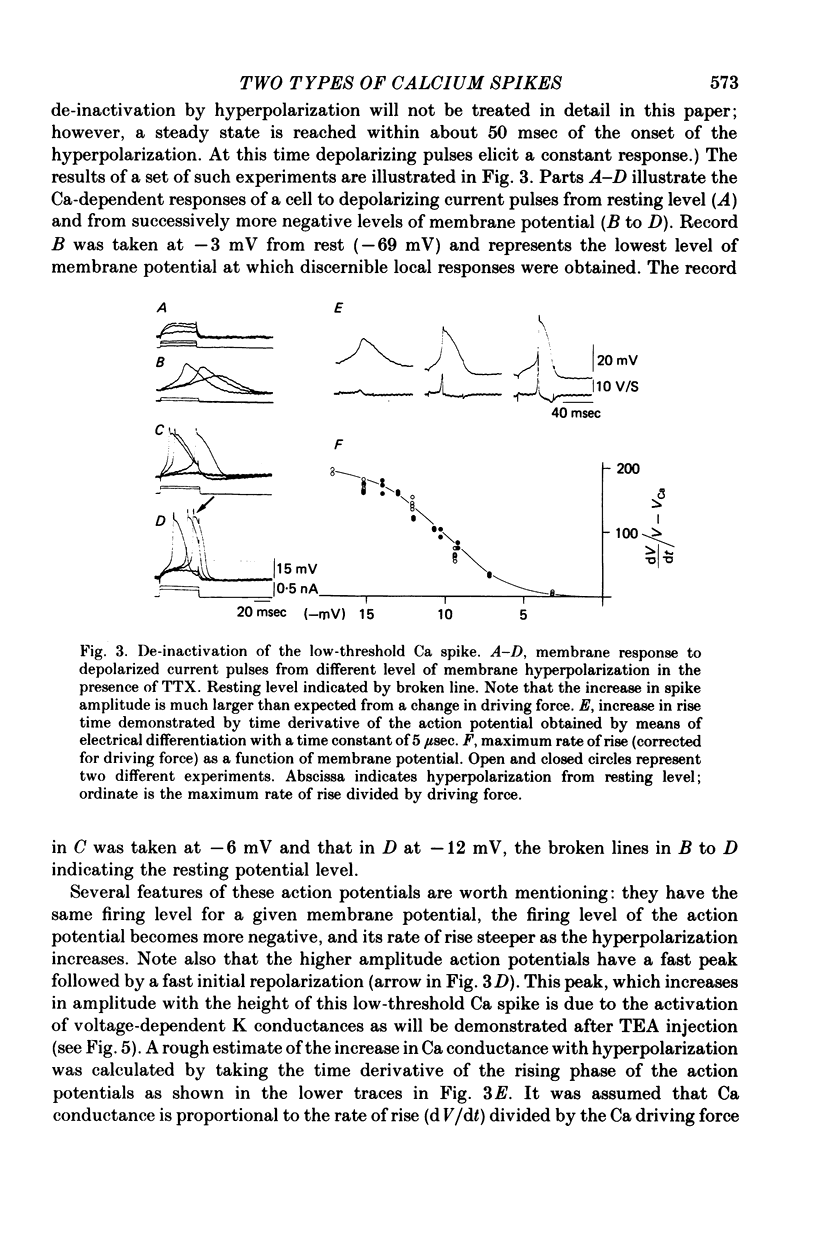
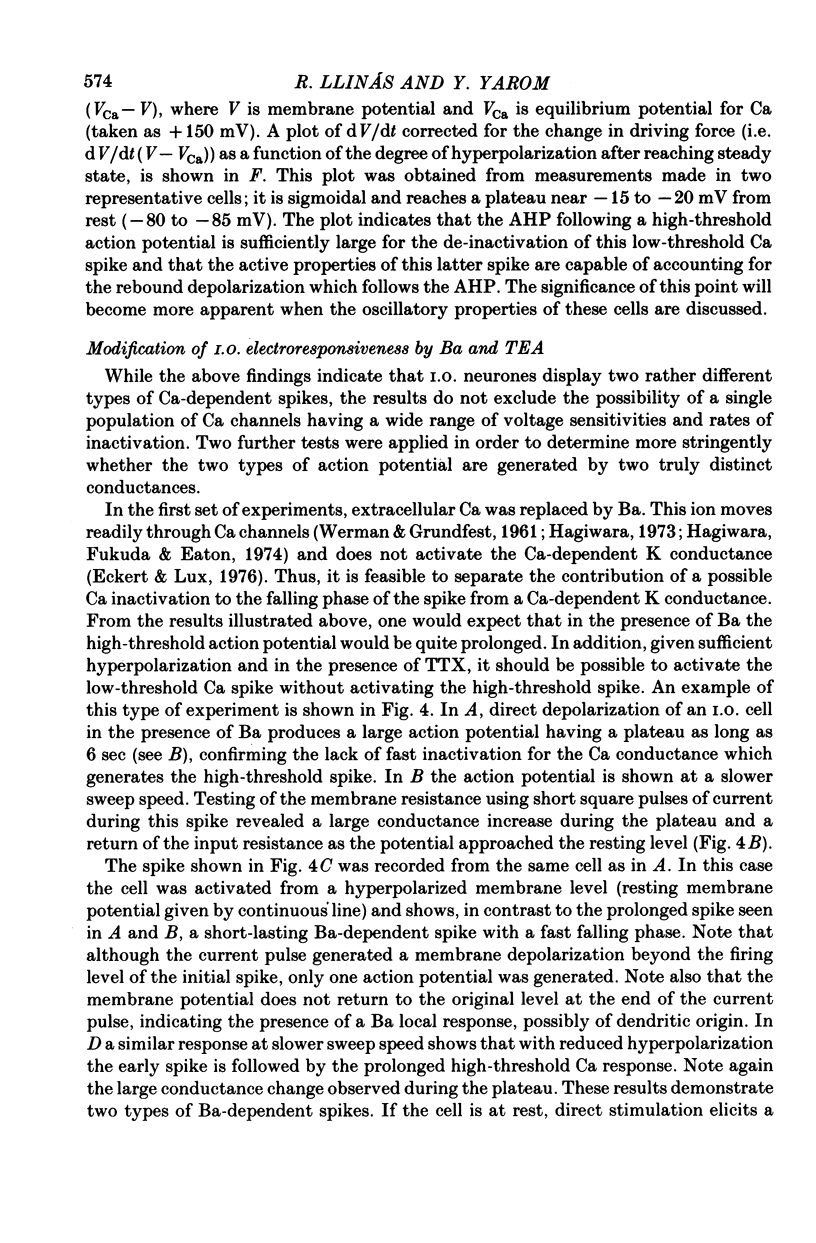
