Abstract
1. Simultaneous measurements were made in anaesthetized dogs of monophasic action potentials from the right ventricle and of the maximum rate of rise of left ventricular pressure (dPlv/dtmax). Atrio-ventricular dissociation was induced and the heart paced via right ventricular electrodes.
2. A control period of steady pacing was followed by a test stimulus after a variable interval called the `test-pulse interval'. The duration of the action potential of the test beat (measured at 70% repolarization) increased with test-pulse interval and reached an approximately steady value at intervals of 1·0-1·5 sec. This constitutes the `electrical restitution curve'.
3. An increase in the frequency of stimulation prior to the introduction of the test pulses caused a downward displacement of the electrical restitution curve.
4. Stimulation at 2 Hz and paired pulse stimulation at 1 Hz (same number of stimuli per min) were introduced prior to the test pulses and produced very similar electrical restitution curves.
5. For a constant frequency of stimulation in the control period, adrenaline produced downward displacement of the restitution curve.
6. It is concluded that there is no obvious relationship between the restitution of the action potential duration and of the contractile response. We suggest therefore that electrical and mechanical restitution occur through separate processes, the former through time-dependent recovery in membrane conductances and the latter through time-dependent increase in availability of intracellular calcium for release.
7. Contractions were introduced with a test-pulse interval shorter than the optimum, and were followed by a second test pulse fixed at the optimum interval of 0·8-1·0 sec. The second test beats were potentiated (post-extrasystolic potentiation). In isolated ejecting cat hearts, there was an optimum interval for the first test