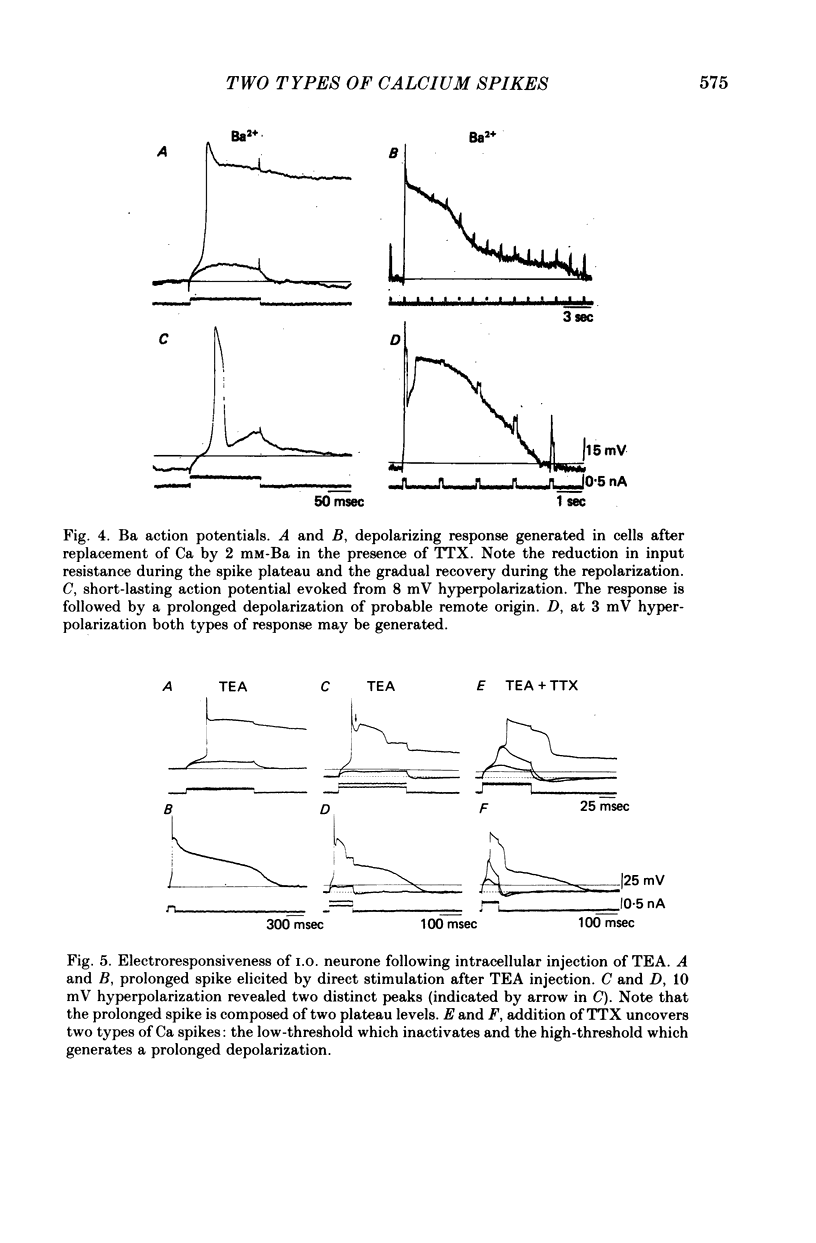
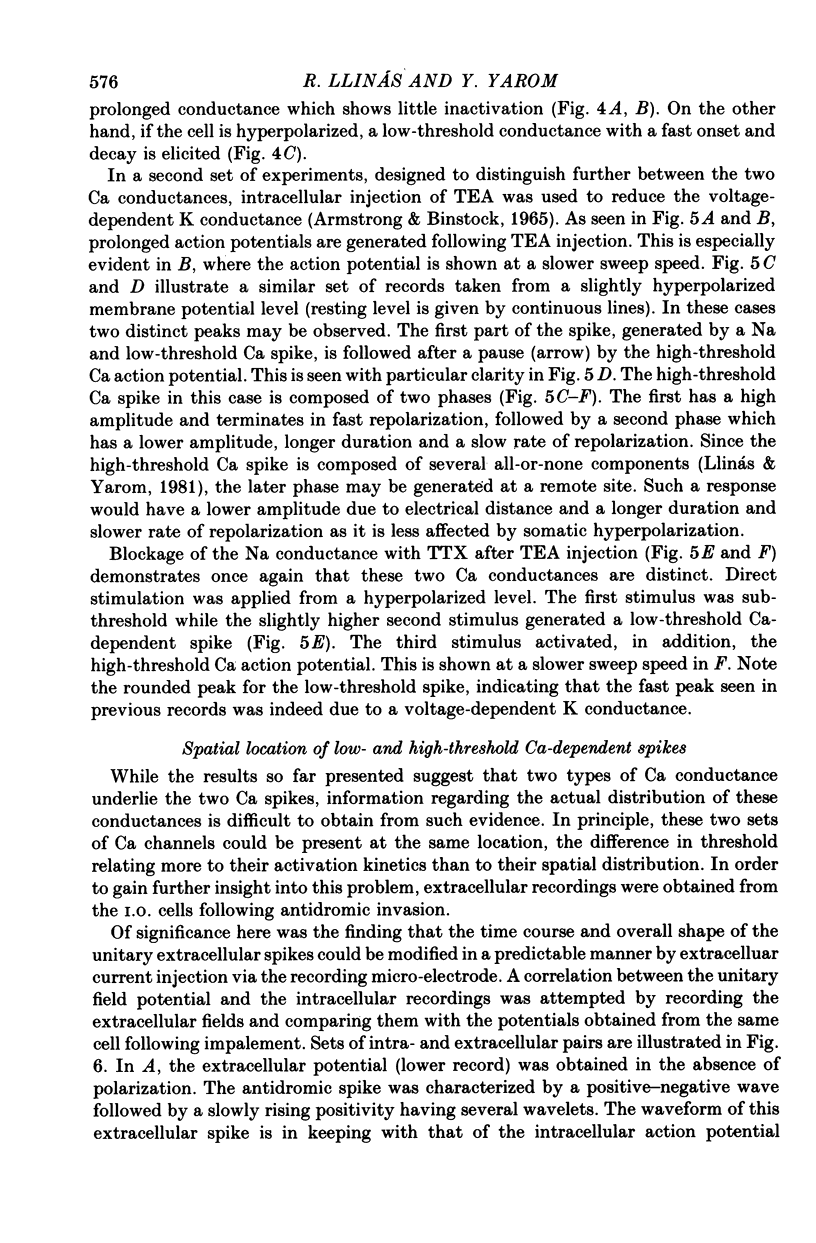
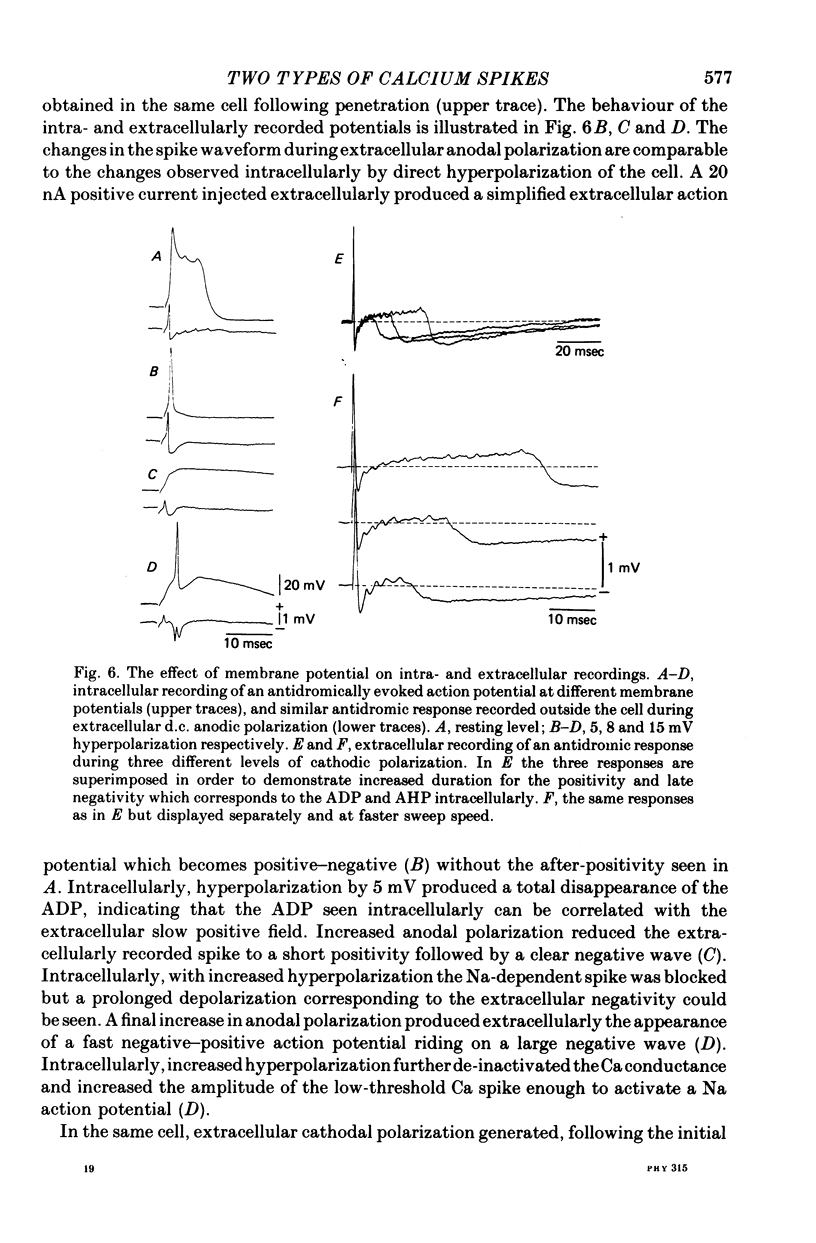
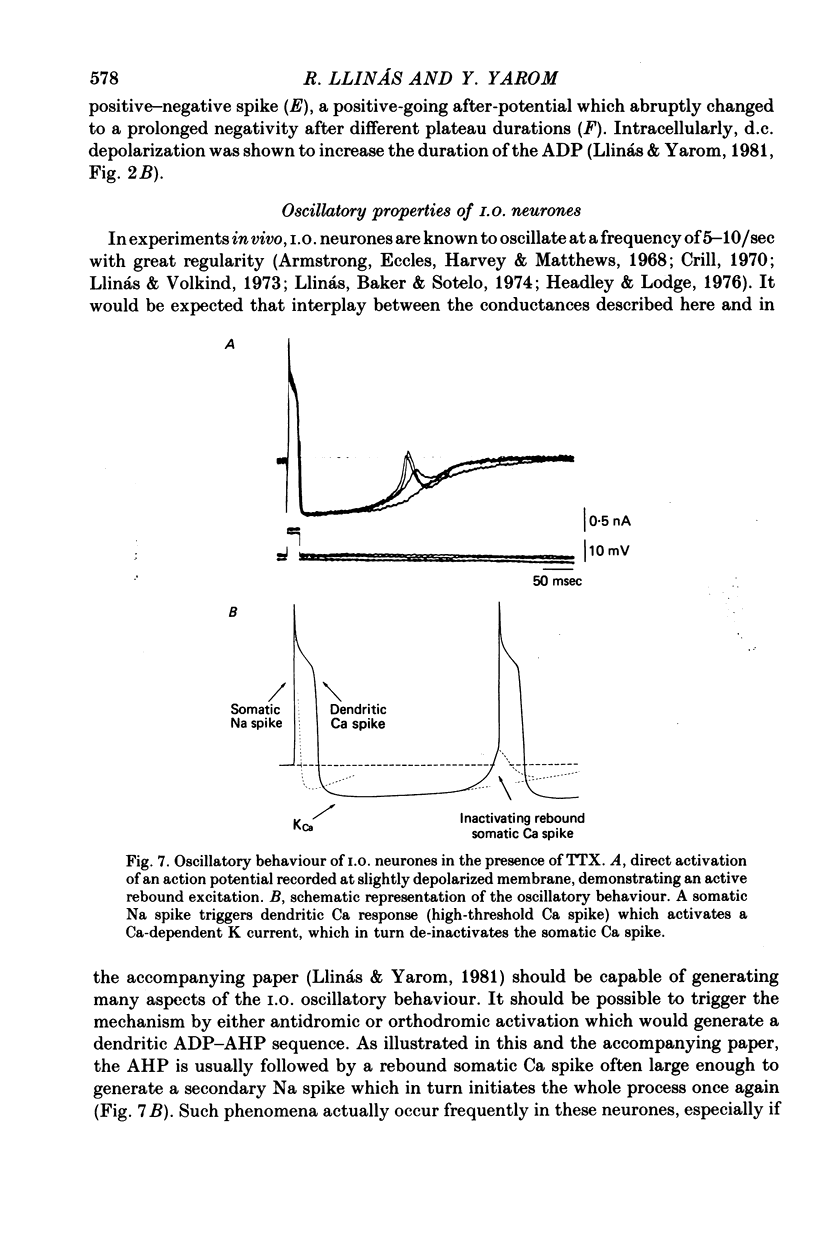
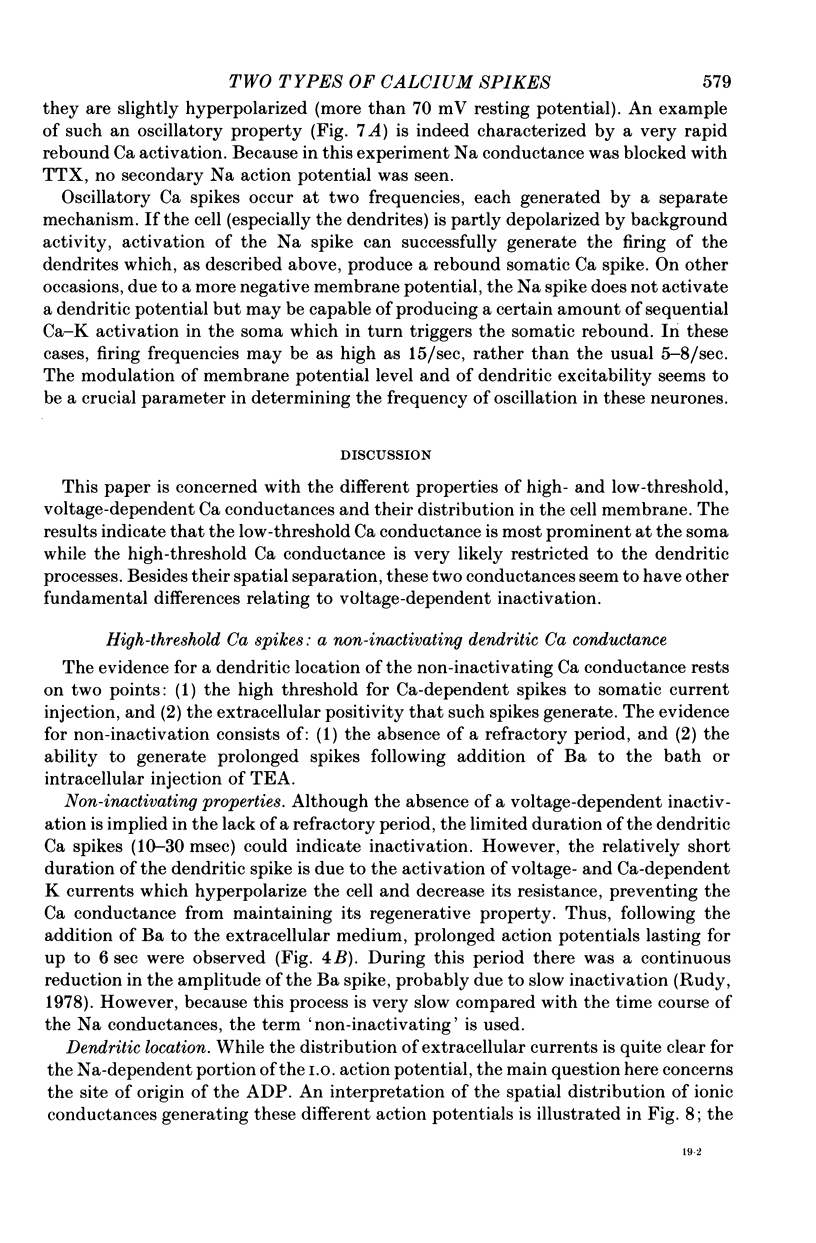