pulse to produce the greatest potentiation of the second test beat. This interval was 0·2-0·3 sec, and was shortened by an increase in frequency of stimulation prior to the first test beat.
8. The interval preceding the first test pulse was then varied within a range (0·8-2·0 sec) which did not produce potentiation. These first test pulses were sometimes preceded by one extrasystole. The timing of this extrasystole was altered to vary the post-extrastolic potentiation of the first test pulse.
9. Multiple regression analysis, carried out between dPlv/dtmax of the second test pulse (DP2, the dependent variable) and the action potential duration (AP1) and dPlv/dtmax (DP1) of the first test pulse (independent variables) yielded correlation coefficients between 0·88 and 0·99. Each determination of the coefficient included data from beats with and without post-extrasystolic potentiation.
10. It is postulated that the coefficient relating DP2 to DP1 in the multiple regression analysis (mean value 0·75) is an index of the proportion of calcium stored during relaxation which is released again on the next beat.
11. When the decay of post-extrasystolic potentiation was examined in consecutive beats at the optimum interval, the action potential durations of these beats were found to be nearly constant. A plot of dPlv/dtmax of each beat against dPlv/dtmax of the previous beat yielded a curvilinear relationship which was less steep than that relating DP2 to DP1 in the two test pulse analysis; this was attributed to inconstancy of calcium ion entry during the action potential.
Full text
PDF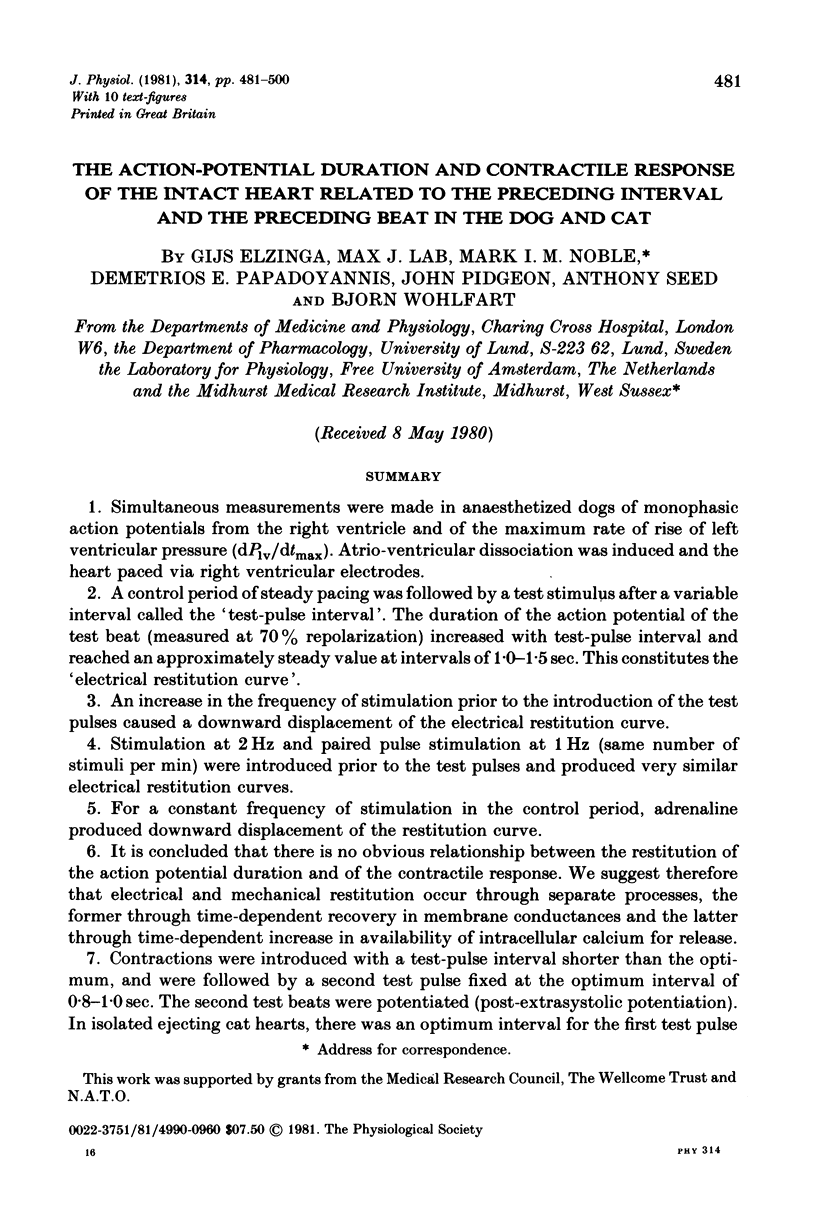
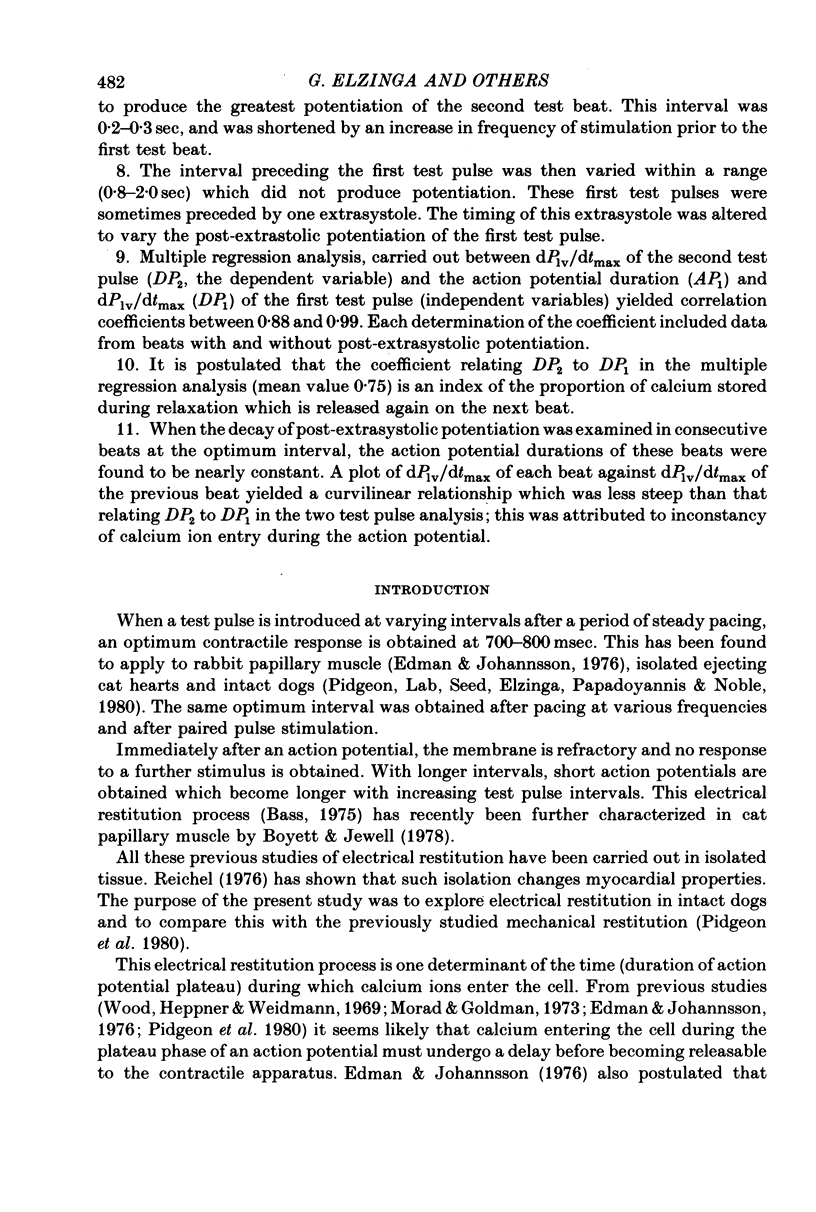
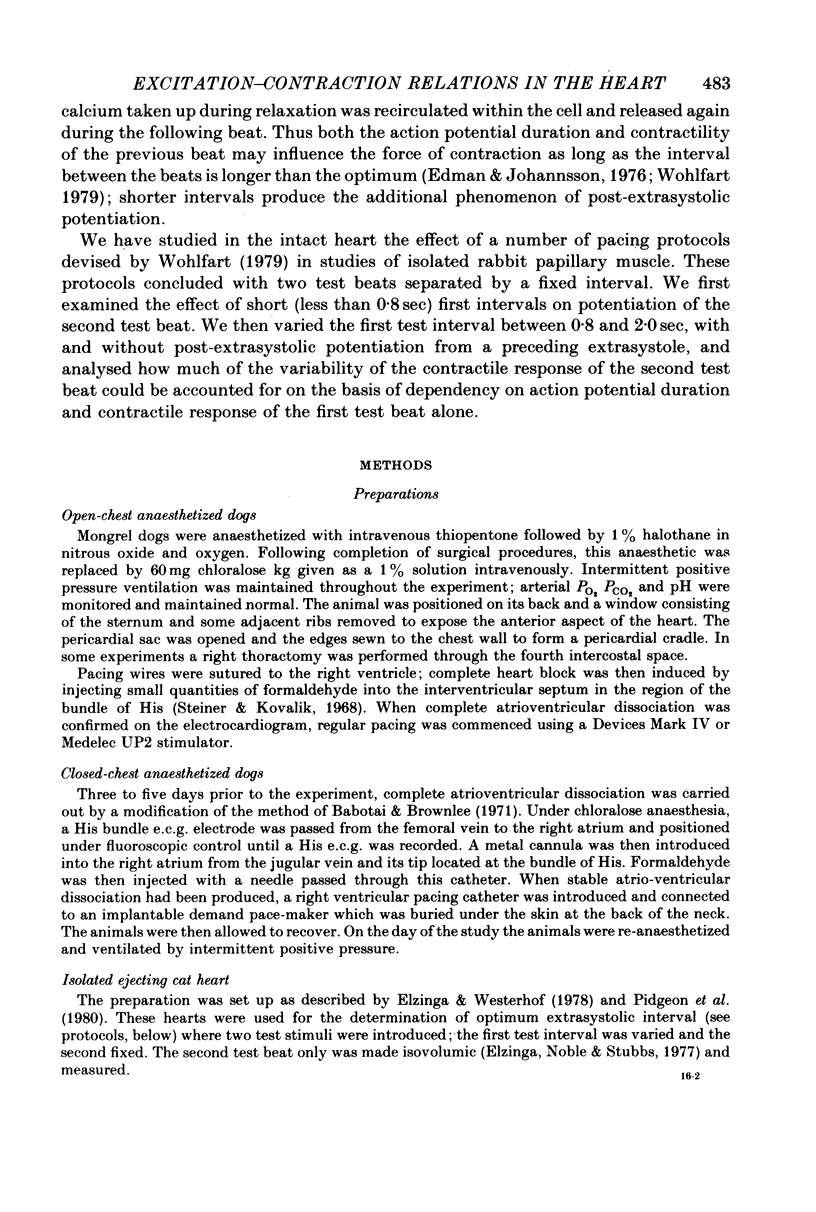
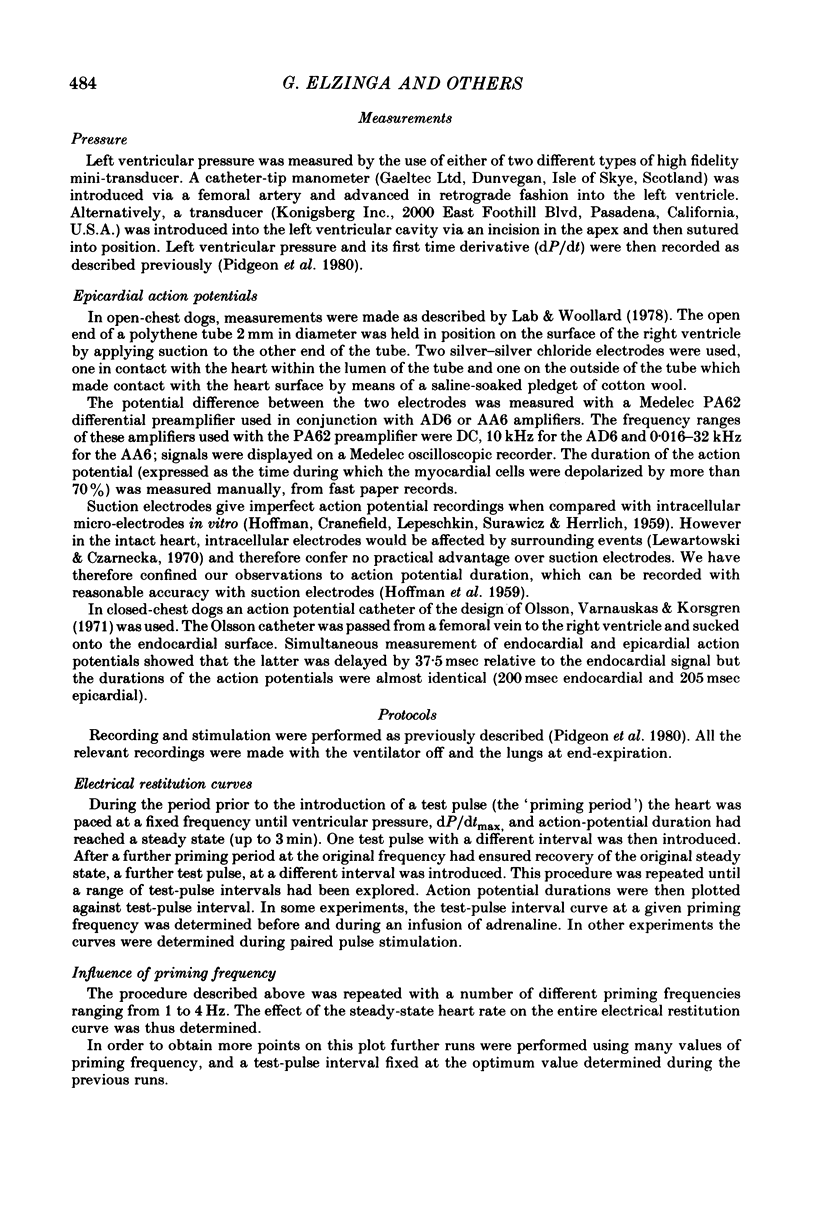
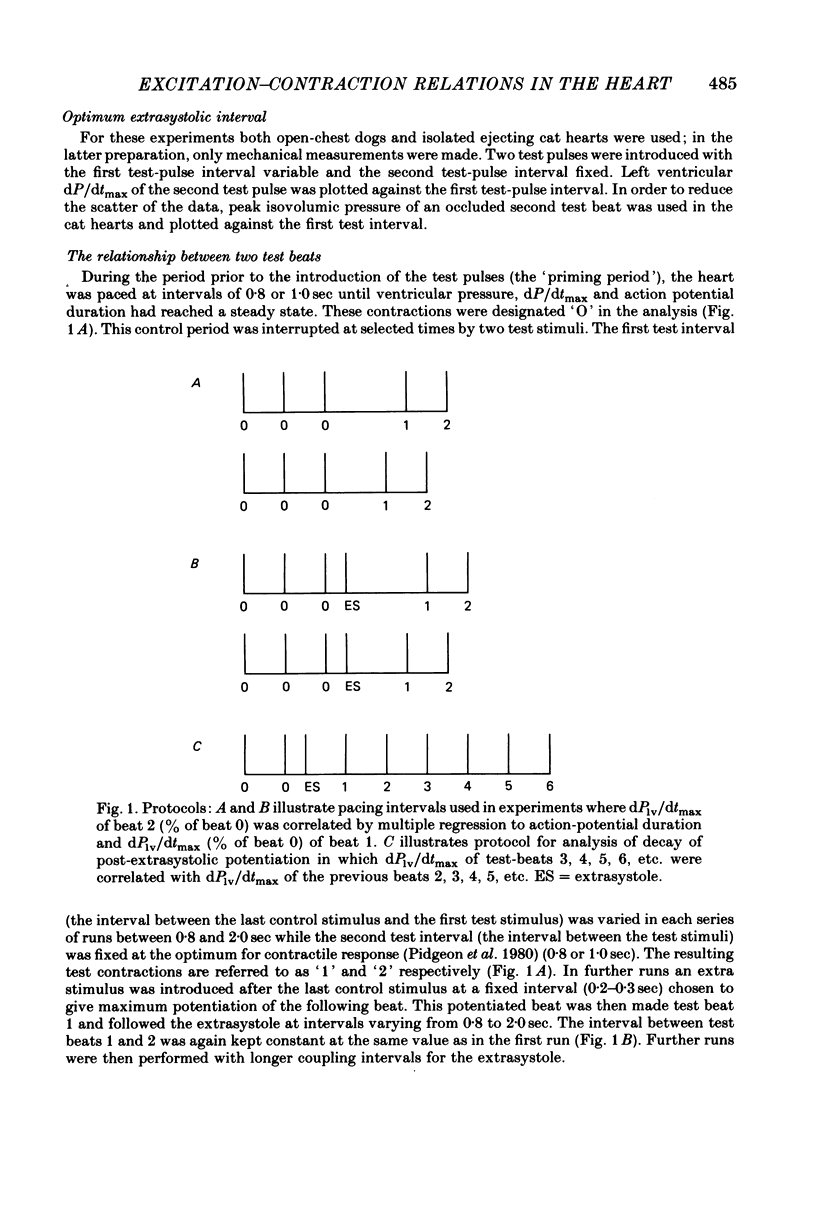
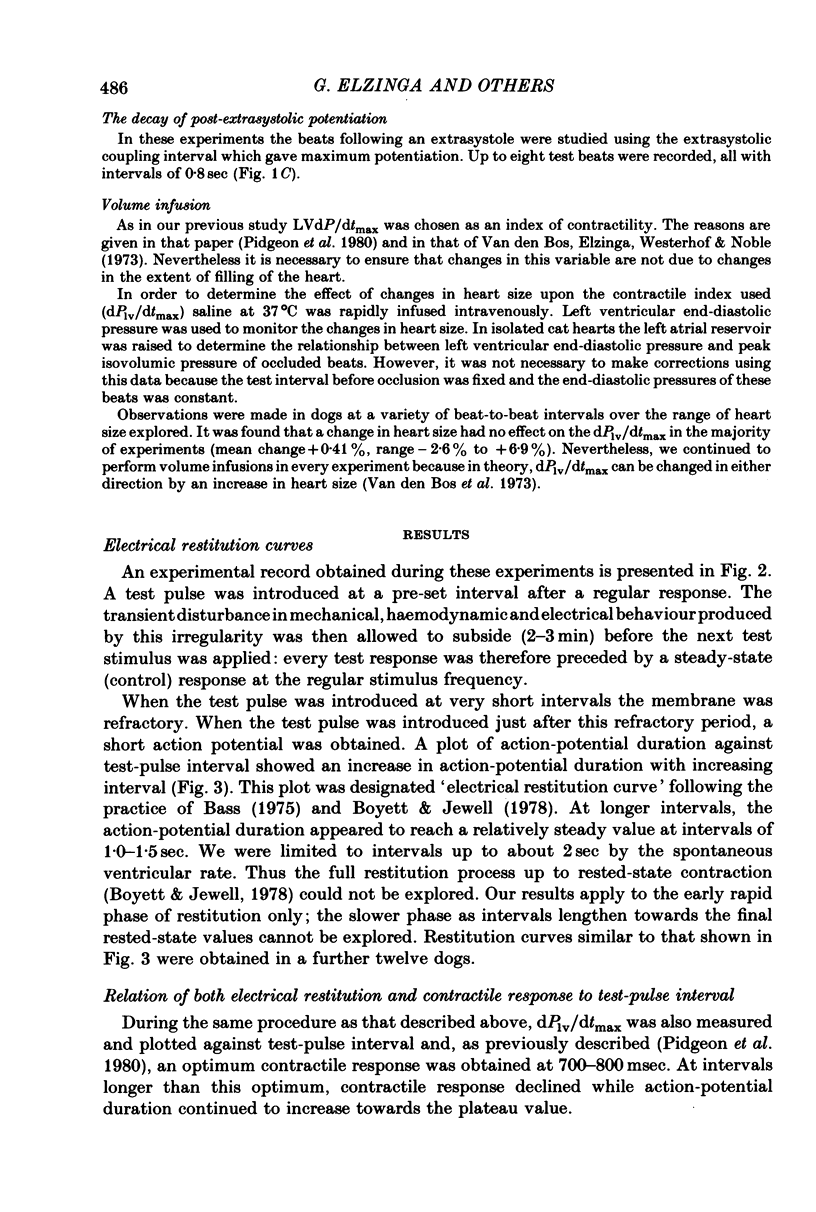
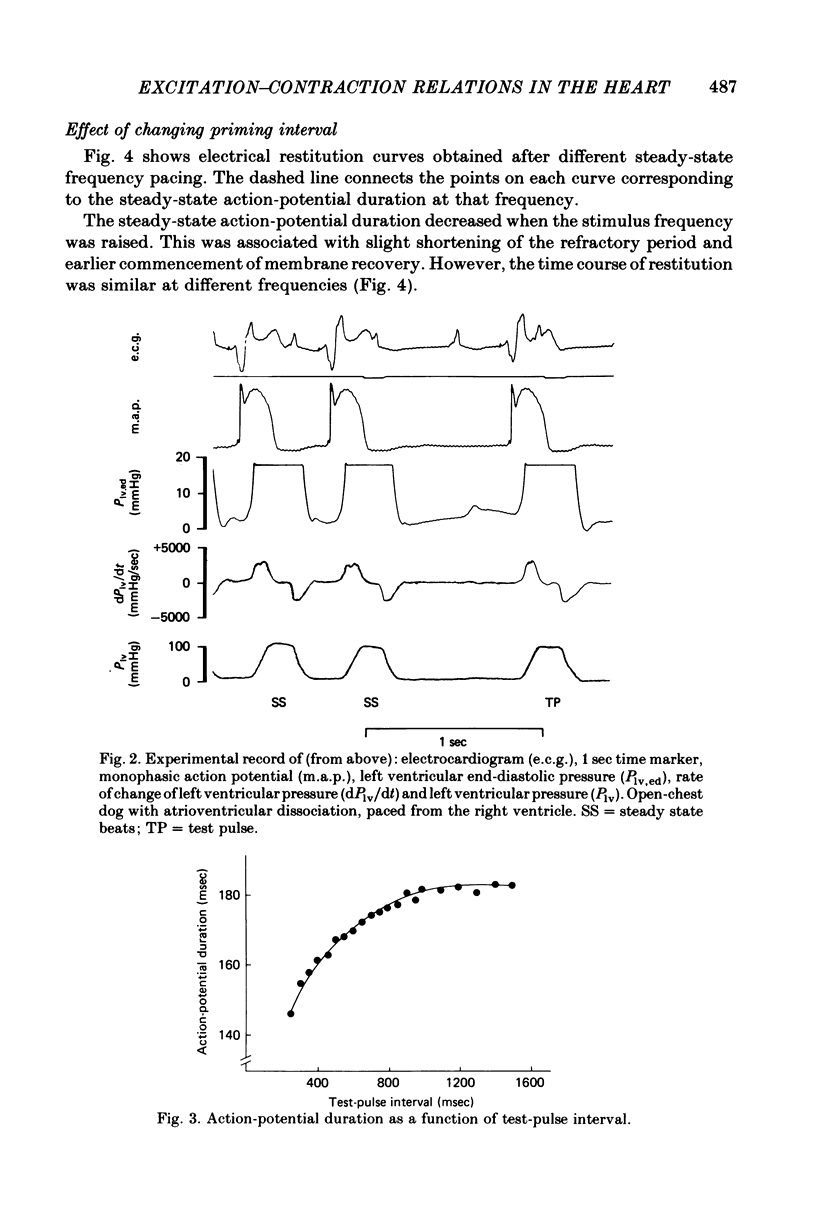