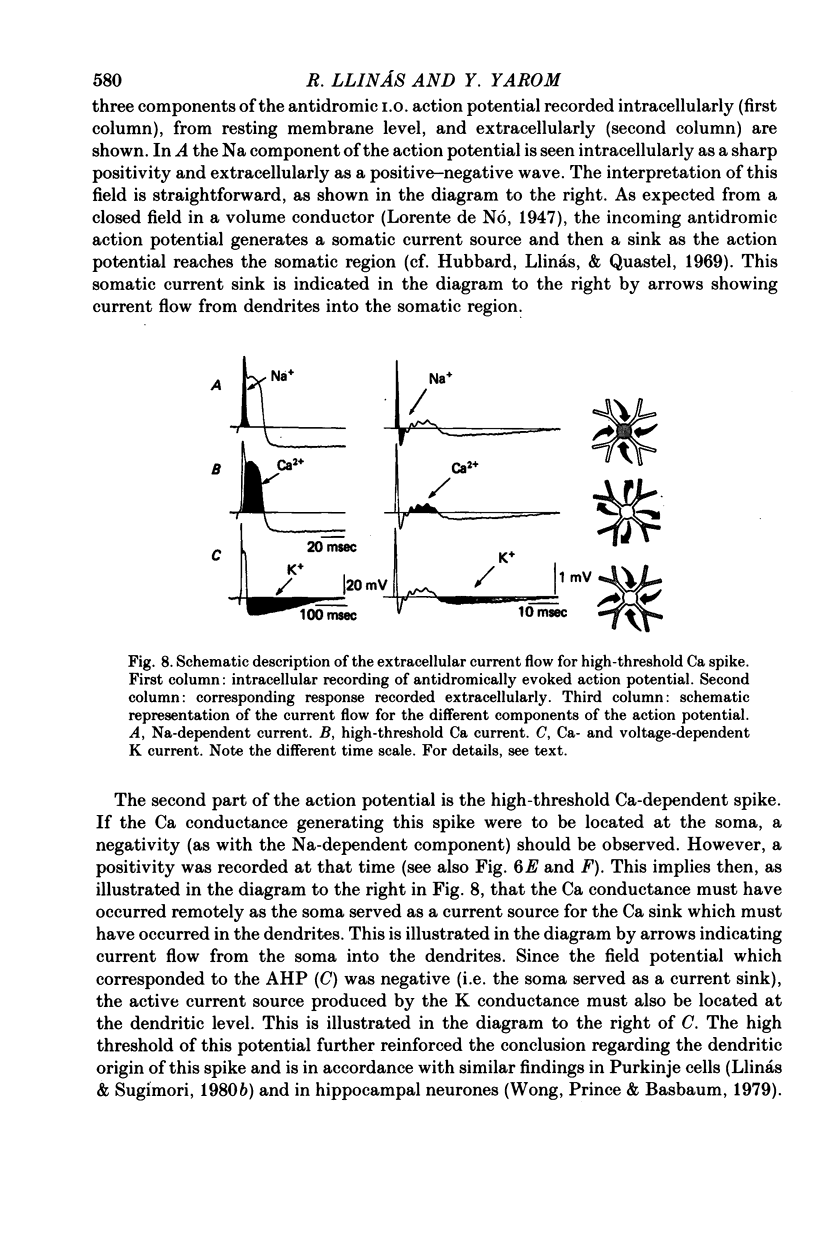
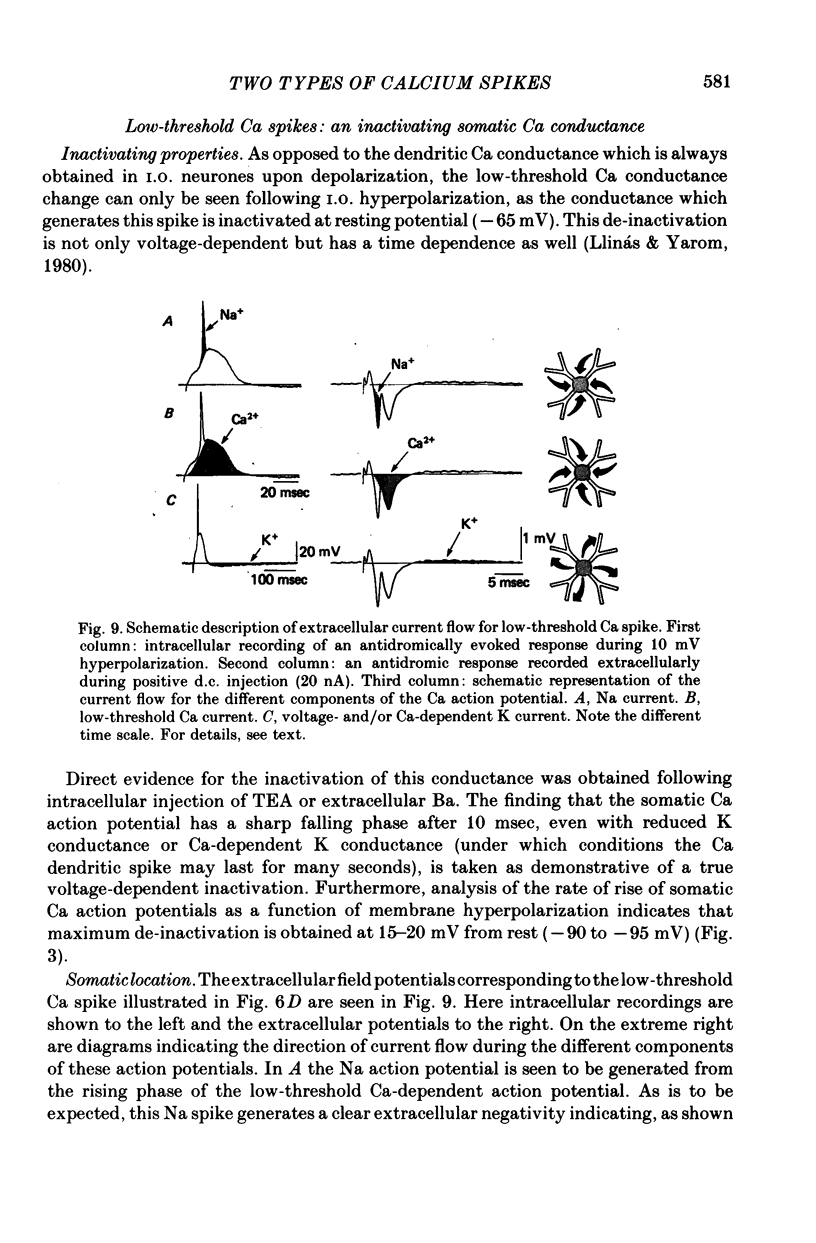
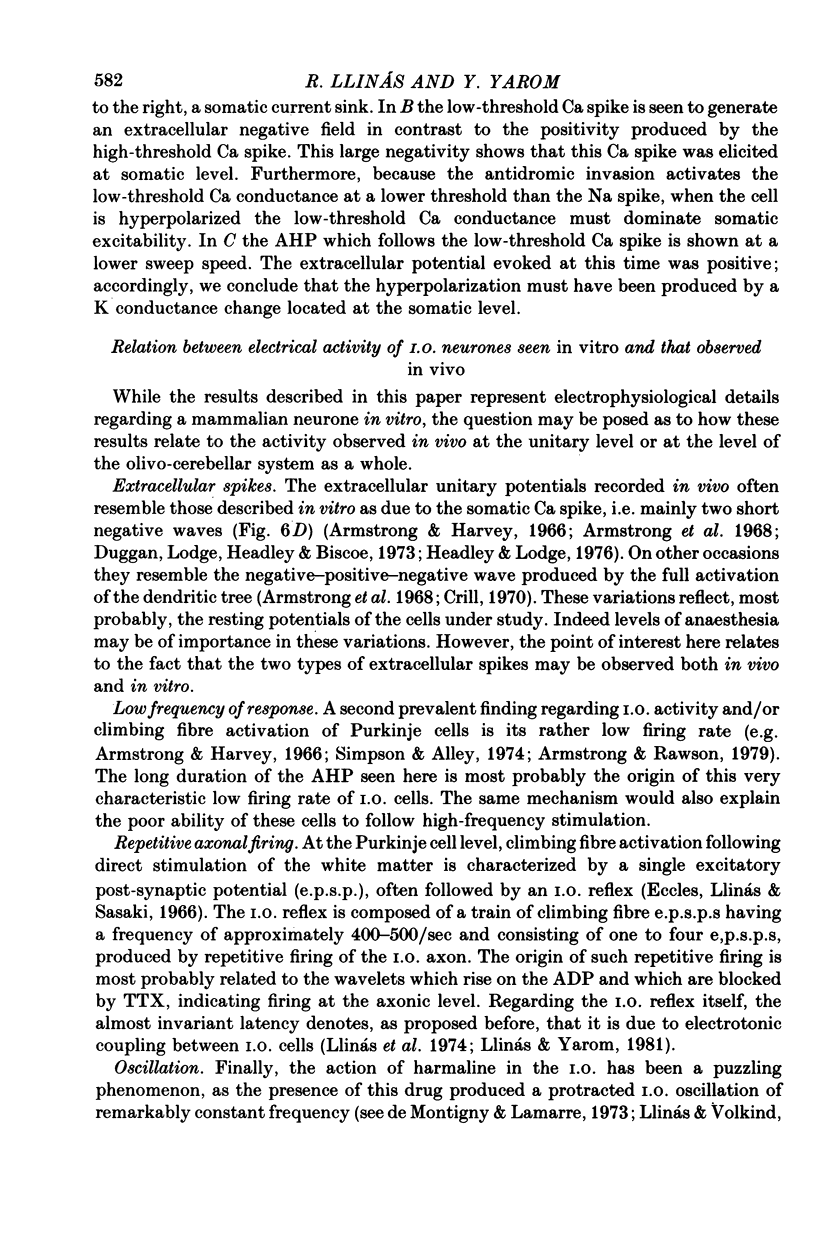
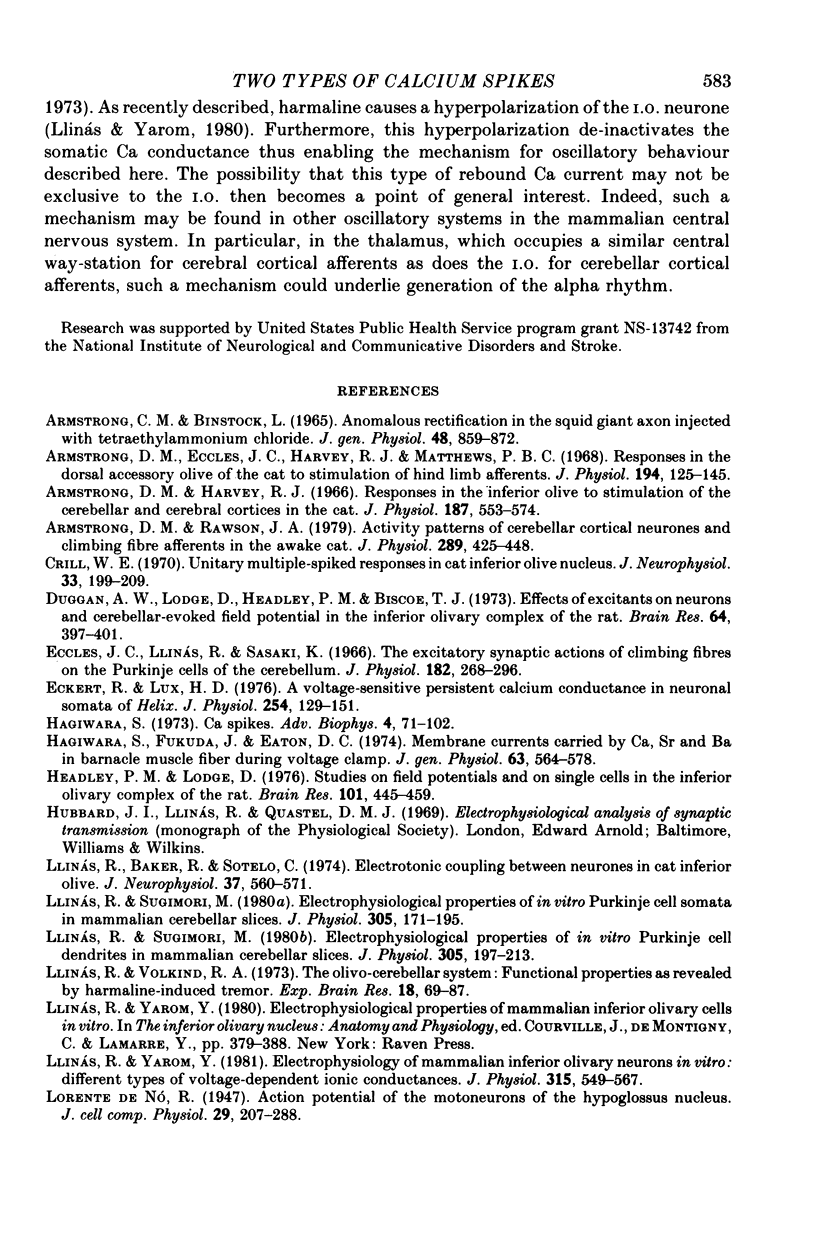