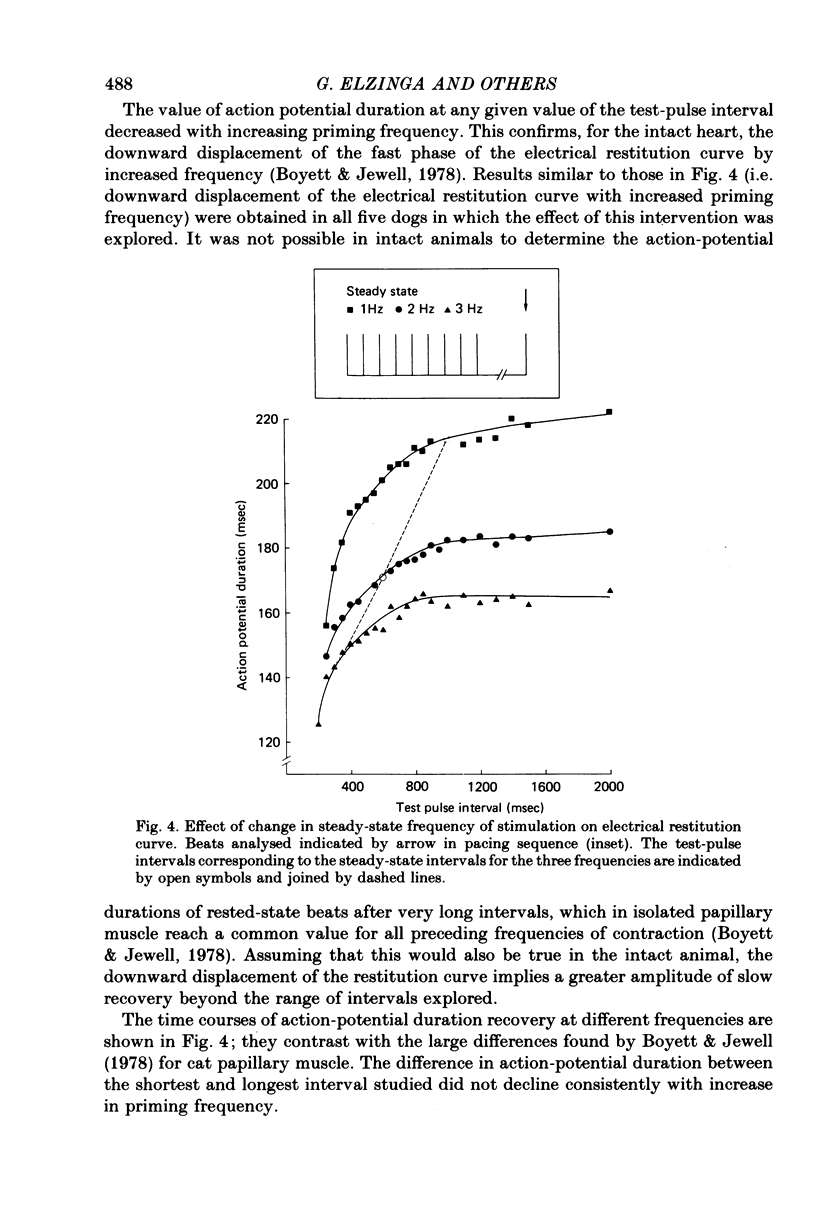
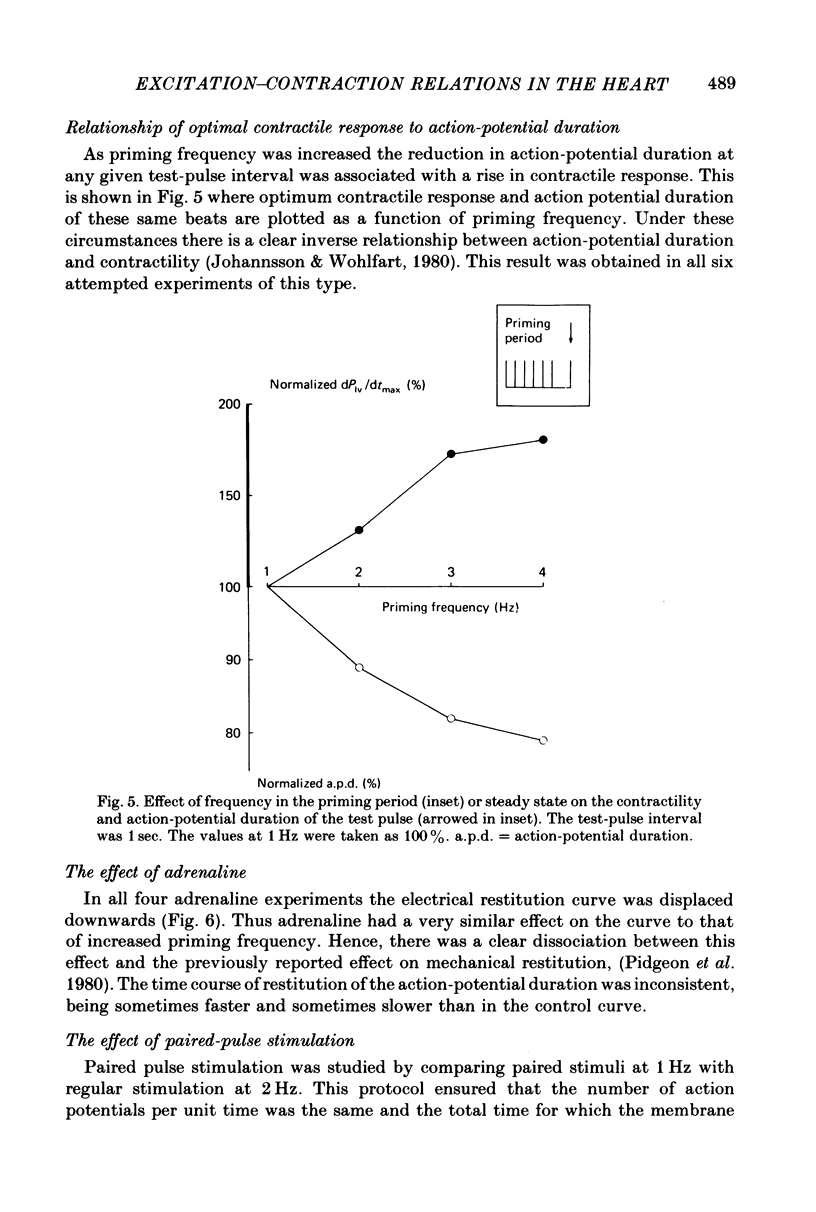
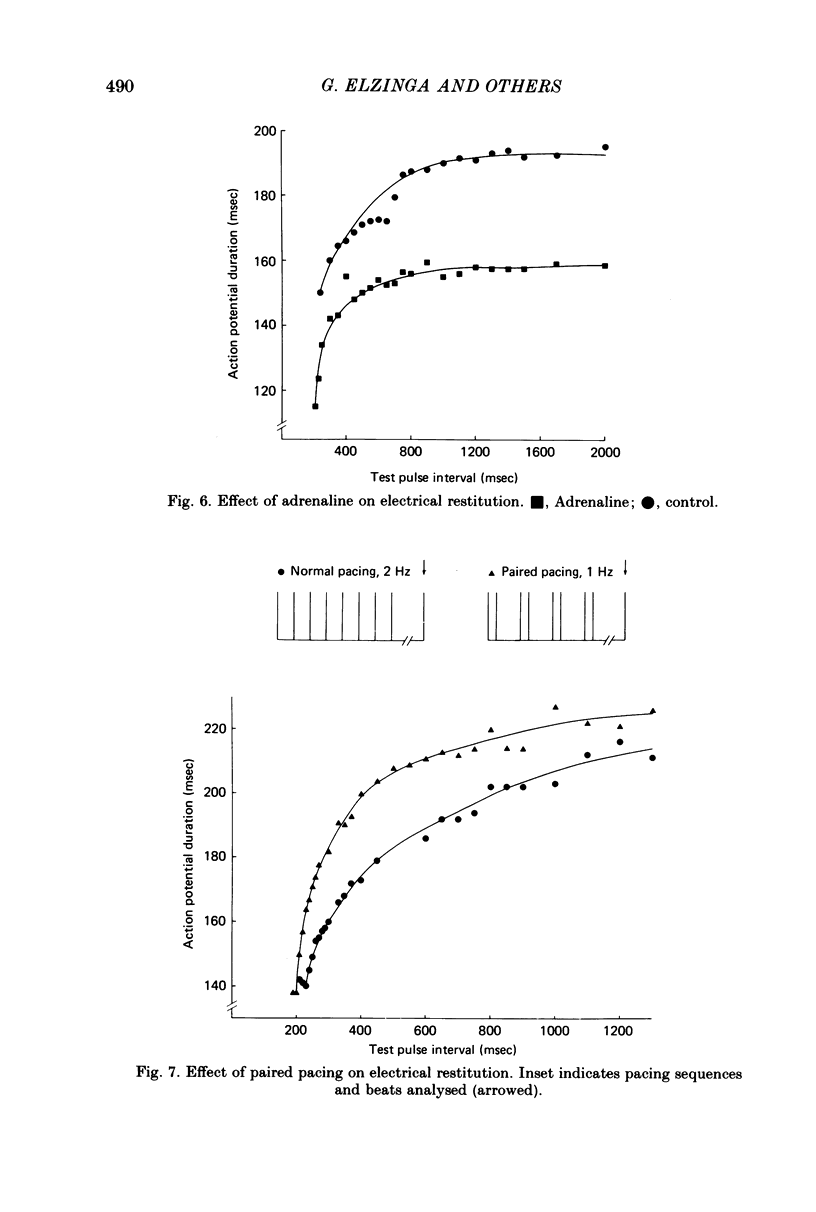
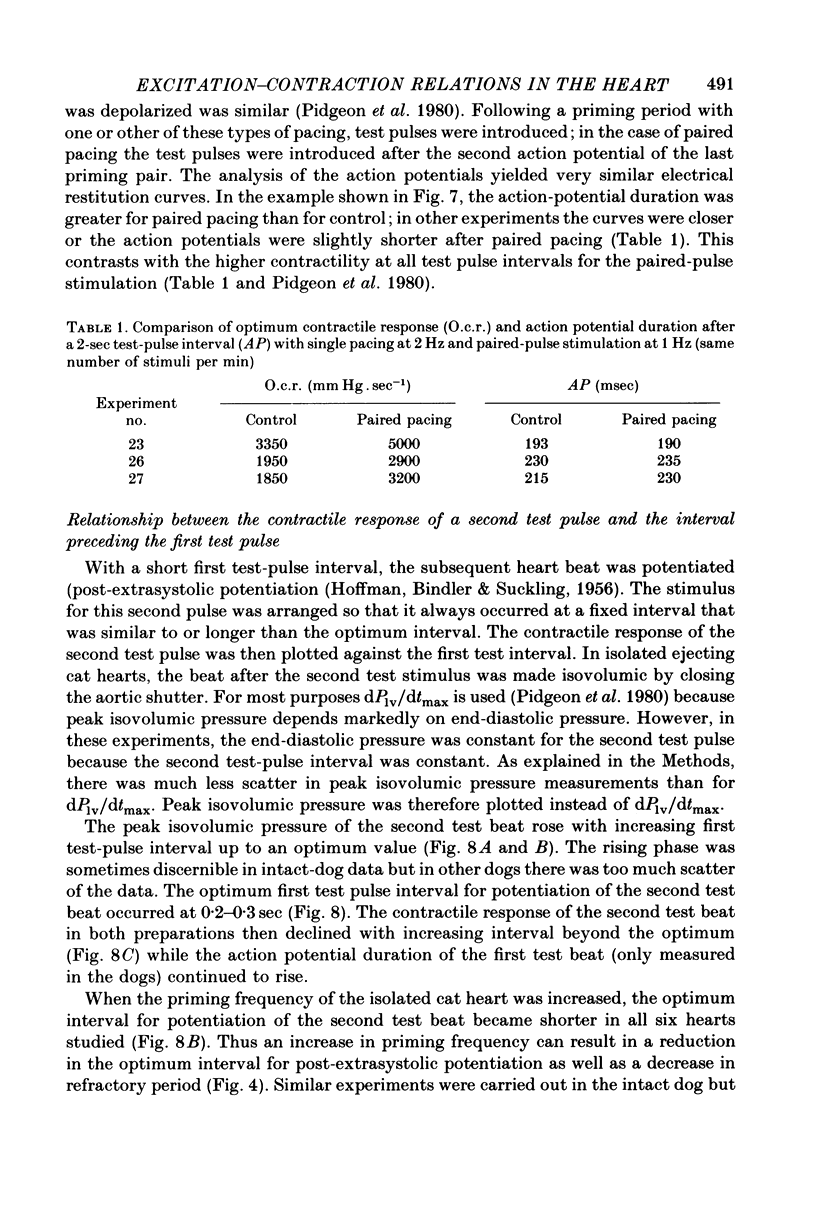
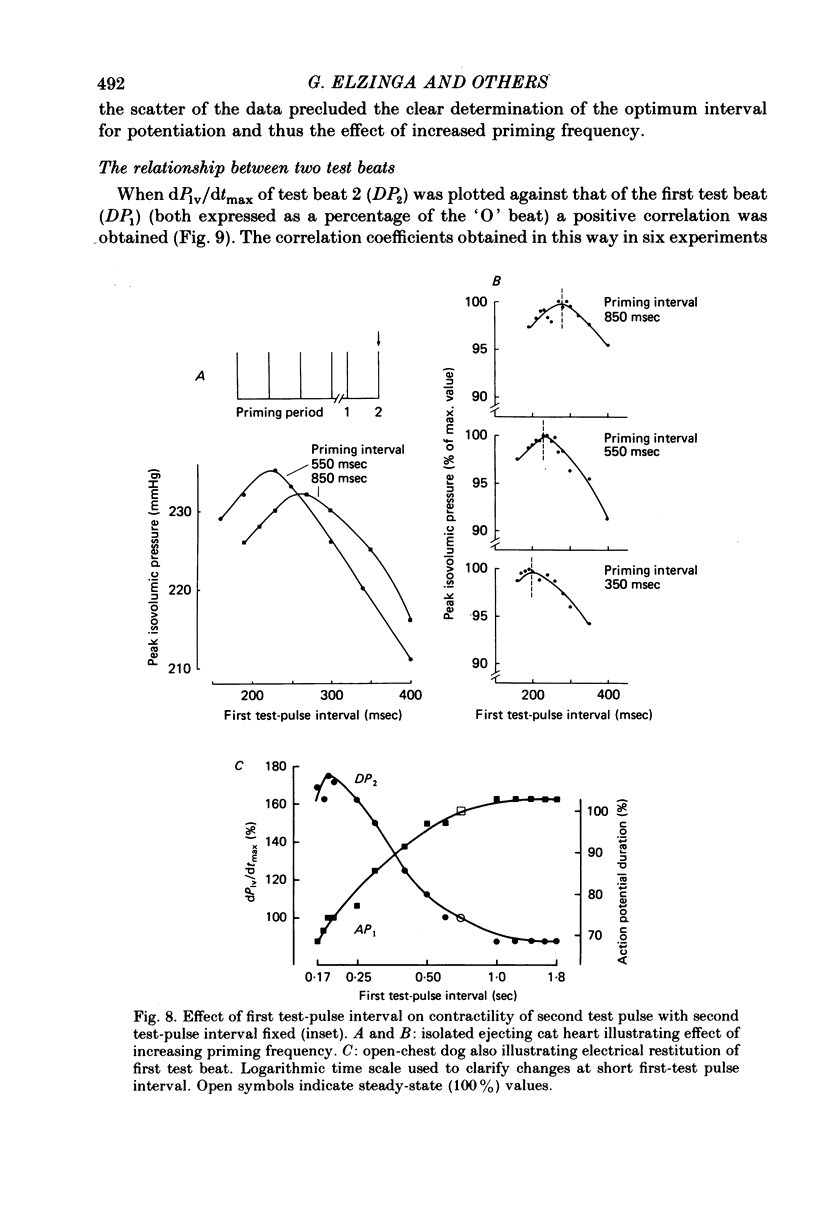
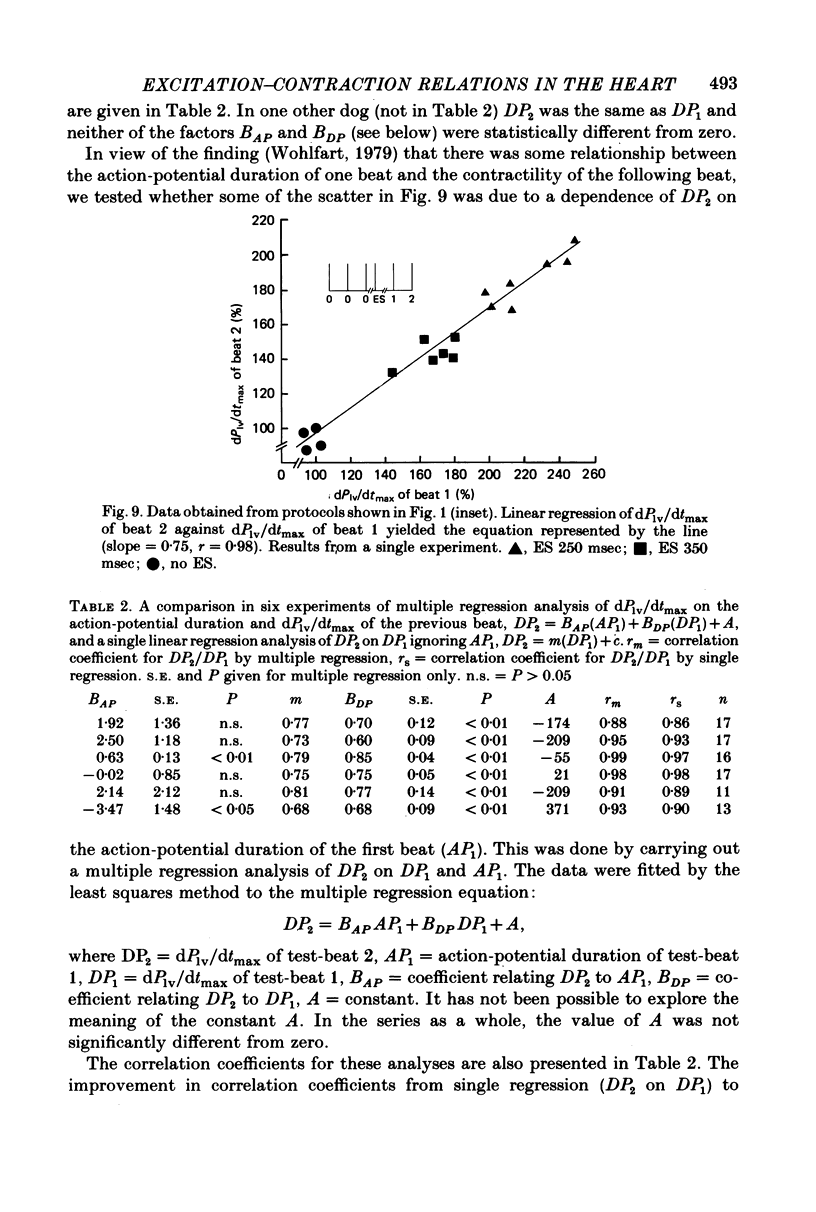
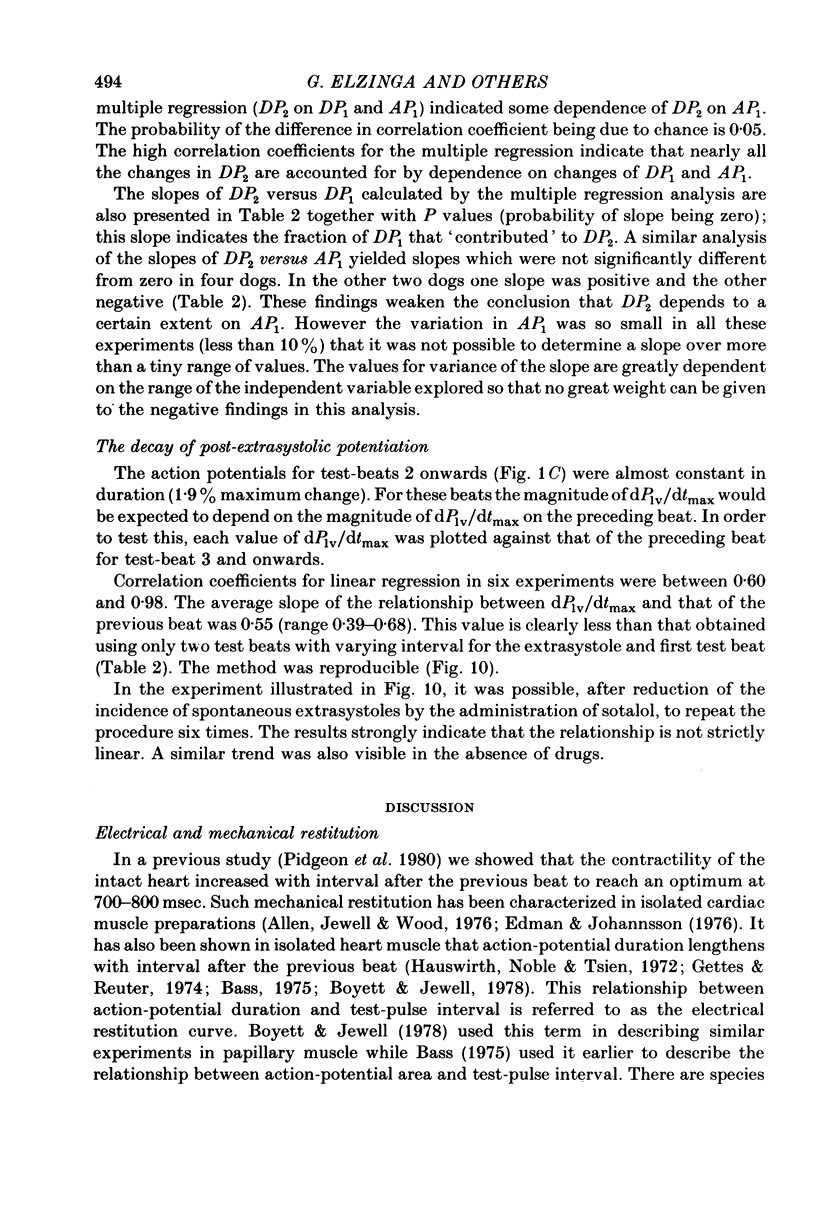
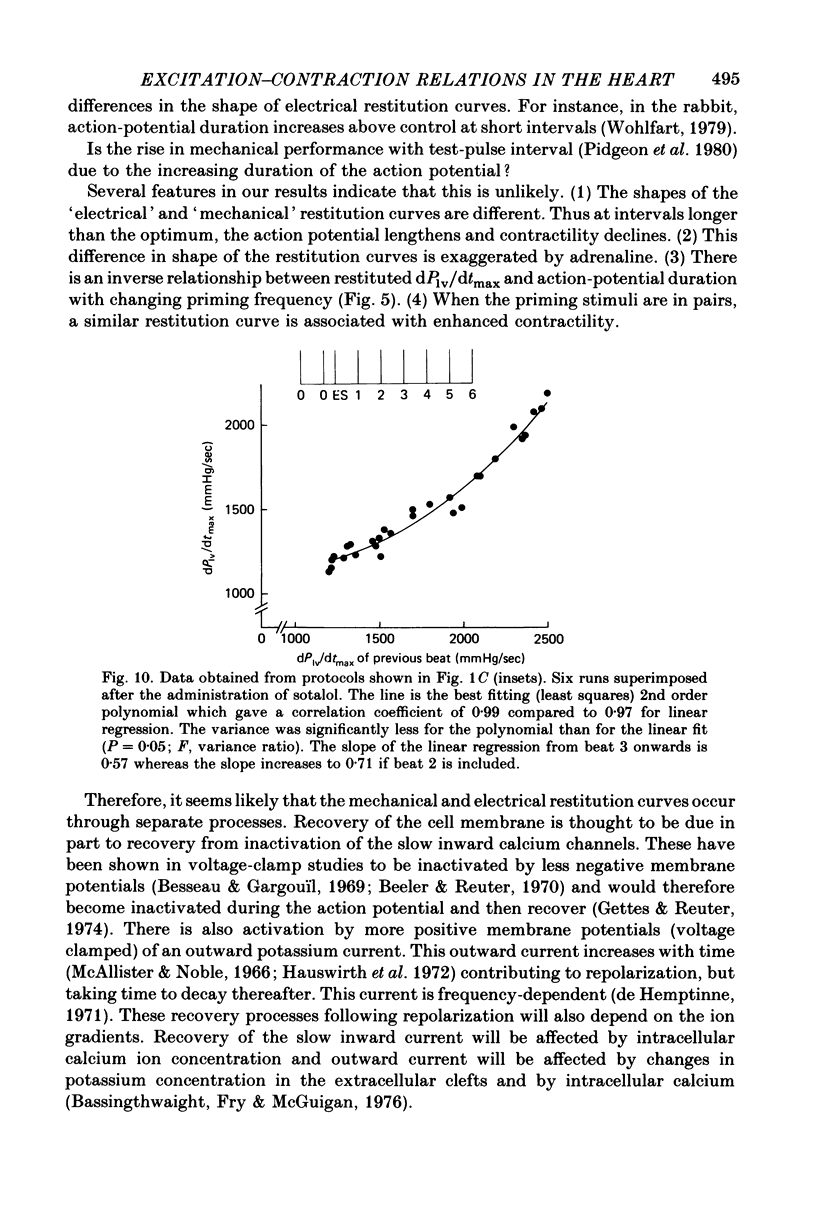
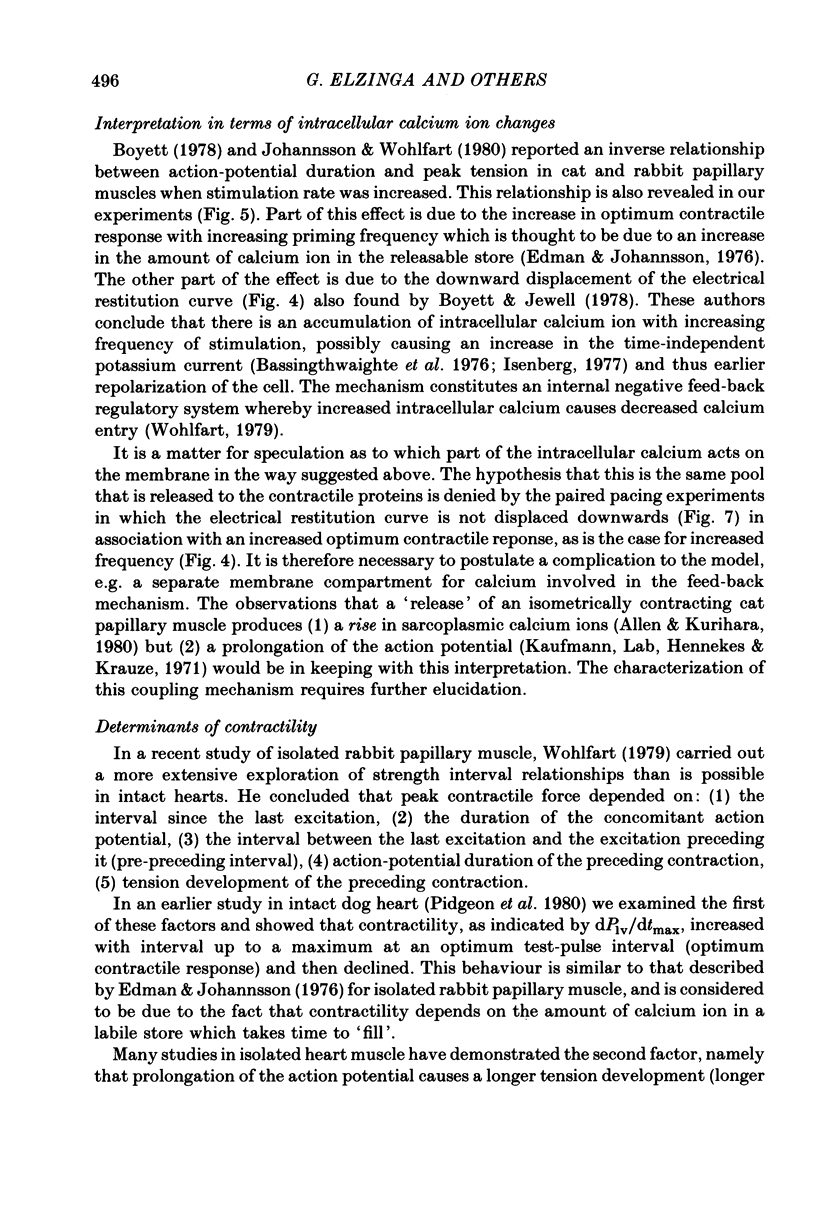
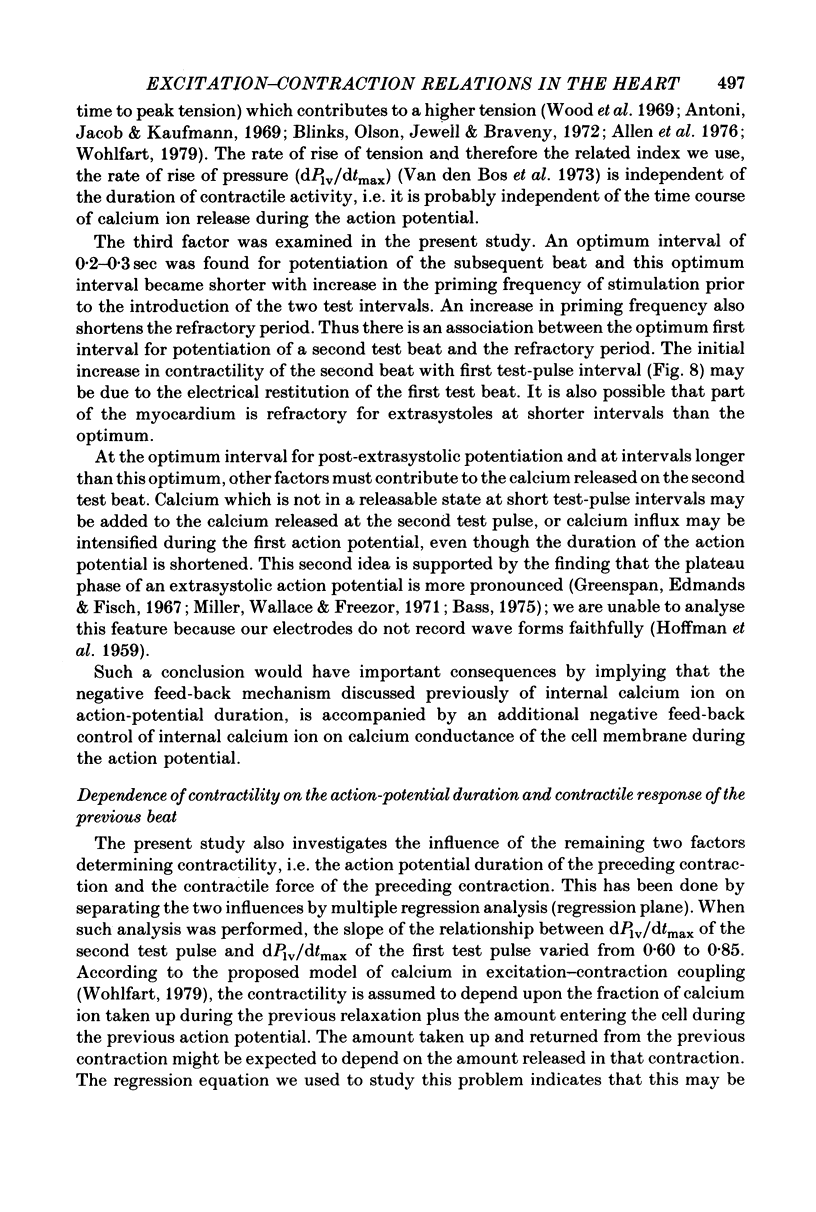
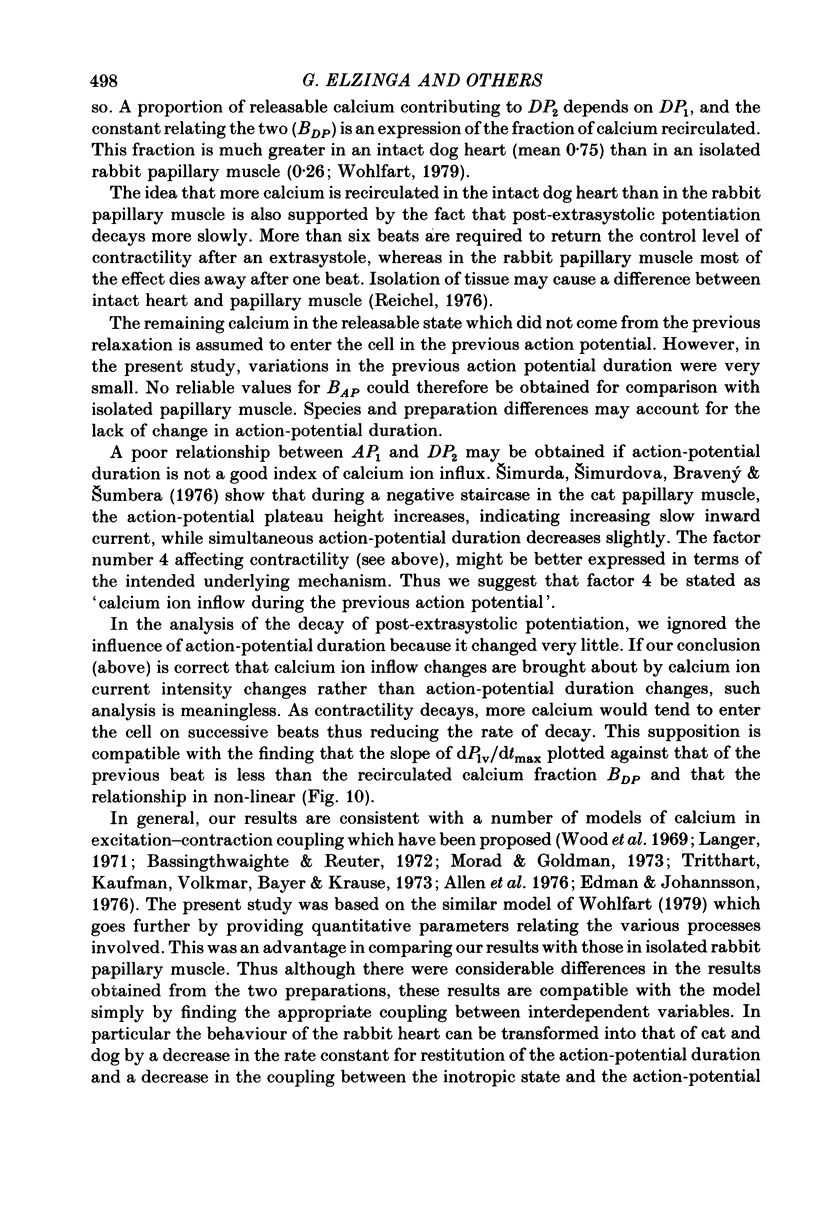
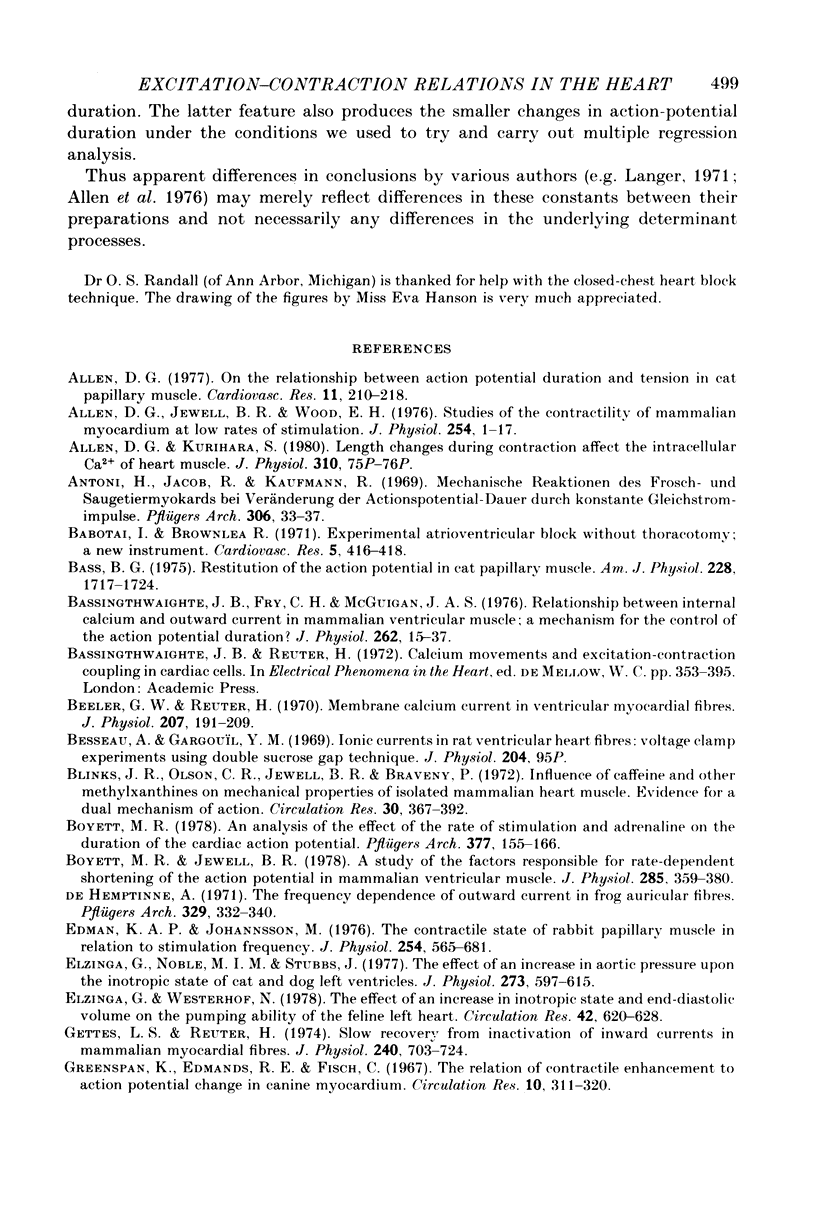
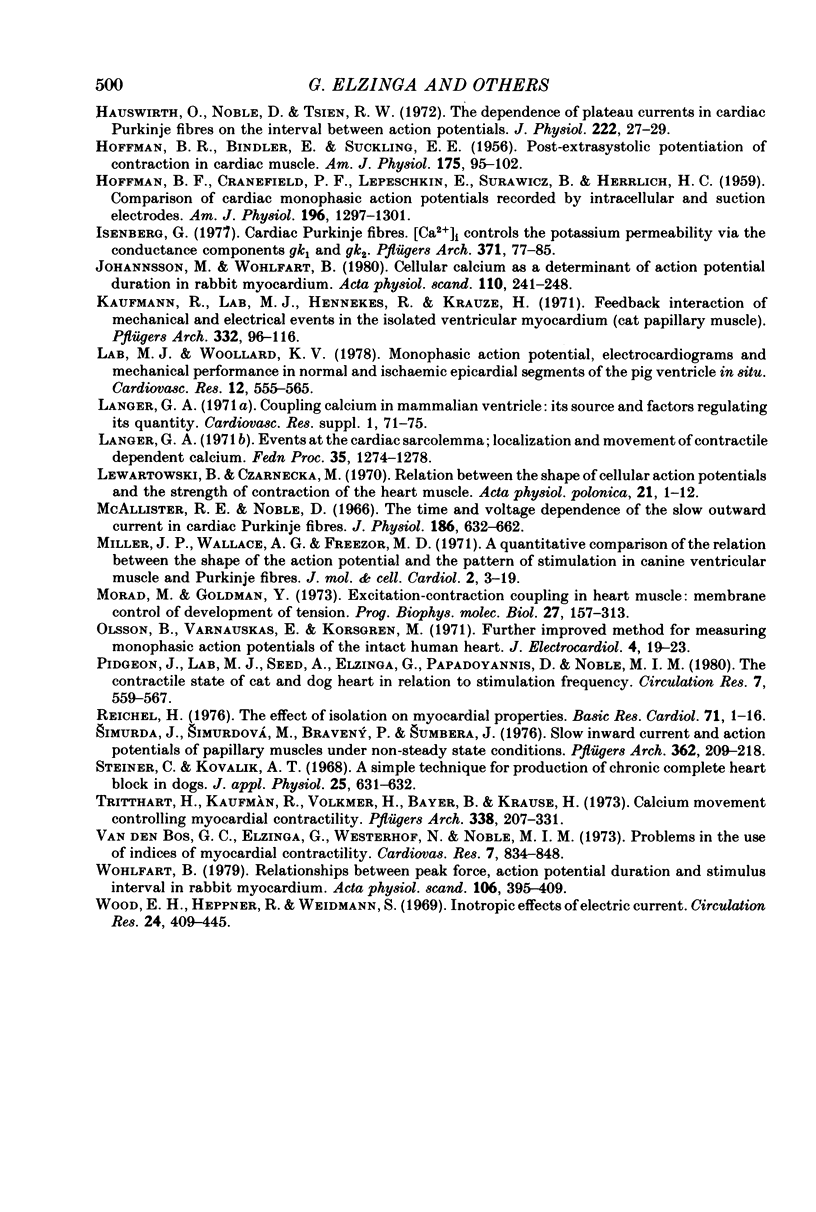
Selected References
These references are in PubMed. This may not be the complete list of references from this article.