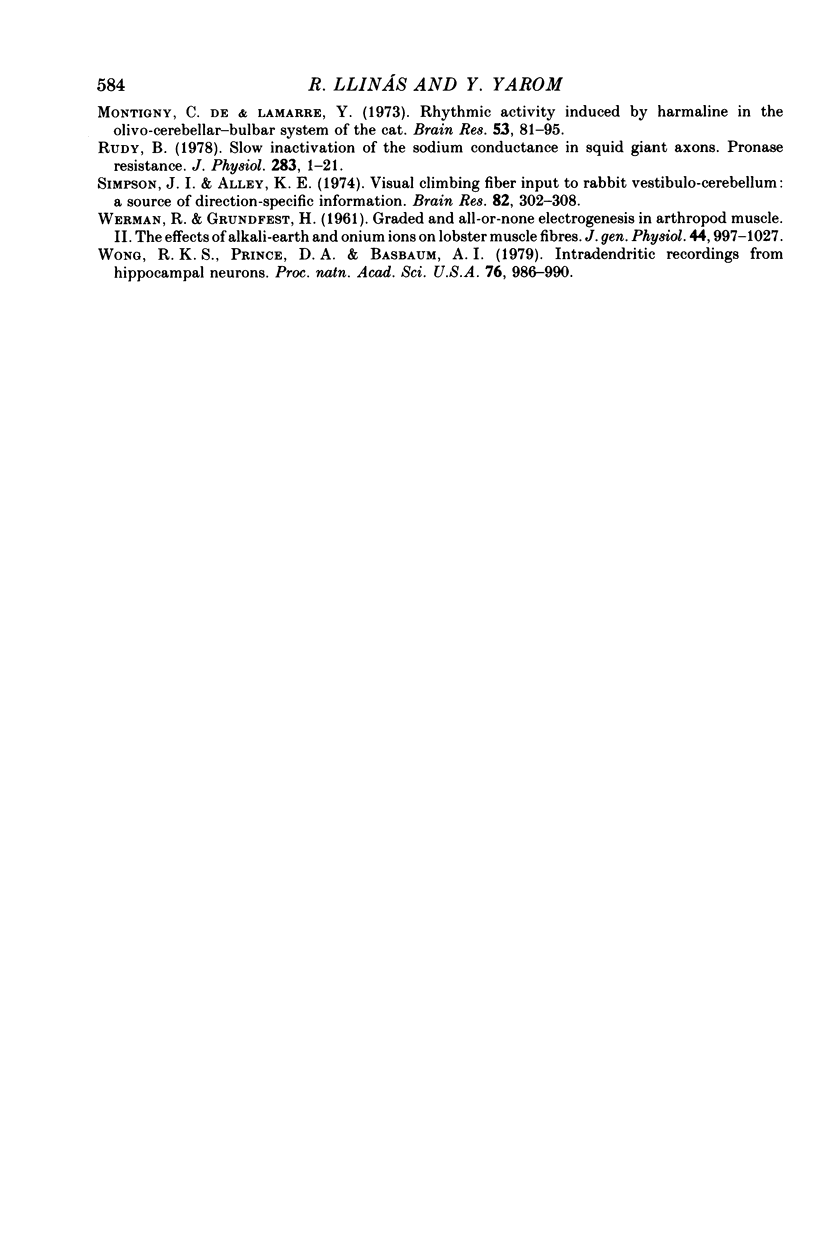
Images in this article
Selected References
These references are in PubMed. This may not be the complete list of references from this article.