- Allen D. G., Jewell B. R., Wood E. H. Studies of the contractility of mammalian myocardium at low rates of stimulation. J Physiol. 1976 Jan;254(1):1–17. doi: 10.1113/jphysiol.1976.sp011217. [DOI] [PMC free article] [PubMed] [Google Scholar]
- Allen D. G. On the relationship between action potential duration and tension in cat papillary muscle. Cardiovasc Res. 1977 May;11(3):210–218. doi: 10.1093/cvr/11.3.210. [DOI] [PubMed] [Google Scholar]
- Antoni H., Jacob R., Kaufmann R. Mechanische Reaktionen des Frosch- und Säugetiermyokards bei Veränderung der Aktionspotential-Dauer durch konstante Gleichstromimpulse. Pflugers Arch. 1969;306(1):33–57. doi: 10.1007/BF00586610. [DOI] [PubMed] [Google Scholar]
- Babotai I., Brownlee R. Experimental atrioventricular block without thoracotomy: a new instrument. Cardiovasc Res. 1971 Jul;5(3):416–418. doi: 10.1093/cvr/5.3.416. [DOI] [PubMed] [Google Scholar]
- Bass B. G. Restitution of the action potential in cat papillary muscle. Am J Physiol. 1975 Jun;228(6):1717–1724. doi: 10.1152/ajplegacy.1975.228.6.1717. [DOI] [PubMed] [Google Scholar]
- Bassingthwaighte J. B., Fry C. H., McGuigan J. A. Relationship between internal calcium and outward current in mammalian ventricular muscle; a mechanism for the control of the action potential duration? J Physiol. 1976 Oct;262(1):15–37. doi: 10.1113/jphysiol.1976.sp011583. [DOI] [PMC free article] [PubMed] [Google Scholar]
- Beeler G. W., Jr, Reuter H. Membrane calcium current in ventricular myocardial fibres. J Physiol. 1970 Mar;207(1):191–209. doi: 10.1113/jphysiol.1970.sp009056. [DOI] [PMC free article] [PubMed] [Google Scholar]
- Blinks J. R., Olson C. B., Jewell B. R., Bravený P. Influence of caffeine and other methylxanthines on mechanical properties of isolated mammalian heart muscle. Evidence for a dual mechanism of action. Circ Res. 1972 Apr;30(4):367–392. doi: 10.1161/01.res.30.4.367. [DOI] [PubMed] [Google Scholar]
- Boyett M. R. An analysis of the effect of the rate of stimulation and adrenaline on the duration of the cardiac action potential. Pflugers Arch. 1978 Nov 14;377(2):155–166. doi: 10.1007/BF00582846. [DOI] [PubMed] [Google Scholar]
- Boyett M. R., Jewell B. R. A study of the factors responsible for rate-dependent shortening of the action potential in mammalian ventricular muscle. J Physiol. 1978 Dec;285:359–380. doi: 10.1113/jphysiol.1978.sp012576. [DOI] [PMC free article] [PubMed] [Google Scholar]
- De Hemptinne A. The frequency dependence of outward current in frog auricular fibres. An experimental and theoretical study. Pflugers Arch. 1971;329(4):332–340. doi: 10.1007/BF00588004. [DOI] [PubMed] [Google Scholar]
- Edman K. A., Jóhannsson M. The contractile state of rabbit papillary muscle in relation to stimulation frequency. J Physiol. 1976 Jan;254(3):565–581. doi: 10.1113/jphysiol.1976.sp011247. [DOI] [PMC free article] [PubMed] [Google Scholar]
- Elzinga G., Noble M. I., Stubbs J. The effect of an increase in aortic pressure upon the inotropic state of cat and dog left ventricles. J Physiol. 1977 Dec;273(3):597–615. doi: 10.1113/jphysiol.1977.sp012112. [DOI] [PMC free article] [PubMed] [Google Scholar]
- Elzinga G., Westerhof N. The effect of an increase in inotropic state and end-diastolic volume on the pumping ability of the feline left heart. Circ Res. 1978 May;42(5):620–628. doi: 10.1161/01.res.42.5.620. [DOI] [PubMed] [Google Scholar]
- Gettes L. S., Reuter H. Slow recovery from inactivation of inward currents in mammalian myocardial fibres. J Physiol. 1974 Aug;240(3):703–724. doi: 10.1113/jphysiol.1974.sp010630. [DOI] [PMC free article] [PubMed] [Google Scholar]
- Greenspan K., Edmonds R. E., Fisch C. The relation of contractile enhancement to action potential change in canine myocardium. Circ Res. 1967 Mar;20(3):311–320. doi: 10.1161/01.res.20.3.311. [DOI] [PubMed] [Google Scholar]
- HOFFMAN B. F., BINDLER E., SUCKLING E. E. Postextrasystolic potentiation of contraction in cardiac muscle. Am J Physiol. 1956 Apr;185(1):95–102. doi: 10.1152/ajplegacy.1956.185.1.95. [DOI] [PubMed] [Google Scholar]
- HOFFMAN B. F., CRANEFIELD P. F., LEPESCHKIN E., SURAWICZ B., HERRLICH H. C. Comparison of cardiac monophasic action potentials recorded by intracellular and suction electrodes. Am J Physiol. 1959 Jun;196(6):1297–1301. doi: 10.1152/ajplegacy.1959.196.6.1297. [DOI] [PubMed] [Google Scholar]
- Hausworth O., Noble D., Tsien R. W. The dependence of plateau currents in cardiac Purkinje fibres on the interval between action potentials. J Physiol. 1972 Apr;222(1):27–49. doi: 10.1113/jphysiol.1972.sp009786. [DOI] [PMC free article] [PubMed] [Google Scholar]
- Isenberg G. Cardiac Purkinje fibres: [Ca2+]i controls the potassium permeability via the conductance components gK1 and gK2. Pflugers Arch. 1977 Oct 19;371(1-2):77–85. doi: 10.1007/BF00580775. [DOI] [PubMed] [Google Scholar]
- Jóhannsson M., Wohlfart B. Cellular calcium as a determinant of action potential duration in rabbit myocardium. Acta Physiol Scand. 1980 Nov;110(3):241–247. doi: 10.1111/j.1748-1716.1980.tb06660.x. [DOI] [PubMed] [Google Scholar]
- Lab M. J., Woollard K. V. Monophasic action potentials, electrocardiograms and mechanical performance in normal and ischaemic epicardial segments of the pig ventricle in situ. Cardiovasc Res. 1978 Sep;12(9):555–565. doi: 10.1093/cvr/12.9.555. [DOI] [PubMed] [Google Scholar]
- Langer G. A. Coupling calcium in mammalian ventricle: its source and factors regulating its quantity. Cardiovasc Res. 1971 Jul;Suppl 1:71–75. doi: 10.1093/cvr/5.supp1.71. [DOI] [PubMed] [Google Scholar]
- Langer G. A. Events at the cardiac sarcolemma: localization and movement of contractile-dependent calcium. Fed Proc. 1976 May 1;35(6):1274–1278. [PubMed] [Google Scholar]
- McAllister R. E., Noble D. The time and voltage dependence of the slow outward current in cardiac Purkinje fibres. J Physiol. 1966 Oct;186(3):632–662. doi: 10.1113/jphysiol.1966.sp008060. [DOI] [PMC free article] [PubMed] [Google Scholar]
- Miller J. P., Wallace A. G., Feezor M. D. A quantitative comparison of the relation between the shape of the action potential and the pattern of stimulation in canine ventricular muscle and Purkinje fibers. J Mol Cell Cardiol. 1971 Mar;2(1):3–19. doi: 10.1016/0022-2828(71)90074-5. [DOI] [PubMed] [Google Scholar]
- Olsson B., Varnauskas E., Korsgren M. Further improved method for measuring monophasic action potentials of the intact human heart. J Electrocardiol. 1971;4(1):19–23. doi: 10.1016/s0022-0736(71)80045-6. [DOI] [PubMed] [Google Scholar]
- Pidgeon J., Lab M., Seed A., Elzinga G., Papadoyannis D., Noble M. I. The contractile state of cat and dog heart in relation to the interval between beats. Circ Res. 1980 Oct;47(4):559–567. doi: 10.1161/01.res.47.4.559. [DOI] [PubMed] [Google Scholar]
- Reichel H. The effect of isolation on myocardial properties. Basic Res Cardiol. 1976 Jan-Feb;71(1):1–16. doi: 10.1007/BF01907778. [DOI] [PubMed] [Google Scholar]
- Simurda J., Simurdova M., Braveny P., Sumbera J. Slow inward current and action potentials of papillary muscles under non-steady state conditions. Pflugers Arch. 1976 Apr 6;362(3):209–218. doi: 10.1007/BF00581172. [DOI] [PubMed] [Google Scholar]
- Steiner C., Kovalik A. T. A simple technique for production of chronic complete heart block in dogs. J Appl Physiol. 1968 Nov;25(5):631–632. doi: 10.1152/jappl.1968.25.5.631. [DOI] [PubMed] [Google Scholar]
- Tritthart H., Kaufmann R., Volkmer H. P., Bayer R., Krause H. Ca-movement controlling myocardial contractility. I. Voltage-, current- and time-dependence of mechanical activity under voltage clamp conditions (cat papillary muscles and trabeculae). Pflugers Arch. 1973 Feb 6;338(3):207–231. doi: 10.1007/BF00587388. [DOI] [PubMed] [Google Scholar]
- Van den Bos G. C., Elzinga C., Westerhof N., Noble M. I. Problems in the use of indices of myocardial contractility. Cardiovasc Res. 1973 Nov;7(6):834–848. doi: 10.1093/cvr/7.6.834. [DOI] [PubMed] [Google Scholar]
- Wohlfart B. Relationships between peak force, action potential duration and stimulus interval in rabbit myocardium. Acta Physiol Scand. 1979 Aug;106(4):395–409. doi: 10.1111/j.1748-1716.1979.tb06419.x. [DOI] [PubMed] [Google Scholar]
- Wood E. H., Heppner R. L., Weidmann S. Inotropic effects of electric currents. I. Positive and negative effects of constant electric currents or current pulses applied during cardiac action potentials. II. Hypotheses: calcium movements, excitation-contraction coupling and inotropic effects. Circ Res. 1969 Mar;24(3):409–445. doi: 10.1161/01.res.24.3.409. [DOI] [PubMed] [Google Scholar]