- ARMSTRONG C. M., BINSTOCK L. ANOMALOUS RECTIFICATION IN THE SQUID GIANT AXON INJECTED WITH TETRAETHYLAMMONIUM CHLORIDE. J Gen Physiol. 1965 May;48:859–872. doi: 10.1085/jgp.48.5.859. [DOI] [PMC free article] [PubMed] [Google Scholar]
- Armstrong B. D., Harvey R. J. Responses in the inferior olive to stimulation of the cerebellar and cerebral cortices in the cat. J Physiol. 1966 Dec;187(3):553–574. doi: 10.1113/jphysiol.1966.sp008108. [DOI] [PMC free article] [PubMed] [Google Scholar]
- Armstrong D. M., Eccles J. C., Harvey R. J., Matthews P. B. Responses in the dorsal accessory olive of the cat to stimulation of hind limb afferents. J Physiol. 1968 Jan;194(1):125–145. doi: 10.1113/jphysiol.1968.sp008398. [DOI] [PMC free article] [PubMed] [Google Scholar]
- Armstrong D. M., Rawson J. A. Activity patterns of cerebellar cortical neurones and climbing fibre afferents in the awake cat. J Physiol. 1979 Apr;289:425–448. doi: 10.1113/jphysiol.1979.sp012745. [DOI] [PMC free article] [PubMed] [Google Scholar]
- Crill W. E. Unitary multiple-spiked responses in cat inferior olive nucleus. J Neurophysiol. 1970 Mar;33(2):199–209. doi: 10.1152/jn.1970.33.2.199. [DOI] [PubMed] [Google Scholar]
- Duggan A. W., Lodge D., Headley P. M., Biscoe T. J. Effects of excitants on neurones and cerebellar-evoked field potentials in the inferior olivary complex of the rat. Brain Res. 1973 Dec 21;64:397–401. doi: 10.1016/0006-8993(73)90194-7. [DOI] [PubMed] [Google Scholar]
- Eccles J. C., Llinás R., Sasaki K. The excitatory synaptic action of climbing fibres on the Purkinje cells of the cerebellum. J Physiol. 1966 Jan;182(2):268–296. doi: 10.1113/jphysiol.1966.sp007824. [DOI] [PMC free article] [PubMed] [Google Scholar]
- Eckert R., Lux H. D. A voltage-sensitive persistent calcium conductance in neuronal somata of Helix. J Physiol. 1976 Jan;254(1):129–151. doi: 10.1113/jphysiol.1976.sp011225. [DOI] [PMC free article] [PubMed] [Google Scholar]
- Hagiwara S. Ca spike. Adv Biophys. 1973;4:71–102. [PubMed] [Google Scholar]
- Hagiwara S., Fukuda J., Eaton D. C. Membrane currents carried by Ca, Sr, and Ba in barnacle muscle fiber during voltage clamp. J Gen Physiol. 1974 May;63(5):564–578. doi: 10.1085/jgp.63.5.564. [DOI] [PMC free article] [PubMed] [Google Scholar]
- Headley P. M., Lodge D. Studies on field potentials and on single cells in the inferior olivary complex of the rat. Brain Res. 1976 Jan 23;101(3):445–459. doi: 10.1016/0006-8993(76)90470-4. [DOI] [PubMed] [Google Scholar]
- Llinas R., Baker R., Sotelo C. Electrotonic coupling between neurons in cat inferior olive. J Neurophysiol. 1974 May;37(3):560–571. doi: 10.1152/jn.1974.37.3.560. [DOI] [PubMed] [Google Scholar]
- Llinás R., Sugimori M. Electrophysiological properties of in vitro Purkinje cell dendrites in mammalian cerebellar slices. J Physiol. 1980 Aug;305:197–213. doi: 10.1113/jphysiol.1980.sp013358. [DOI] [PMC free article] [PubMed] [Google Scholar]
- Llinás R., Sugimori M. Electrophysiological properties of in vitro Purkinje cell somata in mammalian cerebellar slices. J Physiol. 1980 Aug;305:171–195. doi: 10.1113/jphysiol.1980.sp013357. [DOI] [PMC free article] [PubMed] [Google Scholar]
- Llinás R., Volkind R. A. The olivo-cerebellar system: functional properties as revealed by harmaline-induced tremor. Exp Brain Res. 1973 Aug 31;18(1):69–87. doi: 10.1007/BF00236557. [DOI] [PubMed] [Google Scholar]
- Llinás R., Yarom Y. Electrophysiology of mammalian inferior olivary neurones in vitro. Different types of voltage-dependent ionic conductances. J Physiol. 1981 Jun;315:549–567. doi: 10.1113/jphysiol.1981.sp013763. [DOI] [PMC free article] [PubMed] [Google Scholar]
- Rudy B. Slow inactivation of the sodium conductance in squid giant axons. Pronase resistance. J Physiol. 1978 Oct;283:1–21. doi: 10.1113/jphysiol.1978.sp012485. [DOI] [PMC free article] [PubMed] [Google Scholar]
- Simpson J. I., Alley K. E. Visual climbing fiber input to rabbit vestibulo-cerebellum: a source of direction-specific information. Brain Res. 1974 Dec 27;82(2):302–308. doi: 10.1016/0006-8993(74)90610-6. [DOI] [PubMed] [Google Scholar]
- WERMAN R., GRUNDFEST H. Graded and all-or-none electrogenesis in arthropod muscle. II. The effects of alkali-earth and onium ions on lobster muscle fibers. J Gen Physiol. 1961 May;44:997–1027. doi: 10.1085/jgp.44.5.997. [DOI] [PMC free article] [PubMed] [Google Scholar]
- Wong R. K., Prince D. A., Basbaum A. I. Intradendritic recordings from hippocampal neurons. Proc Natl Acad Sci U S A. 1979 Feb;76(2):986–990. doi: 10.1073/pnas.76.2.986. [DOI] [PMC free article] [PubMed] [Google Scholar]
- de Montigny C., Lamarre Y. Rhythmic activity induced by harmaline in the olivo-cerebello-bulbar system of the cat. Brain Res. 1973 Apr 13;53(1):81–95. doi: 10.1016/0006-8993(73)90768-3. [DOI] [PubMed] [Google Scholar]